Abstract
To assess the effect of dietary supplement levels of chromium-yeast (Cr-yeast) on growth performance, blood glucose and triglycerides, fatty acid (FA) profile in intramuscular fat, carcase and meat traits, iron, copper, chromium and zinc concentrations in liver and bone, 24 Rambouillet male lambs (29.2 ± 0.17 kg body weight) were randomly assigned to four diets with 0, 0.2, 0.4 and 0.6 mg Cr/kg DM. The growth performance trial lasted 49 d. Supplemental Cr-yeast did not affect growth performance and carcase characteristics (p > .05), but reduced (p < .05) perirenal and intramuscular fat, as well as 3 h post-feeding blood glucose and triglycerides concentration. In liver, Fe and Cu concentration decreased (p < .05), while Cr concentrations in liver increased with increasing Cr-yeast dietary levels. In bone, Fe decreased (p < .05) as Cr-yeast dietary levels increasing, and Cr-yeast supplementation increased Cr concentrations (p < .05). As Cr-yeast dietary level increased, palmitic (C16:0) and stearic (C18:0) SFA decreased linearly (p < .05), while palmitoleic (C16:1n-7), vaccenic (C18:1n-7), linoleic (C18:2n-6) and arachidic (C20:4) unsaturated fatty acids (UFA) increased linearly (p < .01). In conclusion, Cr-yeast did not affect growth performance and carcase quality, but decreased the perirenal and intramuscular fat, blood glucose and triglyceride content, and Fe and Cu concentrations in liver as increased Cr-yeast levels in the diet. Because supplemental Cr-yeast improved index of atherogenicity and unsaturated to saturated FA ratio in muscle of lambs, it could be of human nutritional interest.
Cr-yeast supplementation reduced blood glucose and triglyceride concentration.
In liver, Fe and Cu content decreased while Cr increased with increasing Cr-yeast dietary levels.
Cr-yeast modified the FA profile of human nutritional interest and improved the unsaturated to saturated FA ratio in intramuscular fat.
HIGHLIGHTS
Introduction
Chromium (Cr) is an essential micronutrient. It is an integral part of the glucose tolerance factor (GTF) which increases adhesion of insulin to the cell membrane surface and permits entry of glucose, fatty acids and amino acids into the cell (Mertz Citation1969; Anderson Citation1998), due to the binding of this (chromodulin) with the transmembrane insulin receptors (Evans and Bowman Citation1992) specifically to the α-subunits, converting it into an autokinase by the phosphorylation of the β-subunits (Vincent Citation2013).
Although Cr is vital for the efficient function of the insulin and nutrient metabolism (Mertz Citation1993) in humans and farm animals, dietary ingestion of Cr is often less than the recommended dose (0.2 mg Cr/kg DM) (Anderson Citation1998; National Research Council Citation2005). Growth hormone (GH) and insulin growth factor (IGF) are involved in the metabolism of Cr (Mowat Citation1997); however in ruminants, Cr has little impact on growth (Kegley et al. Citation1997; Haldar et al. Citation2007), despite it improves the capacity response of the immune system (Haldar et al. Citation2009); in poultry and pigs, Cr supplementation has better positive effects on metabolic response, distribution of nutrients and carcase characteristics, maybe due to an increased sensitivity to insulin and efficiency in the use of glucose (Brockman and Laarveld Citation1986). The magnitude of these effects depends on the chemical form, concentration and dietary contribution of Cr (Galip Citation2006; Yan et al. Citation2008; Domínguez-Vara et al. Citation2009; Arvizu et al. Citation2011). In this regard, trivalent Cr cannot pass through the cell membrane, so its binding to ligands is necessary to activate it (Burton Citation1995); also some forms of inorganic Cr are not available for absorption (Mordenti et al. Citation1997), in contrast organic sources are more bioavailable.
In sheep and cattle in feedlots, dietary levels of 0.2–1.2 mg Cr/kg DM have been assessed, and dose equal to or greater than 0.35 mg Cr/kg DM had effect mainly on blood glucose level but its effect on the content of fatty acids has been little evaluated (Yan et al. Citation2008; Domínguez-Vara et al. Citation2009; Soltan et al. Citation2012; Estrada-Angulo et al. Citation2013). However, supplementation of organic Cr (Cr-yeast) in growing steers has minimal effects on the rate of blood glucose elimination and the half-life of blood glucose (Swanson et al. Citation2000). In addition, there is little information on the effect of concentrations of Cr enriched yeast supplements on fatty acids content, meat quality, or metabolism of the microminerals Fe, Cu, and Zn in sheep fed high energy diets. This study evaluated the effect of supplementing different doses of dietary organic Cr (Cr-yeast) on growth performance, blood metabolisms, carcase and meat characteristics and muscular fatty acids profile of fattening lambs.
Material and methods
Feed and experimental animals
Twenty-four 3.5 months-old male Rambouillet lambs (29.2 ± 0.17 kg body weight) were used, they were dewormed, vaccinated and housed in individual pens (1.5 × 1.2m) for a 15d adaptation period. Lambs were fed a basal diet (BD) [Table ] formulated according to the requirements of the finishing sheep (National Research Council Citation2007). Basal diet offered was recorded daily and feed samples were taken weekly, dried at 55 °C for 48 h to determine their chemical composition (Table ). Dry matter (method 967.03), ash (method 942.05) and crude protein (Kjeldahl procedure, method 976.06, N × 6.25), were determined by official methods (AOAC Citation2012). Neutral detergent fibre (NDF) was analysed according to Van Soest et al. (Citation1991) with a modified ANKOM 200 fibre analyser with a heat-stable amylase (ANKOM Tech. Corp, Fairport, NY, USA). Metabolisable energy was calculated from diet ingredient composition (National Research Council Citation2007).
Table 1. Composition and calculated chemical analysis of the basal diet (BD).
Treatments and animal measurements
Lambs were randomly assigned to four experimental diets (treatments) with 0, 0.2, 0.4 and 0.6 mg Cr-yeast per kg DM in the basal diet. Chromium yeast was Bio-Chrome® (Co-Factor III Cr3+, Alltech, Nicholasville, KY, USA), which according to the manufacturer, the product contains 1000 mg Cr/kg. Chromium was supplied daily and mixed with on top food served at 0800 h. Feed was offered ad libitum twice at 0800 and 1600 h. Feed offered and refused was recorded daily. Body weight was recorded at the start of fattening and every two weeks thereafter. On days 14, 35, and 49, three h after the morning feeding, blood samples (7 mL) were collected from jugular vein in vacutainer tubes with sodium fluoride or potassium oxalate. The blood samples were centrifuged and stored at −20 °C until analysis for glucose and triglycerides (Kitchalong et al. Citation1995) by semiautomatic equipment (Vitros DT60 II-Johnson and Johnson Co).
Post-mortem evaluation
After 49 d of Cr-yeast supplementation, lambs were slaughtered and the handling procedures were according the official guidelines for animal care in Mexico (NOM-051-ZOO-1995). Post-slaughter and chilled (4 °C), cold carcase weight was recorded 24 h after slaughter. Carcase conformation, fatness degree, and perirenal fat measurements were based on a photographic reference diagram and assessed following Colomer-Rocher method (Colomer-Rocher Citation1984). Incisions at 12th rib were done to measure the backfat thickness with a digital Vernier (Rust et al. Citation1970). Liver (150 g) and rib bone (100 g) samples were collected to determine Fe, Cu, Cr and Zn concentration (Table ) by atomic absorption spectroscopy (Perking Elmer, 3110) (Fick et al. Citation1979). Two-hundred g of Longissimus dorsi were collected and pH was measured (Oakton, Vernon Hills, IL, USA) (Honikel Citation1997).
Muscle colour measurements were made with a calibrated chromameter (Minolta CR-200 Osaka Japan); samples of the Longissimus thoracis was extracted at the last rib level, according to the recommendations of the American Meat Science Association (Hunt et al. Citation1991). Illuminant A was used to detect differences in redness in the meat samples between the applied treatments. The 10° observer was used for meat colour measurement. The following coordinates were determined: Luminosity (L*), reddish (a*, red ± green) and yellowish (b*, yellow ± blue) (American Meat Science Association Citation2012).
Tenderness was measured using a Warner-Bratzler shear test on a TAX-T2 texture analyser (Texture Technologies Corp, Scarsdale, NY, USA) (Beltrán and Roncalés Citation2000). Chemical composition (humidity, crude protein, fat and ash) and total collagen was quantified following AOAC official methods (AOAC Citation2012).
Fat was extracted and purified following AOAC official method 991.36 (AOAC Citation2012), Bligh and Dyer (Citation1959) and Soto-León et al. (Citation2014) procedures, using a 2:1 chloroform-methanol solution, 10 g of ground meat was suspended using a 10:1 solvent:meat ratio. The suspension was exposed to ultrasonic-irradiation for 60 min by using a high efficiency ultrasonic processor (UP200S, Hielscher Ultrasonics) with 200 W of output power and 24 kHz of frequency. The acoustic potency was transmitted using a sonotrode (S14, Hielscher Ultrasonics). After the solution was adjusted to a 2:1:0.8 chloroform:methanol:water proportion, it was mixed and vacuum filtered. The liquid phase was readjusted to a 2:2:1.8 chloroform:methanol:water proportion, transferred to a separation funnel and after sitting for 24 h, the non-aqueous phase was recovered. The fat-chloroform phase was recovered and chloroform was removed using a vacuum rotator evaporator machine (RE300, Yamato) at 45 °C. Thereafter, 300 μL of KOH and 10 mL of methanol were added and ultrasonic irradiation was applied for 60 s. Methanol was evaporated at 50 °C, and 10 mL of hexane was added and filtered with Whatman paper with a pore size of 0.22 μm and stored in chromatographic vials.
Fatty acids were separated in a capillary column 100 m × 0.25 mm inner diameter × 0.2 μm of film thickness (SUPELCO TM-2560), and determined by gas chromatography (Perkin Elmer, Clarus 500 model), in which Helium was used as the carrier gas in an Omega Wax 250 column; a volume of 1 μL was injected into the gas chromatographer, with a flux of 1 mL/min; column temperature ranged from 50 to 270 °C (10 min), with a heating rate of 5 °C/min. column temperature ranged from 50 to 270 °C (10 min), with a heating rate of 5 °C/min and a 37-component standard (SUPELCO, TM-2560) was used as a reference.
The retention times were compared with known standards (Supelco 37 Component FAME Mix, SIGMA USA). The results of the fatty acid concentration (FA) are expressed in g/100 g of FA, which correspond to the area under the curve of the detected fatty acid peaks.
Statistical analysis
Data were analysed with MIXED procedure (SAS Institute Inc. Citation2004) under a completely randomised design with six lambs per treatment using a mixed model that included lamb (random), Cr-yeast supplementation level (fixed), and residual (lamb within treatment) with ANTE(1) covariance structure. The first-order ante dependence covariance structure (ANTE) allows unequal variances over time and unequal correlations and covariance among different pairs of measurements. Average daily weight gain, feed intake, feed conversion, and blood glucose and triglyceride levels were analysed using the same model included effect of time (repeated) and the time interaction with Cr-yeast supplementation level effect in the model. Levels of Cr-yeast supplementation (treatments) were partitioned into linear, quadratic, and cubic orthogonal polynomials for four equally spaced levels with the statements LSMEANS and ESTIMATE. Categorical data (conformation, fatness degree, and perirenal fat) were analysed using the SAS NPAR1WAY procedure (SAS Institute Inc. Citation2004), which performs nonparametric tests for location and scale differences across a one-way classification, and also provides a standard analysis of variance on the raw data and tests based on the empirical distribution function. With the data of the serum concentrations of triglycerides and glucose in the different treatments (Cr levels in the diet), collected over time (weeks), regression analysis were performed to develop prediction equations. The Tukey’s test (p ≤ .05) was used to assess differences between treatments means.
Results
Growth performance, blood metabolites, carcase characteristics and meat quality
Chromium yeast did not affect any of the growth performance variables (p > .05); there were also no effects of time or of the interaction treatments with time (p > .05). There was also no effect of Cr on carcase characteristics (p > .05), except for total collagen concentration which was increased linearly (p = .01) as Cr-yeast level in the diet increased. At 14 and 35 d, 3 h post-feeding, Cr-yeast did not affect blood glucose and triglycerides concentrations (p > .05), but at 49 d post feeding, blood glucose and triglycerides concentration were reduced quadratically (p = .04 and p = .01) as Cr-yeast level in the diet increased. Intramuscular fat and ash in meat decreased (p = .01 and p = .03) quadratically and linearly, respectively, while the perirenal fat decreased linearly (p = .02) with increased Cr-yeast dietary (Table and Figure ).
Figure 1. Effects of Cr-yeast supplementation levels at 49 days, 3 h post-feeding, on blood glucose and triglycerides concentrations in fattening lambs.
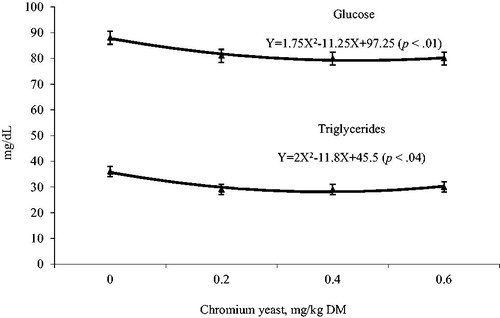
Table 2. Effect of Cr-yeast on growth performance, blood metabolites, carcase and meat quality of fattening lambs.
Fatty acids composition
Chromium yeast supplementation did not affect (p > .05) myristic (C14:0), pentadecanoic (C15:0) and margaric (C17:0) SFA or oleic (C18:1n-9) MUFA contents. However, as Cr-yeast supplementation was increased in the diet, palmitic (C16:0) and stearic (C18:0) SFA content was linearly decreased (p = .03 and p = .01); in addition palmitoleic (C16:1n-7) and vaccenic (C18:1n-7) MUFA content (p = .04 and p = .05) and linoleic (C18:2n-6) and arachidic (C20:4) PUFA content (p = .04 and p = .01) was linearly increased (p < .01). Thus, there was a linear decrease (p = .05) of the SFA:UFA ratio and quadratic decrease (p = .05) of the index of atherogenicity in intramuscular fat of Longissimus dorsi as the level of Cr-yeast in the diet increased (Table ).
Table 3. Effect of Cr-yeast on fatty acid profile (g/100 g of FA) of intramuscular fat in Longissimus dorsi of fattening lambs.
Micromineral concentrations in liver and bone
In liver, Fe and Cu concentrations quadratically decreased (p = .04 and p = .01) as Cr-yeast level increased in the diet. In contrast, Cr concentrations in liver linearly increased (p < .05) as Cr-yeast level increased in the diet. Cr-yeast did not affect Zn concentration in liver (p = .91). In bone, Fe was linearly (p = .02) reduced as Cr-yeast increased in the diet. Moreover, Cr-yeast supplementation quadratically increased Cr concentration (p = .05), and Cr-yeast did not affect Zn concentration in bone (p = .19) [Table ].
Table 4. Effect of Cr-yeast on Fe, Cu, Cr and Zn concentration in liver and bone of fattening lambs.
Discussion
Results of studies on the effects of Cr-yeast supplementation on growth performance of lambs have been variable and sometimes inconsistent. While, some studies found no effect (Arvizu et al. Citation2011; Moreno-Camarena et al. Citation2015), others found positive effects on average daily BW gain (Domínguez-Vara et al. Citation2009; Estrada-Angulo et al. Citation2013). The reduction of glucose and triglycerides found in our study coincides with previous studies (Kitchalong et al. Citation1995; Yan et al. Citation2008) in lambs and goats, suggesting that Cr can improve glucose tolerance by increasing insulin sensitivity (Haldar et al. Citation2007) and hepatic triglycerides transport capacity and promote triglyceride catabolism by increasing lipoprotein lipase activity in plasma (Yan et al. Citation2008), with effects on lipolytic activity in adipose tissue and protein synthesis (Page et al. Citation1993; Min et al. Citation1997). In the present study, Cr-yeast reduced perirenal fat deposition, intramuscular fat content, and saturated FA in sheep meat fat. The effect of Cr-yeast on increasing insulin sensitivity may explain the increment of collagen content found in our results, but no other studies have reported similar findings like these and and its impact on meat quality of sheep. As a cofactor of insulin, Cr acts on carcase traits mainly by influencing insulin sensitivity, which is closely relates to carbohydrate and protein metabolism (Mertz Citation1993; Vincent Citation2000), and therefore the synthesis of muscle protein (Okada et al. Citation1984). In finishing pigs supplemented with 200 ppb of Cr from chromium picolinate (Cr-Pic) Page et al. (Citation1993) decreased backfat thickness and increased lean tissue accretion possibly due to enhancement of lipolysis and protein synthesis.
There are few studies on the effect of Cr supplementation on FA profiles of the meat of growing-finishing lambs in feedlot system. In this regard, Kitchalong et al. (Citation1995) found that plasma NEFA content was lower and cholesterol was 17% lower in lambs fed Cr-Pic (0.25 mg Cr/kg DM); the supplementation of Cr-yeast (0.3 mg Cr/kg DM) in sheep, results in the decrease of serum concentration of triglycerides (Zhou et al. Citation2013). Rodríguez-Gaxiola et al. (Citation2020) found that supplementing 0.3 mg of Cr/kg DM, from Cr-yeast, in the Rambouillet sheep fattening diet, reduced intramuscular fat of meat, but this level of dietary Cr-yeast did not have a greater effect on the FA profile of the intramuscular fat of the Longissimus dorsi muscle. Ghassemi et al. (Citation2016) concluded that chromium methionine (Cr-Met) supplementation (0.4 mg/kg DM) to fattening Holstein steers improved beef quality by increasing PUFA and gamma-linoleate FA compositions of beef. In pigs only the total content of monounsaturated FA of the loin was increased by the interaction of Cr-yeast (0.2 mg Cr/kg DM) and glycemic index of the diet (Lemme et al. Citation2000).
In the present study, supplementation with Cr-yeast linearly reduced the proportion of saturated FA and linearly increased the content of monounsaturated and polyunsaturated FA in the intramuscular fat of sheep meat which are of interest in the nutritional quality of sheep meat for human health; moreover, Cr-yeast decreased plasma triglycerides content and also improved the UFA:SFA ratio in intramuscular fat of the Longissimus dorsi. Natural trans-18:1 isomer is present in all ruminant milk and meat fats as a result of biohydrogenation by rumen bacteria of dietary PUFA (Wolff Citation1995). According to Lemme et al. (Citation2000) and Song et al. (Citation2018), the high concentration of energy, with high and rapid availability of carbohydrates, like starch, as in the diet of the present study, produces a strong stimulation of insulin secretion due to the diet, which can increase the carcase fat deposition and influence the content of UFA deposited in intramuscular fat, with a positive effect on the degree of meat marbling; furthermore, Cr has been shown to potentiate the action of insulin and therefore its effect on lipid metabolism (Vincent Citation2007). The higher UFA synthesis due to Cr-yeast supplementation is in agreement with the study of Lien et al. (Citation1998) where Cr-Pic (0.2 mg Cr/kg DM) supplemented to pigs increased of the activity of adipose tissue lipogenesis related enzymes such as FA synthetase, ATP-citrate cleavage enzyme, NADP-malic dehydrogenase and increased the activity of adipose tissue lipoprotein lipase and serum lecithin-cholesterol acyltransferase.
In ruminants, the complex mixture of dietary lipids (phosphoglycerides and glycosylglycerides, waxes, pigments and cutin), from forages usually at levels of 30–40 g kg/DM, and from cereal grains varying widely in lipid content 20–70 g kg/DM. In herbage the lipid occurs mainly as mono- and digalacto-1,2-diacylglycerides, whereas in cereal grains most of the lipids are in the form of triacylglycerides (TAG). Both classes of lipids contain high proportions of C:18 unsaturated fatty acids; linolenic acid predominates in galactolipids and linoleic acid in cereal lipids (Annison et al. Citation2002). In the rumen, all classes of lipids are rapidly hydrolysed by bacterial lipases, with the liberation of free long-chain fatty acids (LCFA), a high proportion of which are unsaturated. For sheep on forage diets, intakes of LCFA are low, about 12–16 g/day. These fatty acids undergo extensive biohydrogenation, with the production of a range of cis and trans positional isomers of octadecenoic acid. The extent of ruminal biohydrogenation of dietary linolenic and linoleic acids is 85–100% and 70–95%, respectively; the lower value for linoleic acid being attributed to the uptake of this acid by bacteria (Bauchart et al. Citation1990). The first step in the biohydrogenation of linoleic acid in the rumen is isomerisation to cis-9-, trans-11-octadecenoic acid, known as rumenic acid and main isomer of conjugated linoleic acid (CLA). CLA is a mixture of geometrical and positional isomers of linoleic acid, with conjugated bond located from positions 2–17 of the carbonate chain (Buccioni et al. Citation2012). Most of the CLA is hydrogenated to stearic acid, but small amounts escape hydrogenation and reach the small intestine (SI), where CLA is absorbed and incorporated into adipose tissue and milk fat, together with other fatty acids absorbed from the SI. CLA acid has certain biological properties, which include inhibition of carcinogenesis and the reduction of carcase fat deposition and milk fat production (Dunshea and Ostrowska Citation1999).
In ruminants, the extent to which dietary UFA escape to the hydrogenation seems to depend on microbial growth conditions that influence rates of lipolysis and biohydrogenation (Jenkins Citation1993). Feeding high grain diets decreases ruminal biohydrogenation and its promotes increased unsaturation of carcase fat (Kemp et al. Citation1980), this can be due to the effect of less lipolysis, resulting from low ruminal pH (Latham et al. Citation1972). Palmquist and Schanbacher (Citation1991) reported that high corn diets fed to lactating cows increased ruminal formation of trans-CI8:1 with enrichment of this FA isomer in milk fat. LeDoux et al. (Citation2002) indicated that by increasing the proportion of forage in the diet of dairy goats it reduced the content in trans-C18:1 FA in milk fat, but vaccenic acid remained the major component and represented from 28 to 45% of total trans-C18:1 FA.
Trans FA occurrence in milk and meat is partly a consequence of rumen microbial activity. Rumen flora metabolises PUFA, such as linoleic (C18:2) and linolenic (C18:3) acids from the diet, to stearic acid (C18:0) via a trans-C18:1 acid formation step. Linoleic acid is biohydrogenated to stearic acid via conjugated linoleic acid and trans-C18:1 formation. Linolenic acids, which are not direct precursors of conjugated linoleic acid, also increased the yield of ruminal vaccenic acid (trans-11 C18:1) by using another pathway including a step with production of conjugated C18:3 acids (Chilliard et al. Citation2000).
According to LeDoux et al. (Citation2002) decreasing the fibre content and increasing the grain of the diet can produce a slow reduction of the last biohydrogenation step of FA, from C18:1 to C18:0, and because of that, increasing the trans-C18:1 acid accumulation. Moreover, high grain diets may favour low ruminal pH, which is also a factor that could result in the inhibition of the last step of PUFA biohydrogenation (Kalscheur et al. Citation1997).
In our study it was found that Cr-yeast increased the proportion of UFA relative to SFA. Effects of Cr supplementation on FA content of intramuscular fat and subcutaneous fat of carcase has been controversial. Few studies on reduction and increment of SFA and UFA by Cr supplementation (levels less than 0.4 mg Cr/kg DM) has been previously reported in pigs (Lien et al. Citation2001; Tian et al. Citation2015), beef (Ghassemi et al. Citation2016) or lambs (Rodríguez-Gaxiola et al. Citation2020). The index of atherogenicity (IA) in intramuscular fat of Longissimus dorsi quadratically decrease (p = .05) as the level of Cr-yeast in the diet increased; the IA indicates the relationship between the sum of SFAs and the sum of unsaturated fatty acids (UFAs). The main classes of SFAs, which include C12:0, C14:0, and C16:0, with the exception of C18:0, are considered pro-atherogenic (they favour the adhesion of lipids to cells of the circulatory and immunological systems). UFAs are considered to be anti-atherogenic as they inhibit the accumulation of plaque and reduce the levels of phospholipids, cholesterol, and esterified fatty acids. Therefore, the consumption of foods or products with a lower IA can reduce the levels of total cholesterol and LDL-C in human blood plasma; in sheep meat, the IA reported ranged from 0.49 to 1.32 (Chen and Liu Citation2020), therefore, the IA values observed in the present study are at the lower limit of the range, which indicates a healthy meat for human consumption; however further research is still needed to examine the lipid metabolism and relationship between Cr-yeast and improvement of the SFA:UFA ratio and IA in intramuscular fat.
The reduction of Fe in liver and bone by Cr-yeast, as a result of possible competition between transporter agents, found in our study had been confirmed previously in humans and laboratory animals (Ani and Moshtaghie Citation1992; Lukaski et al. Citation1996; Anderson et al. Citation1996). Indeed, transferrin is the major physiological chromium and iron transport agent (Vincent Citation2000). Although Cr-yeast reduced Fe concentration in liver (from 685.4 for control to an average of 570.0 mg Fe/kg for Cr-yeast), it was higher than the reference (30–300 mg Fe/kg) values (Puls Citation1994). Cr has antioxidant and reducing capacity over Fe+3, Fe+2, this allows for greater absorption and retention of Fe (Kumar et al. Citation2017; Alhidary et al. Citation2018), which may explain the disappearance of Fe in the liver by increasing up to 0.4 mg Cr/kg DM in this experiment. Similarly, Cr-yeast supplementation reduced Cu concentration in liver (from 196 mg Cu/kg with the control treatment to an average of 169 mg Cu/kg with Cr-yeast), but that reduction was higher than the reference (25–100 mg/kg) values (Puls Citation1994). The interaction of Zn with Cu, Cd and Fe has been studied, but little is known regarding the interaction between the Cr and Cu (Pechová et al. Citation2002). In agreement with our findings, previous studies found that Cr-yeast supplementation has not effect on Zn concentrations in liver and bone (Anderson Citation1989; Chang et al. Citation1992; Amatya et al. Citation2004). However, in camel calves supplemented with Cr-yeast (0.5 mg Cr/kg DM) under hot climatic conditions, the plasma concentration of Cr negatively interacts with Cu, Zn and Mn (Alhidary et al. Citation2018).
As expected, Cr-yeast supplementation increased Cr concentrations in liver (from 2.7 to 3.6 mg Cr/kg) and bone (from 12.9 to 14.2 mg Cr/kg), indicating that more Cr was available in the gut to be absorbed (Olsen et al. Citation1996; Guifen et al. Citation2011). The Cr concentrations in liver are within the reference values (from 0.04 to 3.8 mg Cu/kg DM) (Puls Citation1994).
Conclusions
In conclusion, Cr-yeast supplementation did not affect growth performance and carcase characteristics. However, it did decreased blood glucose and triglycerides, Fe and Cu concentration in liver. Cr-yeast supplementation also increased muscle collagen and Cr concentration in liver and bone. Moreover, Cr-yeast improved the index of atherogenicity and the proportion of unsaturated fatty acids relative to saturated fatty acids in fat of fattening lambs.
Ethical approval
All procedures were reviewed and approved by an Academic Committee of the Facultad de Medicina Veterinaria y Zootecnia of the Universidad Autónoma del Estado de México, and complied with Mexican Government Animal Welfare Regulations.
Acknowledgments
The authors thank to Universidad Autónoma del Estado de México (UAEM) and the Consejo Nacional de Ciencia y Tecnología, México (CONACYT) for the financial support that allowed the present work to be carried out.
Disclosure statement
No potential conflict of interest was reported by the author(s).
References
- Alhidary IA, Alsofi MA, Abdoun KA, Samara EM, Okab AB, Al-Haidary AA. 2018. Influence of dietary chromium yeast supplementation on apparent trace elements metabolism in growing camel (Camelus dromedarius) reared under hot summer conditions. Trop Anim Health Prod. 50(3):519–524.
- Amatya JL, Haldar S, Ghosh TK. 2004. Effects of chromium supplementation from inorganic and organic sources on nutrient utilization, mineral metabolism and meat quality in broiler chickens exposed to natural heat stress. Anim Sci. 79(2):241–253.
- American Meat Science Association. 2012. Meat color measurement guidelines. Champaign (IL): AMSA. p. 125.
- Anderson RA, Bryden NA, Polansky MM, Gautschi K. 1996. Dietary chromium effects on tissue chromium concentrations and chromium absorption in rats. J Trace Elem Exp Med. 9(1):11–25.
- Anderson RA. 1998. Effects of chromium on body composition and weight loss. Nutr Rev. 56(9):266–270.
- Anderson RA. 1989. Essentiality of chromium in humans. Sci Total Environ. 86(1–2):75–81.
- Ani M, Moshtaghie AA. 1992. The effect of chromium on parameters related to iron metabolism. Biol Trace Elem Res. 32:57–64.
- Annison EF, Linsay DB, Nolan JV. 2002. Digestion and metabolism. In: M. Freer and H. Dove editor. Sheep nutrition. Camberra Australia: Cabi Publishing-SCIRO Publishing. p. 95–118.
- AOAC. 2012. Association of official analytical chemists. Official methods of analysis. 19th ed. Washington, D.C.: AOAC: International, USA. p. 34–36.
- Arvizu RR, Domínguez IA, Rubio MS, Bórquez JL, Pinos-Rodríguez JM, González M, Jaramillo G. 2011. Effects of genotype, level of supplementation, and organic chromium on growth performance, carcass, and meat traits grazing lambs. Meat Sci. 88(3):404–408.
- Bauchart DF, Legay-Carmier F, Doreau M, Gaillard B. 1990. Lipid metabolism of liquid-associated and solid-adherent bacteria in rumen contents of dairy cows offered lipid-supplemented diets. Br J Nutr. 63(3):563–578.
- Beltrán JA, Roncalés P. 2000. Determinación de textura. In: Cañeque V, Sañudo C. editor. Metodología para el estudio de calidad de canal y carne en rumiantes. Madrid: Instituto Nacional de Investigación y Tecnología Agraria y Alimentaria. (in Spanish); p. 168–174.
- Bligh EG, Dyer WY. 1959. A rapid method of total lipid extraction and purification. Can J Biochem Physiol. 37(8):911–917.
- Brockman RP, Laarveld B. 1986. Hormonal regulation of metabolism in ruminants a review. Liv Prod Sci. 14(4):313–334.
- Buccioni A, Decandia M, Minieri S, Molle G, Cabiddu A. 2012. Lipid metabolism in the rumen: new insights on lipolysis and bio hydrogenation with an emphasis on the role of endogenous plant factors. Anim Feed Sci Tech. 174(1–2):1–25.
- Burton JL. 1995. Supplemental chromium: it’s benefits to the bovine immune system. Anim Feed Sci Tech. 53(2):117–133.
- Chang X, Mowat DN, Spiers GA. 1992. Carcass characteristics and tissue-mineral contents of steers fed supplemental chromium. Can J Anim Sci. 72(3):663–669.
- Colomer-Rocher F. 1984. Metodología de Clasificación de Canales Ovinas. Madrid (Spain): Oleaginosas Españolas. (in Spanish).
- Chen J, Liu H. 2020. Nutritional indices for assessing fatty acids: a mini review. Int J Mol Sci. 21(16):5695.
- Chilliard Y, Ferlay A, Mansbridge RM, Doreau M. 2000. The ruminant as a supplier of fatty acids for human consumption. Ann Zootech. 49(3):181–205.
- Domínguez-Vara IA, González-Muñoz SS, Pinos-Rodríguez JM, Bórquez-Gastelum JL, Bárcena-Gama R, Mendoza-Martínez G, Zapata LE, Landois-Palencia LL. 2009. Effects of feeding selenium-yeast and chromium-yeast to finishing lambs on growth, carcass characteristics, and blood hormones and metabolites. Anim Feed Sci Tech. 152(1–2):42–49.
- Dunshea FR, Ostrowska E. 1999. Conjugated linoleic acid-snake oil or wonder fat? In: Corbett JL editor. Recent advances in animal nutrition in Australia. Vol. 12. Armidale (Australia): University of New England. p. 159–166.
- Estrada-Angulo A, Valdés YS, Carrillo-Muro O, Castro-Perez BI, Barreras A, López-Soto MA, Plascencia A, Dávila-Ramos H, Rios FG, Zinn RA. 2013. Effects of feeding different levels of chromium enriched live yeast in hairy lambs fed a corn-based diet: effects on growth performance, dietary energetics, carcass traits visceral organ mass. Anim Prod Sci. 53(4):308–315.
- Evans GW, Bowman TD. 1992. Chromium picolinate increases membrane fluidity and rate of insulin internalization. J Inorg Biochem. 46(4):243–250.
- Fick KA, McDowell LR, Miles PH, Wilkinson NS, Funk JD, Conrad JH, Valdivia V. 1979. Métodos de análisis de minerales para tejidos de plantas y animales. Gainesville (FL): University of Florida. p. 135.
- Galip N. 2006. Effects of dietary Saccharomyces cerevisiae live yeast culture supplementation on animal digestion and protozoa count in rams fed with diets with low or high ratio forage/concentrate. Rev Med Vet. 157:609–313.
- Ghassemi NJ, Bae-Hun LE, Byong-Wan K, Sang-Jip O, Kyung IS. 2016. Effects of chromium methionine supplementation on blood metabolites and fatty acid profile of beef during late fattening period in Holstein steers. Asian Australas J Anim Sci. 29:378–383.
- Guifen L, Fachun W, Enliang S, Xiaomu L, Xiuwen T, Zhenshan L. 2011. Effects of chromium picolinate supplementation on the growth, carcass quality and gene expression of beef during the finishing period. Mol Biol Rep. 38(7):4469–4474.
- Haldar S, Samanta S, Banarjee R, Sharma B, Ghosh TK. 2007. Glucose tolerance and serum concentrations of hormones and metabolites in goats (Capra hircus) fed diets supplemented with inorganic and organic chromium salts. Animal. 1(3):347–256.
- Haldar S, Mondal S, Samanta S, Ghosh TK. 2009. Effects of dietary chromium supplementation on glucose tolerance and primary antibody response against peste des petits ruminants in dwarf Bengal goats (Capra hircus). Animal. 3(2):209–217.
- Honikel KO. 1997. Reference methods supported by OECD and their use in Mediterranean meat products. Food Chem. 59(4):573–582.
- Hunt MC, Acton JC, Benedict RC, Calkins CR, Cornforth DP, Jeremiah LE. 1991. American meat science association guidelines for meat colour evaluation. In: Proceedings of the 44th annual reciprocal meat conference, p. 9–12. July.
- Jenkins TC. 1993. Lipid metabolism in the rumen. J Dairy Sci. 76(12):3851–3863.
- Kalscheur KF, Teter BB, Piperova LS, Erdman RA. 1997. Effect of dietary forage concentration and buffer addition on duodenal flow of trans-C18:1 fatty acids and milk fat production in dairy cows . J Dairy Sci. 80(9):2104–2114.
- Kegley EB, Spears JW, Brown TT. 1997. Effect of shipping and chromium supplementation on performance, immune response, and disease resistance of steers. J Anim Sci. 75(7):1956–1964.
- Kitchalong L, Fernandez JM, Bunting LD, Southern LL, Bidner TD. 1995. Influence of chromium tripicolinate on glucose metabolism and nutrient partitioning in growing lambs. J Anim Sci. 73(9):2694–2705.
- Kumar M, Kaur H, Mani V, Deka RS, Tyagi AK, Chandra G, Kushwaha R. 2017. Supplemental chromium in cold-stressed buffalo calves (Bubalus bubalis): effects on growth performance, nutrient utilization and cell mediated and humoral immune response. Vet Arhiv. 87(4):441–456.
- Latham MJ, Storry JE, Sharpe ME. 1972. Effect of low-roughage diets on the microflora and lipid metabolism in the rumen. Appl Microbiol. 24(6):871–877.
- LeDoux M, Rouzeau A, Bas P, Sauvant D. 2002. Occurrence of trans-C18:1 fatty acid isomers in goat milk: effect of two dietary regimens. J Dairy Sci. 85(1):190–197.
- Lemme A, Wenk C, Lindemann M, Bee G. 2000. Chromium yeast affects growth performance and plasma traits but not carcass characteristics of growing-finishing pigs depending on the glycemic index. Arch Tierernahr. 53(2):157–177.
- Kemp 1D, Mahyuddin M, Ely DG, Fox JD, Moody WG. 1980. Effect of feeding systems, slaughter weight and sex on organoleptic properties, and fatty acid composition of Iamb. J Anim Sci. 51(2):321–330.
- Lien TF, Wu CP, Lin BH, Wang BJ, Lu JJ, Shiao TY. 1998. Effect of different protein and limiting amino acid levels coupled with a supplement of chromium picolinate on lipid metabolism and carcass characteristics of pigs. Anim Sci. 67(3):601–607.
- Lien TF, Wu CP, Wang BJ, Shiao MS, Shiao TY, Lin BH, Lu JJ, Hu CY. 2001. Effect of supplemental levels of chromium picolinate on growth performance, serum traits, carcass characteristics and lipid metabolism of growing-finishing pigs. Anim Sci. 72(2):289–296.
- Lukaski HC, Bolonchuk WW, Siders WA, Milne DB. 1996. Chromium supplementation and resistence training: effects on body composition, strength and trace element status of men. Am J Clin Nutr. 63(6):954–965.
- Mertz W. 1993. Chromium in human nutrition: a review. J Nutr. 123(4):626–633.
- Mertz W. 1969. Chromium occurrence and function in biological systems. Physiol Rev. 49(2):163–239.
- Min JK, Kim WY, Chae BJ, Chung IB, Shin LS, Choi YJ, Han IK. 1997. Effects of chromium picolinate (CrP) on growth performance, carcass characteristics and serum traits in growing-finishing pigs. Asian Australas J Anim Sci. 10(1):8–14.
- Mordenti A, Piva A, Piva G. 1997. The European perspective on organic chromium in animal nutrition. In: Lyons TP, Jacques KA. (Eds.). Biotechnology in the feed industry: proceedings of Alltech’s 13th annual symposium. Nottingham (UK): Nottingham University Press. p. 227–240.
- Moreno-Camarena L, Domínguez-Vara I, Bórquez-Gastelum J, Sánchez-Torres J, Pinos-Rodríguez J, Mariezcurrena-Berasain A, Morales-Almaráz E, Salem AZM. 2015. Effects of organic chromium supplementation to finishing lambs diet on growth performance, carcass characteristics and meat quality. J Integr Agric. 14(3):567–574.
- Mowat DN. 1997. Organic chromium in animal nutrition. Guelph. Canada: Chromium Books, CAB Direct.
- National Research Council. 2005. Mineral tolerance of animals. 2nd revised ed. Washington, DC.The National Academic Press.
- National Research Council. 2007. Nutrient requirements of small ruminants: sheep, goats, cervids, and new world camelids. Washington, DC: The National Academy Press. USA
- Okada S, Tsukada H, Ohba H. 1984. Enhancement of nucleolar RNA synthesis by chromium (III) in regenerating rat liver. J Inorg Biochem. 21(2):113–124.
- Olsen QR, Rule DC, Field RA, Snowder GD, Hu CY. 1996. Dietary chromium picolinate does not influence growth or carcass composition in feedlot lambs. Sheep & Goat Res J. 12:22–24.
- Page TG, Southern LL, Ward TL, Thompson DL. Jr. 1993. Effect of chromium picolinate on growth and serum and carcass traits of growing-finishing pigs. J Anim Sci. 71(3):656–663.
- Palmquist DL, Schanbacher FL. 1991. Dietary fat composition influences fatty acid composition of milk fat globule membrane in lactating cows. Lipids. 26(9):718–722.
- Pechová A, Illek J, Šindelář M, Pavlata L. 2002. Effects of chromium supplementation on growth rate and metabolism in fattening bulls. Acta Vet Brno. 71(4):535–541.
- Puls R. 1994. Minerals levels in animal health. Diagnostic Data. Canada, Clarbrook: Sherpa International.
- Rodríguez-Gaxiola MA, Domínguez-Vara IA, Barajas-Cruz R, Contreras-Andrade I, Morales-Almaráz E, Bórquez-Gastelum JL, Sánchez-Torres JE, Trujillo-Gutiérrez D, Salem AZM, Ramírez-Bribiesca E, et al. 2020. Effect of enriched-chromium yeast on growth performance, carcass characteristics and fatty acid profile in finishing Rambouillet lambs. Small Rum Res. 188:106–109.
- Rust RE, Olson DG, Kratzer DD, Schuler RO, Vetter RLM. 1970. M. longissimus area of lamb carcasses-a comparison of four measurement techniques and the evaluation of operator differences. J Anim Sci. 30(1):36–39.
- SAS Institute Inc. 2004. SAS/STAT® 9.1 User’s Guide. Cary (NC): SAS Institute Inc. p. 5136.
- Soltan MA, Almujalli AM, Mandour MA, El-Shinway AM. 2012. Effect of dietary chromium supplementation on growth performance, rumen fermentation, characteristics and some blood serum units of fattening dairy calves under heat stress. Pakistan J Nutr. 11:751–756.
- Song Z, Xiaoli AM, Yang F. 2018. Regulation and metabolic significance of de novo lipogenesis in adipose tissue – review. Nutrients. 10(10):1383–1322.
- Soto-León S, Zazueta-Patrón IE, Piña-Valdez P, Nieves-Soto M, Reyes-Moreno C, Contreras-Andrade I. 2014. Extracción de lípidos de Tetraselmis suecica: Proceso asistido por ultrasonido y solventes. Rev Mex Ing Quím. 13:723–737.
- Swanson KC, Harmon DL, Jacques KA, Larson BT, Richards CJ, Bohnert DW, Paton SJ. 2000. Efficacy of chromium-yeast supplementation for growing beef steers. Anim Feed Sci Tech. 86(1–2):95–105.
- Tian YY, Gong LM, Xue JX, Cao J, Zhang LY. 2015. Effects of graded levels of chromium methionine on performance, carcass traits, meat Quality, fatty acid profiles of fat, tissue chromium concentrations, and antioxidant status in growing-finishing pigs. Biol Trace Elem Res. 168(1):110–121.
- Van Soest PJ, Robertson JB, Lewis BA. 1991. Methods for dietary fiber, neutral detergent fiber, and nonstarch polysaccharides in relation to animal nutrition. J Anim Sci. 74(10):3583–3597.
- Vincent JB. 2000. The biochemistry of chromium. J Nutr. 130(4):715–718.
- Vincent JB. 2007. The nutritional biochemistry of chromium (III). Amsterdam (The Netherlands): Elsevier B.V.
- Vincent JB. 2013. The bioinorganic chemistry of chromium. New Delhi (India): John Wiley & Sons, Ltd.
- Wolff RL. 1995. Content and distribution of trans-18:1 acids in ruminant milk and meat fats. Their importance in European diets and their effect on human milk. J Am Oil Chem Soc. 72(3):259–272.
- Yan X, Zhang W, Cheng J, Wang R, Kleemann DO, Zhu X, Jia Z. 2008. Effects of chromium yeast performance, insulin activity, and lipid metabolism in lambs fed different dietary protein levels. Asian Australas J Anim Sci. 21(6):853–860.
- Zhou B, Wang H, Luo G, Niu R, Wang J. 2013. Effect of dietary yeast chromium and L-carnitine on lipid metabolism of sheep. Biol Trace Elem Res. 155(2):221–227.