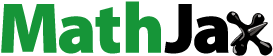
Abstract
Nowadays, the development of phytobiotics, an antibiotic-replacing agent, has attracted a considerable attention as poultry feed additive. The present study aims to develop the microcapsules loaded with a blend of essential oils (thyme, peppermint, savoury, and black pepper), and evaluate its health-promoting activity among the Clostridium perfringens-challenged broiler chickens. Based on the results, the spherical microcapsules containing a blend of thyme, summer savoury, peppermint, and black pepper essential oils had the particle size of 158.6 µm and encapsulation efficiency of 94.2%. The major bioactive compounds were thymol, carvacrol, p-cymene, and γ-terpinene. The microencapsulation of essential oil effectively prevented the release of bioactive phenolic compounds in the upper parts of the GIT. Additionally, it increased the delivery of the phenolic compounds to the lower segments of the GIT in the broiler chickens. The administration of the essential oil-loaded microcapsules (0.5, 1, and 2 kg/ton) in the C. perfringens-infected broiler chickens enhanced growth parameters, total feed intake, FCR, body antioxidant status, ileum morphostructure, and intestinal microbial population. Further, this treatment resulted in regulating the antioxidant and inflammation genes in ileum tissue. Consequently, the essential oil-loaded microcapsules can be considered as a promising phytobiotic against C. perfringens infection in broiler chickens.
The spherical microcapsules containing a blend of EO had the particle size of 158.6 µm and encapsulation efficiency of 94.2%.
The microencapsulation of essential oil effectively prevented the release of bioactive phenolic compounds in the upper parts of the GIT.
The EO-loaded microcapsules led to an improvement in growth parameters, body antioxidant status, ileum morphostructure, and intestinal microbial population.
The microcapsules regulated the antioxidant and inflammation genes in ileum tissue.
HIGHLIGHTS
Introduction
Clostridium perfringens is a gram-positive spore-forming bacterium, which exists in many environmental sources, as well as the intestines of humans and animals, which is found commonly on raw meat and poultry, and eggshells, as well as sometimes on paper. Under ideal conditions, this bacterium can multiply rapidly and produce a toxin in the intestine, which causes sickness (Bortoluzzi et al. Citation2019). Clostridium perfringens leads to injury in the intestinal mucosa, disrupts the villus-crypt, reduces nutrient digestion and absorption, and finally impairs the growth performance of chickens (Fasina and Lillehoj Citation2019). The use of in-feed antibiotics is known as the main strategy for controlling the bacterium in poultry. The enzymes, probiotics, prebiotics, and essential oils (EO) are among the alternatives. In addition, EO consumption would be the best choice due to consumer preference for natural products (Du et al. Citation2015). Recently, the utilisation of the dietary plant obtained from natural bioactive compounds (phytobiotics) such as EOs has attracted increased attention to an enhancement in performance and health in poultry production. Herbal EOs can be applied as an antibiotic alternative to get better animal performance, mostly in intensive management systems (Nouri Citation2019). EOs are the complex mixtures of volatile compounds, which are sometimes produced through employing physical methods (pressing and distillation) from a whole or parts of a plant with known taxonomic origin (Chowdhury et al. Citation2018; Zhai et al. Citation2018). Those with low side effects and high treatment properties are considered as a suitable alternative to chemical drugs (Wang et al. Citation2019). The antibacterial properties of EO have been well identified and widely tested in vitro against a wide range of pathogenic bacteria including both gram-positive and -negative ones. The previous studies have reported the greatest bactericidal activities in the constituents having a phenolic structure such as carvacrol and thymol although the antibacterial mechanism of EO has not been established (Veldhuizen et al. Citation2006). Further, microencapsulation significantly increases the beneficial effects of EOs (for in-feed use), especially on immunity, gut microbial populations, and performance in broiler chickens (Nouri Citation2019). The antimicrobial properties of citrus and herbs have been documented as effective against several bacterial pathogens such as C. perfringens (Yang et al. Citation2016). Carvacrol, a phenolic compound, is one of the essential components of certain EOs, which exhibits antimicrobial activity (Veldhuizen et al. Citation2006; Witkowska et al. Citation2019). Furthermore, thymol can retain the gut and host healthy, promote broiler performance (Wang et al. Citation2019), keep intestinal microflora stabilisation, represent effective anti-inflammatory and antioxidant activities (Yu et al. Citation2018). In the present study, the efficient compounds of thyme (Thymus vulgaris), savoury (Satureja hortensis), peppermint (Mentha piperita), and black pepper seeds (Piper nigrum) were screened, formulated, and encapsulated against poultry pathogenic microorganisms. Thymol in satureja, savoury as a main component of oil, plays an important role in antimicrobial properties. It improves digestion and absorption in the gastrointestinal tract, and helps to control intestinal infections. This compound enhances wall permeability and disrupts cell wall function by affecting microorganism cell wall. Thus, the duplication of many microorganisms is inhibited (Veiga et al. Citation2019). Microencapsulation is a method in which tiny particles or droplets are surrounded by a coating wall to make small capsules. Additionally, it is a process of building a barrier between the core and wall materials to keep from chemical and physical reactions, as well as maintaining the biological, functional, and physicochemical properties of the materials in core (Ushiyama et al. Citation2018). The EO decreases their activity through external factors and environmental destruction such as light, moisture, air, or heat (Garcia et al. Citation2018). The EO microencapsulation is considered as necessary and standardised for protection, as well as declining degradation by limiting the exposure to oxidation, temperature, moisture, and light during storage and processing (Islam et al. Citation2018). Many techniques such as freeze-drying, emulsification, spray drying, fluidized-bed coating, and supercritical fluid technologies are employed for encapsulation, among which spray drying was utilised in the present study. The enteric diseases are a major concern for the broiler production industry because of increasing mortality, diminishing production efficiency, and contaminating the meat for human consumption. The necrotic enteritis in boiler chicken is potentially fatal so that the total flock mortality rate reaches up to 30%. The necrotic enteritis diseases are caused by C. perfringens type A. The factors such as coccidiosis and infectious bursal disease virus, as well as the high dietary levels of wheat and fish meal may disturb the normal intestinal flora, which may favour the proliferation of the bacteria to reach a critical population of about 107–109 cp/g of digesta (Fasina and Lillehoj Citation2019). In this case, a lower growth rate and a higher feed conversion ratio (FCR) are observed.
Antibiotics, ionophores, and anticoccidials are commonly used for controlling C. perfringens population. The results of the recent studies indicated the developed antibiotic resistance among the bacterial strains. Thus, a safe alternative is required to control the proliferation of C. perfringens in the gastrointestinal tract of broiler chicken due to the concern with the effect of utilising antibiotic in animal feed on public health, as well as the approaching ban of their use (Mitsch et al. Citation2004). Some researchers suggested the potential inhibition of the bacterial growth and proliferation by T. vulgaris, Origanum vulgare, Syzygium aromaticum, and P. nigrum with the active compounds of thymol, carvacrol, eugenol, and limonene, respectively (Mitsch et al. Citation2004). This bacterium is normally a part of intestinal microbiome and produces various extracellular toxins. The mortality and growth inhibition occur when C. perfringens overgrows in the small intestine and produces the extracellular toxins which damage the intestine (Feng et al. Citation2010). This study developed the microcapsules loaded with a blend of EOs (thyme, peppermint, savoury, and black pepper) and assessed their health-promoting activity among the C. perfringens-challenged broiler chickens.
Materials and method
Materials
The dried aerial parts of thyme (T. vulgaris), peppermint (M. piperita), savoury (S. hortensis), and black pepper seeds (P. nigrum) were purchased from the herbal medicine market of Mashhad, Iran. The whey protein concentrate 80% (WPC) (Milk product, Auckland, New Zealand), maltodextrin (MD) (DE = 18–20, Foodchem, China), and modified starch (MS) (HI-CAP® 100, Ingredion, Humberg, Germany) were applied as wall materials. In addition, C. perfringens bacteria, isolated from poultry farm, and known as a poultry and foodborne pathogen, was obtained from the microbial culture collection of Razi Vaccine and Serum Research Institute (RTCC 1621), Karaj, Iran. The 2,2-diphenyl-1-picrylhydrazyl (DPPH) and acid-washed glass bead (G8772) were provided by Sigma (Germany). All other solvents and chemicals were prepared from Merck, Germany.
Bioactive compound extraction and encapsulation
Each of the dried plant materials was individually ground and subjected to hydrodistillation process on a Clevenger-type apparatus to extract its bioactive EO according to European Pharmacopoeia 6.0 in 2008 (Taraj et al. Citation2019). The obtained EO was dried over anhydrous sodium sulphate (Na2SO4) prior to encapsulation process.
Based on the results of in vitro antibacterial studies, the EOs derived from thyme, savoury, peppermint, and black pepper were mixed at a ratio of 50:25:12.5:12.5, respectively (Data not shown here). Further, a laboratory spray dryer (Model 191, Buchi, Switzerland) was utilised to encapsulate the mixed EOs as previously reported by Sablania et al. (Citation2018). The wall materials with the WPC:MS:MD ratio of 20:25:55 were dissolved in 150 mL of distilled water and stirred for 24 h at room temperature. Furthermore, 7.5 g of mixed EOs and 1% Tween 80 were gradually added to wall material solution with stirring at 10,000 xg for 5 min by using a rotor-stator high-speed stirring apparatus. The solution was homogenised with an Ultra-Turrax homogeniser (IKA, Germany), followed by spray drying at 10,000 xg for 5 min. The spray dryer inlet and outlet temperature was set at 130 and 80 °C, respectively. The pressure was adjusted at 0.4 kg/cm2 and flow rate was 8 mL/min. Finally, the dried powder was stored at room temperature for further analysis.
Microcapsule characterisation
The microencapsulated blend of EOs was characterised for their particle size, surface and retention oil, and encapsulation efficiency (EE) according to Jafari, Assadpoor, Bhandari, et al. (Citation2008) and Jafari, Assadpoor, He, et al. Citation2008). Additionally, the hydrodistillation method using Clevenger apparatus was employed to determine the total oil content of the microcapsules (Jafari et al. Citation2007). The oil retention was calculated by using the following formula (Jafari et al. Citation2007).
The particle size and polydispersity index (PDI) were obtained through applying dynamic light scattering (DLS) on a Zetasizer (Malvern, UK) (Jafari, Assadpoor, He, et al. Citation2008). EE is defined as the amount of EO trapped inside the microcapsules and computed as follows (Jafari et al. Citation2007).
where total oil represents a combination of the internal and surface oil contents of the microcapsules, and surface oil refers to the unencapsulated oil at the surface of the microcapsules. The scanning electron microscopy (SEM) analysis was performed to determine the morphology and microstructure properties of the microcapsules (Goyal et al. Citation2015).
In vitro gastrointestinal release study
The gastric resistance and release behaviour of microcapsules were examined in simulated gastric (SGF) and intestinal fluids (SIF) based on the procedure provided by Zhang et al. (Citation2014) with slight modifications. SGF was prepared by adding 3.2 g/L of pepsin into 2 g/L of sodium chloride and adjusting pH at 1.5. Regarding SIF, 10 g/L of pancreatin was dissolved in 0.05 M KH2PO4 and pH was set at 7.4. Further, 100 mg of microcapsules was mixed with 9 mL of SGF in a glass test tube, followed by shaking at 300 rpm for 1 h at 39 °C. At the end of incubation time, the test tubes were centrifuged at 3000×g for 30 min at 20 °C. The supernatant was gathered, from which phenolic compounds were extracted with 5 mL of ethyl acetate to collect the released phenolic compounds. Then, 15 mL of SIF was poured to the pellet and shaken at 300 rpm for 2 h while incubating at 39 °C. After incubation, the tubes were centrifuged at 3000×g for 30 min at 20 °C, the supernatant of which was collected and extracted by using 10 mL of ethyl acetate. The total phenolic compound was obtained through using Folin-Ciocalteu method as described earlier.
Preparation of C. perfringens inoculant
C. perfringens was anaerobically subcultured to a fresh Mueller-Hinton broth for three consecutive days. In addition, the 10-fold dilutions of the bacterial suspension were prepared and cultured on a Mueller-Hinton agar containing 5% (V/V) sheep blood under an anaerobic condition (85% N2, 10% CO2, and 5% H2). They were incubated at 37 °C to confirm the number of colony-forming units (CFU) per mL of broth. Then, the bacteria were diluted with 0.1% peptone water, and utilised in a chicken trial for infection through oral gavage on day 18 with 2 mL of peptone water containing 1 × 108 CFU of C. perfringens.
Chicken trials and sampling
This experiment was carried out after approving by the Animal Ethics Committee of the Agricultural Biotechnology Research Institute of Iran. A total of 350 one-day-old male broiler chickens (Ross 308) were obtained from Fariman broiler Breeder Company (Mashhad, Iran). The chickens were reared in the pen system under hygienic conditions in a ventilated and temperature-controlled area. Further, they were randomly allocated into seven treatments with five replicates (10 chickens in each replicate) (Table ). The diet and water were supplemented ad libitum (Table ). The birds in the groups T3. T4, and T5 were fed the same basal diets supplemented with encapsulated phytobiotics at the rates of 0.5, 1 and 2% per ton of feed, respectively. Similarly, the birds in the groups T6 and T7 were offered the same basal diet supplemented with a commercial phytobiotics called Digestarom (Biomin GmbH, Herzogenburg, Austria) and oxytetracycline (Razak, Tehran, Iran) at the inclusion levels of 1 and 0.4% per Kg of the feed, respectively. The chicken infection included oral gavaging on day 18 with 2 mL of peptone water containing 1 × 108 CFU of C. perfringens. On the same date, 2 mL of sterile peptone water was orally gavaged in the uninfected group. The experiment lasted 23 days and the parameters of final weight, FCR, and total feed intake were determined. The chicks were euthanized through cervical dislocation on day 23. Additionally, blood samples were taken to measure immunoglobulin G (IgG) and A (IgA), and liver enzymes such as alanine aminotransferase (ALT) and aspartate aminotransferase (AST). The malondialdehyde level of the breast meat and serum was obtained to evaluate lipid peroxidation (Hafez et al. Citation2020) and their redox potential was specified by using 2,2-diphenyl-1-picrylhydrazyl (DPPH) free radicals (Antonini et al. Citation2020). The Folin-Ciocalteu method was applied to determine the total phenolic content of the breast meat (Jung et al. Citation2010). The lipid peroxidation, redox potential, and total phenolic level in serum and breast meat were expressed as percentage changes relative to the control group. Further, the morphostructural characteristics of ileum like villus height and width, crypt depth, and goblet cell count were assessed on ileum tissues based on the standard histopathological protocols (Poloni et al. Citation2020). The pH of ileum and caecum digesta was measured. The ileum digesta were collected and kept at −20 °C for the molecular quantification of lactic acid bacteria (LABs) and C. perfringens. Following euthanasia, the ileum tissues were immediately sampled and frozen in the liquid nitrogen to analyse the expression of genes such as occludin, inducible nitric oxide synthase (iNOS), and superoxide dismutase (SOD).
Table 1. Experimental treatments.
Table 2. Diet ingredients and chemical analysis.
Gene expression analysis
The present study examined the expression of the genes like occludin as a staple of tight junctions, SOD as an antioxidant, and iNOS as an important biomarker of inflammation in the ileum tissue. Briefly, the ileum tissues were separated and held in liquid nitrogen immediately after chicken euthanasia. In addition, their mRNA was extracted by using a RNeasy Mini Kit (Qiagen, Valencia, CA, USA) according to the kit protocol. The total RNA was converted to cDNA with a cDNA synthesis kit (BIOFACT, Korea). Further, SYBR Green (BIOFACT, Korea) was used to specify the expression of the intended genes (Beyrami et al. Citation2020). The genes were amplified as follows: initial denaturation at 95 °C for 5 min (1X), followed by 35 cycles of 95 °C for 30 s, primer annealing (at 58, 58, 60, and 56 °C for occludin, iNOS, SOD, and β-actin, respectively) for 25 s, and extension at 72 °C for 25 s (35X). The mRNA expression was determined through using ΔΔCt method. The gene expression was normalised to β-actin as a reference gene and then normalised to the respective genes in the control group (Sajjadi et al. Citation2019). The previously-published primers were considered for gene expression analysis, the characteristics of which are provided in Table .
Table 3. Characteristics of the primers used in this study, and ileal and caecum microbial population analysis.
Microbial population analysis
The fold changes in C. perfringens population in the ileum digesta were measured by using a real-time PCR platform (LightCycler 96 instrument, Roche, Basel, Switzerland). The PCR condition for the amplification of extracted DNA was initial denaturation (95 °C) for 5 min (1X), followed by 35 cycles (95 °C) for 20 s, primer annealing (58, 58, and 55 °C for C. perfringens, LABs, and total bacteria, respectively) for 20 s, and extension (72 °C) for 25 s. This study utilised QIAamp DNA stool extraction kit (QIAGEN, Germany) for extracting DNA from ileum digesta, as well as SYBR GREEN master mix (BIOFACT, Korea). The quantitative real-time PCR assay was carried out by using the previously-published primers. Table summarises the characteristics of the primers. Then, real-time PCR data were analysed through employing ΔΔCt method to determine the fold changes in C. perfringens and LAB populations, and presented relative to total bacteria (Feng et al. Citation2010).
Table 4. List of the primers applied for ileum and caecum microbial population analysis.
Statistical analysis
All the data were analysed through applying the ANOVA using the GLM procedure of SAS (SAS 9.2, SAS Institute Inc., Cary, NC). Duncan's multiple test range was utilised for specifying the significant difference among the treatments. The statistical significance was considered at p < .05 and the results were reported as mean and SEM.
Results and discussion
Encapsulation and characterisation
The particle size, zeta potential, and PDI were determined 158.6 nm, −42.6, and 0.13, respectively. The zeta potential ranging ±40 to ±60 indicated the good stability of the microcapsules in the colloidal dispersion. Additionally, the PDI value (0.13) was lower than 0.3, by representing homogeneous dispersion (Hasan et al. Citation2014). The surface and retention oil, and EE were respectively obtained 2.15, 80.62, and 94.2%. These results confirmed the good entrapment and loading efficiency of EO into MD, MS, and WPC as core wall materials. Further, the spray-dried microcapsules were mostly spherical with smooth surface, and the agglomeration of the particles was seemingly favourable (Figure ).
Phytochemical analysis
Table indicates the phytochemical content of the microcapsules. The gas chromatography-mass spectroscopy (GC-MS) analysis revealed the presence of thymol (42.39%), carvacrol (27.18%), p-cymene (14.73%), γ-terpinene (11.68%), menthol (8.43%), and caryophyllene (5.28%) as the major bioactive compounds loaded in the microcapsules. The results are consistent with those of other studies which confirmed the existence of these bioactive phenolics in the plants such as thyme (T. vulgaris), savoury (S. hortensis), peppermint (M. piperita), and black pepper seeds (P. nigrum) (Silva and Fernandes Júnior Citation2010).
Table 5. Main phytochemical contents of the microcapsules.
Study of in vitro gastrointestinal release
The encapsulation process can be considered as a promising choice to deliver EO bioactive compounds to a specific organ, as well as their protection from oxidation, volatilisation, and degradation. The encapsulation techniques include spray drying, emulsification, freeze-drying, fluidized-bed coating, extrusion, and supercritical fluid technologies. However, spray drying is most commonly utilised in the industry. Figure displays the percentage release of phenolic compounds from the microcapsules in SGF.
Figure 2. In vitro percentage release of phenolic compounds in the microcapsule core in simulated gastric fluid and simulated intestinal fluid.
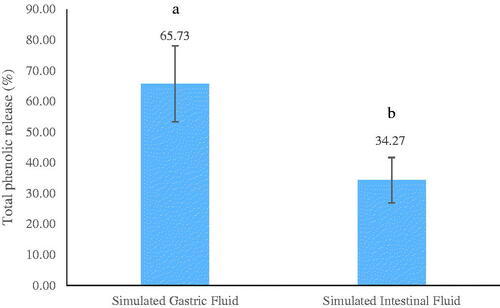
Based on the results, the release of phenolic compounds was initiated when immersing the microcapsules in SGF and SIF (containing protease, lipase, amylases, and dehydrated bile, pH 7). In addition, almost 65% of phenolic compounds in the core released during three-hour incubation despite the resistance of the matrices used for encapsulation against shrink at acidic pH, and consequently minimisation of the release of bioactive phenolics in the stomach. The release can be mainly attributed to the presence of bile salts and pH of the solution (7). Both reasons result in altering the hydrogen link between the phenolic compounds and MS, MD, or WPC, as well as the electrostatic bonding among wall materials. Further, the release of phenolic compounds in SGF can be partially related to the hydrolysis of wall materials by the digestive enzymes. Due to the lack of data on the appropriate release rate of encapsulated bioactive compounds in chicken gastrointestinal tract, it is difficult to give a clear-cut suggestion at this stage. However, reducing the release rate in the stomach and transferring bioactive compound release to the intestine are considered as the main goal.
Study of in vivo gastrointestinal release
The retention and disappearance rates of phenolic compounds related to the initial gavage dose in different gastrointestinal tract (GIT) sections are represented in Table . The dual comparisons were performed between the unencapsulated and encapsulated EOs in various GIT segments and time points when gavaging. The detection of phenolic compounds in all parts of the chicken GIT upon 1 h gavage with unencapsulated and encapsulated EOs reflected the quick passage of digesta in the chickens. This result is in line with that of other study reported feed transition time in broiler chicken as 2–5 h (Rochell et al. Citation2012). In general, the encapsulated EO exhibited a significantly (p < .05) higher retention rate in two time points compared to the unencapsulated one. A greater phenolic compound level was observed in the lower GIT segments (ileum, caecum, and colon) of the chickens gavaged with encapsulated EO in comparison with those receiving unencapsulated one. Thus, the encapsulated EO could remain longer in the GIT than the unencapsulated one did. The unencapsulated EO passed through GIT and disappeared by 48.98% upon 3 h from oral gavage, while the encapsulated EO disappearance reached up to 23.14% during the period. The disappearance of a large level of phenolic compounds in the GIT of the chickens treated with unencapsulated EO can be ascribed to the absorption, metabolism, or biotransformation of the compounds. The EO without a proper protection may not reach the lower GIT segments in the broiler chickens. The appropriate formulation of EO effectively prevents bioactive phenolic compounds from releasing in the upper GIT parts of the broiler chickens. Furthermore, it increases the concentration of the bioactive compounds which should be delivered to their lower GIT sections.
Table 6. Retention rates of phenolic compounds (%) in the digesta of the different GIT segments of 18-day-old broiler chickens after the oral gavage of unencapsulated and encapsulated EOs.
Animal trial
Table summarises the results of final weight, total feed intake, FCR. As shown, C. perfringens challenge (T2) significantly (p < .05) impairs final weight and total feed intake compared to the uninfected chickens (T1). The dietary addition of encapsulated EO at the concentrations of 0.5, 1, and 2 kg/ton results in raising final weight and total feed intake significantly (T3, T4, and T5) (p < .05). In addition, the synthesised microcapsules containing EO blend with 2 kg/ton concentration improved final weight, total feed intake, and FCR in comparison to the Digestarom PEP at 1 kg/ton concentration as a positive control (T6). The use of the synthetic antibiotics, oxytetracycline, at the concentration of 0.4 kg/ton (T7) leads to a higher final weight than the challenged treatment. However, this increase is not comparable to that caused by utilising the EO-loaded microcapsules (T5) and Digestarom PEP (T6). The results of a similar study revealed that EOs can be used as an alternative to AGP in commercial broiler diets to improve growth and nutrient utilisation while decreasing nitrogen excretion to the environment (6).
Table 7. Final weight, total feed intake, FCR, pH in the ileum and caecum of the differently-treated chickens.
Total phenolic content and antioxidant activity
Table represents the antioxidant activity of serum and breast meat, and total phenolic level of the meat. Based on the results, the dietary addition of microencapsulated EO with 1 and 2 kg/ton concentration significantly (p < .05) enhanced the total phenolic content of the breast meat. Therefore, C. perfringens infection (T2) increased lipid peroxidation in the meat and declined the redox potential of serum significantly (p < .05) compared to the unchallenged chickens (T1). Further, an improvement was obtained in the lipid peroxidation of the meat (p < .05) and the antioxidant properties of serum (p < .05) following the dietary supplementation of the EO-loaded microcapsules at the 1 and 2 kg/ton concentrations. These microcapsules (1 and 2 kg/ton) behaved similar to the Digestarom PEP with 1 kg/ton concentration (a positive control) in decreasing lipid peroxidation and promoting the antioxidant activity of serum and breast meat. The dietary supplementation of oxytetracycline as an antibiotic led to a reduction in lipid peroxidation compared to the challenged group (T2). The decline in lipid peroxidation can be associated with the antioxidant properties of oxytetracycline (Kładna et al. Citation2012).
Table 8. Antioxidant activity of serum and breast meat, and total phenolic content of the meat.
Table provides the results of liver enzymes (ALT and AST) and immunoglobulins (IgG and IgA). As demonstrated, C. perfringens challenge (T2) significantly (p < .05) increases both liver enzymes and suppresses IgG and IgA values compared to the uninfected group (T1). Greater ALT, AST, IgG, and IgA values are observed after treating with the EO-containing microcapsules (T3, T4, and T5) (p < .05). Furthermore, the EO-loaded microcapsules at 2 kg/ton concentration and Digestarom PEP (T6) (a positive control) result in producing more serum liver enzymes and immunoglobulins.
Table 9. Liver enzymes and immunoglobulins of serum, and total phenolics of breast meat.
Gene expression analysis
The expression analysis of occludin, iNOS,and SOD genes in the ileum tissue is outlined in Table . The occludin gene is considered as a main part of the tight junction complexes in the ileum, which regulate the permeability of epithelia. The iNOS is responsible in inflammation, while the SOD plays a major role in regulating redox potential in the cells. In fact, they are the key genes involved in permeability, inflammation, and antioxidant system. The results exhibited that C. perfringens infection suppressed (p < .05) the expression of occludin and SOD genes, while it upregulated (p < .05) iNOS gene expression. Despite the availability of few data about TJ protein expression in the C. perfringens-infected birds, Liu et al. reported a decreased occludin gene expression in the small intestine of the infected birds (Liu et al. Citation2012). The dietary application of the EO-loaded microcapsules led to a significant (p < .05) upregulation in the expression of occludin and SOD genes, as well as a downregulation in that of iNOS one. These results confirmed that C. perfringens challenge impaired nutrient absorption through ileum, diminished cellular redox potential, and triggered ileal inflammation. Based on the results in Table , a significant difference (p < .05) was found between T5 and T7. Thus, the use 2 kg per ton of encapsulated EO led to better results compared to the antibiotic oxytetracycline. The results are in agreement with those of the morphometric analysis of ileum and lipid peroxidation as the biomarkers of oxidative stress and inflammation and antioxidant activity of serum. According to Yun et al. test subjects with 300 or 400 μL of carvacrol EOs, the occludin gene expression promotes significantly (p < .05) in the mucosa of small intestine (Liu et al. Citation2018).
Table 10. Gene expression analysis of ileum in the treated groups.
Microbial population analysis
Table presents the ileum and caecum microbial population analysis. Comparing to the uninfected chickens, C. perfringens infection (T2) significantly (p < .05) enhanced C. perfringens population in the ileum and caecum by 5.9 and 3.9 folds, respectively. Additionally, a significant diminution was attained in the LAB population among the chickens infected by C. perfringens (T2) (p < .05). Along with decreasing (p < .05) C. perfringens population, the supplementation of the EO-containing microcapsules improved (p < .05) LABs population in both ileum and caecum, which resulted in producing more organic acids and obtaining lower pH in the caecum. The results of the caecal pH are consistent with those of LAB population. Further, they are in line with those of the early studies which indicated the synergistic effects of phytogenic feed additives and LABs, and antagonistic effects of the additives against enteropathogens in the intestine of broiler chickens (Applegate et al. Citation2010; Scocco et al. Citation2017; Ren et al. Citation2019). The EO-loaded microcapsules inhibited C. perfringens population compared to the Digestarom PEP and oxytetracycline. Accordingly, the developed microcapsules can play the role of a natural phytobiotic.
Table 11. Ileum and caecum microbial population analysis using real-time PCR among the treated groups.
Morphometric analysis
Table illustrates the morphometric analysis of the ileum. As shown, C. perfringens infection (T2) impairs (p < .05) villus height and width, and V:C ratio (ratio of villus height to crypt depth). Following the dietary addition of the EO-loaded microcapsules, a significant (p < .05) enhancement was observed in villus height and width, V:C ratio, and crypt depth. The microcapsules behaved similarly to Digestrom PEP and oxytetracycline as positive controls. Song et al. outlined a significant rise in the goblet cell content in small intestine epithelium (p < .05) test subjects with 400 μl carvacrol EOs (Liu et al. Citation2018). Along with benefiting the intestinal microbiome, prebiotics such as organic acids and EOs may promote the integrity of intestinal epithelial cells, which further increase nutrient absorption and growth performance in animals (6).
Table 12. Ileum morphology and goblet cell count in the differently-treated chickens.
Conclusion
The EO microencapsulation efficiently prevented bioactive phenolic compounds from releasing in the upper GIT parts of the broiler chickens. In addition, it resulted in delivering more phenolic compounds to the lower GIT segments. An increase was detected in growth parameters, total feed intake, FCR, body antioxidant status, ileum morphostructure, and intestinal microbial population by administrating the EO-loaded microcapsules at the dose of 0.5, 1, and 2 kg/ton in the C. perfringens-challenged broiler chickens. Further, this treatment regulated antioxidant and inflammation genes in ileum tissue. Consequently, the EO-loaded microcapsules can be considered as a promising phytobiotic against C. perfringens infection in broiler chickens.
Ethical approval
All experimental procedures were approved by the Animal Care Committee of the Agricultural Biotechnology Research Institute of Iran.
Acknowledgments
The authors would like to thank Islamic Azad University, Kashmar Branch, as well as Agricultural Research, Education, and Extension Organization (AREEO), Mashhad Iran.
Disclosure statement
No potential conflict of interest was reported by the author(s).
References
- Antonini E, Torri L, Piochi M, Cabrino G, Meli MA, De Bellis R. 2020. Nutritional, antioxidant and sensory properties of functional beef burgers formulated with chia seeds and goji puree, before and after in vitro digestion. Meat Sci. 161:108021.
- Applegate TJ, Klose V, Steiner T, Ganner A, Schatzmayr G. 2010. Probiotics and phytogenics for poultry: Myth or reality? J Appl Poult Res. 19(2):194–210.
- Beyrami M, Karimi E, Oskoueian E. 2020. Synthesized chrysin-loaded nanoliposomes improves cadmium-induced toxicity in mice. Environ Sci Pollut Res Int. 27(32):40643–40649.
- Bortoluzzi C, Lumpkins B, Mathis GF, França M, King WD, Graugnard DE, Dawson KA, Applegate TJ. 2019. Zinc source modulates intestinal inflammation and intestinal integrity of broiler chickens challenged with coccidia and Clostridium perfringens. Poultr Sci. 98(5):2211–2219.
- Chen J, Tellez G, Richards JD, Escobar J. 2015. Identification of potential biomarkers for gut barrier failure in broiler chickens. Front Vet Sci. 2:14.
- Chowdhury S, Mandal GP, Patra AK, Kumar P, Samanta I, Pradhan S, Samanta AK. 2018. Different essential oils in diets of broiler chickens: 2. Gut microbes and morphology, immune response, and some blood profile and antioxidant enzymes. Anim Feed Sci Technol. 236:39–47.
- Du E, Gan L, Li Z, Wang W, Liu D, Guo Y. 2015. In vitro antibacterial activity of thymol and carvacrol and their effects on broiler chickens challenged with Clostridium perfringens. J Anim Sci Biotechnol. 6(1):58.
- Fasina YO, Lillehoj H. 2019. Characterization of intestinal immune response to Clostridium perfringens infection in broiler chickens. Poultr Sci. 98(1):188–198.
- Feng Y, Gong J, Yu H, Jin Y, Zhu J, Han Y. 2010. Identification of changes in the composition of ileal bacterial microbiota of broiler chickens infected with Clostridium perfringens. Vet Microbiol. 140(1–2):116–121.
- Garcia CM, Fernandez M, Lopez OD, Castiñeira M, Martinez B, Nogueira A, Turiño L. 2018. Microencapsulation of shark liver oil pool by spray drying. LAAR. 48(2):89–93.
- Goyal A, Sharma V, Sihag MK, Tomar SK, Arora S, Sabikhi L, Singh AK. 2015. Development and physico-chemical characterization of microencapsulated flaxseed oil powder: a functional ingredient for omega-3 fortification. Powder Technol. 286:527–537.
- Hafez A, Nassef E, Fahmy M, Elsabagh M, Bakr A, Hegazi E. 2020. Impact of dietary nano-zinc oxide on immune response and antioxidant defense of broiler chickens. Environ Sci Pollut Res Int. 27(16):19107–19108.
- Hasan M, Belhaj N, Benachour H, Barberi-Heyob M, Kahn CJF, Jabbari E, Linder M, Arab-Tehrany E. 2014. Liposome encapsulation of curcumin: physico-chemical characterizations and effects on MCF7 cancer cell proliferation. Int J Pharm. 461(1-2):519–528.
- Islam M, Mahmud N, Nawas T, Fang Y, Xia W. 2018. Health benefits and spray drying microencapsulation process of fish oil (omega-3). Am J Food Sci Nutr Res. 5(2):29–42.
- Jafari SM, Assadpoor E, Bhandari B, He Y. 2008. Nano-particle encapsulation of fish oil by spray drying. Food Res Int. 41(2):172–183.
- Jafari SM, Assadpoor E, He Y, Bhandari B. 2008. Encapsulation efficiency of food flavours and oils during spray drying. Drying Technol. 26(7):816–835.
- Jafari SM, He Y, Bhandari B. 2007. Encapsulation of nanoparticles of d-limonene by spray drying: role of emulsifiers and emulsifying techniques. Drying Technol. 25(6):1069–1079.
- Jung S, Choe JH, Kim B, Yun H, Kruk ZA, Jo C. 2010. Effect of dietary mixture of gallic acid and linoleic acid on antioxidative potential and quality of breast meat from broilers. Meat Sci. 86(2):520–526.
- Kładna A, Michalska T, Berczyński P, Kruk I, Aboul-Enein HY. 2012. Evaluation of the antioxidant activity of tetracycline antibiotics in vitro. Luminescence. 27(4):249–255.
- Liu D, Guo S, Guo YJAP. 2012. Xylanase supplementation to a wheat-based diet alleviated the intestinal mucosal barrier impairment of broiler chickens challenged by Clostridium perfringens. Avian Pathology. 41(3):291–298.
- Liu S, Song MHo, Yun W, Lee J, Lee CHee, Kwak WGi, Han NSoo, Kim HBum, Cho JHo. 2018. Effects of oral administration of different dosages of carvacrol essential oils on intestinal barrier function in broilers. J Anim Physiol Anim Nutr. 102(5):1257–1265.
- Mitsch P, Zitterl-Eglseer K, Köhler B, Gabler C, Losa R, Zimpernik I. 2004. The effect of two different blends of essential oil components on the proliferation of Clostridium perfringens in the intestines of broiler chickens. Poultr Sci. 83(4):669–675.
- Nouri A. 2019. Chitosan nano-encapsulation improves the effects of mint, thyme, and cinnamon essential oils in broiler chickens. British Poultry Science. 60(5):530–538.
- Poloni V, Magnoli A, Fochesato A, Cristofolini A, Caverzan M, Merkis C, Montenegro M, Cavaglieri L. 2020. A Saccharomyces cerevisiae RC016-based feed additive reduces liver toxicity, residual aflatoxin B1 levels and positively influences intestinal morphology in broiler chickens fed chronic aflatoxin B1-contaminated diets. Animal Nutrition. 6(1):31–38.
- Ren H, Vahjen W, Dadi T, Saliu E-M, Boroojeni FG, Zentek J. 2019. Synergistic Effects of probiotics and phytobiotics on the intestinal microbiota in young broiler chicken. Microorganisms. 7(12):684.
- Rezaei S, Faseleh Jahromi M, Liang JB, Zulkifli I, Farjam AS, Laudadio V, Tufarelli V. 2015. Effect of oligosaccharides extract from palm kernel expeller on growth performance, gut microbiota and immune response in broiler chickens. Poultr Sci. 94(10):2414–2420.
- Rochell SJ, Applegate TJ, Kim EJ, Dozier WA. 2012. Effects of diet type and ingredient composition on rate of passage and apparent ileal amino acid digestibility in broiler chicks. Poultr Sci. 91(7):1647–1653.
- Sablania V, Bosco SJD, Rohilla S, Shah MA. 2018. Microencapsulation of Murraya koenigii L. leaf extract using spray drying. Food Measure. 12(2):892–901.
- Sajjadi M, Karimi E, Oskoueian E, Iranshahi M, Neamati A. 2019. Galbanic acid: induced antiproliferation in estrogen receptor-negative breast cancer cells and enhanced cellular redox state in the human dermal fibroblasts. J Biochem Mol Toxicol. 33(11):e22402.
- Scocco P, Forte C, Franciosini MP, Mercati F, Casagrande-Proietti P, Dall'Aglio C, Acuti G, Tardella FM, Trabalza-Marinucci M. 2017. Gut complex carbohydrates and intestinal microflora in broiler chickens fed with oregano (Origanum vulgare L.) aqueous extract and vitamin E. J Anim Physiol Anim Nutr. 101(4):676–684.
- Silva NCC, Fernandes Júnior A. 2010. Biological properties of medicinal plants: a review of their antimicrobial activity. J Venom Anim Toxins Incl Trop Dis. 16(3):402–413.
- Tang D, Wu J, Jiao H, Wang X, Zhao J, Lin H. 2019. The development of antioxidant system in the intestinal tract of broiler chickens. Poult Sci. 98(2):664–678.
- Taraj K, Malollari I, Ciko L, Llupa J, Ylli A, Ylli F, Andoni A. 2019. Water distillation extraction of essential oil from Sideritis raeseri herb. Environ Process. 6(4):1051–1058.
- Teshfam M, Brujeni GN, Hassanpour H. 2006. Evaluation of endothelial and inducible nitric oxide synthase mRNA expression in the lung of broiler chickens with developmental pulmonary hypertension due to cold stress. Br Poult Sci. 47(2):223–229.
- Ushiyama T, Shimizu NJFS, Research T. 2018. Microencapsulation using spray-drying: the use of fine starch solution for the wall material. FSTR. 24(4):653–659.
- Veiga RDSD, Aparecida Da Silva-Buzanello R, Corso MP, Canan C. 2019. Essential oils microencapsulated obtained by spray drying: a review. J Essent Oil Res. 31(6):457–473.
- Veldhuizen EJA, Tjeerdsma-van Bokhoven JLM, Zweijtzer C, Burt SA, Haagsman HP. 2006. Structural requirements for the antimicrobial activity of carvacrol. J Agric Food Chem. 54(5):1874–1879.
- Wang H, Liang S, Li X, Yang X, Long F, Yang X. 2019. Effects of encapsulated essential oils and organic acids on laying performance, egg quality, intestinal morphology, barrier function, and microflora count of hens during the early laying period. Poultr Sci. 98(12):6751–6760.
- Witkowska D, Sowińska J, Murawska D, Matusevičius P, Kwiatkowska-Stenzel A, Mituniewicz T, Wójcik A. 2019. Effect of peppermint and thyme essential oil mist on performance and physiological parameters in broiler chickens. SA J Sci. 49(1):29–39.
- Yang Y, Wang Q, Diarra MS, Yu H, Hua Y, Gong J. 2016. Functional assessment of encapsulated citral for controlling necrotic enteritis in broiler chickens. Poult Sci. 95(4):780–789.
- Yu C, Wei J, Yang C, Yang Z, Yang W, Jiang S. 2018. Effects of star anise (Illicium verum Hook. f.) essential oil on laying performance and antioxidant status of laying hens. Poultr Sci. 97(11):3957–3966.
- Zhai H, Liu H, Wang S, Wu J, Kluenter A-M. 2018. Potential of essential oils for poultry and pigs. Anim Nutr. 4(2):179–186.
- Zhang Y, Gong J, Yu H, Guo Q, Defelice C, Hernandez M, Yin Y, Wang Q. 2014. Alginate-whey protein dry powder optimized for target delivery of essential oils to the intestine of chickens. Poult Sci. 93(10):2514–2525.