Abstract
Heat stress jeopardised animal health by inducing oxidative stress and inflammation. This study was done to investigate the effects of chitosan oligosaccharides (COS) on oxidative stress and inflammation response in liver and spleen of yellow-feather broilers under high ambient temperature. A total of 144 35-day-old female Chinese indigenous yellow-feather broilers (body weight 450.21 ± 10.05 g) were randomly allocated into 3 dietary treatments with 6 replication pens, each pen had 8 broilers. The treatments were: Control, basal diet; COS100, basal diet with 100 mg/kg COS; COS200, basal diet with 200 mg/kg COS. During day 22 to 42 (57 to 77 days of age), broilers in the COS200 group had higher (p < .05) ADFI than broilers in the Control group. Broilers in the COS200 group had lower (p < .05) serum alanine aminotransferase, aspartate aminotransferase, and tumour necrosis factor-α levels, and spleen malondialdehyde (MDA) content, but had higher (p < .05) liver superoxide dismutase activity and serum interleukin-10 level than broilers in the Control group. Broilers in the COS100 and COS200 groups had lower (p < .05) serum and liver MDA content, and serum interleukin-1β level, but had higher (p < .05) serum glutathione peroxidase activity than broilers in the Control group. In conclusion, dietary COS supplementation can alleviate heat stress-induced oxidative stress and inflammation response in liver and spleen by decreasing lipid peroxidation, increasing anti-oxidant enzyme activities and IL-10 level.
Dietary COS decreased serum ALT, AST, IL-1β, TNF-α and MDA.
Dietary COS increased serum GSH-Px and IL-10, liver SOD, and spleen GSH-Px.
HIGHLIGHTS
Introduction
High ambient temperature jeopardised animal performance and health, which made great economic losses to global animal production (Attia et al. Citation2011). With global warming, heat stress became a widely attention issue for animal industry, particularly in sub-tropical and tropical regions. Broilers were extremely sensitive to high ambient temperature due to feather covered, lack of sweat glands, and higher metabolic activity (Liu et al. Citation2014). Previous studies indicated that heat stress had negative effects on performance, health, and well-beings of broilers (Lara and Rostagno Citation2013). Moreover, heat stress resulted in oxidative stress (Ando et al. Citation1997), which, in turn, inducing adverse effects on DNA, protein, and lipids, and finally led to tissue damage, inflammatory disease, and some other diseases (Zhang et al. Citation2018; Cheng et al. Citation2019a; Cheng et al. Citation2019b). Heat stress also induced inflammation response (Jang et al. Citation2014; Ohtsu et al. Citation2015; Wang et al. Citation2018). Oxidative stress and inflammation response were closely related, previous studies reported that heat stress can induce oxidative and inflammation stress in broilers (Jang et al. Citation2014; Song et al. Citation2017). Both oxidative stress and inflammation response can activate tissue apoptosis (Xia et al. Citation2014; Jiang et al. Citation2018), therefore, suppressing oxidative stress and inhibiting inflammation response were important for preventing tissue damage under heat stress. Liver was the primary detoxified and metabolic organ, the oxidative stress and excessive inflammation response can lead to liver disorder (Sakka Citation2007; Abdel-Misih and Bloomston Citation2010; Sun and Karin Citation2013). Spleen was the peripheral lymphoid organ and regulating immune response by changing cytokines expression (Abdul-Careem et al. Citation2007; Coble et al. Citation2011; Ohtsu et al. Citation2015). Former studies reported that heat stress-induced spleen dysplasia, accompanied with oxidative stress, apoptosis, and changed splenic cytokines expression (Ohtsu et al. Citation2015; Zhang et al. Citation2018). Therefore, suppressing oxidative stress and inhibiting inflammation response were important for maintain liver and spleen function under heat stress. Nutritional manipulation was one of the effective methods for deletion or adjustment of high ambient temperature effects on broilers, especially the anti-oxidant supplementation (Alishah et al. Citation2013; Nourozi et al. Citation2013). Previously, we reported that dietary chitosan oligosaccharides (COS) supplementation can alleviate liver and spleen oxidative stress and inflammation response in heat-stressed rats (Lan et al. Citation2019), as well as reducing lipid peroxidation and increasing antioxidant capacity in H2O2-chanllenged rats (Lan et al. Citation2019). The COS, was the degraded products of chitosan or chitin (Zou et al. Citation2016), had numerous biological activities, including anti-oxidant (Li et al. Citation2017), anti-inflammatory (Xiong et al. Citation2015), immune stimulating (Li et al. Citation2019), and free radical scavenging capacity (Je et al. Citation2004). It was unknown whether dietary COS supplementation can attenuate oxidative stress and inflammation response of broilers under high ambient temperature, therefore, the purpose of this study was to investigate the effects of COS on oxidative stress and inflammation response in liver and spleen of broilers under high ambient temperature.
Materials and methods
Experiment design and dietary treatments
A total of 200 1-day-old female Chinese indigenous yellow-feather broilers were purchased from local breeding company (Zhanjiang, Guangdong province, China) and raised at a recommended environment condition from 1 to 34 days of age with commercial feed. At day of 35, a total of 14,435-day-old broilers (body weight 450.21 ± 10.05 g) were selected and randomly divided into 3 treatments with 6 replication pens, each pen had 8 broilers. The treatments were: Control, basal diet without COS; COS100, basal diet with 100 mg/kg COS; COS200, basal diet with 200 mg/kg COS. The basal diet (Table ) was formulated to meet or exceed the nutrient requirement of the Feeding Standard of Chicken, China (NY/T 33-2004). COS was purchased from Jiangsu Xinrui Biotechnology Co., Ltd. (HPLC purity 95%, deacetylation degree over 95% and average molecular weight below 32 kDa).
Table 1. Basal diet composition (as-fed basis).
Experimental conditions
Broilers of each replication pen were assigned in battery pens (124 cm length × 64 cm width × 40 cm height). Artificial light provided 23 hour per day by fluorescent lights and had free access to feed and water. The indoor ambient temperature and relative humidity were recorded daily at 08:00, 12:00, and 18:00 daily, and average temperature and relative humidity were calculated and presented in Figure .
Performance parameters
On day 1 (35 days of age), 21 (56 days of age), 42 (77 days of age), and 56 (91 days of age), body weight (BW) recorded on pen basis. Feed intake (FI) recorded on pen basis every week. The average daily gain (ADG), average daily feed intake (ADFI), and feed conversion ratio (FCR) were calculated.
Sample preparation
On day 56 (91 days of age), after 12-hour fast, 6 broilers per treatment (1 broiler from each replication pen) randomly selected, blood samples were collected from the brachial vein and centrifuged at 3,000 g × 10mins at 4 °C to obtain serum. The serum samples stored at −20 °C until analysis. Then the broilers were euthanized by cervical dislocation, liver and spleen were collected and flushed with ice-cold phosphate-buffered saline (PBS), quick freezing in liquid nitrogen, and frozen at −80 °C until analysis.
Serum parameters
Serum alanine aminotransferase (ALT) and aspartate aminotransferase (AST) concentrations were measured with commercial enzyme immunoassay kits (Cusabio Biotech. Co., Ltd., Wuhan, Hubei, China) according to the manufacturers’ instructions. The activities of superoxide dismutase (SOD), glutathione peroxidase (GSH-Px) and catalase (CAT), the levels of malondialdehyde (MDA), interleukin-1β (IL-1β), interleukin-10 (IL-10), and tumour necrosis factor-α (TNF-α) were measured with corresponding commercial assay kits (Nanjing Jiancheng Bioengineering Institute, Nanjing, China) following the manufacturers’ instruction.
Oxidative status and cytokines
One gram of liver and spleen samples were homogenised at a ratio of 1: 9 (weight/volume) with ice-cold PBS. Homogenate was centrifuged at 3,000 g × 15 mins at 4 °C to obtain supernatant, and the analysis conducted immediately. The supernatant protein concentration was determined by the Bradford method. The SOD, GSH-Px, and CAT activities, and MDA, IL-1β, IL-10, and TNF-α levels were measured with corresponding assay kits (Nanjing Jiancheng Bioengineering Institute, Nanjing, China) following the manufacturers’ instruction.
Statistical analysis
The pen was used as the experiment unit and all data were analysed with SAS 2003 (v. 9.1, SAS Institute Inc., Cary, NC). Data was analysed using one-way analysis of variance (ANOVA) followed by Duncan’s multiple range test to analyse differences among treatments. Orthogonal polynomial contrasts were conducted to analyse the linear and quadratic effects of dietary COS supplementation level. p < .05 was considered significant, and p < .10 was considered as a trend.
Results
Growth performance
During day 1 to 21 (35 to 56 days of age), day 43 to 56 (78 to 91 days of age), and day 1 to 56 (35 to 91 days of age), no significant differences were observed in ADG, ADFI or G:F among treatments (Table ). During day 22 to 42 (57 to 77 days of age), there were linear (p < .05) improvement in ADFI and quadratic (p < .05) improvement in ADG with COS supplementation. In addition, broilers in the COS200 group had higher (p < .05) ADG than broilers in the COS100 group. Broilers in the COS200 group had higher (p < .05) ADFI than broilers in the Control group.
Table 2. Effects of chitosan oligosaccharides on growth performance of broilers under high ambient temperature.
Liver function
There were linear (p < .05) decreasing in serum ALT and AST levels with COS supplementation (Figure ), and dietary 200 mg/kg COS supplementation significantly decreased (p < .05) ALT and AST levels compared with the Control group.
Figure 2. Effects of chitosan oligosaccharides (COS) on serum alanine aminotransferase (ALT) and aspartate aminotransferase (AST) in yellow-feather broilers under high ambient temperature. Control group, basal diet; COS100 group, basal diet with 100 mg/kg COS; COS200 group, basal diet with 200 mg/kg COS. Values are mean ± standard error of mean. The values have different superscript letters are different (p < .05).
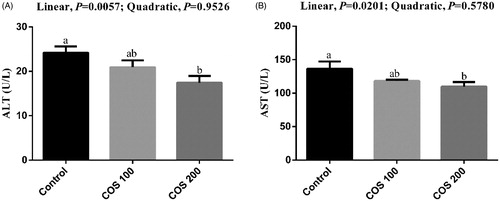
Anti-oxidant status
No significant differences were observed in serum SOD and CAT activities among treatments (Figure ). There were linear (p < .05) decreasing in serum MDA content, and linear (p < .05) improvement in serum GSH-Px activity with COS supplementation. Dietary 100 mg/kg and 200 mg/kg COS supplementation significantly decreased (p < .05) MDA content and increased (p < .05) GSH-Px activity compared with the Control group.
Figure 3. Effects of chitosan oligosaccharides (COS) on anti-oxidant status in serum of yellow-feather broilers under high ambient temperature. Control group, basal diet; COS100 group, basal diet with 100 mg/kg COS; COS200 group, basal diet with 200 mg/kg COS. MDA: malondialdehyde; SOD: superoxide dismutase; GSH-Px: glutathione peroxidase; CAT: catalase. Values are mean ± standard error of mean. The values have different superscript letters are different (p < .05).
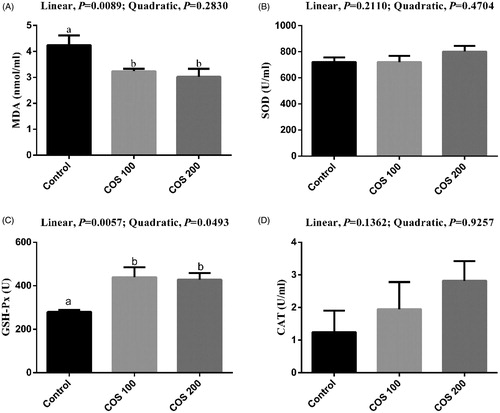
No significant differences were observed in liver GSH-Px and CAT activities among treatments (Figure ). There were linear (p < .05) decreasing in liver MDA content, and linear (p < .05) improvement in liver SOD activity with COS supplementation. Dietary 100 mg/kg and 200 mg/kg COS supplementation significantly decreased (p < .05) MDA content and dietary 200 mg/kg COS supplementation significantly increased (p < .05) SOD activity compared with the Control group.
Figure 4. Effects of chitosan oligosaccharides (COS) on anti-oxidant status in liver of yellow-feather broilers under high ambient temperature. Control group, basal diet; COS100 group, basal diet with 100 mg/kg COS; COS200 group, basal diet with 200 mg/kg COS. MDA: malondialdehyde; SOD: superoxide dismutase; GSH-Px: glutathione peroxidase; CAT: catalase. Values are mean ± standard error of mean. The values have different superscript letters are different (p < .05).
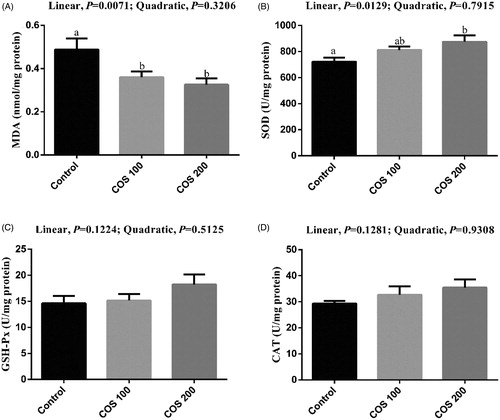
No significant differences were observed in spleen SOD and CAT activities among treatments (Figure ). There was linear (p < .05) decreasing in liver MDA content and a linear increasing trend (p < .10) in spleen GSH-Px activity with COS supplementation. Dietary 200 mg/kg COS supplementation significantly decreased (p < .05) MDA content compared with the Control group.
Figure 5. Effects of chitosan oligosaccharides (COS) on anti-oxidant status in spleen of yellow-feather broilers under high ambient temperature. Control group, basal diet; COS100 group, basal diet with 100 mg/kg COS; COS200 group, basal diet with 200 mg/kg COS. MDA: malondialdehyde; SOD: superoxide dismutase; GSH-Px: glutathione peroxidase; CAT: catalase. Values are mean ± standard error of mean. The values have different superscript letters are different (p < .05).
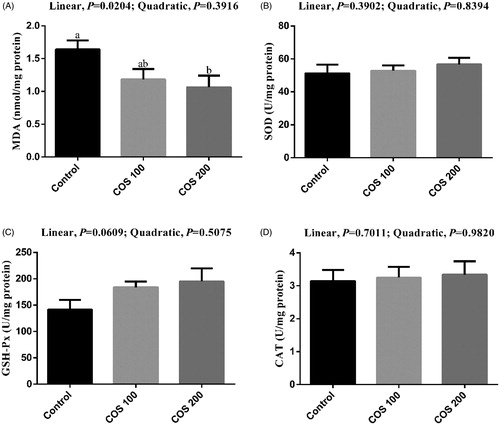
Inflammatory cytokines
There were linear (p < .05) decreasing in serum IL-1β and TNF-α levels, and linear (p < .05) improvement in serum IL-10 level with COS supplementation (Figure ). Dietary 100 mg/kg and 200 mg/kg COS supplementation significantly decreased (p < .05) IL-1β level, and dietary 200 mg/kg COS significantly increased (p < .05) IL-10 and decreased TNF-α level compared with the Control group.
Figure 6. Effects of chitosan oligosaccharides (COS) on inflammatory cytokines in serum of yellow-feather broilers under high ambient temperature. Control group, basal diet; COS100 group, basal diet with 100 mg/kg COS; COS200 group, basal diet with 200 mg/kg COS. IL-1β: interleukin-1β; IL-10: interleukin-10; TNF-α: tumour necrosis factor-α. Values are mean ± standard error of mean. The values have different superscript letters are different (p < .05).
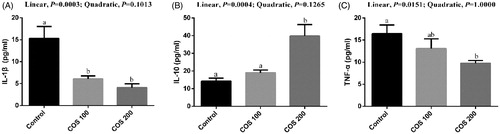
No significant differences were observed in liver and spleen IL-1β and TNF-α levels, but a linear improving trend (p < .10) in liver and spleen IL-10 with COS supplementation (Figures and , respectively).
Figure 7. Effects of chitosan oligosaccharides (COS) on inflammatory cytokines in liver of yellow-feather broilers under high ambient temperature. Control group, basal diet; COS100 group, basal diet with 100 mg/kg COS; COS200 group, basal diet with 200 mg/kg COS. IL-1β: interleukin-1β; IL-10: interleukin-10; TNF-α: tumour necrosis factor-α. Values are mean ± standard error of mean.
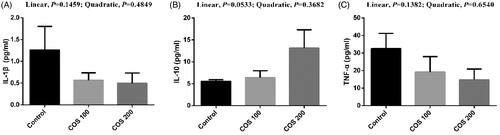
Figure 8. Effects of chitosan oligosaccharides (COS) on inflammatory cytokines in spleen of yellow-feather broilers under high ambient temperature. Control group, basal diet; COS100 group, basal diet with 100 mg/kg COS; COS200 group, basal diet with 200 mg/kg COS. IL-1β: interleukin-1β; IL-10: interleukin-10; TNF-α: tumour necrosis factor-α. Values are mean ± standard error of mean.
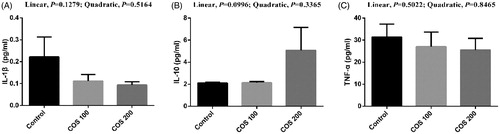
Discussion
The main purpose of poultry production was to maintain the broilers’ health status, improve production performance and immune response (Abdel-Wareth et al. Citation2019). Our study demonstrated that dietary COS supplementation can improve ADG, ADFI, anti-oxidant capacity and alleviate inflammation response in serum, liver and spleen of broilers under high ambient temperature.
The COS, the functional oligosaccharides, was beneficial to broilers’ growth performance by increasing BW gain, FI and FCR (Huang et al. Citation2005; Li et al. Citation2007; Li et al. Citation2019). Moreover, dietary oligosaccharides exhibited better FI, BW gain, and FCR in heat-stressed broilers (Cheng et al. Citation2018; Cheng et al. Citation2019c). Similarly, in this study, dietary COS supplementation improved ADG and ADFI during day 22 to 42 (57 to 77 days of age). The improvement ADG may due to the enhancement of nutrient digestibility, intestinal integrity, anti-oxidant capacity and immunity (Huang et al. Citation2005; Li et al. Citation2007; Zhou et al. Citation2009; Li et al. Citation2019). It was believed that ambient temperature above 30 °C is sufficient to induce heat stress for broilers, resulting in physiological disturbance and poor growth performance (Quinteiro-Filho et al. Citation2010). In this study, the broilers reared under ambient temperature above 30 °C, dietary COS supplementation improved growth performance, suggesting that COS can attenuate the heat stress and simultaneously better growth performance. However, the ADG, ADFI, and G:F did not differ among the treatments during day 1 to 21 (35 to 56 days of age), day 43 to 56 (78 to 91 days of age), or day 1 to 56 (35 to 91 days of age). Similarly, Tufan et al. (Citation2015) indicated that dietary COS supplementation had no effects on ADG, ADFI or G:F in Japanese quails. While, other studies reported that dietary COS supplementation had beneficial effects on ADG, ADFI or G:F in broilers (Li et al. Citation2007; Li et al. Citation2019). The inconsistent results may be associated with the stress condition, the different deacetylation degree, molecular weight, or dose of COS used in these experiments.
In health status, AST and ALT enzymes were normally expressed in liver, when liver damaged, AST and ALT enzymes released into the blood (Kim et al. Citation2015), the elevated serum ALT and AST levels were served as marker of liver damage (Dufour et al. Citation2000). Heat stress was known to induce liver damage (Zeng et al. Citation2014), and increased serum AST and ALT levels (Tessari et al. Citation2010; Cheng et al. Citation2019b). As expected, dietary COS supplementation alleviated liver damage by decreasing serum AST and ALT levels under high ambient temperature. Other studies also reported that dietary COS supplementation decreased serum AST and ALT levels in D-galactose-induced subacute aging mice (Kong et al. Citation2018) and carbon tetrachloride-induced mice (Miao et al. Citation2007). These results suggested that dietary COS supplementation can attenuate the high ambient temperature-induced liver damage.
Exposure to heat stress resulted in reactive oxygen species (ROS) overproduction and disturbing the balance between ROS production and antioxidant defense system via increasing lipid peroxidation and depletion of antioxidant enzymes (Azad et al. Citation2010; Yang et al. Citation2010; Liu et al. Citation2014), eventually contributed to oxidative stress and damage (Hall et al. Citation2010; Sahin et al. Citation2010). The MDA, the end product of lipid peroxidation, served as the marker of oxidative stress (Cheng et al. Citation2017). Previous studies indicated that MDA content was increased and anti-oxidant enzymes were decreased in heat-stressed broilers (Cheng et al. Citation2018; Cheng et al. Citation2019c). In this study, dietary COS supplementation decreased MDA content in serum, liver and spleen. Consistent with our results, former studies also demonstrated that dietary COS supplementation decreased MDA content in jejunum and ileum mucosa (Li et al. Citation2017; Li et al. Citation2019). In the process of anti-oxidant defense functions, liver was the major organ for synthesising the anti-oxidant enzymes. Yang et al. (Citation2010) indicated that acute heat stress-induced overproduction of ROS, increased MDA content, SOD, GSH-Px and CAT activity in liver. Seven et al. (Citation2009) indicated that heat stress increased liver MDA content and CAT activity, but decreased GSH-Px activity. Zhang et al. (Citation2018) indicated that heat stress-induced higher spleen MDA content and lower T-AOC, T-SOD, and CAT activities. In this study, dietary COS supplementation linearly increased serum GSH-Px activity, liver SOD activity, as well as a linearly increasing trend of spleen GSH-Px activity. The improvement of anti-oxidant enzymes and decreased MDA content in liver and spleen, indicating that the anti-oxidant capacity of liver and spleen were enhanced by COS supplementation under high ambient temperature. Similar results also reported in rat, which demonstrated that COS can prevent different stress-induced oxidative stress in liver and spleen (Qiao et al. Citation2011; Lan et al. Citation2019; Lan et al. Citation2019). The COS can work as anti-oxidant due to its anti-oxidant capacity and ROS scavenging property (Zou et al. Citation2016), the improved anti-oxidant capacity in this study is primarily due to the anti-oxidant capacity of COS.
Inflammation was induced by the innate immune system in response to tissue damage or invade pathogens. In response to heat stress, the levels of pro-inflammatory cytokines increased, which may result in haemorrhage and necrosis in liver and spleen (Jang et al. Citation2014; Ohtsu et al. Citation2015). Former studies indicated that the pro-inflammatory cytokines production were associated with the overproduction of ROS under heat stress (Jang et al. Citation2014). In this study, dietary COS supplementation linearly decreased serum IL-1β and TNF-α levels, linearly increased IL-10 level, and a linearly increasing trend in liver and spleen IL-10 level. Bits of cytokines may serve as cell-signaling molecules, which activated nuclear factor kappa B signal pathway, hence promoting the production of cytokines (Xu et al. Citation2018). Our results indicated that high ambient temperature-induced inflammation was repressed with COS supplementation. However, the results were not always inconsistent, Lan et al. (Citation2019) indicated that dietary COS supplementation increased IL-10 and decreased IL-1β concentration in the liver of heat-stressed rats (Lan et al. Citation2019). Lan et al. (Citation2019) also reported that dietary COS supplementation had no significant effects on inflammatory cytokines concentration in the liver and spleen of H2O2-challenged rat. Qiao et al. (Citation2011) demonstrated that dietary COS supplementation decreased serum IL-1β and TNF-α levels in LPS-challenged mice. The inconsistent results indicated that the effects of COS on inflammation response were related to many factors, including species, stressor types, and environmental condition.
Conclusions
In conclusion, supplementation COS at 200 mg/kg of diet alleviates oxidative stress and inflammation response in broilers exposed to the high ambient temperature. These promising effects may exert through decreased MDA content, increasing SOD and GSH-Px activities, and IL-10 level in vital organs.
Ethical approval
The experimental protocol used in this study was approved by the Animal Care and Use Committee of Guangdong Ocean University (SYXK-2018-0147).
Acknowledgements
Great thanks to Wen Gao, who gives help in broilers management.
Disclosure statement
No potential conflict of interest was reported by the author(s).
Additional information
Funding
References
- Abdel-Misih SR, Bloomston M. 2010. Liver anatomy. Surg Clin North Am. 90(4):643–653.
- Abdel-Wareth AA, Hammad S, Khalaphallah R, Salem WM, Lohakare J. 2019. Synbiotic as eco-friendly feed additive in diets of chickens under hot climatic conditions. Poult Sci. 98(10):4575–4583.
- Abdul-Careem M, Hunter D, Lambourne M, Barta J, Sharif S. 2007. Ontogeny of cytokine gene expression in the chicken spleen. Poult Sci. 86(7):1351–1355.
- Alishah AS, Daneshyar M, Aghazadeh A. 2013. The effect of dietary sumac fruit powder (Rhus coriaria L.) on performance and blood antioxidant status of broiler chickens under continuous heat stress condition. Ital J Anim Sci. 12:39–43.
- Ando M, Katagiri K, Yamamoto S, Wakamatsu K, Kawahara I, Asanuma S, Usuda M, Sasaki K. 1997. Age-related effects of heat stress on protective enzymes for peroxides and microsomal monooxygenase in rat liver. Environ Health Persp. 105(7):726–733.
- Attia YA, Hassan RA, Tag El-Din AE, Abou-Shehema BM. 2011. Effect of ascorbic acid or increasing metabolizable energy level with or without supplementation of some essential amino acids on productive and physiological traits of slow-growing chicks exposed to chronic heat stress. J Anim Physiol an N. 95(6):744–755.
- Azad M, Kikusato M, Maekawa T, Shirakawa H, Toyomizu M. 2010. Metabolic characteristics and oxidative damage to skeletal muscle in broiler chickens exposed to chronic heat stress. Comp Biochem Phys A. 155(3):401–406.
- Cheng K, Song Z, Li S, Yan E, Zhang H, Zhang L, Wang C, Wang T. 2019a. Effects of resveratrol on intestinal oxidative status and inflammation in heat-stressed rats. J Therm Biol. 85:102415.
- Cheng K, Song Z, Zheng X, Zhang H, Zhang J, Zhang L, Zhou Y, Wang T. 2017. Effects of dietary vitamin E type on the growth performance and antioxidant capacity in cyclophosphamide immunosuppressed broilers. Poult Sci. 96(5):1159–1166.
- Cheng K, Yan E, Song Z, Li S, Zhang H, Zhang L, Wang C, Wang T. 2019b. Protective effect of resveratrol against hepatic damage induced by heat stress in a rat model is associated with the regulation of oxidative stress and inflammation. J Therm Biol. 82:70–75.
- Cheng Y, Chen Y, Chen R, Su Y, Zhang R, He Q, Wang K, Wen C, Zhou Y. 2019c. Dietary mannan oligosaccharide ameliorates cyclic heat stress-induced damages on intestinal oxidative status and barrier integrity of broilers. Poult Sci. 98(10):4767–4776.
- Cheng Y, Du M, Xu Q, Chen Y, Wen C, Zhou Y. 2018. Dietary mannan oligosaccharide improves growth performance, muscle oxidative status, and meat quality in broilers under cyclic heat stress. J Therm Biol. 75:106–111.
- Coble D, Redmond S, Hale B, Lamont S. 2011. Distinct lines of chickens express different splenic cytokine profiles in response to Salmonella Enteritidis challenge. Poult Sci. 90(8):1659–1663.
- Dufour DR, Lott JA, Nolte FS, Gretch DR, Koff RS, Seeff LB. 2000. Diagnosis and monitoring of hepatic injury. I. Performance characteristics of laboratory tests. Clin Chem. 46(12):2027–2049.
- Hall ME, Blount JD, Forbes S, Royle NJ. 2010. Does oxidative stress mediate the trade-off between growth and self-maintenance in structured families? Funct Ecol. 24(2):365–373.
- Huang RL, Yin YL, Wu GY, Zhang YG, Li TJ, Li LL, Li MX, Tang ZR, Zhang J, Wang B, et al. 2005. Effect of dietary oligochitosan supplementation on ileal digestibility of nutrients and performance in broilers. Poult Sci. 84(9):1383–1388.
- Jang IS, Ko YH, Moon YS, Sohn SH. 2014. Effects of vitamin C or E on the pro-inflammatory cytokines, heat shock protein 70 and antioxidant status in broiler chicks under summer conditions. Asian Australas J Anim Sci. 27(5):749–760.
- Je JY, Park PJ, Kim SK. 2004. Free radical scavenging properties of hetero-chitooligosaccharides using an ESR spectroscopy. Food Chem Toxicol. 42(3):381–387.
- Jiang F, Gao Y, Dong C, Xiong S. 2018. ODC1 inhibits the inflammatory response and ROS-induced apoptosis in macrophages. Biochem Biophys Res Commun. 504(4):734–741.
- Kim DW, Mushtaq MMH, Parvin R, Kang HK, Kim JH, Na JC, Hwangbo J, Kim JD, Yang CB, Park BJ, et al. 2015. Various levels and forms of dietary α-lipoic acid in broiler chickens: Impact on blood biochemistry, stress response, liver enzymes, and antibody titers. Poult Sci. 94(2):226–231.,
- Kong SZ, Li JC, Li SD, Liao MN, Li CP, Zheng PJ, Guo MH, Tan WX, Zheng ZH, Hu Z. 2018. Anti-aging effect of chitosan oligosaccharide on d-galactose-induced subacute aging in mice. Mar Drugs. 16(6):181–194.
- Lan R, Chang Q, An L, Zhao Z. 2019. Dietary Supplementation with Chitosan Oligosaccharides Alleviates Oxidative Stress in Rats Challenged with Hydrogen Peroxide. Animals. 10(1):55–67.
- Lan R, Li S, Chang Q, Zhao Z. 2019. Chitosan Oligosaccharides Protect Sprague Dawley Rats from Cyclic Heat Stress by Attenuation of Oxidative and Inflammation Stress. Animals. 9(12):1074–1086.
- Lara LJ, Rostagno MH. 2013. Impact of heat stress on poultry production. Animals (Basel)). 3(2):356–369.
- Li J, Cheng Y, Chen Y, Qu H, Zhao Y, Wen C, Zhou Y. 2019. Dietary Chitooligosaccharide Inclusion as an Alternative to Antibiotics Improves intestinal morphology, barrier function, antioxidant capacity, and immunity of broilers at early age. Animals. 9(8):404–493.
- Li X, Ding X, Peng X, Chi X, Cui H, Zuo Z, Fang J. 2017. Effect of chitosan oligosaccharides on antioxidant function, lymphocyte cycle and apoptosis in ileum mucosa of broiler. Kafkas Univ Vet Fak Derg. 23:571–577.
- Li X, Piao X, Kim S, Liu P, Wang L, Shen Y, Jung S, Lee H. 2007. Effects of chito-oligosaccharide supplementation on performance, nutrient digestibility, and serum composition in broiler chickens. Poult Sci. 86(6):1107–1114.
- Liu L, He J, Xie H, Yang Y, Li J, Zou Y. 2014. Resveratrol induces antioxidant and heat shock protein mRNA expression in response to heat stress in black-boned chickens. Poult Sci. 93(1):54–62.
- Miao H, Li MJ, Yan Y. 2007. Protective Effect of Chitosan oligosaccharide against liver damage induced by carbon tetrachloride. Food Drug. 5:178–184.
- Nourozi E, Danesyar M, Farhoomand P. 2013. Dietary supplementation effects of zinc acetate and magnesium sulfate on performance and antioxidant status of broilers under continuous heat stress. Span J Agric Res. 11(1):127–131.
- Ohtsu H, Yamazaki M, Abe H, Murakami H, Toyomizu M. 2015. Heat stress modulates cytokine gene expression in the spleen of broiler chickens. Jpn Poult Sci. 52(4):282–287.
- Qiao Y, Bai XF, Du YG. 2011. Chitosan oligosaccharides protect mice from LPS challenge by attenuation of inflammation and oxidative stress. Int Immunopharmacol. 11(1):121–127.
- Quinteiro-Filho WM, Ribeiro A, Ferraz-de-Paula V, Pinheiro M, Sakai M, Sá LRMD, Ferreira AJP, Palermo-Neto J. 2010. Heat stress impairs performance parameters, induces intestinal injury, and decreases macrophage activity in broiler chickens. Poult Sci. 89(9):1905–1914.
- Sahin K, Orhan C, Tuzcu M, Ali S, Sahin N, Hayirli A. 2010. Epigallocatechin-3-gallate prevents lipid peroxidation and enhances antioxidant defense system via modulating hepatic nuclear transcription factors in heat-stressed quails. Poult Sci. 89(10):2251–2258.
- Sakka SG. 2007. Assessing liver function. Curr Opin Crit Care. 13(2):207–214.
- Seven PT, Yılmaz S, Seven I, Cercı IH, Azman MA, Yılmaz M. 2009. Effects of propolis on selected blood indicators and antioxidant enzyme activities in broilers under heat stress. Acta Vet Brno. 78(1):75–83.
- Song Z, Cheng K, Zhang L, Wang T. 2017. Dietary supplementation of enzymatically treated Artemisia annua could alleviate the intestinal inflammatory response in heat-stressed broilers. J Therm Biol. 69:184–190.
- Sun B, Karin M. 2013. Inflammation and liver tumorigenesis. Front Med. 7(2):242–254.
- Tessari EN, Kobashigawa E, Cardoso ALS, Ledoux DR, Rottinghaus GE, Oliveira CA. 2010. Effects of aflatoxin B1 and fumonisin B1 on blood biochemical parameters in broilers. Toxins. 2(4):453–460.
- Tufan T, Arslan C, Sari M, Önk k, Deprem T, Celik E. 2015. Effects of Chitosan oligosaccharides addition to Japanese quail’s diets on growth, carcass traits, liver and intestinal histology, and intestinal microflora. Kafkas Univ Vet Fak Derg. 21:665–671.
- Wang W, Yan F, Hu J, Amen O, Cheng H. 2018. Supplementation of Bacillus subtilis-based probiotic reduces heat stress-related behaviors and inflammatory response in broiler chickens. J Anim Sci. 96(5):1654–1666.
- Xia F, Wang C, Jin Y, Liu Q, Meng Q, Liu K, Sun H. 2014. Luteolin protects HUVECs from TNF-α-induced oxidative stress and inflammation via its effects on the Nox4/ROS-NF-κB and MAPK pathways. J Atheroscler Thromb. 21:23697.
- Xiong X, Yang HS, Wang XC, Hu Q, Liu CX, Wu X, Deng D, Hou YQ, Nyachoti CM, Xiao DF, et al. 2015. Effect of low dosage of chito-oligosaccharide supplementation on intestinal morphology, immune response, antioxidant capacity, and barrier function in weaned piglets. J Anim Sci. 93(3):1089–1097.
- Xu YQ, Xing YY, Wang ZQ, Yan SM, Shi BL. 2018. Pre-protective effects of dietary chitosan supplementation against oxidative stress induced by diquat in weaned piglets. Cell Stress Chaperones. 23(4):703–710.
- Yang L, Tan GY, Fu YQ, Feng JH, Zhang MH. 2010. Effects of acute heat stress and subsequent stress removal on function of hepatic mitochondrial respiration, ROS production and lipid peroxidation in broiler chickens. Comp Biochem Physiol C Toxicol Pharmacol. 151(2):204–208.
- Zeng T, Li JJ, Wang DQ, Li GQ, Wang GL, Lu LZ. 2014. Effects of heat stress on antioxidant defense system, inflammatory injury, and heat shock proteins of Muscovy and Pekin ducks: evidence for differential thermal sensitivities. Cell Stress Chaperon. 19(6):895–901.
- Zhang C, Chen K, Zhao X, Geng Z. 2018. Protective effects of resveratrol against high ambient temperature-induced spleen dysplasia in broilers through modulating splenic redox status and apoptosis. J Sci Food Agric. 98(14):5409–5417.
- Zhou T, Chen Y, Yoo J, Huang Y, Lee J, Jang H, Shin S, Kim H, Cho J, Kim I. 2009. Effects of chitooligosaccharide supplementation on performance, blood characteristics, relative organ weight, and meat quality in broiler chickens. Poult Sci. 88(3):593–600.
- Zou P, Yang X, Wang J, Li Y, Yu H, Zhang Y, Liu G. 2016. Advances in characterisation and biological activities of chitosan and chitosan oligosaccharides. Food Chem. 190:1174–1181.