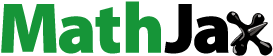
Abstract
Here, we investigated the effects of fermented Chenopodium album L. (FCAL) on growth performance, apparent total tract nutrient digestibility, serum immunity, carcase characteristics and meat quality of broilers. Arbour Acres broilers (160, 1-d-old) were randomly allocated into four treatment groups with five replicates of eight birds each. The birds were fed a corn–soybean meal basal diet supplemented with 0 (control [CON]), 2, 4 and 8 g/kg FCAL. During the starter period (days 1–21), 4 and 8 g/kg FCAL significantly increased the average daily gain (ADG), apparent digestibility of dry matter (DM), crude protein (CP) and ether extract (EE), moreover; 8 g/kg FCAL significantly decreased the feed conversion ratio (FCR). During the finisher period (days 22–42), 2 g/kg FCAL significantly increased ADG and apparent digestibility of DM, CP and EE but significantly decreased FCR. During the whole period (days 1–42), dietary supplementation of 4 and 8 g/kg FCAL significantly increased ADG. Compared with the CON group, in the FCAL groups, the serum interleukin (IL)-1β and IL-8 levels were lower, while insulin-like growth factor-1, immunoglobulin (Ig) A (IgA) and IgM levels were higher. The serum IL-10 content was lower in the 2 and 4 g/kg FCAL groups. The breast muscle percentage significantly increased with 8 g/kg FCAL. FCAL significantly decreased muscle drip loss (breast), shear force (breast), water loss rate and lightness (leg). FCAL had positive effects on growth, nutrient digestibility, immunity, carcase characteristics and meat quality of broilers, thus it could be a reliable and phytogenic feed additive for promoting growth and maintaining health of broilers.
Fermented Chenopodium album L. (FCAL) can improve growth performance and apparent nutrient digestibility of broilers.
FCAL can promote immune responses and enhance carcase characteristics and meat quality of broilers.
FCAL could be a reliable and potential phytogenic feed additive to promote growth and maintain the health of broilers.
Highlights
Introduction
Currently, many countries, including China, have banned the utilisation of antibiotics as a growth promotor in animal feed to avoid resistant bacteria emergence and residues in animal-derived products (Ye et al. Citation2020; Mohebodini et al. Citation2021; Wang et al. Citation2021). Before the development of antibiotics, medicinal herbs were used for health and medical purposes for almost 60,000 years (Kuralkar P and Kuralkar SV Citation2021). In recent years, natural herbal products (HPs) have been found to possess various biological activities, such as antimicrobial, anthelmintic, antioxidative, growth promotion and immune modulation (Upadhaya and Kim Citation2017). Considering the safety and efficacy of HPs, they have been widely used as feed additives. In poultry diets, it has been reported that HPs have positive effects on growth performance (Ashour et al. Citation2020; Redoy et al. Citation2021). Additionally, HPs could improve the apparent digestibility of broilers by stimulating saliva and bile secretions and enhancing digestive enzyme activity (Lee et al. Citation2003; Platel and Srinivasan Citation2004). The pathogen load-reducing effect of HPs in the gut of broilers was also investigated by Long et al. (Citation2020) and De Lange et al. (Citation2010). Additionally, HPs improve the dressing percentage (Al-Kassie Citation2010) and meat quality of broilers, as indicated by increased pH, redness and water holding capacity and decreased lightness and shear force (Rahman and Kim Citation2016).
Chenopodium album L. (CAL), a common wild herbal medicinal plant, is widely distributed globally and withstand harsh soil and climatic conditions, such as freezing, high salt concentration and drought (Chen and Jia Citation2002; Chen Citation2006; Jan et al. Citation2017). CAL plays an important role in the prevention and treatment of diseases (Singh et al. Citation2015; Rashmi Citation2016; Sikarwar et al. Citation2017), owing to its antibacterial (Korcan et al. Citation2013), antioxidant, anti-inflammatory (Amodeo et al. Citation2019) and anthelmintic properties (Jabbar et al. Citation2007), which are realised by secondary metabolites. CAL has been found to contain several secondary metabolites, such as polyphenols, polysaccharides, flavonoids and other nutritional substances (Cutillo et al. Citation2004; He et al. Citation2018; Arora and Itankar Citation2018). Furthermore, a previous study showed that polyphenols, flavonoids and saponins from CAL can inhibit NF-κB protein expression, consequently relieving joint inflammation and significantly returning the body weight of arthritic rats to normal levels (Arora et al. Citation2014). Sikarwar et al. (Citation2017) also found that feeding 0.4 g/kg aqueous extract of CAL to rats affected by kidney stones for 28 d prevented body weight loss and resulted in the regaining of the relative body weight to near-normal levels. Free-range animals (chickens, ducks, geese, pigs, sheep and cattle) often freely feed on CAL in rural areas. However, Fairley et al. (Citation2012) indicated that excessive intake of CAL could result in hypocalcaemia, allergic symptoms, and even death. The palatability, nutrient absorption and utilisation of CAL are affected by its high level of antinutritional factors, such as pectin (0.93–1.52 mg/g), phytic acid (7.4–8.1 mg/g), saponins (2.1–2.7 mg/g) and tannins (1.15–1.21 mg/g) (Dongowski et al. Citation1992; Pachauri et al. Citation2017). Exogenous enzymes, such as pectinase and phytase, have been proven to degrade antinutritional factors of medicinal herbs and prevent growth inhibition, allergic symptoms and death in animals (Kerovuo et al. Citation1998; Gupta et al. Citation2005; Pachauri et al. Citation2017).
It has been proven that the fermentation of medicinal herbs with probiotics, such as Bacillus subtilis, could eliminate anti-nutritional factors (Hmani et al. Citation2017) and degrade macromolecular materials into micromolecular materials (Tanasković et al. Citation2021), which are beneficial for digestion and absorption in the broiler gastrointestinal tract. However, enzyme production during microbial fermentation is lower and insufficient for degrading anti-nutritional factors. Mao et al. (Citation2019) reported that fermentation by probiotics supplemented with exogenous enzymes could resolve this problem, illustrated by increased efficiency of degrading anti-nutritional factors and improved quality of fermented materials. Furthermore, more secondary metabolites, such as polyphenols and flavonoids, are released after fermentation by probiotics supplemented with exogenous enzymes (Xie et al. Citation2020).
Thus, we hypothesised that CAL fermented by compound probiotics and pectinase might be a potential feed additive in poultry diets. To address this hypothesis, we assessed the effects of dietary fermented CAL (FCAL) supplementation on growth performance, apparent nutrient digestibility, serum immune responses, carcase characteristics and meat quality of broilers.
Materials and methods
Materials
Fresh CAL was collected from April to November in Hohhot (Inner Mongolia, Hohhot, China). The aerial parts of CAL were obtained and dried at 25 °C in the shade, then ground to a fine powder. B. subtilis (CGMCC 1.0892), Lactobacillus plantarum (CGMCC No. 1.12934) and Saccharomyces cerevisiae (CGMCC No. 2.1190) were purchased from the China General Microbiological Culture Collection Centre (Beijing, China). Pectinase was obtained commercially (Beijing Solarbio Technology Co., Ltd, Beijing, China). Maize meal and ground cinnamon were obtained from a local market.
Fermentation of CAL
The compound probiotics were prepared by mixing B. subtilis, L. plantarum and S. cerevisiae at a ratio of 1:1:1. The CAL was mixed with corn meal, ground cinnamon and pectinase at a ratio of 16.5:3.0:0.1:0.4 (4 kg in total), blended with 50% distilled water (w/v) and was inoculated with 0.1% compound probiotics. The fermentation was conducted at 30 °C for 24 h. Fermentation of CAL was conducted in multi-layer polythene bags (5 kg capacity) equipped with a gas pressure opening valve. After fermentation, the extract was dried at 45 °C and ground with a hammer mill. The obtained FCAL was stored at −20 °C until mixing in the diets. The polyphenol and polysaccharide contents of CAL before and after fermentation were 17.81–27.32 mg/g and 141.96–229.56 mg/g, respectively, in this study.
Animals, diet and experimental design
All experimental procedures of this study were approved by Inner Mongolia University Research Ethics Committee. A total of 160 1-d-old Arbour Acres broiler chicks (purchased from a local hatchery) were individually weighed (45.96 ± 3.55 g) and randomly divided into four groups with five replications of eight birds each, including the control (CON) and FCAL supplementation groups. The CON group was provided with a corn-soybean meal basal diet and the FCAL groups were provided with a basal diet supplemented with 2, 4 or 8 g/kg of FCAL, respectively. Two different basal diets were used: a starter ration from 0 to 21 d and a finisher ration from 22 to 42 d. All basal diets were formulated to meet the nutrient recommendations of the Feeding Standard of Chicken, China (NY/T 33-2004; Chinese Ministry of Agriculture, 2004). The ingredients and chemical composition of the basal diets are presented in Table . Diets were supplied in a powder form. Feed and fresh water were provided ad libitum throughout the experiment. The stocking density was 16 birds per m2 in the starter period and 9 birds per m2 in the finish period. The room temperature was maintained at 33–35 °C for the first week and then reduced by 2–3 °C each week until it reached 20 °C. The lighting schedule was 24 L (24 h of lighting and 0 h of dark per day) for the first 3 d, 23 L:1D for days 4–7 d, and 18 L:6D for days 8–42.
Table 1. Ingredient and chemical composition of basal diets (%, air-dry basis).
Growth performances
Body weight and feed intake were recorded weekly, and the average daily gain (ADG), average daily feed intake (ADFI) and feed conversion ratio (FCR) of the starter, finisher and whole periods were calculated.
Apparent nutrient digestibility
Faecal samples were taken from each replication once daily during the last 3 d of the two periods (19–21 d and 40–42 d, respectively) and pooled per replication. About 600 g of subsamples were taken, dried at 65 °C for 96 h and ground to a fine powder for apparent total tract digestibility analysis. Components of the diets and faecal samples, including dry matter (DM, method 930.15), crude protein (CP, method 976.06) and ether extract (EE, method 920.39) were measured according to the methods of the Association of the AOAC (Citation2000). Acid insoluble ash (AIA) was used as an internal marker and determined following the method described by Atkinson et al. (Citation1984). The apparent nutrient digestibility (%) equation was as follows:
Serum immune indices
At 21 and 42 d, one bird from each replicate was randomly selected. Blood samples (5–10 mL) were obtained from the jugular vein. The serum samples were collected by centrifugation at 3000 × g at 4 °C for 10 min and stored at −80 °C for further serum immune indices analysis. The serum concentrations of insulin-like growth factor-1 (IGF-1), cytokines (interleukin [IL]-1β, IL-8, tumour necrosis factor-α [TNF-α], IL-10 and interferon-γ [IFN-γ]) and antibodies (immunoglobulin [Ig] G [IgG], IgA and IgM) were analysed using commercial ELISA kits (Jiangsu Mei Biao Biological Technology Co., Ltd., Jiangsu, China) according to the manufacturer’s protocol.
Carcase characteristics
At 42 d, one bird per replicate was randomly taken. All birds were fasted for 12 h and weighed (live weight) and electrically stunned (110 V, 350 Hz). After exsanguination via severing the jugular vein and carotid artery on one side of the neck and defeathering via scalding in a 65–70 °C water 30–60 s, the carcase weight was measured. Eviscerated weight was determined after removing the head, feet, and organs except the lungs and kidneys in the carcase. During evisceration, abdominal fat pads were removed and weighed. Then, breast (including pectoralis major and pectoralis minor) and leg muscles (including thigh and drumstick) were analysed. The indices of carcase, eviscerated, breast muscle, leg muscle and abdominal fat percentages were calculated as follows:
Meat quality
The muscles from the breast and thighs were used to determine drip loss, water loss, cooking loss, shear force, pH at 45 min and 24 h after euthanasia (pH45 min and pH24 h, respectively) and meat colour. The drip loss was measured according to the methods of Honikel (Citation1998). Briefly, the muscle samples were trimmed to a 5 × 2 × 1 cm in size, weighed and then hung in plastic bottles at 4 °C. After 24 h, the excess moisture was wiped off and the samples were weighed again. The drip loss was calculated as the percentage of muscle weight change measured between 0 and 24 h. Water loss was determined using the filter paper press method as described by Farouk et al. (Citation2004). Samples were weighed before and after being subjected to a 35 kg force for 5 min using a pressure instrument (RH-1000, Guangzhou Runhu Instrument Co., Ltd, Guangzhou, China). Determination of cooking loss was made by recording muscle weight loss before and after cooking in an 85 °C water bath for 15 min (Moyo et al. Citation2020). After the cooking loss was determined, the samples were used to determine the shear force according to the methods of Moyo et al. (Citation2020). The cooked muscle samples were manually trimmed into long strips (3 cm length × 1 cm width × 1 cm thickness) along the direction parallel to the muscle fibre. The strips were sheared perpendicular to the muscle fibre using a texture analyser (C-LM3, Tenoco International Co., Ltd, Beijing, China) . The test speed was 5 mm/s. Each muscle sample was measured three times, and the average value was taken as the shear force of the samples. The pH values of muscle samples were measured at 45 min (pH45 min) and 24 h (pH24 h) after slaughtering via portable pH metre (Corning Glass Works, Medfield, MA) (Shang et al. Citation2014). The meat colour was evaluated by the measurement of L* (lightness), a* (redness) and b* (yellowness) colour values using a Minolta colour-guide (BYK-Gardener GmbH, Geretsried, Germany).
Statistical analysis
All data are expressed as the means and SEM. Data on growth performance and apparent nutrient digestibility were based on the entire broiler pen, whereas the other data were based on individual broilers. Data were analysed using the GLM procedure of SAS version 9.4 (SAS Institute Citation2000; Cary, NC). The model used was:
where Yij is the dependent variable, μ is the population mean, Di is the effect of FCAL supplementation level (0, 2, 4 or 8 g/kg) and Eij is the random error. The significance of the differences among the treatments was tested using Tukey’s multiple range test. Orthogonal polynomial contrasts were applied to determine the linear (L) and quadratic (Q) effects of increasing the level of FCAL. Differences were considered to be statistically significant when p < .05.
Results
Growth performance
During the starter period, there was a linear and quadratic influence of FCAL supplementation on ADG and FCR (p < .05). Compared with the CON group, broilers fed 4 and 8 g/kg FCAL had higher (p < .0001) ADG. The FCR of broilers in the 8 g/kg FCAL group was lower (p = .0014; Table ).
Table 2. Effects of fermented Chenopodium album L. (FCAL) supplementation on growth performance of broilers.
During the finisher period, there was a quadratic influence of FCAL inclusion on ADG (p = .0014) and FCR (p = .0222). Dietary supplementation of 2 and 4 g/kg FCAL significantly increased the ADG of broilers (p < .0001). Broilers fed 2 g/kg FCAL had the a lower (p < .0001) FCR than the CON group.
Throughout the study, there were linear (p = .0041) and quadratic (p = .0136) effects of FCAL supplementation on the ADG of broilers. Broilers fed 4 or 8 g/kg FCAL had higher (p = .0197) ADG than the CON group.
Apparent nutrient digestibility
During the starter period, there were linear (p < .0001) and quadratic (p < .0001) effects on apparent DM and CP digestibility and a linear (p = .0166) effect on apparent EE digestibility of broilers (Table ). Broilers fed the 4 or 8 g/kg FCAL had higher (p < .0001) apparent DM, CP and EE digestibility compared with the CON group. Supplementation with 2 g/kg FCAL significantly increased apparent EE digestibility of broilers (p < .0001).
Table 3. Effects of fermented Chenopodium album L. (FCAL) supplementation on apparent nutrient digestibility of broilers.
During the finisher period, there was a quadratic (p = .0002) effect of FCAL inclusion on apparent DM digestibility. Compared with the CON group, broilers fed FCAL had higher (p < .0001) apparent DM digestibility. The apparent DM, CP and EE digestibility of broilers fed 2 g/kg FCAL was higher (p < .0001).
Serum immune indices
As shown in Table , orthogonal polynomial contrast analysis revealed that the serum IGF-1 and IgM content responded in a dose-dependent linear and quadratic manner with FCAL supplementation at 21 d (p < .05). In response to the FCAL dose, the serum IL-1β, IL-8 and IL-10 levels quadratically decreased (p < .05) and the serum IgA level quadratically increased (p < .0068). Compared with the CON group, broilers fed 8 g/kg FCAL had an increased (p < .0318) serum IGF-1 level. Supplementation with 2 and 4 g/kg FCAL significantly decreased (p = .0004) the serum IL-8 concentration, and the 2 g/kg FCAL group had a lower (p < .05) serum IL-10 concentration. FCAL groups had a lower (p < .05) serum IL-1β concentration, while they had a higher (p = .0045 and p = .0007, respectively) serum IgA and IgM concentration.
Table 4. Effects of fermented Chenopodium album L. (FCAL) supplementation on serum immune indices of broilers (21 d).
As shown in Table , orthogonal polynomial contrast analysis revealed that the serum IGF-1 and IgA content increased in response to FCAL dose in a quadratic manner at 42 d (p < .05), while IL-10 content decreased in a dose-dependent quadratic manner (p = .0014). The FCAL groups had higher serum IGF-1 and IgA levels than the CON group (p = .0013 and p = .0025, respectively). Dietary supplementation with 2 or 4 g/kg FCAL significantly decreased (p = .0052) the serum IL-10 level.
Table 5. Effects of fermented Chenopodium album L. (FCAL) supplementation on serum immune indices of broilers (42 d).
Carcase characteristics
The percentage of breast muscle increased (p < .05) in a dose-dependent linear and quadratic manner with FCAL treatment (Table ). Broilers in the 8 g/kg FCAL group had a higher (p = .0047) percentage of breast muscle than the CON group. Dietary supplementation with FCAL had no effect (p > .05) on the carcase, eviscerated, leg muscle and abdominal fat percentages of broilers.
Table 6. Effects of fermented Chenopodium album L. (FCAL) supplementation on carcase characteristics of broilers.
Meat quality
As shown in Table , orthogonal polynomial contrast analysis revealed that the drip loss of the breast muscle decreased in response to FCAL dose in a linear and quadratic manner (p < .05). The water loss and shear force of the breast muscle also decreased in a dose-dependent linear manner (p < .05). Compared with the CON group, broilers in the 4 and 8 g/kg FCAL groups had decreased breast muscle drip loss (p < .001). The FCAL groups had a lower water loss rate and shear force of breast muscle (p < .05).
Table 7. Effect of fermented Chenopodium album L. (FCAL) supplementation on breast meat quality of broilers.
In response to dose, the water loss rate of leg muscle linearly and quadratically decreased (p < .05), and the L* of the leg muscle quadratically decreased (p = .00208) (Table ). The water loss rate and L* of the leg muscle were lower in the FCAL groups than in the CON group.
Table 8. Effect of fermented Chenopodium album L. (FCAL) supplementation on leg meat quality of broilers.
Discussion
The growth-promoting effects of HPs on broilers have been extensively reported (Wu et al. Citation2015; Niu et al. Citation2017). As a medicinal herbs, the growth-promoting effect of CAL has been reported in rats with mercury-induced oxidative stress (Jahan et al. Citation2019). Arora et al. (Citation2014) had demonstrated that oral gavage CAL acetone extract significantly prevented the body weight loss of arthritic rats. Similarly, Sikarwar et al. (Citation2017) also proved that feeding CAL aqueous extract to rats with kidney stones could decrease their weight loss. In this study, dietary FCAL supplementation significantly increased the ADG of broilers during the starter, finisher and whole periods. However, there have been no reports to the best of our knowledge on the effects of FCAL on broilers, thus, it is difficult to make any direct comparison. It is suggested that the growth-promoting effects of HPs might partly be attributed to bioactive components, such as polyphenols and polysaccharides (Gopi et al. Citation2020; Long et al. Citation2021). Oloruntola et al. (Citation2018, Citation2020) indicated that polyphenols, flavonoids and saponins extracted from medicinal herbs could scavenge free radicals and maintain intestinal epithelial barrier integrity. It is believed that the structural and functional homeostasis of the gastrointestinal tract is important for nutrient digestion and absorption as well as the growth performance of broilers (Cheled-Shoval et al. Citation2011; Wang et al. Citation2020). As reported in a previous study (Rahiminejad and Gornall Citation2004; Gao Citation2018; He et al. Citation2018), CAL contains abundant bioactive ingredients (polysaccharides, polyphenols, flavonoids and γ-aminobutyric acid). Furthermore, the polyphenols and polysaccharide levels of CAL were increased by fermentation with compound bacteria cooperating pectinase in this study. Thus, the performance-promoting effects of FCAL could be related to the improved nutrient digestibility, which was confirmed by the increased improved apparent digestibility of DM, CP and EE. Moreover, the increased serum IGF-1 level of broilers fed with FCAL was observed in this study. The positive relationship between growth performance and plasma IGF-1 content has been indicated in a previous study (Kareem et al. Citation2016).
The determination of nutrients digestibility is a quantitative assessment of digestion and absorption functions as well as health status of the gastrointestinal tract in broilers. Furthermore, it is regarded as an important evaluation index for feed suitability (Arlinghaus and Niesar Citation2005). In this study, supplementation with FCAL increased the apparent digestibility of DM, CP and EE of broilers. The beneficial effects of HPs on the nutrient digestibility of broilers have been established in many studies. The apparent CP digestibility of broilers was shown to increase with peppermint essential oil (Emami et al. Citation2012) and Nigella sativa seed (Rahman and Kim Citation2016). The improvement in nutrient digestibility may be partly explained by increased secretions of saliva and bile and enhanced enzyme activity, which are stimulated by polyphenols extracted from medicinal herbs (Lee et al. Citation2003). Additionally, it has been reported that the fermentation of agri-industrial by-products combined with exogenous enzymes could eliminate the antinutritional components (Xie et al. Citation2016; Jazi et al. Citation2017). As one of the common antinutritional components in the feedstuffs of plant origin (Kashani et al. Citation2020), pectin increases digesta viscosity and feed retention time in the gastrointestinal tract, and by this consequently impairing nutrient digestion (Saki et al. Citation2010). In this study, the polyphenols of CAL were released after fermentation with compound probiotics and pectinase. Although pectin content was not detected, we speculated that FCAL could enhance the nutrient digestibility of broilers by increased polyphenol levels and decomposed pectin.
Cytokines are regulators of host responses to infection, immune response and inflammation. Some cytokines (IL-1β, IL-8 and TNF-α) promote inflammation and are called proinflammatory cytokines, whereas others cytokines (IL-10 and IFN-γ) suppress the activity of proinflammatory cytokines and are called anti-inflammatory cytokines (Berger Citation2000). In this study, the levels of IL-1β and IL-8 of broilers were decreased by dietary FCAL supplementation. It is suggested that FCAL could reduce the likelihood of inflammation in broilers. Previous studies have pointed out the anti-inflammatory effects of CAL in rats with arthritis (Arora et al. Citation2014; Amodeo et al. Citation2019) and ethanol-induced gastric lesion rats (Kim and Jeong Citation2011). Similarly, Yao et al. (Citation2014) found that saponin extracted from Quinoa belonging to the family Chenopodiaceae could suppress the production of TNF-α and IL-6 in LPS-induced RAW264.7 macrophage cells. Furthermore, the extracts of CAL could inhibit NF-κB expression (Arora et al. Citation2014) and NO production (Amodeo et al. Citation2019), which consequently suppresses inflammation (Lee et al. Citation2009). In this study, the serum IL-10 content also decreased in the 2 and 4 g/kg FCAL groups. As a kind of anti-inflammatory cytokine, IL-10 plays a crucial role in suppressing pro-inflammatory processes in CD4 + T cells (Bedke et al. Citation2019). The lower serum IL-10 concentration of broilers in this study might be attributed to the low degradation of the inflammatory condition.
Igs produced by B cells are the key components of humoural immunity (Mast et al. Citation2000). The positive correlation between antibody contents (IgA, IgM and IgG), and immunity and disease resistance of animals has been well-demonstrated (Bi et al. Citation2020; Gong et al. Citation2020). In this study, dietary supplementation with FCAL promoted the humoural immune responses of broilers, which was confirmed by increased serum IgA and IgM concentrations at 21 d and serum IgA concentration at 42 d. Similar results were reported by Estrada et al (Citation1998), who found that saponins from Quinoa could enhance specific Igs (IgG and IgA), which responded to the antigens in the serum, and in the intestinal and lung secretions of mice. The saponins from Quinoa also remarkably increased the serum IgG content of the immunised mice (Verza et al. Citation2012). Furthermore, previous studies have shown that the bioactive substance in HPs could enhance humoural immune response of animals by stimulating B cell proliferation and Ig production (Han et al. Citation2003; Kong et al. Citation2007; Wu Citation2018; Long et al. Citation2020; Long et al. Citation2021).
In this study, the breast muscle percentage was significantly increased by 8 g/kg FCAL. The better carcase quality of the broilers might be due to improved nutrient deposition (Li et al. Citation2019). The positive effects of FCAL on ADFI, FCR and nutrient digestibility may be responsible for the increased breast meat content. In agreement with our results, previous studies have reported that supplementation with HPs, such as lycopene and fermented ginkgo biloba leaves, in the diet improves the growth and carcase yield of broilers (Sahin et al. Citation2006; Niu et al. Citation2017).
Drip, cooking, and water loss rates are important indicators to measure the water-holding capacity of breast and leg muscles (Cheng et al. Citation2019). A low water-holding capacity in muscles can increase the liquid outflow and lead to the loss of soluble nutrients and flavour (Xing et al. Citation2020). The results of this study showed that the addition of FCAL decreased the drip loss of the breast muscle and water loss rate of the breast and leg muscles, which was consistent with the results of Wang et al. (Citation2015), who reported that the drip loss of broiler breast and thigh meat was decreased by turmeric rhizome extract supplementation. The manner in which the supplementation of FCAL improved the water-holding capacity in the muscles of broilers might be related to the increase in polyphenols levels, which exhibited strong free radicals scavenging activity and reduced oxidative-induced conformational alterations and fragmentation of myofibrillar proteins (Patel et al. Citation2010). Moreover, shear force is an important variable that reflects the tenderness of meat products (Pascual Guzmán et al. Citation2021). The results of this study indicated that FCAL addition to the diet of the broilers decreased the shear force of the breast muscle, which is in accordance with the findings of Rahman and Kim (Citation2016), who reported that dietary supplementation with Nigella sativa seeds decreased the shear force of thigh muscle. It is suggested that the decreased shear force might be caused by the improved the water-holding capacity (Webb and Agbeniga Citation2020). The colour of meat affects the meat-purchasing decisions of consumers, thus is a useful criterion for meat quality assessments (Barbut S Citation1993). In this study, the inclusion of 2–8 g/kg of FCAL in the diet also decreased the value of L* in the leg muscle. The changes in the L* value can be attributed to the higher water-holding capacity, resulting in lower surface light reflectivity (Hughes et al. Citation2014). However, desirable changes in the pH value at 45 min and 24 h post-slaughter were not observed, and this requires further investigation.
Conclusions
In conclusion, dietary supplementation with a 2–8 g/kg FCAL basal diet significantly improved the growth performance, nutrient digestibility, immunity and meat quality of broilers. Furthermore, the dietary addition of 8 g/kg FCAL could significantly increase the breast muscle percentage. Overall, FCAL may be used as a reliable phytogenic feed additive to promote growth and maintain the health of broilers.
Ethics statement
All experimental procedures were conducted in conformity with institutional guidelines for the care and use of laboratory animals in China (The State Science and Technology Commission of China, 1988). Animal care, slaughter and sampling of broiler chickens used in this study passed through the process of Inner Mongolia University Animal Science Care and Use Committee.
Software and data repository resources
None of the data were deposited in an official repository.
Disclosure statement
No potential conflict of interest was reported by the authors.
Additional information
Funding
References
- Al-Kassie GAM. 2010. The role of peppermint (Mentha piperita) on performance in roiler diets. Agric Biol J N Am. 15:1009–1013.
- Amodeo V, Marrelli M, Pontieri V, Cassano R, Trombino S, Conforti F, Statti G. 2019. Chenopodium album L. and Sisymbrium officinale (L.) Scop.: phytochemical content and in vitro antioxidant and anti-Inflammatory potential. Plants. 8(11):505.
- AOAC. 2000. Official methods of analysis of AOAC international. 17th ed. Rockville (MD): AOAC International.
- Arlinghaus R, Niesar M. 2005. Nutrient digestibility of angling baits for carp, Cyprinus carpio, with implications for groundbait formulation and eutrophication control. Fisheries Manage. 12(2):91–97.
- Arora S, Itankar P. 2018. Extraction, isolation and identification of flavonoid from Chenopodium album aerial parts. J Tradit Complement Med. 8(4):476–482.
- Arora SK, Itankar PR, Verma PR, Bharne AP, Kokare DM. 2014. Involvement of NFκB in the antirheumatic potential of Chenopodium album L., aerial parts extracts. J Ethnopharmacol. 155(1):222–229.
- Ashour EA, Abd El-Hack ME, Swelum AA, Osman AO, Taha AE, Alhimaidi AR, Ismail IE. 2020. Does the dietary graded levels of herbal mixture powder impact growth, carcase traits, blood indices and meat quality of the broilers? Ital J Anim Sci. 19(1):1228–1237.
- Atkinson JL, Hilton JW, Slinger SJ. 1984. Evaluation of acid-insoluble ash as an indicator of feed digestibility in rainbow trout (Salmo gairdneri). Can J Fish Aquat Sci. 41(9):1384–1386.
- Barbut S. 1993. Colour measurements for evaluating the pale soft exudative (PSE) occurrence in turkey meat. Food Res Int. 26(1):39–43.
- Bedke T, Muscate F, Soukou S, Gagliani N, Huber S. 2019. IL-10-producing T cells and their dual functions. Semin Immunol. 44:101335–101343.
- Berger A. 2000. Th1 and Th2 responses: what are they? BMJ. 321(7258):424–424.
- Bi S, Zhang J, Qu Y, Zhou B, He X, Ni J. 2020. Yeast cell wall product enhanced intestinal IgA response and changed cecum microflora species after oral vaccination in chickens. Poult Sci. 99(12):6576–6585.
- Cheled-Shoval SL, Amit-Romach E, Barbakov M, Uni Z. 2011. The effect of in ovo administration of mannan oligosaccharide on small intestine development during the pre- and posthatch periods in chickens. Poult Sci. 90(10):2301–2310.
- Chen MJ, Jia SX. 2002. Chinese forage plants. Beijing, China: China Agriculture Press; p. 885–886.
- Chen SL. 2006. Chinese herbal medicine. Beijing, China: Military Medical Science Press.
- Cheng YF, Chen YP, Li J, Qu HM, Zhao YR, Wen C, Zhou YM. 2019. Dietary β-Sitosterol improves growth performance, meat quality, antioxidant status, and mitochondrial biogenesis of breast muscle in broilers. Animals. 9(3):71.
- Cutillo F, D’Abrosca B, Dellagreca M, Zarrelli A. 2004. Chenoalbicin, a novel cinnamic acid amide alkaloid from chenopodium album. Chem Biodivers. 1(10):1579–1583.
- De Lange CFM, Pluske J, Gong J, Nyachoti CM. 2010. Strategic use of feed ingredients and feed additives to stimulate gut health and development in young pigs. Livest. Sci. 134(1–3):124–134.
- Dongowski G, Ehwald R, Luck K, Stoof G. 1992. Composition of cell wall microcapsules manufactured from Chenopodium album cell walls. Phytochemistry. 31(9):3039–3042.
- Emami NK, Samie A, Rahmani HR, Ruiz-Feria CA. 2012. The effect of peppermint essential oil and fructooligosaccharides, as alternatives to virginiamycin, on growth performance, digestibility, gut morphology and immune response of male broilers. Anim Feed Sci Technol. 175(1–2):57–64.
- Estrada A, Li B, Laarveld B. 1998. Adjuvant action of Chenopodium quinoa saponins on the induction of antibody responses to intragastric and intranasal administered antigens in mice. Comp Immunol Microbiol Infect Dis. 21(3):225–236.
- Fairley RA, Williams SJ, Finnigan CE, Greene MA, Robinson AE, Gill J. 2012. The occurrence of hypocalcaemia in mid lactation dairy cattle after the consumption of large amounts of fat-hen (Chenopodium album). N Z Vet J. 60(4):261–262.
- Farouk MM, Wieliczko KJ, Merts I. 2004. Ultra-fast freezing and low storage temperatures are not necessary to maintain the functional properties of manufacturing beef. Meat Sci. 66(1):171–179.
- Gao RG. 2018. Study on the extraction process of γ-aminobutyric acid. Modern Salt Chem Ind. 45(01):24–26.
- Gong HZ, Wu M, Lang WY, Yang M, Wang JH, Wang YQ, Zhang Y, Zheng X. 2020. Effects of laying breeder hens dietary β-carotene, curcumin, allicin and sodium butyrate supplementation on the growth performance, immunity, and jejunum morphology of their offspring chicks. Poultr Sci. 99(1):151–162.
- Gopi M, Dutta N, Pattanaik AK, Jadhav SE, Madhupriya V, Tyagi PK, Mohan J. 2020. Effect of polyphenol extract on performance, serum biochemistry, skin pigmentation and carcass characteristics in broiler chickens fed with different cereal sources under hot-humid conditions. Saudi J Biol Sci. 27(10):2719–2726.
- Gupta S, Lakshmi AJ, Manjunath MN, Prakash J. 2005. Analysis of nutrient and antinutrient content of underutilized green leafy vegetables. Food Sci Technol. 38(4):339–345.
- Han SB, Yoon YD, Ahn HJ, Lee HS, Lee CW, Yoon WK, Park SK, Kim HM. 2003. Toll-like receptor-mediated activation of B cells and macrophages by polysaccharide isolated from cell culture of Acanthopanax senticosus. Int Immunopharmacol. 3(9):1301–1312.
- He NW, Qin JJ, Wang XJ. 2018. Optimization of ultrasonic assisted extraction technology of polysaccharide from Chenopodium album Linn and its antioxidant activity in vitro. Sci Technol Food Ind. 39(01):235–240 + 252.
- Hmani H, Daoud L, Jlidi M, Jalleli K, Ben Ali M, Hadj Brahim A, Bargui M, Dammak A, Ben Ali M. 2017. A Bacillus subtilis strain as probiotic in poultry: selection based on in vitro functional properties and enzymatic potentialities. J Ind Microbiol Biotechnol. 44(8):1157–1166.
- Honikel KO. 1998. Reference methods for the assessment of physical characteristics of meat. Meat Sci. 49(4):447–457.
- Hughes J, Oiseth S, Purslow P, Warner RD. 2014. A structural approach to understanding the interactions between colour, water-holding capacity and tenderness. Meat Sci. 98(3):520–532.
- Jabbar A, Zaman MA, Iqbal Z, Yaseen M, Shamim A. 2007. Anthelmintic activity of Chenopodium album (L.) and Caesalpinia crista (L.) against trichostrongylid nematodes of sheep. J Ethnopharmacol. 114(1):86–91.
- Jahan S, Azad T, Ayub A, Ullah A, Afsar T, Almajwal A, Razak S. 2019. Ameliorating potency of Chenopodium album linn. and vitamin c against mercuric chloride-induced oxidative stress in testes of Sprague Dawley rats. Environ Health Prev Med. 24(1):62.
- Jan R, Saxena DC, Singh S. 2017. Analyzing the effect of optimization conditions of germination on the antioxidant activity, total phenolics, and antinutritional factors of Chenopodium (Chenopodium album). Food Measure. 11(1):256–264.
- Jazi V, Boldaji F, Dastar B, Hashemi SR, Ashayerizadeh A. 2017. Effects of fermented cottonseed meal on the growth performance, gastrointestinal microflora population and small intestinal morphology in broiler chickens. Br Poult Sci. 58(4):402–408.
- Kareem KY, Loh TC, Foo HL, Akit H, Samsudin AA. 2016. Effects of dietary postbiotic and inulin on growth performance, IGF1 and GHR mRNA expression, faecal microbiota and volatile fatty acids in broilers. BMC Vet Res. 12(1):163.
- Kashani SA, Ali I, Hasni MS, Asrar M, Ahmad J, Shahzad MZ. 2020. Nutritional and Anti-Nutritional Study of Juniperus Excelsa (M. Bieb) of Ziarat, Balochistan, Pakistan. Environ Sci Ecol Curr Res. 1:0008.
- Kerovuo J, Lauraeus M, Nurminen P, Kalkkinen N, Apajalahti J. 1998. Isolation, characterization, molecular gene cloning, and sequencing of a novel phytase from Bacillus subtilis. Appl Environ Microbiol. 64(6):2079–2085.
- Kim P, Jeong CS. 2011. Effects of Chenopodium album linne on gastritis and gastric cancer cell growth. Biomol Ther. 19(4):487–492.
- Kong XF, Yin YL, Wu GY, Liu HJ, Yin FG, Li TJ, Huang RL, Ruan Z, Xiong H, Deng ZY, et al. 2007. Dietary supplementation with Acanthopanax senticosusextract modulates cellular and humoral immunity in weaned piglets. Asian Australas J Anim Sci. 20(9):1453–1461.
- Korcan SE, Aksoy O, Erdoğmuş SF, Ciğerci IH, Konuk M. 2013. Evaluation of antibacterial, antioxidant and DNA protective capacity of Chenopodium album's ethanolic leaf extract. Chemosphere. 90(2):374–379.
- Kuralkar P, Kuralkar SV. 2021. Role of herbal products in animal production-an updated review. J Ethnopharmacol. 278:114246.
- Lee KW, Everts H, Kappert HJ, Frehner M, Losa R, Beynen AC. 2003. Effects of dietary essential oil components on growth performance, digestive enzymes and lipid metabolism in female broiler chickens. Br Poult Sci. 44(3):450–457.
- Lee MY, Park BY, Kwon OK, Yuk JE, Oh SR, Kim HS, Lee HK, Ahn KS. 2009. Anti-inflammatory activity of (-)-aptosimon isolated from Daphne genkwa in RAW264.7 cells. Int Immunopharmacol. 9(7–8):878–885.
- Li ZM, Yu M, Cui YY, Rong T, Liu ZC, Ma XY, Wang G. 2019. Effects of citrus extracts on organ indices, slaughter performance, meat quality and muscle antioxidant indices of yellow-feather broilers. Chin J Anim Nutr. 31(8):3794–3800.
- Long LN, Kang BJ, Jiang Q, Chen JS. 2020. Effects of dietary Lycium barbarum polysaccharides on growth performance, digestive enzyme activities, antioxidant status, and immunity of broiler chickens. Poultr Sci. 99(2):744–751.
- Long LN, Zhang HH, Wang F, Yin YX, Yang LY, Chen JS. 2021. Research note: effects of polysaccharide-enriched Acanthopanax senticosus extract on growth performance, immune function, antioxidation, and ileal microbial populations in broiler chickens. Poultr Sci. 100(4):101028.
- Mao Y, Lu CB, Li GH, Zhao YY, Deng Y. 2019. Optimized fermentation process of soybean meal by bacteria with enzymes. Food Ferment Ind. 45(14):108–114.
- Mast J, Buyse J, Goddeeris BM. 2000. Dietary L-carnitine supplementation increases antigen-specific immunoglobulin G production in broiler chickens. Br J Nutr. 83(2):161–166.
- Mohebodini H, Jazi V, Ashayerizadeh A, Toghyani M, Tellez-Isaias G. 2021. Productive parameters, cecal microflora, nutrient digestibility, antioxidant status, and thigh muscle fatty acid profile in broiler chickens fed with eucalyptus globulus essential oil. Poultr Sci. 100(3):100922.
- Moyo S, Masika PJ, Muchenje V, Jaja IF. 2020. Effect of Imbrasia belina meal on growth performance, quality characteristics and sensory attributes of broiler chicken meat. Ital J Anim Sci. 19(1):1450–1461.
- Niu Y, Wan XL, Zhang XH, Zhao LG, He JT, Zhang JF, Zhang LL, Wang T. 2017. Effect of supplemental fermented ginkgo biloba leaves at different levels on growth performance, meat quality, and antioxidant status of breast and thigh muscles in broiler chickens. Poultr Sci. 96(4):869–877.
- Oloruntola OD, Agbede JO, Ayodele SO, Oloruntola DA. 2018. Neem, pawpaw, and bamboo leaf meal dietary supplementation in broiler chickens: effect on performance and health status. J Food Biochem. 43:e12723.
- Oloruntola OD, Ayodele SO, Adeyeye SA, Jimoh AO, Oloruntola DA, Omoniyi SI. 2020. Pawpaw leaf and seed meals composite mix dietary supplementation: effects on broiler chicken’s performance, caecum microflora, and blood analysis. Agroforest Syst. 94(2):555–564.
- Pachauri T, Lakhani A, Kumari KM. 2017. Nutritional and antinutritional characterization of Chenopodium album seeds: a neglected wild species. Int J Nutr Agric Res. 4:9–21.
- Pascual Guzmán A, Trocino A, Susta L, Barbut S. 2021. Comparing three textural measurements of chicken breast fillets affected by severe wooden breast and spaghetti meat. Ital J Anim Sci. 20(1):465–471.
- Patel A, Patel A, Patel A, Patel NM. 2010. Determination of polyphenols and free radical scavenging activity of Tephrosia purpurea linn leaves (Leguminosae). Pharmacognosy Res. 2(3):152–158.
- Platel K, Srinivasan K. 2004. Digestive stimulant action of spices: a myth or reality? Indian J Med Res. 119 (5):167–179.
- Rahiminejad MR, Gornall RJ. 2004. Flavonoid evidence for allopolyploidy in the Chenopodium album aggregate (Amaranthaceae). Plant Syst Evol. 246(1–2):77–87.
- Rahman M, Kim SJ. 2016. Effects of dietary Nigella sativa seed supplementation on broiler productive performance, oxidative status and qualitative characteristics of thighs meat. Ital J Anim Sci. 15 (2):241–247.
- Rashmi S. 2016. Medicinal plants diversity in Bhilai city District Durg, Chhattisqarh, India. Int J Pharm Life Sci. 7(3):4952–4966.
- Redoy MRA, Rahman MA, Atikuzzaman M, Shuvo AAS, Hossain E, Khan MJ, Al-Mamun M. 2021. Dose titration of plantain herb (Plantago lanceolata L.) supplementation on growth performance, serum antioxidants status, liver enzymatic activity and meat quality in broiler chickens. Ital J Anim Sci. 20 (1):1244–1255.
- Sahin K, Onderci M, Sahin N, Gursu MF, Khachik F, Kucuk O. 2006. Effects of lycopene supplementation on antioxidant status, oxidative stress, performance and carcass characteristics in heat-stressed Japanese quail. J Therm Biol. 31(4):307–312.
- Saki AA, Matin H, Tabatabai MM, Zamani P, Harsini RN. 2010. Microflora population, intestinal condition and performance of broilers in response to various rates of pectin and cellulose in the diet. Arch Geflugelkunde. 74(3):183–188.
- SAS Institute. 2000. SAS/STAT(R) Inc. User’s guide, version 9. Cary (NC): SAS Institute Inc.
- Shang HM, Song H, Jiang YY, Ding GD, Xing YL, Niu SL, Wu B, Wang LN. 2014. Influence of fermentation concentrate of Hericium caput-medusae (Bull.:Fr.) Pers. on performance, antioxidant status, and meat quality in broilers. Anim Feed Sci Technol. 198:166–175.
- Sikarwar I, Dey YN, Wanjari MM, Sharma A, Gaidhani SN, Jadhav AD. 2017. Chenopodium album Linn. leaves prevent ethylene glycol-induced urolithiasis in rats. J Ethnopharmacol. 195:275–282.
- Singh V, Shah KN, Rana D. 2015. Medicinal importance of unexploited vegetable under North Eastern regions of India. J Med Plants Stud. 3(3):33–36.
- Tanasković SJ, Šekuljica NŽ, Jovanović JR, Gazikalovic I, Grbavčić SŽ, Đorđević N, Sekulić MV, Hao J, Lukovic NO, Knežević-Jugović Z. 2021. Upgrading of valuable food component contents and anti-nutritional factors depletion by solid-state fermentation: a way to valorize wheat bran for nutrition. J Cereal Sci. 99:103159.
- Upadhaya S, Kim IH. 2017. Efficacy of phytogenic feed additive on performance, production and health status of monogastric animals - a review. Ann. Anim. Sci. 17 (4):929–948.
- Verza SG, Silveira F, Cibulski S, Kaiser S, Ferreira F, Gosmann G, Roehe PM, Ortega GG. 2012. Immunoadjuvant activity, toxicity assays, and determination by UPLC/Q-TOF-MS of triterpenic saponins from Chenopodium quinoa seeds. J Agric Food Chem. 60(12):3113–3118.
- Wang DF, Huang HF, Zhou LL, Wei L, Zhou HL, Hou GY, Liu J, Hu L. 2015. Effects of dietary supplementation with turmeric rhizome extract on growth performance, carcass characteristics, antioxidant capability, and meat quality of Wenchang broiler chickens. Ital J Anim Sci. 14(3):3870.
- Wang JG, Lin J, Wang J, Wu SG, Qi GH, Zhang HJ, Song ZG. 2020. Effects of in ovo feeding of N-acetyl-L-glutamate on early intestinal development and growth performance in broiler chickens. Poultr Sci. 99(7):3583–3593.
- Wang YY, Heng CN, Zhou XH, Cao GT, Jiang L, Wang JS, Li KX, Wang DC, Zhan X. 2021. Supplemental Bacillus subtilis DSM29784 and enzymes, alone or in combination, as alternatives for antibiotics to improve growth performance, digestive enzyme activity, anti-oxidative status, immune response, and the intestinal barrier of broiler chickens. Br J Nutr. 125(5):494–507.
- Webb EC, Agbeniga B. 2020. Timing and duration of low voltage electrical stimulation on selected meat quality characteristics of light and heavy cattle carcasses. Anim Prod Sci. 60(7):967–977.
- Wu QJ, Wang ZB, Wang GY, Li YX, Qi YX. 2015. Effects of feed supplemented with fermented pine needles (pinus ponderosa) on growth performance and antioxidant status in broilers. Poultr Sci. 94(6):1138–1144.
- Wu S. 2018. Effect of dietary Astragalus membranaceus polysaccharide on the growth performance and immunity of juvenile broilers. Poult Sci. 97(10):3489–3493.
- Xie FL, Chang J, Yin QQ, Wang P, Lyv ZQ, Zhang HY, Yang MF, Ning CS. 2020. Effects of compound probiotics and cellulase fermentation on nutritional quality and microstructure of Aremisia argyi. Chin J Anim Nutr. 32(04):1768–1777.
- Xie PL, Huang LX, Zhang CH, Zhang YL. 2016. Nutrient assessment of olive leaf residues processed by solid-state fermentation as an innovative feedstuff additive. J Appl Microbiol. 121(1):28–40.
- Xing T, Zhao X, Xu XL, Li JL, Zhang L, Gao F. 2020. Physiochemical properties, protein and metabolite profiles of muscle exudate of chicken meat affected by wooden breast myopathy. Food Chem. 316:126271.
- Yao Y, Yang XS, Shi ZX, Ren GX. 2014. Anti-inflammatory activity of saponins from quinoa (Chenopodium quinoa Willd.) seeds in lipopolysaccharide-stimulated RAW 264.7 macrophages cells. J Food Sci. 79(5):H1018–1023.
- Ye M, Wei CJ, Khalid A, Hu Q, Yang R, Dai BD, Cheng HW, Wang ZG. 2020. Effect of Bacillus velezensis to substitute in-feed antibiotics on the production, blood biochemistry and egg quality indices of laying hens. BMC Vet Res. 16(1):1–8.