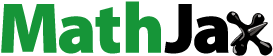
Abstract
The study was conducted with the objective of evaluating the nutrient content, fermentation characteristics, microbial counts, and ruminal degradability of two mixtures of Italian ryegrass (Lolium multiflorum Lam.) and winter cereal silages. Two mixtures (mixture A: Italian ryegrass, triticale, oats, wheat and barley; and mixture B: Italian ryegrass and oats) were wilted and ensiled in laboratory‐scale silos without additives. At the end of 90 d fermentation mixture B silage had higher (p < .05) dry matter (DM), ether extract (EE), crude fibre (CF), neutral detergent fibre (NDF) and acid detergent fibre (ADF) contents than mixture A silage. The pH value of mixture B was higher (p < .05) than mixture A silage. Mixture A had higher lactate, acetate and ethanol contents than mixture B. However, mixture B had higher NH3-N (day 14) than mixture A silage. The mould and yeast count (Log10 CFU g−1) was higher (p < .05) for mixture A than mixture B at opening day 7. The in situ incubation revealed that potentially degradable fraction (b) of DM, CP and NDF was 39.41%, 43.59%, 80.23% (mixture A) and 39.16%, 32.39%, 94.35% (mixture B). The effective protein degradability (EPD) at 0.08 rumen outflow rates was 67.26% (mixture A) and 67.19% (mixture B). These results suggest that with the proper stage of harvesting, Italian ryegrass and winter cereal mixtures were fermented well. This kind of mixtures can be ensiled without additives in the future. The high potentially degradable NDF and effective protein degradability implies that this mixture could be included successfully in high-yielding dairy cattle diets.
Italian ryegrass and winter cereal mixtures are well preserved without additives and it saves the cost of additives for dairy farmers.
The ensiled mixtures have high potentially degradable NDF and effective protein degradability, which improve dry matter intake, milk production and increase the net return of farm.
The mixture forage can be double-cropped with corn for silage making, which can have both environmental and economic benefits if the ensiled mixture yields are enough to cover expenses.
Highlights
Introduction
Silage making has increased considerably since the 1960s and has become economically relevant for many farming systems in temperate areas of the world (Wilkins Citation2005). Corn is the principal ensiled crop in European countries, North America, New Zealand and Australia (Allen et al. Citation2003; Keady et al. Citation2012) with average feeding rates of 3719.46 and 2449.40 Kg dry matter (DM) corn silage/cow/year in Europe and Northern America respectively, (Kleinmans et al. Citation2016). Apart from corn, one of the commonest crops usually conserved for silage is grass. Both single species of grass (e.g. Italian ryegrass (Lolium multiflorum L.) or perennial ryegrass (Lolium perenne L.)) are grown as silage crops (McDonald et al. Citation1991).
Double cropping winter annuals with corn silage can have both environmental and economic benefits when winter-annual silage yields are enough to cover expenses (Ranck et al. Citation2020). While there is limited information on using winter cereals as an alternative to corn silage, there is growing interest in double cropping of winter cereals for harvest as high-quality forage in the spring (Ketterings et al. Citation2015). Double cropping of winter annuals and corn for silage is widely used to increase dry matter yield per hectare and increase milk production, net return in milk production and reduce nitrogen and phosphorous loss from the soil due to leaching (Ranck et al. Citation2020). Intercropping of most annual legumes with winter cereals is extensively used as a cropping practice for forage production in many countries (Clergue et al. Citation2005) and in Europe, the use of rye silage for dairy cow nutrition is popular due to its higher energy content compared with annual cereals silages (National Research Council (NRC) Citation2001).
Seeding mixtures of Italian ryegrass and winter cereals for silage have not been reported even though silage-making ability and nutritional quality of the individual forages are good (Baldinger et al. Citation2011, Citation2014; Harper et al. Citation2017). Italian ryegrass and winter cereals can be grown together (Baron et al. Citation2000; Geren Citation2014) and this practice can provide feed earlier than annual ryegrass (early autumn) because they are generally more adaptable to early sowing due to higher tolerance of dry conditions (Geren Citation2014). The wide harvest window (the harvest window for Italian ryegrass, triticale, barley and wheat is wider than that for rye) results in high silage quality, in case of rainy weather or technical failure.
The inclusion of 40% (mixture A) and 55% (mixture B) Italian ryegrass could improve the fermentation process of ensiled mixtures. Italian ryegrass has an elevated concentration of water-soluble carbohydrates (WSC, also known as high sugar grasses) (Turner et al. Citation2006). The high WSC improves the fermentation process of ensiled mixtures. It improves the balance and synchrony of the nitrogen and energy supply to the rumen (Miller et al. Citation2001). The CP and net energy content of certain varieties of Italian ryegrass (e.g. the perennial Bahial hybrid, the one-year Suxyl variety) are high (175–179 g/kg DM and 6.25–6.28 MJ/kg DM) (Lehel et al. Citation2011). High energy concentration due to good nutrient digestibility can be explained by the relatively low lignin content of the grass hybrid silage (ADL: 20 and 27 g/kg DM) (Lehel et al. Citation2011). Additionally, the mixtures are harvested at the early heading stage which will have high WSC for appropriate fermentation.
Legumes are not included in the mixture because they have lower fibre digestibility than winter cereals or Italian ryegrass. High fibre digestibility can improve dry matter intake (DMI) and milk production of dairy cows (Raffrenato and Van Amburgh Citation2010; Grant and Cotanch Citation2012), especially when fed at least 10 kg/day/cow under heat stress conditions (Orosz, unpublished data). It is also important for sustainable farming that the components of the mixture react differently to the amount of rainfall: with little autumn/winter rainfall, the mixture will become dominated by winter cereal components, while with abundant rainfall, the dominance of Italian ryegrass. In both cases, high yield biomass can be harvested, but the species composition of the mixture will be different from the original ratios. The components of the mixture complement each other's properties: the digestibility of barley, winter oats and Italian ryegrass is excellent, while wheat and triticale give high yields and wheat is an indicator plant for determining harvest date. Another advantage is that it allows double cropping (Ketterings et al. Citation2015; Ranck et al. Citation2020), so two biomasses can be harvested per year. Winter wheat and winter rye will produce massive root systems and they lessen soil erosion during the winter, improve soil quality, and protect against nitrogen leaching and deflation. Italian ryegrass establishes rapidly and may reduce the risk of soil erosion (Baldinger et al. Citation2014). No studies have been conducted to determine the nutritive value of different combinations of Italian ryegrass (Lolium multiflorum Lam.) and winter cereal silages. Therefore, the objective of the experiment was to evaluate the nutritional composition, fermentation characteristics, microbial counts and ruminal degradability of two different mixtures of Italian ryegrass and winter cereals.
Materials and methods
Experimental site and ensiling procedures
The trial was carried out on a large-scale farm (Galgamenti Agricultural Limited Company, Tura, Hungary − 47.593637 N, 19.576483 E, at 119 m altitude). Two different forage mixtures (commercial products, producer: Agroteam S.p.a., Torrimpietre (RM), Via di Granaretto, 26, 00054 Italy) were studied: mixture A (40% of three cultivars of Italian ryegrass + 20% of two cultivars of winter triticale + 20% of two cultivars of winter oats + 15% of winter wheat + 5% of winter barley; and mixture B: 55% of three cultivars Italian ryegrass + 45% of two cultivars of winter oats). Experimental field was 30,600 m2 (width: 36 m; length: 850 m) for each mixture. Deep loosening and disc plus cylinder cultivation were executed as stubble tillage after winter rapeseed (Brassica napus L.). Slurry (10 m3/ha) and 300 kg/ha artificial fertiliser (NPK: 14:10:20) were applied before sowing on sandy soil. Seedbed was prepared by Kongskilde VibroFlex 7400 cultivator (lifted). The two different forage mixes were sown on 11th September 2017 (overall 75 kg seed/ha to both mixtures) with the depth of 2–5 cm with John Deere 740 A type seed drill. Plant protection treatment was not applied during the growing season. The annual precipitation was 718 mm in 2017. Weather conditions (precipitation by month during the growing season) are shown in Table . Cutting was carried out in heading stage of triticale [25 April, 2018, BBCH (Biologische Bundesanstalt, Bundessortenamt and Chemical Industry) 57–58], at 10 cm stubble height. After cutting mixture A (dry matter (DM): 189 g/kg; crude protein (CP): 161 g/kg DM; neutral detergent fibre (NDF): 485 g/kg DM, and total sugar: 137 g/kg DM) and mixture B (DM: 195 g/kg; CP: 159 g/kg DM; NDF: 519 g/kg DM and total sugar: 138 g/kg DM) were wilted (24 h) without any movement on the windrow. The wilted forage was chopped by a forage harvester (John Deere 7300) on a concrete surface with a theoretical chop length (TCL) of 9 mm (weight: 800 kg of harvested forage). Wilted and chopped material of 510 g were ensiled without additives and packed by hand into anaerobic glass jars capacity of 0.72 litter (0.00072 m3 volume, total no. of laboratory silos = 30 (15/mixture), replicated five times) and stored in a controlled laboratory at a temperature of 21 °C. The applied density was 708 kg wilted material/m3 (mixture A: 200 kg DM/m3; mixture B: 219 kg DM/m3).
Table 1. Total precipitation (mm) by month during the growing season of 2017–2018.
Chemical analysis
Five laboratory silos per experimental mixtures were opened on 7, 14 and 90 d after ensiling. Dry matter (DM), crude protein (CP), crude fibre (CF), neutral detergent fibre (NDF), acid detergent fibre (ADF), ether extract (EE), ash, and total sugar content of all mixtures were determined. Nitrogen free extract (NFE) was calculated as 100% – (% EE + % CP + % Ash + % CF). Chemical analyses of the silages followed Association of Official Agricultural Chemists (AOAC) (Citation2006) protocol. Approximately a 25 g composite sample was taken from each silo type immediately after opening. The sample silage was mixed with 100 ml of distilled water. After hydration for 10 min using a blender, the diluted material was then filtered through a cheese cloth and then pH was determined by using a digital pH metre (Metrohm 744, Switzerland). Lactate was analysed by high-performance liquid chromatography (HPLC) according to a method developed by Megias et al. (Citation1993). Acetic acid, butyric acid, propionic acid and ethanol were measured by gas chromatography (Crompak, Model CP 9002, The Netherlands) as described by Playne (Citation1985). Ammonia concentration was determined by a modified Berthelot method (Robinson et al. Citation1986).
Microbiological counts
Aerobic mesophilic microorganism count (AMC) or mould and yeasts count of ensiled forage at the three opening days (7, 14 and 90 d) were determined at the Laboratory of Hungarian University of Agriculture and Life Sciences Kaposvár Campus following the standard laboratory protocols (EN ISO 4833-1:2013 (International Organization for Standardization (ISO) 2013) and EN ISO 21527-1:2008 (International Organization for Standardization (ISO) 2008)) using a standard dispersion plate method (Pitt and Hocking Citation2009). Total microbiological counts were expressed as colony-forming units per gram (CFU g-1) and were transformed into log10 to obtain the lognormal distribution.
Ruminal degradability
After the ninety days of fermentation, the ensiled mixtures were subjected to a ruminal degradability study. The ruminal degradability trial was carried out with three multiparous non-lactating Holstein-Friesian dairy cows (600 ± 35 kg body weight) previously surgically fitted (ethical permission number - SOI/31/01044 − 3/2017) with a ruminal cannula (10 cm id., Bar-Diamond Inc., Parma, Idaho, USA) at the experimental dairy farm of Hungarian University of Agriculture and Life Sciences Kaposvár Campus, Hungary. Cows were fed total mixed ration (TMR) formulated according to the dairy nutrient requirement and feeding standard (National Research Council (NRC), 2001) in equal portions at 8:00 and 14:00 on ad libitum basis. The baseline diet [9.12 kg DMI/day; 6.32 MJ NEl/kg DM; 14.4% CP, 39.06% NDF, 23.66% ADF, and 35.71% non-fibrous carbohydrate (NFC)] consisted of 5.5 kg day−1 of corn silage, 3.5 kg day−1 of alfalfa haylage, 3.5 kg day−1 of vetch-triticale haylage, 3 kg day−1 of concentrate, 1 kg day−1 of grass hay and 0.75 kg day−1 of liquid molasses. The cows consumed the daily allotted TMR with no daily feed refusal throughout the experimental period. Water was available ad libitum. Rumen incubations were carried out according to Herrera-Saldana et al. (Citation1990). Nylon bags of 5 × 10 cm with pore size of 53 µm (Ankom, USA) filled with sample weight of 5.0 g (on-air dry matter basis) was incubated for 0, 2, 4, 8, 16, 24, 48 and 72 h incubation times. In each incubation, 60 bags per sample were used (5 bags × 4 replications per sample × 3 cows). The 0 h samples were not placed in the rumen, but they were soaked and rinsed as described below. Removed bags were placed in cold tap water immediately after removal from the rumen, and they were washed by hand until the water was clear. After washing, the bags were dried in a forced-air oven at 60 °C for 48 h, air equilibrated and weighed. Residues from the bags were pooled within time and animal, finely ground by mortar and pestle to pass through a 1-mm screen and retained in sealed containers to determine DM, CP, NDF and ADF. Feeds were analysed for nitrogen according to Kjeldahl (Association of Official Agricultural Chemists (AOAC), Citation2006), and thereafter, CP was determined by the total nitrogen (N) × 6.25. The NDF and ADF contents were residual portions after rinsing according to Van Soest et al. (Citation1991).
Calculations and statistical analysis
Calculations
Residues from the nylon bags at each incubation time were analysed for DM, CP, NDF and ADF as described above. Ruminal nutrient disappearance data were used to determine nutrient degradation parameters using the equation (Ørskov and McDonald Citation1979):
where P in the equation is the DM, CP, NDF or ADF disappearance (%) at time t, a is the soluble fraction (%), b is the potentially degradable fraction (%), and c is the rate of degradation of the b fraction (%/h). Effective degradability (ED) of DM, CP, NDF and ADF was then calculated according to the equation (Ørskov and McDonald Citation1979):
where k is the rumen outflow rate assumed to be 1, 5 and 8%/h and a, b, and c are as described above. NLIN program in SAS (version 9.4; SAS Institute, Inc., Cary, NC, USA) was used to calculate the values of a, b, and c.
Statistical analysis
Data were analysed using the GLM procedure for ANOVA in SAS 9.1 software (SAS, Citation2013). Significant mean value differences were evaluated by Tukey’s test following post-hoc pairwise comparison of means. A significance level of p < .05 was used. Comparisons of mean values for nutritional composition, fermentation characteristics and microbial counts were performed using the following model:
where Yij is the observation in the ith opening days, jth mixtures and their interaction effect, µ is the overall mean, αi is the ith opening days effect, βj is the jth mixtures effect, γij is the interaction of opening days and mixtures effect, εij is the random error.
Comparison of means for degradability components was performed following model:
where Yi is the observation in the ith mixtures, µ is the overall mean, αi is the ith mixtures effect and εi is the random error. Comparison of means for effective nutrient degradability was computed for 1%, 5% and 8% rumen outflow rates.
Results
Nutritional composition
The fermentation process affected (p < .05) the DM and NDF content of both mixture silages at each opening day (Table ). There were no differences (p > .05) on the DM, CP and total sugar contents of mixture silages at the three opening days (7, 14 and 90) associated with differences in mixture type. However, the NDF (mixture A) content was decreased at day 90 as compared to day 7 and 14; and ADF (mixture B) content was increased at day 90 as compared to day 7. The interaction of opening days and mixture type had a significant effect (p < .05) on the nutritional composition (except total sugar and NFE contents) of mixture silages. At the end of 90 d of fermentation most of the nutrient contents of both silages were affected (p < .05) except CP, total sugar and NFE; and mixture B silage had the highest DM, EE, CF, NDF and ADF contents than mixture A silage. The CP was well preserved attributed to lactic acid type fermentation (Tables ). Well-preserved CP could improve the ruminal degradability of nutrients particularly fibre degradability (NDF and ADF) as well as improve protein-dependent metabolisable energy utilisation.
Table 2. Nutritional composition of silage on 7, 14 and 90 opening days, at different crop mixtures and their interaction (n = 5).
Table 3. Fermentation characteristics of silage on 7, 14 and 90 opening days, at different crop mixtures and their interaction (n = 5).
Fermentation characteristics
Both mixture types and the interaction of opening days and mixture type had a significance (p < .05) effect on fermentation characteristics (except ethanol and acetic acid contents) of mixture silages (Table ). At the end of 90 d of fermentation, mixture A had a lower (p < .05) pH value than mixture B silage. The pH values were also lower (p < .05) for mixture A than mixture B silage during the early fermentation phase (opening days 7 and 14) and 90 days as well. Fermentation products were limited to three principal products: lactic acid (LA), acetic acid (AA), and ethanol. Other volatile fatty acids (VFAs) like propionic acid (PA), butyric acid (BA), valeric acid (VA) and caproic acid (CA) were below detectable concentrations (<0.1 g/kg of DM). Lactate, acetate and ethanol contents were higher for mixture A than mixture B. However, there was no difference (p > .05) in LA/AA, LA (%TFA) and NH3 – N contents between mixture silages. The concentration of lactate gradually increased over time, reaching their highest values at day 90 for both ensiled mixtures. During the 90 days of fermentation, LA continued to be the major fermentation product resulting in a high LA/AA ratio over the storage periods. The observed percent of lactic acid per total fermentation acid (LA% of TFA) was above 72% for both mixture silage. The amount of NH3-N (g/100 g total N) was very low (<9 g/100 g total N). On opening day 14, mixture B had higher NH3-N than mixture A silage. Fermentation caused an increase in NH3-N (g/100 g total N) at day 90 as compared to day 14 for both mixture silages. However, there were no negative results reported on fermentation products (Table ) as well as microbial count (Table ) associated with this change.
Table 4. Microorganism count (Log10 CFU g−1) of silage on 7, 14 and 90 opening days, at different crop mixtures and their interaction (n = 5).
Microbiological quality
There was no difference (p > .05) in mould and yeast count (Log10 CFU g−1) in the silages, on all opening days except day 7 after opening the laboratory silos (Table ). The mould and yeast count (Log10 CFU g−1) was higher (p < .05) for mixture A than mixture B at opening day 7. However, there were no differences (p > .05) in aerobic mesophilic microorganisms count (AMC) (Log10 CFU g−1) between mixtures A and B silages at each opening day. Both the mixture types and interaction of opening days and mixture type had a significance effect (p < .05) on AMC (Log10 CFU g−1) of mixture silages.
Ruminal degradability
Table shows ruminal degradability of DM, CP, NDF and ADF (a, b, kp) and effective degradability (ED) calculated for various rumen solid outflow rates (0.01, 0.05 and 0.08 h−1). The soluble fraction, the slowly degradable DM fraction and its rate of degradation and effective DM degradability-8 (ED8) were similar among the mixtures. As compared to mixture A, mixture B had a higher (p < .05) in situ soluble CP fraction, and lower (p < .05) potentially degradable CP fraction. The effective protein degradability (at 8% rumen solid outflow rate/h); EPD8) was 67.26% (mixture A) and 67.19% (mixture B). The soluble NDF and ADF fractions of mixture A and B silages were low. Mixture A had higher (p < .05) in situ soluble NDF and ADF fraction than mixture B. The effective ADF degradability at 0.08 h−1 solid rumen outflow rate (ED8) was higher for mixture A than mixture B. However, there were no difference on effective NDF and ADF degradability at 0.01 h−1 solid rumen outflow rate (ED1) between mixture A and B silages. The potentially degradable as well as effective degradable NDF and ADF at 0.01 and 0.08 h−1 rumen solid outflow rate (ED1 and ED8) was high. The potentially degradable NDF fraction, its degradation rate and effective ruminal degradability-8 (ED8) were 80.23%. 0.017, and 18.68% (mixture A) and 94.35%, 0.014 and 16.37% (mixture B). Potentially degradable ADF fraction, its rate of degradation and effective ruminal degradability (ED8) were 85.18%. 0.017, and 19.45% (mixture A) and 87.26%, 0.014 and 16.44% (mixture B).
Table 5. Degradable characteristics of silage (n = 60).
Discussion
Chemical composition
The DM content did not change greatly up to 90 d of ensilage, because the laboratory silos were in anaerobic conditions and had no seepage loss. At the end of fermentation, the DM content of mixture A (27.46%) and mixture B (31.34%) silages was lower than the DM content of Italian ryegrass silage (36.5%) and winter cereals silage: barley (35.5%), oat (34.6%), triticale (32.0%) and wheat (33.3%) silages (National Research Council (NRC) Citation2001). However, as compared to DM contents of winter cereals silage: triticale (30.6%) and wheat (28.2%) harvested at the heading stage (Orosz et al. Citation2019), mixture B had higher DM content. The observed CP contents of mixture A (15.24%) and mixture B (16.08%) silages was higher than the CP content of Italian ryegrass silage (12.8%) and winter cereals silage: barley (12.0%), oat (12.9%), triticale (13.8%) and wheat (12.0%) (National Research Council (NRC) 2001). The value was also higher than winter cereal silages: barley (13.3%), oat (11.0%), triticale (10.7%) and wheat (12.1%) harvested at the heading stage (Orosz et al. Citation2019). The high CP value is a direct reflection of the quality of the present mixtures at the time of harvest (early heading stage) before ensiling as well as a higher proportion of Italian ryegrass than cereal forage in the total mixed ensiled forage because Italian ryegrass has more protein than cereals (Baldinger et al. Citation2011, Citation2014; DLF seeds UK Citation2018; Byron Seeds LLC Citation2019). The total sugar content of fresh forage mixtures was high. This could also be attributed to a high proportion of Italian ryegrass (40% in mixture A and 55% in mixture B). As reported by Baldinger et al. (Citation2014) the sugar content of Italian ryegrass is superior provided that it is harvested at the early stages. They further noted that Italian ryegrass harvested at the second cut had significantly higher (71.87%) sugar content than corn. The observed residual sugar which is assumed to be the source of energy for the rumen microbes was significantly affected by fermentation process. However, there was no difference (p > .05) in sugar contents associated with opening days for both mixture silages after the 90 d ensiling process. After the end of fermentation, the ADF content was higher for both mixture silages. This result is consistent with the finding of Leão et al. (Citation2017), who reported a significant increase in ADF contents of winter cereals silages (triticale, barley, white and black oats) harvested at the soft dough stage and subjected to different storage periods (60, 120 and 180 d).
Fermentation characteristics
The rate and extent of reduction in pH were continuous within 90 d of ensiling for both silage mixtures. The rapid decrease in pH prevents the breakdown of plant proteins and helps to inhibit growth of spoilage microbes. This finding is similar to other studies such as early-stage ensiling of Italian ryegrass (Shao et al. Citation2002), Italian ryegrass and Guinean grass silage (Shao et al. Citation2005), corn silage (Bal Citation2006; Ülger and Kaplan Citation2017) and high moisture Italian ryegrass, Guinean grass and whole crop corn silages (Li and Nishino Citation2013). The reduction of pH was mainly caused by rapid and intensive production of LA. During the 90 d of fermentation, LA continued to be the major fermentation product with a small production of AA, resulting in the high value of LA/AA over the storage periods. These indicate that acidification was initiated by homofermentative lactic acid bacteria, and this was dominant during the fermentation course. The LA/AA is a good efficiency indicator for silage fermentation (Jalc et al. Citation2009). This ratio ideally should not be less than 3:1, and the higher is better (Kung and Shaver Citation2001). In the present study, this ratio increased over time and the highest value of 3.97:1 and 3.67:1 was observed for both silages at the end of storage. Proportions of LA (% TFA) at opening day 90 were consistent with the report of Agriculture and Horticulture Development Board (AHDB) (Citation2012) for grass silage, which described that for well-fermented silage lactic acid as the proportion of total acids should be >75%. However, proportions of LA at each opening day for both mixtures of silage were higher than the value reported by Kung and Shaver (Citation2001), which stated that lactic acid should be the primary acid and should be at least 65 to 70% of the total fermentation acids in high-quality silage. Ethanol was detected during the storage period probably due to the survival of some yeast throughout the ensilage period. The PA, BA, VA and CA were undetectable over the ensiling period. This is attributed to the rapid reduction in pH because of the rapid production of LA, restricting the growth of clostridia and other bacteria (Henderson, Citation1993). The NH3-N/total N of mixture A and B was low (<9 g/100g total N) at the three opening days. As per the criteria after the end of the fermentation process when the NH3-N/total N is below 7 g/100 g total N, the silage could be categorised as excellent silage. Therefore, silage ensiled for 90 d in the present study may be categorised as excellent quality even though the NH3-N/total N at day 90 was slightly higher than 7 g/100g total N.
Microbiological quality
The aerobic mesophilic microorganisms count (AMC) (Log10 CFU g−1) for day 7 and 14 of both mixtures’ silage was in agreement with the normal level (6.00 (Log10 CFU g−1) or 1 × 106 (CFU g−1) of European decree (EN ISO 4833, Microbiological limits 65-2012 VM Decree Annexe 12). As compared to days 7 and 14, significantly higher CFU counts were recorded at opening day 90 in both mixtures. This could be attributed to the presence of microbial population such as LAB and other anaerobes which would maintain the lactic acid type fermentation of ensiled material until day 90. The microbiological quality result indicates that the mould and yeast count (Log10 CFU g−1) was low and there was no negative report on fermentation end-products associated with mould and yeast count. The mould and yeast count (Log10 CFU g−1) was consistent with the limit recommended as a quality standard for animal feeds (3.00 (Log10 CFU g−1) or 1 × 104 (CFU g−1) (GMP Citation2008). Mould and yeast counts at different opening days were lower than the results obtained by González et al. (Citation2008) who found that 90% of their samples counts over 3.00 (Log10 CFU g−1) or 1 × 104 (CFU g−1) and Keller et al. (Citation2013) who reported mould count of 4.76 (Log10 CFU g−1) or 5.74 × 104 (CFU g−1) for corn silage at its post-fermentation phase.
Ruminal degradability
Ruminal degradability of DM
The most important factors affecting the DM degradability are the contents of the NDF and N-free extracts in the silage DM. The potential ruminal degradability for DM of mixture A and B silages was 39.41% and 39.16%, respectively and the effective rumen DM degradabilities at 8% rumen solid outflow rate (ED8) of mixture A and mixture B were 48.47% and 48.23% respectively. These values were lower than the DM degradability of Italian ryegrass (60.7%) reported by Andrighetto et al. (Citation1993). The low DM degradability in the present silage mixtures could be attributed to the inclusion of cereals which would increase the fibre fraction, particularly oat, triticale and wheat (National Research Council (NRC) 2001) as compared to the sole Italian ryegrass forage used in the previous study. NDF including cellulose and lignin seemed to protect the silage DM against ruminal digestion. However, the effective DM degradability at 1% rumen solid outflow rate (ED1), which defines the maintenance DM requirement were 64.61% (mixture A) and 65.58% (mixture B) better than the report of Andrighetto et al. (Citation1993).
Ruminal degradability of CP
The potential ruminal degradability of CP for mixture A and B silages was 43.59% and 31.87% respectively and the effective rumen CP degradability at 8% rumen solid outflow rate (ED8) of mixture A and mixture B were 67.27% and 67.04% respectively. Both the potential and effective degradability of CP in the present silage mixture was lower than that of Italian ryegrass forage at 1st (81.4%) and 2nd (82.3%) cut of leaf stage, grazing (81.8%) and heading (82%) stages as well as CP degradability of alfalfa (81.4%) at the first cut of its vegetative stage (Amrane and Michalet-Doreau Citation1993). It was also lower than the CP degradability of Italian ryegrass forage at end of its heading stage (76.9%); alfalfa at the 2nd cut of vegetative (77.9%) and end of budding (77.4%) stages reported by the same author. The low CP degradability in the present silage mixtures could be attributed to the inclusion of cereals similar to the case in DM degradability described above. On the other hand, ensiling could also affect the CP degradability (de Oliveira et al. Citation2016) as compared to pure stand Italian ryegrass forage even though both the present and previously used crops harvested at the proper stage of maturity (heading) for maximum protein utilisation by rumen microbes. The soluble fraction of mixture B silage (50.82%) was higher than the soluble CP fraction (47%) of corn silage (Susmel et al. Citation1990) and ryegrass silage (49.05) at its vegetative stage (Valderrama and Anrique Citation2011) as well as different cereal forages (Hadjipanayiotou et al. Citation1996; Turgut and Yanar Citation2004). The soluble fraction of Italian ryegrass forage at 1st (20.6%) and 2nd (19.2%) cut of leaf stage as well as 2nd cut of vegetative (27.4%), early budding (24%), budding (18.4%) and end of budding (20%) stages of alfalfa (Amrane and Michalet-Doreau Citation1993) were all lower than the value in mixture B silage. Muazzez (Citation2018) reported lower water-soluble CP fractions for mature alfalfa hay (50.82% vs. 37.26%) and normal corn silage (50.82% vs. 40.34%) than mixture B silage, respectively. The soluble fraction of mixture A was in the range of a soluble fraction of grass silage 21–38% (Turgut and Yanar Citation2004). However, the immediately soluble fraction of CP of both mixtures was lower than alfalfa (56.69%) and oat (68.47%) forages (Valderrama and Anrique Citation2011) as well as different forage silages (53.7%) (Edmunds et al. Citation2012). The insoluble but potentially degradable CP fraction of both mixtures were lower than the slowly degradable CP fraction Italian ryegrass forage at 1st (74.8%) and 2nd cut (76.8%) of leaf stage as well as alfalfa silage at budding (74%) (Amrane and Michalet-Doreau Citation1993), different cereal forages (Turgut and Yanar Citation2004; Valderrama and Anrique Citation2011); forage silages (Edmunds et al. Citation2012); alfalfa and ryegrass forage (Valderrama and Anrique Citation2011); grass silage (Susmel et al. Citation1990; Turgut and Yanar Citation2004) and corn silage (Susmel et al. Citation1990; Muazzez Citation2018). The degradation rate of fraction ‘b’ at time t(c) for both mixtures was 0.620 and 0.083. These values were higher than the values in normal corn silage (Muazzez Citation2018). The high degradation rate could be attributed to proper stage of harvesting (early heading) prior to ensiling as well as a higher proportion of Italian ryegrass than winter cereals in both mixture silages which improves protein recovery and increases degradability of ensiled material by rumen microbes. The degradability rate of mixture A (0.620) is substantially higher than Amrane and Michalet-Doreau (Citation1993) report for Italian ryegrass forage at 1st (0.142) and 2nd cut (0.140) of leaf stage, grazing stage (0.110), heading stage (0.103) and alfalfa forage at 1st (0.162) and 2nd cut (0.154) of the vegetative stage, early budding (0.152), budding (0.166) and end of budding (0.137) stage. Valderrama and Anrique (Citation2011) also reported a lower ‘c’ parameter for alfalfa (0.197), oat forage (0.294) and ryegrass forage (0.157) at the vegetative stage as compared to the value in mixture A silage. Turgut and Yanar (Citation2004) also reported lower ‘c’ values for alfalfa hay (0.113). This higher rate of CP degradability would make the current silage mixture attractive to combine in other higher fibre crops for better forage utilisation in the nutrition of dairy cows. The EPD values at 0.05 and 0.08 h−1 in both mixtures were higher than the EPD of corn silage (60.11% and 55.88%, respectively) at 0.05 and 0.08 h−1 rumen outflow rates reported by Muazzez (Citation2018). The higher EPD in the present mixture silages could be attributed to either the proper stage of harvesting (heading) prior to an ensiling or a higher proportion of Italian ryegrass in both mixture silages. Italian ryegrass has higher CP at the proper stage of harvesting, i.e. 2nd cut (Baldinger et al. Citation2011) which is similar to CP values at the end of 90 d fermentation period in the present study. However, Valderrama and Anrique (Citation2011) reported higher EPD values at 0.05 and 0.08 h−1 for alfalfa forage (88.25%, 85.16%), oat forage (90.80%) and ryegrass forage (85.20%, 80.62%) at its vegetative stage. The EPD values at 0.05 h−1 rumen outflow rate of mixture B silages (70.17%) was better than barley (69%, 61% and 56%); and oats (66%, 60% and 56%) at flowering, pod formation and early maturity respectively (Hadjipanayiotou et al. Citation1996). According to National Research Council (NRC) (Citation2001) recommendation, the maximum milk and milk protein yields occur when rumen degradable protein (RDP) is 12.2% of diet dry matter. Therefore, the observed EPD at the three rumen outflow rates was higher than the National Research Council (NRC) (Citation2001) requirement for maximum milk and milk protein yield.
Ruminal degradability of NDF and ADF
For effective rumen function, sufficient NDF should be included in the diets of dairy cows. National Research Council (NRC) (Citation1989) reported that a large proportion of dietary NDF should come from forages and at least 25% of dairy ration should be composed of NDF (Oba and Allen, Citation1999). Therefore, the amount and ruminal degradability of NDF is a very important factor in the dairy cow’s nutrition because forage NDF varies widely in its degradability in the rumen and NDF digestibility influences animal performance (Nousiainen et al. Citation2009; Zebeli et al. Citation2012; Bender et al. Citation2016). Grasses typically have higher NDF content as compared to corn and alfalfa silage (National Research Council (NRC) Citation2001; Bender et al. Citation2016). However, both alfalfa and corn silage have wider acceptance in dairy nutrition due to their higher DM digestibility as compared to grass silage (Bender et al. Citation2016). Identifying highly digestible and/or ruminal degradable (particularly NDF and ADF) grass has been a critical challenge in partial replacement of corn and alfalfa silage in the nutrition of dairy cows (Bender et al., Citation2016). Few research reports (Brink et al. Citation2010; Pelletier et al. Citation2010; Bender et al. Citation2016) revealed that modern varieties of cool-season grasses have been selected to have greater fibre (NDF and ADF) digestibility. The high potential in situ NDF and ADF degradability of the present silage mixtures could be associated with those cultivars selected for high ADF and NDF degradability. The potential ruminal degradability, effective degradability at 0.01 h−1 (ED1) and 0.08 h−1 (ED8) of NDF and ADF were 77.39%, 53.82%, 18.68% and 76.95%, 53.93%, 19.41% (mixture A) or 89.07%, 53.92%, 16.34% and 84.97%, 53.16%, 16.42% (mixture B) respectively. The high potential and effective ruminal degradability could be associated with agronomic practices such as early harvesting (Hoffman et al. Citation1993; Rinne et al. Citation2002) as well as harvesting from spring growth (Rinne et al. Citation2002; Cherney et al. Citation2004; Pelletier et al. Citation2010). The higher effective ruminal NDF degradability (ED8) of mixture A (18.68%) as compared to mixture B (16.34%) associated with lower NDF content (Table ) attributed to acid hydrolysis of hemicellulose due to lactic acid type fermentation. Allen and Oba (Citation1996) reported that enhancing NDF hydrolysis may stimulate rapid disappearance of NDF from the rumen, reduce physical fill, and allow greater voluntary feed intake. The potential ruminal NDF degradability of both mixtures was higher than NDF degradability of Italian ryegrass (59.8%) reported by Andrighetto et al. (Citation1993). Ali et al. (Citation2014) also reported lower NDF degradability of grass silage (76.4%) as compared to the present silage mixtures. The rate of ruminal NDF degradation (kp) for mixture A and mixture B silages were 0.017 and 0.013 respectively. The value in mixture A was higher than grass/grass – clover silage (0.016) as well as whole crop cereal silage (0.015) reported by Weisbjerg et al. (Citation2007).
Conclusions and recommendations
The ensiled mixtures (mixture A: Italian ryegrass, triticale, oats, wheat and barley; and mixture B: Italian ryegrass and oats) were well fermented and produced silage with excellent feed characteristics associated with the proper stage of harvesting (early heading). The high potentially degradable fibre fractions in the ensiled mixtures applied in the diet may increase diet NDF rumen degradability having a positive effect on dry matter intake and performance of the dairy cow. It’s also important to notice that the mixtures are autumn planting and early harvest forages, therefore it can be well integrated into crop rotation, double cropping can also be done with short-growing corn, combined with sorghum or Sudan grass. Further studies are needed to confirm the effect of the mixture of Italian ryegrass and winter cereals on the performance of dairy cows and on the composition of milk.
Ethical approval
All research reported in submitted papers has been conducted in an ethical and responsible manner, and is in full compliance with all relevant codes of experimentation and legislation. The in situ ruminal degradability experiment was conducted with the formal approval of both National Food Chain Safety Office (see the permission number in the document) and/or the National Welfare/Ethics Committee (who also checked the permission).
Disclosure statement
No potential conflict of interest was reported by the author(s).
Additional information
Funding
References
- Agriculture and Horticulture Development Board (AHDB) 2012. Non-Departmental Public Body established under the Agriculture and Horticulture Development Board Order 2008; it became operational on 1 April 2008 and covers around 75% of total agricultural output in the United Kingdom (UK). Stoneleigh Park, Warwickshire, UK. Available online: https://assets.publishing.service.gov.uk/government/uploads/system/uploads/attachment_data/file/247030/0238.pdf
- Ali, M, Cone, JW, Duinkerken, G van, Klop A, Kruisdijk J, Blok MC, Bruinenberg MH, Hendriks WH 2014. Relationship between chemical composition and in situ rumen degradation characteristics of grass silages in dairy cows. NJAS Wageningen J Life Sci. 70–71:9–15.
- Allen MS, Coors JG, Roth GW. 2003. Corn silage. In: Buxton DR, Muck RE, Harrison JH, editors. Silage science and technology. Madison, WI, USA: American Society of Agronomy, Crop Science Society of America, Soil Science Society of America; p. 547–608.
- Allen M, Oba M. 1996. Fiber digestibility of forages. Proceedings of 57th Minnesota Nutrition. Minnesota: St. Paul: Minnesota University.; p. 151–171.
- Amrane R, Michalet-Doreau B. 1993. Effect of maturity stage of Italian ryegrass and lucerne on ruminal nitrogen degradability. Ann Zootech. 42(1):31–37.
- Andrighetto I, Bailoni L, Cozzi G, Tolosa HF, Hartman B, Hinds M, Sapienza D. 1993. Observations on in situ degradation of forage cell components in alfalfa and Italian ryegrass. J Dairy Sci. 76(9):2624–2631.
- Association of Official Agricultural Chemists (AOAC). 2006. Official methods of analysis of AOAC International. 18th ed. Washington DC: AOAC International.
- Bal MA. 2006. Effects of hybrid type, stage of maturity, and fermentation length on whole plant corn silage quality. Turkish J Vet Anim Sci. 30:331–336.
- Baldinger L, Baumung R, Zollitsch W, Knaus WF. 2011. Italian ryegrass silage in winter feeding of organic dairy cows: forage intake, milk yield and composition. J Sci Food Agric. 91(3):435–442.
- Baldinger L, Zollitsch W, Knaus WF. 2014. Maize silage and Italian ryegrass silage as high energy forages in organic dairy cow diets: differences in feed intake, milk yield and quality, and nitrogen efficiency. Renew Agric Food Syst. 29(4):378–387.
- Baron V, Erasmus KO, Dick CA. 2000. Optimizing yield and quality of cereal silage. Adv. Dairy Technol 12:351.
- Bender RW, Lopes F, Cook DE, Combs DK. 2016. Effects of partial replacement of corn and alfalfa silage with tall fescue hay on total-tract digestibility and lactation performance in dairy cows. J Dairy Sci. 99:436–5444.
- Brink GE, Casler MN, Martin NP. 2010. Meadow fescue, tall fescue, and orchard grass response to defoliation management. Agron J. 102(2):667–674.
- Byron seeds LLC. 2019. Cool season plants, Italian ryegrass. [Internet]. [cited 2019]; Available from: http://www.byronseeds.net/our_products/coolseasongrasses/italian_ryegrass.php
- Cherney DJR, Cherney JH, Chase LE. 2004. Lactation performance of Holstein cows fed fescue, orchard grass, or alfalfa silage. J Dairy Sci. 87(7):2268–2276.
- Clergue B, Amiaud B, Pervanchon F, Lasserre-Joulin F, Plantureux S. 2005. Biodiversity: function and assessment in agricultural areas – A review. Agron Sustain Dev. 25(1):1–15.
- de Oliveira, SJ, Santos ME, dos Santos MPA. 2016. Intake and digestibility of silage. Advanced in silage production and utilization (edited by T. da Silva, EM Santos). Chapter 6 101–122., IntechOpen
- DLF, Seeds and Science. 2018. Forage grass species, Italian ryegrass. [Internet]. [cited 2018]; Available from: https://www.dlf.co.uk/forage-grass-seed/species/dlf-uk/forage-grassspecies/italianryegrass-prod336.aspx
- Edmunds B, Südekum KH, Spiekers H, Schwarz FJ. 2012. Estimating ruminal crude protein degradation of forages using in situ and in vitro techniques. Anim Feed Sci Technol. 175(3–4):95–105.
- Geren H. 2014. Dry matter yield and silage quality of some winter cereals harvested at different stages under Mediterranean climate condition. Turkish J Field Crops. 19(2):197–202.
- GMP. 2008. GMP + Certification scheme animal feed sector. Production of and trade in Pet Foods. GMP + Standard B8.
- González ML, Alonso VA, Sager R. 2008. Fungi and selected mycotoxins from pre-and post- fermented corn silage. J Appl Mic. 104:1034–1041.
- Grant RJ, Cotanch KWW. 2012. Dynamics of passage, digestion, and cow productive responses. Proceedings Cornell Nutrition Conference for Feed Manufacturers; Oct 16–18; Syracuse, NY. p. 45–57.
- Hadjipanayiotou M, Antoniou I, Theodoridou M, Photiou A. 1996. In situ degradability of forages cut at different stages of growth. Liv Prod Sci. 45(1):49–53.
- Harper MT, Oh J, Giallongo F, Roth GW, Hristov AN. 2017. Inclusion of wheat and triticale silage in the diet of lactating dairy cows. J Dairy Sci. 100(8):6151–6163.
- Henderson N. 1993. Silage additives. Anim Feed Sci. Technol. 45(1):35–36.
- Herrera-Saldana RE, Huber JT, Poore MH. 1990. Dry matter, crude protein, and starch degradability of five cereal grains. J Dairy Sci. 73(9):2386–2393.
- Hoffman PC, Sievert SJ, Shaver RD, Welch DA, Combs DK. 1993. In situ dry matter, protein, and fiber degradation of perennial forages. J Dairy Sci. 76(9):2632–2643.
- International Organization for Standardization (ISO). 2008. Microbiology of food and animal feeding stuffs – Horizontal method for the enumeration of yeasts and mold – Part 1: colony count technique in products with water activity greater than 0.95. International Standard ISO 21527-1. Geneva, Switzerland: ISO.
- International Organization for Standardization (ISO). 2013. Microbiology of the food chain – Horizontal method for the enumeration of microorganisms – Part 1: Colony count at 30 °C by the pour plate technique. International Standard ISO 4833-1:2013. Geneva, Switzerland: ISO.
- Jalc D, Laukova A, Simonova M, Varadyova Z, Homolka P. 2009. The use of bacterial inoculants for grass silage: their effects on nutrient composition and fermentation parameters in grass silages. Czech J Anim Sci. 54(No. 2):84–91.
- Keady, TWJ, Marley, CM and Scollan, ND 2012. Grass and Alternative Forage Silages for Beef Cattle and Sheep: Effects on Animal Performance. Proceedings of the 16th International Silage Conference, Hämeenlinna, Katherine Region, 28th August 2012, 112–128.
- Keller LAM, González Pereyra ML, Keller KM, Alonso VA, Oliveira AA, Almeida TX, Barbosa TS, Nunes LMT, Cavaglieri LR, Rosa CAR. 2013. Fungal and mycotoxins contamination in corn silage: monitoring risk before and after fermentation. J Stored Prod Res. 52:42–47.
- Ketterings Q, Ort S, Swink S, Godwin G, Kilcer T, Miller J. 2015. Winter cereals as double crops in corn rotations on New York dairy farms. J. Agric. Sci. 7:18-25.
- Kleinmans JJ, Densley RJ, Hurley T, Williamsa ID, Calvert F. 2016. “Brief Communication: Feed Value of Maize Silage in New Zealand - a Review.” Proceedings of the New Zealand Society of Animal Production 76:100–102.
- Kung L, Shaver R. 2001. Interpretation and use of silage fermentation analysis reports. Focus on forage. 3:13. [Internet]. [cited 2001]. Available from: https://fyi.extension.wisc.edu/forage/files/2014/01/Fermentation.pdf
- Leão GFM, Jobim CC, Neumann M. 2017. Nutritional composition and aerobic stability of winter cereal silage at different storage times. Acta Sci. 39:2.
- Lehel L, Orosz S, Tóthné Polner A, Sümeghy L, Hajda Z, Sipiczki B, Várhegyi J, Fébel H. 2011. The rye grass silage apparent digestibility, rumen protein degradability, content of metabolizable protein and net energy. AWETH. 7:149–157.
- Li Y, Nishino N. 2013. Effects of ensiling fermentation and aerobic deterioration on the bacterial community in Italian ryegrass, Guinea grass, and whole-crop maize silages stored at high moisture content. Asian Australas J Anim Sci. 26(9):1304–1312.
- McDonald P, Henderson AR, Heron SJ. 1991. The biochemistry of silage. 2nd ed. Marlow, UK: Chalcombe Publications.
- Megias MD, Martinez TA, Gallego JA, Nuñez JM. 1993. Chemical changes during the ensiling of orange peel. Anim Feed Sci Technol. 43:269–274.
- Miller LA, Moorby JM, Davies DR, Humphreys MO, Scollan ND, MacRae JC, Theodorou MK. 2001. Increased concentration of water-soluble carbohydrate in perennial ryegrass (Lolium perenne L.). Milk production from late-lactation dairy cows. Grass Forage Sci. 56(4):383–394.
- Muazzez C. 2018. Determination of ruminal protein degradation of three forages using in vitro protein fractions and in situ protein degradability characteristics. J Dairy Vet Anim Res. 7:154–159.
- Nousiainen J, Rinne M, Huhtanen P. 2009. A meta-analysis of feed digestion in dairy cows. 1. The effects of forage and concentrate factors on total diet digestibility. J Dairy Sci. 92(10):5019–5030.
- National Research Council (NRC).1989. Nutrient requirements of dairy cattle. 6th rev. ed. Washington, DC: National Academies Press.
- National Research Council (NRC). 2001. Nutrient requirements of dairy cattle. 7th rev ed. Washington, DC: National Academies Press.
- Oba M, Allen MS. 1999. Evaluation of the importance of digestibility of neutral detergent fiber from forage: effects on dry matter intake and milk yield of dairy cows. J Dairy Sci. 82(3):589–596.
- Orosz S, Horváthné KB, Kruppa J, et al. 2019. [Húshasznú tehenek, növendék- és hízó marhák hazai tömegtakarmány-ellátása a klímaváltozás tükrében]. Állatteny és Tak. 66:365–382. (English Abstract, Hungarian).
- Ørskov ER, McDonald I. 1979. The estimation of protein degradability in the rumen from incubation measurements weighted according to rate of passage. J Agric Sci. 92(2):499–503.
- Pelletier S, Tremblay GF, Bélanger G, Bertrand A, Castonguay Y, Pageau D, Drapeau R. 2010. Forage nonstructural carbohydrates and nutritive value as affected by time of cutting and species. Agron J. 102(5):1388–1398.
- Pitt JI, Hocking AD. 2009. Fungi and food spoilage. 3rd ed. London: Blackie Academic and Professional.
- Playne MJ. 1985. Determination of ethanol, volatile fatty acids, lactic and succinic acids in fermentation liquids by gas chromatography. J Sci Food Agric. 36(8):638–644.
- Raffrenato E, Van Amburgh ME. 2010. Development of a mathematical model to predict sizes and rates of digestion of a fast and slow degrading pool and the indigestible NDF fraction. Proc Cornell Nutr. Conf for Feed Manufac; Oct 19–21; Syracuse, NY. p. 25–65.
- Ranck EJ, Holden LA, Dillon JA, Rotz CA, Soder KJ. 2020. Economic and environmental effects of double cropping winter annuals and corn using the integrated farm system model. J Dairy Sci. 103(4):3804–3815
- Rinne M, Huhtanen P, Jaakkola S. 2002. Digestive processes of dairy cows fed silages harvested at four stages of grass maturity. J Anim Sci. 80(7):1986–1998.
- Robinson KL, Cheeke PR, Kelly JD, Patton NM. 1986. Effect of fine grinding and supplementation with hay on the digestibility of wheat bran by rabbits. J Appl Rabbit Res. 9:166–167.
- Statistical Analysis System (SAS). 2013. SAS User's Guide Statistics. Version 9.4. Cary, NC, USA: SAS Institute INC.
- Shao T, Ohba N, Shimojo M, Masuda Y. 2002. Dynamics of early fermentation of Italian ryegrass (Lolium multiflorum Lam.) silage. Asian – Australasian. J Anim Sci. 15:1606–1610.
- Shao T, Zhang ZX, Shimojo M, Wang T, Masuda Y. 2005. Comparison of fermentation characteristics of Italian ryegrass (Lolium multiflorum Lam.) and guinea grass (Panicum maximum Jacq.) during the early stage of ensiling. Asian Australas J Anim Sci. 18(12):1727–1734.
- Susmel P, Stefanon B, Mills CR, Spanghero M. 1990. Rumen degradability of organic matter, nitrogen and fibre fractions in forages. Anim Sci. 51(3):515–526.
- Turgut L, Yanar M. 2004. In situ dry matter and crude protein degradation kinetics of some forage in Eastern Turkey. Small Rumin Res. 52(3):217–222.
- Turner LB, Cairns AJ, Armstead P, Ashton J, SkØt K, Whittaker D, Humphreys MO. 2006. Dissecting the regulation of fructan metabolism in perennial ryegrass (Lolium perenne) with quantitative trait locus mapping. New Phytol. 169(1):45–58.
- Ülger I, Kaplan M. 2017. Effects of ensiling duration on chemical composition of maize silages. European J Sus Dev Res. 2:63–65.
- Valderrama XL, Anrique RG. 2011. In situ rumen degradation kinetics of high-protein forage crops in temperate climates. Chilean J Agric Res. 71:572–577.
- Van Soest PJ, Robertson JB, Lewis BA. 1991. Methods for dietary fiber, neutral detergent fiber, and non-starch polysaccharides in relation to animal nutrition. J Dairy Sci. 74(10):3583–3597.
- Weisbjerg MR, Koukolová V, Lund P. 2007. Rate of NDF degradation. J Anim Feed Sci. 16(Suppl. 2):151–155.
- Wilkins RJ. 2005. Silage: a global perspective. In: Reynolds SG, Frame J, editors. Grasslands. Developments, opportunities, perspectives. Enfield, NH, USA: Science Publisher Inc. p. 111–132.
- Zebeli Q, Aschenbach JR, Tafaj M, Boguhn J, Ametaj BN, Drochner W. 2012. Role of physically effective fiber and estimation of dietary fiber adequacy in high-producing dairy cattle: invited review. J Dairy Sci. 95(3):1041–1056.