Abstract
The present study aimed to evaluate how dietary supplementation with a microencapsulated sodium butyrate (500 mg/kg; 30% Na-butyrate) in 768 male and female broiler chickens affected growth performance, jejunum gut morphology and caecal microbiome at different ages (11, 24, and 35 d), slaughter results, meat quality, and myopathy (white striping, wooden breast, and spaghetti meat) occurrence. No health problem was recorded (mortality < 1%) and Na-butyrate supplementation did not affect performance throughout the trial, nor did it affect gut morphology or microbiota composition and abundance at different ages. Additionally, dietary treatment did not affect slaughter results, meat quality, or myopathy rate, whereas the rate of females affected by spaghetti meat tended to be higher in those fed the control compared with the Na-butyrate diet (33.3% versus 16.7%; p = .06). Moreover, males showed a higher wooden breast (21.9% versus 7.3%; p ≤ .01) and a lower spaghetti meat (3.1% versus 25.0%; p ≤ .001) rate compared with females. Finally, the richness index (i.e. the number of different species represented in an ecological community, landscape, or region) in the caecum increased (p ≤ .001) with increasing chicken age, and the community composition was also affected (adonis p ≤ .001). In conclusions, under the good health condition of the present trial, the tested Na-butyrate had no effect on performance and gut response at different ages of broiler chickens. Moreover, the occurrence of spaghetti meat and wooden breast differed between males and females.
Under optimal health conditions, Na-butyrate supplementation did not affect performance or gut response at different ages of broiler chickens.
Males showed a significantly higher occurrence of wooden breasts, whereas females had higher rates of spaghetti meat.
In females, the Na-butyrate supplementation tended to reduce the occurrence of spaghetti meat.
Highlights
Introduction
Recent trends in animal production mean that the maintenance of gut health under antibiotic-free rearing programs is now the most important challenge facing poultry producers (Cervantes Citation2015). As a consequence, research on feed additives has significantly expanded to include the evaluation of different products and their effects on health and immune responses, in addition to animal performance and product quality (Caly et al. Citation2015).
Compared with other available feed additives, short-chain fatty acids (SCFA) show major potential as an effective supplement under antibiotic-free conditions (Leeson et al. Citation2005). When used as a feed additive for broiler chickens, butyric acid has been found to have a beneficial effect on the development of the intestinal epithelium (Qaisrani et al. Citation2015; Bortoluzzi et al. Citation2017) and the control of pathogenic bacteria (Yang et al. Citation2018; Wu et al. Citation2020). In fact, butyrate can serve as an energy source for gut enterocytes, therefore, increasing the growth and overall absorptive surface area of the villi (Guilloteau et al. Citation2010). It can also exert an antimicrobial action (Leeson et al. Citation2005) using multiple mechanisms, such as an increased production of mucins and antimicrobial peptides and decreases in intestinal epithelial permeability (Peng et al. Citation2007). Moreover, undissociated butyric acid can penetrate bacterial cell walls and alter the internal pH and osmotic equilibrium of cells, affecting pathogenic bacteria and balancing the gut microbiota (van Der Wielen et al. Citation2000).
In poultry, dietary supplementation with butyric acid has been found to increase growth rate as well as augmenting carcase and breast weight (Leeson et al. Citation2005; Namkung et al. Citation2011). Indeed, butyric acid is involved in many pathways, such as lipolysis, lipogenesis, and gluconeogenesis (Heimann et al. Citation2015). It has also been hypothesised to have a role in regulating cell growth and differentiation programming (Liang et al. Citation2010; Mali et al. Citation2010; Berni Canani et al. Citation2012), with possible effects on muscle fibre in pigs (Duan et al. Citation2018), and meat quality in broiler chickens (Wu et al. Citation2020). To our knowledge, before now there has been no investigation concerning whether these effects imply differences in the rate and occurrence of myopathies. Myopathies, such as white striping (WS), wooden breast (WB) and spaghetti meat (SM), affect the pectoralis major muscle fibres at a histological level, involving changes in the chemical composition and rheological traits of meat (Trocino et al. Citation2015; Kuttappan et al. Citation2016; Baldi et al. Citation2018). Breast meat affected by myopathies shows an increase in intramuscular fat and decrease in protein content, which are associated with inflammation, myofibrillar structural degeneration, and regeneration of muscle fibres (Mudalal et al. Citation2015; Radaelli et al. Citation2017; Soglia et al. Citation2017).
Therefore, the present study aimed to evaluate comprehensively how dietary supplementation with a microencapsulated sodium-butyrate affects broiler chickens, including consideration of growth performance, slaughter results, gut morphology and gut microbiota at different ages, and the occurrence of myopathies in both females and males.
Materials and methods
Experimental facilities
The trial was undertaken in the poultry house of the Experimental Farm of the University of Padova (Legnaro, Padova, Italy), after 6 months of downtime. The poultry house was equipped with a cooling system, forced ventilation, radiant heating, and controlled light systems. Sixteen wire-net pens (2.5 × 2.4 m; 6 m2) were used, each equipped with five nipple drinkers and a circular feeder for manual distribution of feed. Each pen had a concrete floor covered with wood shaving litter. A total of 24 h of light were provided for the first 2 days after the chickens had arrived at the poultry house. Then, the hours of light were progressively reduced until an 18L:6D photoperiod was achieved, which was then maintained from 13 days of age onwards.
Animals, experimental groups, and in vivo recordings
The study was approved by the Ethical Committee for Animal Experimentation of the University of Padova (project 17/2016; prot. no. 154392; 10 May 2016). All animals were handled according to the principles of EU Directive 2010/63/EU regarding the protection of animals used for experimental and other scientific purposes.
A total of 384 male and 384 female 1-day-old chicks (Ross 308; n = 768) were delivered by a commercial truck to the experimental facilities of the University. All chicks had been vaccinated against Marek's disease, infectious bronchitis, and Newcastle disease at the hatchery. They were randomly allocated among the 16 pens (48 birds per pen) according to the following experimental arrangement: two sexes × two dietary treatments (control diet versus butyrate diet) and controlled from the day of arrival until slaughter at 45 days of age.
Chicks were individually weighed on the day of their arrival, identified by a leg mark, and then weighed once per week to measure live weight until commercial slaughtering. Pen feed consumption was measured daily during the trial.
Diets and feeding plans
Three commercial diets were offered during three periods and used as control diets (C): diet C1 (crude protein, 22.5%; ether extract, 5.25%; crude fibre, 2.61%), diet C2 (crude protein, 19.4%; ether extract, 6.10%; crude fibre, 2.44%), and diet C3 (crude protein, 18.5%; ether extract, 7.48%; crude fibre, 2.39%), to be fed from 0 to 12 days, 13 to 22 days, and 23 to 45 days, respectively. The components of each diet, in descending order of quantity included, were: C1 – soybean meal, corn, soft wheat, animal fat, monocalcium phosphate, calcium bicarbonate, sodium bicarbonate, sodium chloride, lignocellulose, and seaweed meal; C2 – corn, soybean meal, soft wheat, animal fat, mono-dicalcium phosphate, calcium carbonate, sodium bicarbonate, sodium chloride, seaweed meal, and lignocellulose; C3 – corn, soft wheat, soybean meal, animal fat, calcium carbonate, mono-dicalcium phosphate, sodium bicarbonate, sodium chloride, seaweed meal, and lignocellulose. All three diets also included vitamin–mineral premix and phytase, and diets C1 and C2 contained coccidiostat (Maxiban G160; Ely Lilly & Co Ltd, Indianapolis, IN). Control diets were produced by a commercial feed mill (Mangimificio Settecolli S.c.r.l., Montegalda, Vicenza, Italy). A commercial microencapsulated sodium butyrate (500 mg/kg; 30% Na-butyrate), designed for release butyrate along the small intestine, was added to each of the control diets to obtain three supplemented diets (B1, B2, B3) using a 180-l mechanical mixer before administration. All animals were fed ad libitum throughout the trial.
Diets were analysed to determine the contents of dry matter, crude protein and crude fibre using AOAC (Citation2000). Ether extract was analysed after acid hydrolysis (EC Citation1998).
Sampling of gut tissues for morphometric analysis
At 11, 24, and 35 days of age, 96 chickens (2 chickens per pen; 303 ± 25 g at 11 days, 880 ± 74 g at 24 days, and 2268 ± 74 g at 35 days) were selected for gut tissue sampling on the basis of average live weight and slaughtered by cervical dislocation. One sample (length, c. 2 cm) was taken from the jejunum, at the midpoint between the end of the duodenal loop and Meckel’s diverticulum (Wang et al. Citation2016), and washed in phosphate buffer saline (PBS) solution. Samples (c. 1 cm) were fixed in paraformaldehyde in PBS (0.1 M, pH 7.4) for later processing in standard histological analyses. Each sample was cut into 4-μm serial sections and subjected to haematoxylin and eosin staining for morphometric evaluation. The villus length and crypt depth were measured using image-analysis software (DP-soft, Olympus Optical, Co., Hamburg, Germany), according to the procedure described by Hampson (Citation1986).
Sampling of caecal content, DNA extraction, and library preparation
Each surface of the two caeca per animal was sterilised with 70% ethanol and opened with a scalpel. Then, equal amounts of the caecum contents from the two animals (approximately 32 mg/bird) in each pen were pooled and immediately frozen at −80 °C until analysis.
DNA was extracted using a DNeasy PowerSoil® DNA Isolation Kit (Qiagen, Hilden, Germany). A total of 100 mg of sterile 0.1 mm beads (BioSpec, Bartlesville, OK) were added, and a bead mill (TissueLyser, Qiagen, Hilden, Germany) was used to carry out disruption in high-speed shaking steps (2 × 30 s at 30 Hz) after the addition of lysis buffer. A total of 40 uL of proteinase K (Qiagen, Hilden, Germany) was added and incubated at 56 °C for 90 min. After this step, the DNeasy PowerSoil® DNA Isolation Kit manufacturer's instructions were followed. The concentration and purity of DNA were analysed using a spectrophotometer (NanoDrop ND-1000, Thermo Scientific, Waltham, MA).
A two step-amplification approach was used to construct the 16S DNA library. The samples were amplified in 20-μL reactions, each composed of 5 μL of diluted DNA, 0.4 μM of each primer (Table ), 0.25 mM deoxynucleotide (dNTP), 1 × Phusion HF buffer, and 1 U Phusion High-Fidelity DNA polymerase (New England BioLabs, Inc., Ipswich, MA). The PCR was conducted in a 2720 Thermal Cycler (Applied Biosystems, Foster City, CA) with 25 cycles of 95 °C for 30 s, 60 °C or 49 °C for 30 s, 72 °C for 45 s, and a final extension of 7 min at 72 °C. Three PCR replicates were performed per sample.
Table 1. Primers used in the study.
Products were purified using the SPRIselect Purification Kit (Beckman Coulter Life Sciences, Indianapolis, IN) and, after bead purification, the target band was confirmed using a 1.8% agarose gel. Barcodes were introduced by a second PCR analysis with platform-specific barcode-bearing primers. Each 50-μL PCR reaction contained the PCR product, 0.2 μM of each primer (Table ), 0.3 mM dNTP, 1 × Phusion HF buffer, and 1 U Phusion high-fidelity DNA polymerase; 10 cycles of the PCR profile described above were performed. PCR products were purified using the SPRIselect purification kit (Beckman Coulter Life Sciences, Indianapolis, IN). After the final purification, each sample was quantified using a Qubit® 2.0 Fluorometer (Invitrogen, Life Technologies, Monza, Italy). Amplicon library quality was tested using an Agilent 2100 Bioanalyzer (Agilent Technologies, Palo Alto, CA). Libraries were sequenced using the Illumina MiSeq platform with a paired-end 300-cycle run (Macrogen Inc., Seoul, South Korea).
Commercial slaughtering, and carcass and meat quality recording
At 45 days of age, all remaining chickens were slaughtered in a commercial slaughterhouse. Chickens were individually weighed before crating. Chickens were slaughtered according to the standard practice of the commercial slaughterhouse. Ready-to-cook carcases were recovered after 2 h refrigeration at 2 °C and individually weighed to measure the slaughter dressing percentage.
A total of 192 carcases (12 per pen, previously selected as representative of their pen on the basis of slaughter live weight) were submitted to gross examination to evaluate the occurrence (presence or absence) of WS, WB and SM on the pectoralis major muscle. Then, 96 (6 per pen) of the 192 carcases were selected and transported to the laboratories of the Department, University of Padova, to be stored at 2 °C before conducting meat quality analyses. Twenty-four hours after slaughter, carcases were dissected to obtain the main cuts and pectoralis major muscles for meat quality analysis (Petracci and Baéza Citation2011). A pH metre (Basic 20, Crison Instruments, SpA, Carpi, Modena, Italy), equipped with a specific electrode (cat. 5232, Crison Instruments, SpA), was used to measure pH values of the pectoralis major muscles in triplicate on their ventral side. The L*a*b* colour indexes were measured in triplicate on the ventral side of the same muscles using a Minolta CM-508 C spectrophotometer (Minolta Corp., Ramsey, NJ) (Petracci & Baéza Citation2011).
After measuring the pH and colour indexes, one meat portion (8 cm × 4 cm × 3 cm) per carcase was separated from the cranial side of the pectoralis major muscle, parallel to the muscle fibre direction, and stored under vacuum in plastic bags at −18 °C prior to meat analysis. Losses due to thawing and cooking were measured in this cut (Petracci and Baéza Citation2011). After thawing, each meat portion was placed in a plastic bag and cooked in a water bath at 80 °C for 45 min. After 40 min of cooling, another meat portion (4 cm × 2 cm × 1 cm) per meat portion was separated and the maximum shear force was assessed with a LS5 dynamometer (Lloyd Instruments Ltd, Bognor Regis, UK) using an Allo-Kramer (10 blades) probe (load cell, 500 kg; distance between blades, 5 mm; thickness, 2 mm; cutting speed, 250 mm/min) (Mudalal et al. Citation2015).
Statistical and bioinformatics analysis
Individual data for live weight, daily growth, slaughter results, and meat traits were submitted to ANOVA, with diet (control versus Na-butyrate), sex, and their interactions as the main factors of variability and pen as a random effect, using the PROC MIXED procedure in SAS (SAS Institute Citation2013). Pen data for feed intake and conversion were also submitted to ANOVA, with diet, sex, and their interactions as the main factors of variability, using the PROC GLM procedure (SAS Institute Citation2013). Individual data related to gut morphology were analysed using the same model, with diet, age, sex, and their interactions as the main effects. The myopathy rates were analysed using a Chi-square test according to diet and sex. Differences between means of p ≤ .05 were considered statistically significant.
The sequencing raw data were imported into the QIIME 2 pipeline version 2019.10 (https://qiime2.org/; Bolyen et al. Citation2019) with default parameters. The 16S raw reads were denoised, dereplicated, chimaera were removed, and raw reads were then merged using DADA2 software. After detecting and correcting Illumina amplicon sequence errors, DADA2 created a high‐resolution amplicon sequence variant (ASV) table. Subsequently, the taxonomy was assigned by the scikit‐learn method using a pre-trained Naive Bayes classifier against the SILVA database release 132 (https://www.arb-silva.de/documentation/release-132/). Data normalisation, alpha- and beta-diversity determination, and statistical analysis were conducted in CALYPSO version 8.84 (http://cgenome.net/wiki/index.php/Calypso; Zakrzewski et al. Citation2017). The raw sequence data were deposited in the Sequence Read Archive (SRA) database (accession no. PRJNA624052).
Results
Growth performance and health
On hatching day, the average chick weight was 46.0 ± 3.4 g, without significant differences between groups (Table ). Dietary treatment did not affect weight gain (73.5 g/d on average), feed intake (112.5 g/d), or feed conversion (1.43) across the whole trial. Females were lighter than males from 22 until 45 days of age (2975 g versus 3586 g; p ≤ .001); males showed a higher daily weight gain (+20%) and feed intake (+16%), and lower feed conversion ratio (−5%) throughout the trial (Table ). During the trial, there were recorded losses of 0.9% (i.e. 6 chickens) due to mortality (4 chickens) and lameness (2 chickens) (data not reported in Tables).
Table 2. Productive performancea (least square means) of broiler chickens prior to slaughter.
Slaughter results, meat quality, and myopathy rate
No effect of dietary treatment was recorded in the slaughter (Table ), meat quality (Table ), or myopathy occurrence (Figure ) results. An average of 35.9% of breasts showed WS exclusively, an average of 14.6% showed WB (whether or not associated with WS), and an average of 14.1% showed SM (whether or not associated with WS and/or WB) (Figure ).
Figure 1. White striping, wooden breast, and spaghetti meat rates in the breasts of chickens fed a control or Na-butyrate diet. White striping: breasts show only white striping; wooden breast: breasts may also show white striping; spaghetti meat: breasts may also show white striping and wooden breast.
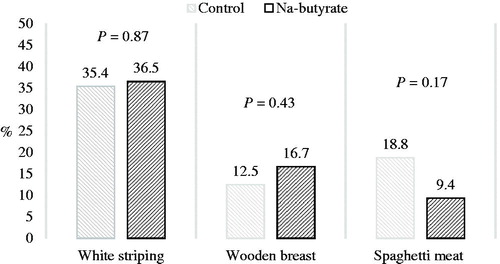
Table 3. Slaughter results and carcase traits (least square means) in chickens slaughtered at 45 d of age.
Table 4. Rheological traits (least square means) of the pectoralis major muscle in chickens slaughtered at 45 d of age.
Males had heavier carcases and higher proportions of breast, pectoralis major muscle, and hind leg meat than females (Table ); the former also had a higher L* index (+2.5%; p = .01) and cooking loss (+6%; p = .02) on the pectoralis major muscle than in females. However, final pH, thawing loss, and shear force were not sex-dependent (Table ).
Males showed a higher WB (+15.6%; p ≤ .01) and lower SM (−21.9%; p ≤ .001) rate compared with females; there were no significant differences concerning the WS rate (Figure ). The rate of SM doubled in females fed the control diets compared with those fed Na-butyrate diets (p = .06) (Figure ).
Gut morphometry
The dietary treatment did not affect gut morphology in terms of villi height (1024 µm on average), crypt depth (184 µm), or villi to crypt ratio (5.76) (Table ). Males had longer villi (p ≤ .001) and a higher villi to crypt ratio (p = .05) compared with females. Finally, with increasing age from 11 to 24 and 35 days, villi height increased (803–988 μm and 1282 μm, respectively) and crypt depth decreased (208–170 μm and 175 μm); thus, the villi to crypt ratio increased (4.02–5.91 and 7.34) (p ≤ .001) (Table ).
Table 5. Gut morphometry results for the effect of Na-butyrate supplementation, by age and gender.
Microbial profiles at the cecum
The 16S amplicon sequencing produced 13,351,438 raw sequences. Good’s Coverage index values were between 0.986 and 0.991, i.e. the sequencing appropriately described the community composition. Dietary treatment and sex had no effects on microbial diversity or the composition and abundance of intestinal microbiota (phyla, classes, and orders).
The species Richness index (defined as the number of different species represented in an ecological community, landscape, or region) increased with increasing chicken age (p ≤ .001; Figure ). The community composition was also affected by age as shown by the results of a principal coordinate analysis (adonis: p ≤ .001) (Figure ).
Figure 4. Alpha-diversity (richness index) (A) and beta-diversity (principal coordinate analysis) (B) of the caecal microbiota in chickens fed a control or Na-butyrate diet at different ages (11, 24, and 35 days). OTU: operational taxonomic unit; PCoA: principal coordinate analysis.
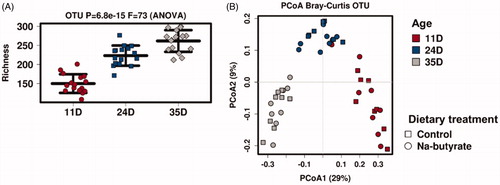
Caecum communities included the phyla Firmicutes (51.69–98.18%), Proteobacteria (0.35–28.55%), Bacteroidetes (0–38.37%), Tenericutes (0–21.27%), and Actinobacteria (0–0.11%). Sample clustering based on community composition at phylum level was related to chicken age: Tenericutes and Bacteroidetes increased, whereas Proteobacteria decreased (p ≤ .05) (Figure ). At the class level, Mollicutes increased, whereas Gammaproteobacteria, Erysipelotrichia, and Bacilli decreased (p ≤ .05) (Figure ). When final recordings were made at 35 days (p ≤ .05) (Figure ), Melainabacteria and Bacteroidia were found to have largely increased. At the order level, Pasteurellales, Lactobacillales, Erysipelotrichales, and Enterobacteriales decreased with increasing chicken age, whereas Mollicutes RF39 and Bacteroidales increased (Figure ). Significant changes in family abundance according to age are shown in Figure .
Discussion
Butyric acid has been widely used as a feed additive for broiler chickens. Nevertheless, analysis of its effects on performance and health are not always consistent, because several parameters may differ among studies. First, butyric acid may be administered either in its free form, associated with a salt, and/or protected in a glyceride matrix. Second, it may be supplemented at different doses and/or under different experimental conditions (genotype, trial duration, presence or absence of specific challenges and stress conditions).
Due to its volatile and pungent nature and since it is quickly absorbed at the crop level in the upper digestive tract (van Der Wielen et al. Citation2000), butyric acid is in most cases associated with salts, primarily calcium (Levy et al. Citation2015; Kaczmarek et al. Citation2016; Pineda-Quiroga et al. Citation2017) or sodium (Hu & Guo Citation2007; Liu et al. Citation2017; Wu et al. Citation2018). Moreover, free SCFA can negatively affect feed odour, reducing its palatability (Andreopoulou et al. Citation2014). When the salt is encapsulated (coated) in a glyceride matrix, it is released more slowly and its absorption in the lower gut is enhanced (Smith et al. Citation2012); this was the case for the microencapsulated Na-butyrate used in the present study. Accordingly, dietary supplementation rates for butyrate-based additives vary depending on multiple factors, including the form (free, salted, or coated), salt to organic acid ratio, amount and type of coating fat, and coating procedure (Guilloteau et al. Citation2010; Chamba et al. Citation2014).
Based on the literature, dietary supplementation by butyrate as encapsulated Ca-butyrate (50% butyric acid) enhanced daily weight gain at increasing doses until 500 mg/kg, especially during the first growth period (0–35 days), and thus final live weight and feed conversion (Levy et al. Citation2015; Kaczmarek et al. Citation2016). In contrast, high inclusion rates (1000 mg/kg protected Ca-butyrate; 70% butyric acid) decreased broiler weight and feed intake and worsened feed conversion at every growth stage (Pineda-Quiroga et al. Citation2017).
Similarly, dietary supplementation with Na-butyrate at increasing doses (up to 500 and 2000 mg/kg) positively influenced growth performance in the starter phase (0–21 days), without residual effects during the whole growth period (0–42 days) (Hu and Guo Citation2007). In contrast, the highest dietary supplementation (2000 mg/kg) negatively affected feed conversion (Hu and Guo Citation2007). A coated Na-butyrate (54% butyrate), supplemented at increasing doses until 1000 mg/kg, did not affect growth performance and feed conversion in broiler chicken during 42 days of treatment (Wu et al. Citation2018); this is consistent with the results of the present trial with microencapsulated Na-butyrate (500 mg/kg). Other authors, using a blend of protected (30%) and free (40%) Na-butyrate, observed a positive and significant effect on weight gain, feed intake, and feed conversion only in the growing and finishing periods (until 42 days of age) (Chamba et al. Citation2014).
When comparing dietary supplementation by butyric acid derivatives (glyceride mixture of mono-, di- and triglyceride) with antibiotic treatment in broiler chickens, no effect on growth performance was reported either at 200 mg/kg of mono- and diglycerides (approx. 75% butyric acid) (Leeson et al. Citation2005), or at different doses and combinations of butyrate glyceride blends (500 mg/kg monobutyrin + 500 mg/kg trybutirin, and 500 mg/kg trybutirin + 2000 mg/kg monobutyrin) (Bedford et al. Citation2017).
Indeed, dietary supplementation with increasing Na-butyrate doses (0, 250, 500, and 1000 mg/kg; 99.9%) did not affect broiler chicken performance under standard farm conditions, whereas the highest dose improved growth performance after an Escherichia coli challenge compared with broilers fed a control diet (Zhang et al. Citation2011). Thus, differences among studies may be related to Na-butyrate reaching the small intestine as well as the microbial environment to which chickens are exposed. Various authors (Leeson et al. Citation2005; Smulikowska et al. Citation2009) report that there is no growth-promoting response to fat-coated butyric acid or its mono- and diglycerides when chickens are reared in an environment with low pathogens or where health is good, such as in our trial conditions.
Previous studies have also shown that butyrate has a strong trophic effect on the gastrointestinal epithelium; it generally increases enterocyte proliferation, differentiation, and maturation in the small intestine (Guilloteau et al. Citation2010). The supplementation of butyric acid as mono- and diglycerides can be helpful in maintaining the intestinal villi structure after a specific challenge (Leeson et al. Citation2005), conditions under which changes in gut morphology have also been associated with improved growth performance. However, in healthy chickens, the effect of dietary supplementation with Na-butyrate on the small intestinal epithelium may be insignificant (Hu and Guo Citation2007); this is consistent with our results.
Gastrointestinal tract integrity is crucial to nutrition absorption, immunity development, and disease resistance, together with the gut microbial community balance. Alterations in the gut microbial community may adversely affect feed efficiency, productivity, and health of chickens (Kohl Citation2012; Wu et al. Citation2018; Kogut Citation2019; Zou et al. Citation2019). The gut microbiota in broiler chickens is characterised by a prevalence of Firmicutes and Bacteroidates, as confirmed in the present study, whereas Enterobacteriaceae are usually associated with gut dysbiosis, which, in turn, causes low microbial diversity (Kogut Citation2019).
Both in pigs (Manzanilla et al. Citation2006) and broilers (Jerzsele et al. Citation2012), Na-butyrate supplementation has been found to promote microbial diversity and induce competitive exclusion between beneficial and pathogenic bacteria in the gastrointestinal tract. A number of previous broiler studies have focussed on reductions in specific pathogenic bacterial counts, such as Escherichia coli, Salmonella enteritis, and Campylobacter jejuni in the caecal tract, whereas different butyrate products have the potential to mitigate the impact of pathogenic bacteria (Fernández-Rubio et al. Citation2009; Namkung et al. Citation2011; Liu et al. Citation2019).
Supplementation with butyrate glyceride (3000 mg/kg) did not affect ileal microbial diversity but altered caecal bacteria, with special reference to the class Mollicutes and genera Eubacterium, Subdoligranulum, Holdemania, Anaerotruncus, and Bifidobacterium (Yang et al. Citation2018). Reductions in the abundance of Mollicutes and Holdemania have been considered beneficial to chicken intestinal health (Yang et al. Citation2018). With specific reference to Na-butyrate, its dietary supplementation (800 mg/kg; 54% Na-butyrate) modified the chicken gut microbial community when compared with chickens fed a control diet or a diet supplemented at lower doses (400 mg/kg) (Wu et al. Citation2018). Additionally, dietary supplementation with Na-butyrate (1000 mg/kg) has been found to reduce compositional changes in caecal microflora when chickens were fed a low-energy low-amino acid diet compared with a control diet (Bortoluzzi et al. Citation2017). Finally, dietary supplementation with encapsulated Na-butyrate (150 or 300 mg/kg; 99.9% butyrate salt) did not modify the composition and structure of the microbial community in chickens submitted to gut inflammation when compared with healthy chickens (Zou et al. Citation2019). Thus, based on previous discussion, dietary butyrate could be more effective in modifying gut composition in chickens challenged by nutritional or health issues compared with healthy chickens, but both the dose and the form of supplementation may play a specific role.
In our trial, microbial composition and changes with age confirmed the good health of chickens. Microbial biodiversity within a sample (alpha-diversity) was lower at 11 days compared with 24 and 35 days, i.e. microbial complexity increased with age, consistently with the results of previous studies (Lu et al. Citation2003; Ranjitkar et al. Citation2016). Conversely, microbial diversity among samples (beta-diversity) was lower with increasing age, indicating a high level of similarity among them. Indeed, broiler chicken microbiota has been found to mature between 15 and 22 days (Ranjitkar et al. Citation2016), which is consistent with our observations of microbial stabilisation in older chickens (24 and 35 days). Bacteroidetes increased and Enterobacteriaceae decreased consistently in the microbiota with increasing age and good chicken health.
Slaughter yields and carcase traits are usually correlated with the daily growth rate and final live weight, for which we found no effects of dietary Na-butyrate supplementation. Similarly, Leeson et al. (Citation2005) observed no changes in carcase weight and breast yield in chickens supplemented with 100 mg/kg butyrate mono- and diglycerides, whereas breast muscle and carcase weight increased at a higher supplementation rate (200 mg/kg). Using butyrate glyceride blends (mono- and triglycerides), Bedford et al. (Citation2017) reported an increase in breast muscle and a decrease in fat. This finding is consistent with Yin et al. (Citation2016), who observed a decrease in abdominal and breast intramuscular fat deposition in chickens subjected to dietary supplementation with butyric acid derivatives. In fact, butyric acid has been associated with many pathways involved in lipolysis and lipogenesis (Heimann et al. Citation2015; Yin et al. Citation2016). Additionally, at the cellular level, butyric acid regulates gene expression by inhibiting histone deacetylase (HDAC) and enhances the efficiency of induced pluripotent stem cell derivation from fibroblasts; this suggests a role in regulating cell growth and differentiation programming (Liang et al. Citation2010; Mali et al. Citation2010; Berni Canani et al. Citation2012). In growing pigs, dietary supplementation with calcium β-hydroxy-β-methyl butyrate (0.62%) has been shown to promote the generation of more oxidative muscle types and increase muscle growth, especially in oxidative skeletal muscle, when compared with a control diet (Duan et al. Citation2018). This finding has been associated with an upregulation in mRNA expression of the oxidative isoform-MyHC IIa in the longissimus dorsi muscle and a downregulation in mRNA expression of the glycolytic isoform MyHC IIb in the soleus muscle. In broiler chickens, dietary supplementation with Na-butyrate (1000 mg/kg) has been found to upregulate gene expression of myogenic regulatory factor 5 (MYF5) and inhibit protein ubiquitination of FBXO9, FBXO22, and FBXO32, which can be activated by oxidative stressors (Wu et al. Citation2020). Under our experimental conditions, no effect on carcase traits and muscle growth was observed, but a reduction in the rate of SM was observed in females fed diets supplemented with Na-butyrate compared with those fed the control diet. Little information is available on SM compared with the other two myopathies (WB and WS), with the exception of a description of a similar pattern at a histological level (Baldi et al. Citation2018; Baldi et al. Citation2019; Soglia et al. Citation2019). Localised hypoxia, oxidative stress, increased intracellular calcium, and the presence of muscle fibre-type switching have been proposed as responsible for WB and WS, based on gene expression and pathways determined by RNA sequencing (Abasht et al. Citation2016; Zambonelli et al. Citation2016; Marchesi et al. Citation2019). Based on previous consideration of the effect of butyrate at the muscular level, these relationships could justify hypothesising a role for Na-butyrate on myopathy occurrences and rates; this, however, warrants further and more specific investigations with reference to SM.
Finally, while the results of the present study confirm main differences in performance and slaughter results between sexes in broiler chickens (Trocino et al. Citation2015), the reason why SM occurred more often in females than in males is not clear, and no research is yet available, to our knowledge. However, the higher occurrence of WB in males compared with females observed in our trial is consistent with earlier research, and is related to higher growth rate and breast weight of the former compared with the latter (Trocino et al. Citation2015).
Conclusions
Under the good health conditions established in the present study, dietary supplementation with the tested Na-butyrate affected neither the productive and slaughter results for broiler chickens, nor the gut health related to gut histology or the caecal microbial community whereas results could change with other butyrate forms and supplementation rates. Meat quality was not affected but, interestingly, there was a trend of a reduced SM rate in females supplemented with Na-butyrate compared with those fed the control diet; this merits further investigation in view of the impact of myopathies on the poultry meat industry and the possible role of butyrate at the muscular level. Finally, our study confirmed that WB occurs at a higher rate in males compared with females and showed, to our knowledge for the first time, that SM is more frequent in females than males. Further studies are required to clarify if the same myopathy can evolve differently in males and females, or if different mechanisms are involved.
Acknowledgements
The authors thank Mr. Giovanni Caporale for his kind technical assistance in providing histological sections.
Disclosure statement
No potential conflict of interest was reported by the author(s).
Additional information
Funding
References
- Abasht B, Mutryn MF, Michalek RD, Lee WR. 2016. Oxidative stress and metabolic perturbations in wooden breast disorder in chickens. PLoS One. 11(4):e0153750.
- Andreopoulou M, Tsiouris V, Georgopoulou I. 2014. Effects of organic acids on the gut ecosystem and on the performance of broiler chickens. J Hell Vet Med Soc. 65:289–302.
- AOAC. 2000. Official Methods of Analysis. 17th ed. Arlington, VA: Assoc. Off. Analyst. Chemists.
- Baldi G, Soglia F, Laghi L, Tappi S, Rocculi P, Tavaniello S, Prioriello D, Mucci R, Maiorano G, Petracci M. 2019. Comparison of quality traits among breast meat affected by current muscle abnormalities. Food Res Int. 115:369–376.
- Baldi G, Soglia F, Mazzoni M, Sirri F, Canonico L, Babini E, Laghi L, Cavani C, Petracci M. 2018. Implications of white striping and spaghetti meat abnormalities on meat quality and histological features in broilers. Animal. 12(1):164–173.
- Bedford A, Yu H, Squires EJ, Leeson S, Gong J. 2017. Effects of supplementation level and feeding schedule of butyrate glycerides on the growth performance and carcass composition of broiler chickens. Poult Sci. 96(9):3221–3228.
- Berni Canani R, Di Costanzo M, Leone L. 2012. The epigenetic effects of butyrate: potential therapeutic implications for clinical practice. Clin Epigenetics. 4(1):4.
- Bolyen E, Rideout JR, Dillon MR, Bokulich NA, Abnet CC, Al-Ghalith GA, Alexander H, Alm EJ, Arumugam M, Asnicar F, et al. 2019. Reproducible, interactive, scalable and extensible microbiome data science using QIIME 2. Nat Biotechnol. 37(8):852–857.
- Bortoluzzi C, Pedroso AA, Mallo JJ, Puyalto M, Kim WK, Applegate TJ. 2017. Sodium butyrate improved performance while modulating the cecal microbiota and regulating the expression of intestinal immune-related genes of broiler chickens. Poult Sci. 96(11):3981–3993.
- Caly DL, D’Inca R, Auclair E, Drider D. 2015. Alternatives to antibiotics to prevent necrotic enteritis in broiler chickens: a microbiologist’s perspective. Front Microbiol. 6:1336.
- Cervantes HM. 2015. Antibiotic-free poultry production: is it sustainable?. J Appl Poult Res. 24(1):91–97.
- Chamba F, Puyalto M, Ortiz A, Torrealba H, Mallo JJ, Riboty R. 2014. Effect of partially protected sodium butyrate on performance, digestive organs, intestinal villi and E. coli development in broilers chickens. Int J Poultry Sci. 13(7):390–396.
- van Der Wielen PW, Biesterveld S, Notermans S, Hofstra H, Urlings BA, van Knapen F. 2000. Role of volatile fatty acids in development of the cecal microflora in broiler chickens during growth. Appl Environ Microbiol. 66(6):2536–2540.
- Duan YH, Zeng LM, Li FN, Kong XF, Xu K, Guo QP, Wang WL, Zhang LY. 2018. β-Hydroxy-β-methyl butyrate promotes leucine metabolism and improves muscle fibre composition in growing pigs. J Anim Physiol Anim Nutr. 102(5):1328–1339.
- EC. 1998. Commission Directive 98/64/EC of 3 September 1998 establishing Community methods of analysis for the determination of amino acids, crude oils and fats, and olaquindox in feeding stuffs and amending Directive 71/393/EEC. Off J Eur Union. 19.9.1998, L257/14.
- Fernández-Rubio C, Ordóñez C, Abad-González J, Garcia-Gallego A, Honrubia MP, Mallo JJ, Balaña-Fouce R. 2009. Butyric acid-based feed additives help protect broiler chickens from Salmonella enteritidis infection. Poult Sci. 88(5):943–948.
- Guilloteau P, Martin L, Eeckhaut V, Ducatelle R, Zabielski R, Van Immerseel F. 2010. From the gut to the peripheral tissues: the multiple effects of butyrate. Nutr Res Rev. 23(2):366–384.
- Hampson DJ. 1986. Alterations in piglet small intestinal structure at weaning. Res Vet Sci. 40(1):32–40.
- Heimann E, Nyman M, Degerman E. 2015. Propionic acid and butyric acid inhibit lipolysis and de novo lipogenesis and increase insulin-stimulated glucose uptake in primary rat adipocytes. Adipocyte. 4(2):81–88.
- Hu Z, Guo Y. 2007. Effects of dietary sodium butyrate supplementation on the intestinal morphological structure, absorptive function and gut flora in chickens. Anim Feed Sci Technol. 132(3–4):240–249.
- Jerzsele A, Szeker K, Csizinszky R, Gere E, Jakab C, Mallo JJ, Galfi P. 2012. Efficacy of protected sodium butyrate, a protected blend of essential oils, their combination, and Bacillus amyloliquefaciens spore suspension against artificially induced necrotic enteritis in broilers. Poult Sci. 91(4):837–843.
- Kaczmarek SA, Barri A, Hejdysz M, Rutkowski A. 2016. Effect of different doses of coated butyric acid on growth performance and energy utilization in broilers. Poult Sci. 95(4):851–859.
- Kogut MH. 2019. The effect of microbiome modulation on the intestinal health of poultry. Anim Feed Sci Technol. 250:32–40.
- Kohl KD. 2012. Diversity and function of the avian gut microbiota. J Comp Physiol B, Biochem Syst Environ Physiol. 182(5):591–602.
- Kuttappan VA, Hargis BM, Owens CM. 2016. White striping and woody breast myopathies in the modern poultry industry: a review. Poult Sci. 95(11):2724–2733.
- Leeson S, Namkung H, Antongiovanni M, Lee EH. 2005. Effect of butyric acid on the performance and carcass yield of broiler chickens. Poult Sci. 84(9):1418–1422.
- Levy AW, Kessler JW, Fuller L, Williams S, Mathis GF, Lumpkins B, Valdez F. 2015. Effect of feeding an encapsulated source of butyric acid (ButiPEARL) on the performance of male Cobb broilers reared to 42 d of age. Poult Sci. 94(8):1864–1870.
- Liang G, Taranova O, Xia K, Zhang Y. 2010. Butyrate promotes induced pluripotent stem cell generation. J Biol Chem. 285(33):25516–25521.
- Liu JD, Bayir HO, Cosby DE, Cox NA, Williams SM, Fowler J. 2017. Evaluation of encapsulated sodium butyrate on growth performance, energy digestibility, gut development, and Salmonella colonization in broilers. Poult Sci. 96(10):3638–3644.
- Liu JD, Lumpkins B, Mathis G, Williams SM, Fowler J. 2019. Evaluation of encapsulated sodium butyrate with varying releasing times on growth performance and necrotic enteritis mitigation in broilers. Poult Sci. 98(8):3240–3245.
- Lu J, Idris U, Harmon B, Hofacre C, Maurer JJ, Lee MD. 2003. Diversity and succession of the intestinal bacterial community of the maturing broiler chicken. Appl Environ Microbiol. 69(11):6816–6824.
- Mali P, Chou BK, Yen J, Ye Z, Zou J, Dowey S, Brodsky RA, Ohm JE, Yu W, Baylin SB, et al. 2010. Butyrate greatly enhances derivation of human induced pluripotent stem cells by promoting epigenetic remodeling and the expression of pluripotency-associated genes. Stem Cells. 28(4):713–720.
- Manzanilla EG, Nofrarías M, Anguita M, Castillo M, Perez JF, Martín-Orúe SM, Kamel C, Gasa J. 2006. Effects of butyrate, avilamycin, and a plant extract combination on the intestinal equilibrium of early-weaned pigs. J Anim Sci. 84(10):2743–2751.
- Marchesi JAP, Ibelli AMG, Peixoto JO, Cantão ME, Pandolfi JRC, Marciano CMM, Zanella R, Settles ML, Coutinho LL, Ledur MC. 2019. Whole transcriptome analysis of the pectoralis major muscle reveals molecular mechanisms involved with white striping in broiler chickens. Poult Sci. 98(2):590–601.
- Mudalal S, Lorenzi M, Soglia F, Cavani C, Petracci M. 2015. Implications of white striping and wooden breast abnormalities on quality traits of raw and marinated chicken meat. Animal. 9(4):728–734.
- Namkung H, Yu H, Gong J, Leeson S. 2011. Antimicrobial activity of butyrate glycerides toward Salmonella typhimurium and Clostridium perfringens. Poult Sci. 90(10):2217–2222.
- Peng L, He Z, Chen W, Holzman IR, Lin J. 2007. Effects of butyrate on intestinal barrier function in a Caco-2 cell monolayer model of intestinal barrier. Pediatr Res. 61(1):37–41.
- Petracci M, Baéza E. 2011. Harmonization of methodologies for the assessment of poultry meat quality features. Worlds Poult Sci J. 67(1):137–151.
- Pineda-Quiroga C, Atxaerandio R, Ruiz R, García-Rodríguez A. 2017. Effects of dry whey powder alone or combined with calcium butyrate on productive performance, duodenal morphometry, nutrient digestibility, and ceca bacteria counts of broiler chickens. Livest Sci. 206:65–70.
- Qaisrani SN, van Krimpen MM, Kwakkel RP, Verstegen MWA, Hendriks WH. 2015. Diet structure, butyric acid, and fermentable carbohydrates influence growth performance, gut morphology, and cecal fermentation characteristics in broilers. Poult Sci. 94(9):2152–2164.
- Radaelli G, Piccirillo A, Birolo M, Bertotto D, Gratta F, Ballarin C, Vascellari M, Xiccato G, Trocino A. 2017. Effect of age on the occurrence of muscle fiber degeneration associated with myopathies in broiler chickens submitted to feed restriction. Poult Sci. 96(2):309–319.
- Ranjitkar S, Lawley B, Tannock G, Engberg RM. 2016. Bacterial succession in the broiler gastrointestinal tract. Appl Environ Microbiol. 82(8):2399–2410.
- SAS Institute. 2013. The SAS/STAT 9.4 User’s guide. Cary, NC: SAS Inst. Inc.
- Smith DJ, Barri A, Herges G, Hahn J, Yersin AG, Jourdan A. 2012. In vitro dissolution and in vivo absorption of calcium [1-(14)c]butyrate in free or protected forms. J Agric Food Chem. 60(12):3151–3157.
- Smulikowska S, Czerwiński J, Mieczkowska A, Jankowiak J. 2009. The effect of fat-coated organic acid salts and a feed enzyme on growth performance, nutrient utilization, microflora activity, and morphology of the small intestine in broiler chickens. J Anim Feed Sci. 18(3):478–489.
- Soglia F, Gao J, Mazzoni M, Puolanne E, Cavani C, Petracci M, Ertbjerg P. 2017. Superficial and deep changes of histology, texture and particle size distribution in broiler wooden breast muscle during refrigerated storage. Poult Sci. 96(9):3465–3472.
- Soglia F, Mazzoni M, Petracci M. 2019. Spotlight on avian pathology: current growth-related breast meat abnormalities in broilers. Avian Pathol. 48(1):1–3.
- Trocino A, Piccirillo A, Birolo M, Radaelli G, Bertotto D, Filiou E, Petracci M, Xiccato G. 2015. Effect of genotype, gender and feed restriction on growth, meat quality and the occurrence of white striping and wooden breast in broiler chickens. Poult Sci. 94(12):2996–3004.
- Wang X, Farnell YZ, Peebles ED, Kiess AS, Wamsley KGS, Zhai W. 2016. Effects of prebiotics, probiotics, and their combination on growth performance, small intestine morphology, and resident Lactobacillus of male broilers. Poult Sci. 95(6):1332–1340.
- Wu W, Xiao Z, An W, Dong Y, Zhang B. 2018. Dietary sodium butyrate improves intestinal development and function by modulating the microbial community in broilers. PLoS One. 13(5):e0197762.
- Wu Y, Wang Y, Wu W, Yin D, Sun X, Guo X, Chen J, Mahmood T, Yan L, Yuan J. 2020. Effects of nicotinamide and sodium butyrate on meat quality and muscle ubiquitination degradation genes in broilers reared at a high stocking density. Poult Sci. 99(3):1462–1470.
- Yang X, Yin F, Yang Y, Lepp D, Yu H, Ruan Z, Yang C, Yin Y, Hou Y, Leeson S, et al. 2018. Dietary butyrate glycerides modulate intestinal microbiota composition and serum metabolites in broilers. Sci Rep. 8(1):1–12.
- Yin F, Yu H, Lepp D, Shi X, Yang X, Hu J, Leeson S, Yang C, Nie S, Hou Y, et al. 2016. Transcriptome analysis reveals regulation of gene expression for lipid catabolism in young broilers by butyrate glycerides. PLoS One. 11(8):e0160751
- Zakrzewski M, Proietti C, Ellis JJ, Hasan S, Brion M-J, Berger B, Krause L. 2017. Calypso: a user-friendly web-server for mining and visualizing microbiome-environment interactions. Bioinforma Oxf Engl. 33:782–783.
- Zambonelli P, Zappaterra M, Soglia F, Petracci M, Sirri F, Cavani C, Davoli R. 2016. Detection of differentially expressed genes in broiler pectoralis major muscle affected by White Striping – wooden breast myopathies. Poult Sci. 95(12):2771–2785.
- Zhang WH, Jiang Y, Zhu QF, Gao DF, Dai SF, Chen J, Zhou GH. 2011. Sodium butyrate maintains growth performance by regulating the immune response in broiler chickens. Br Poult Sci. 52(3):292–301.
- Zou X, Ji J, Qu H, Wang J, Shu DM, Wang Y, Liu TF, Li Y, Luo CL. 2019. Effects of sodium butyrate on intestinal health and gut microbiota composition during intestinal inflammation progression in broilers. Poult Sci. 98(10):4449–4456.