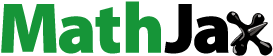
Abstract
This study aimed to investigate the effects of dietary supplementation with 5-aminolevulinic acid (5-ALA) on growth performance, blood parameters, iron status, nutrient digestibility, and antioxidant capacity in broilers. A total of 600 one-day-old Arbour Acres broilers were distributed into 5 groups with 6 replicates of 20 birds by a completely randomised design. The birds in the 5 groups were fed the basal diets with 0, 15, 30, 45, and 60 mg/kg 5-ALA, respectively. Dietary supplementation with 60 mg/kg 5-ALA significantly reduced the feed to gain ratio during days 1–21 and days 22–42. Moreover, compared with the control group, dietary supplementation with 30 mg/kg 5-ALA elevated haemoglobin concentrations during days 1–21. Furthermore, dietary supplementation with 45 and 60 mg/kg 5-ALA increased the digestibility of crude protein and decreased serum uric acid levels. At 21 days of age, dietary supplementation with 45 and 60 mg/kg 5-ALA increased liver catalase activity and decreased liver malondialdehyde concentrations, and upregulated the liver mRNA expression of ferritin light chain and nuclear factor erythroid 2-related 2. In addition, the liver mRNA expression of haem oxygenase-1 and divalent metal transporter were upregulated in the 60 mg/kg 5-ALA supplementation group, while the liver mRNA expression of Kelch-like ECH-associated protein 1 was decreased in the 5-ALA supplementation groups. In conclusion, dietary supplementation with 60 mg/kg 5-ALA improved the growth performance, nutrient digestibility, iron status and antioxidant capacity of 21-d-old broilers.
Addition of 60 mg/kg 5-ALA to broiler diets reduced the feed to gain ratio.
Addition of 45 and 60 mg/kg 5-ALA to broiler diets improved crude protein digestibility and decreased serum uric acid levels.
5-ALA exhibited antioxidant properties by enhancing the Nrf2 and HO-1 expression
Highlights
Introduction
In poultry production, broilers are always subjected to different degrees of oxidative stress including nutrition, disease, high stocking density, hot and humid environments and other factors. Oxidative stress could impair the health and growth performance of broilers and lead to serious economic losses. Previous studies showed that oxidative stress could be prevented by adding feed additives in broiler diets, such as vitamins, sulfur-containing amino acids, and plant extracts. (Jang et al. Citation2014; Jiang et al. Citation2016; Miao et al. Citation2021). Thus, it is of great interest to identify a novel and effective feed additive that could modulate the antioxidant system to protect broilers from oxidative stress. 5-Aminolevulinic acid (5-ALA) is an endogenous nonprotein amino acid that is involved in haem synthesis (Nishio et al. Citation2014). Haem has important physiological functions as a prosthetic group of haemoglobin (HGB), myoglobin, catalases (CAT), peroxidase, cytochrome c, cytochrome p450, etc. (Gozzelino et al. Citation2010; Ogura et al. Citation2011). 5-ALA has received widespread attention as a novel additive for livestock and poultry. Studies have reported that dietary supplementation with 10 or 15 mg/kg 5-ALA increases HGB levels, red blood cell (RBC) counts, and iron (Fe) status in broilers (Chen et al. Citation2008b; Wang et al. Citation2011a). In addition, dietary supplementation with 5-ALA enhanced immune responses and anti-inflammatory effects in broilers and Pacific white shrimp (Sato et al. Citation2012; Pedrosa-Gerasmio et al. Citation2019). However, very few studies exploring the antioxidant effects of 5-ALA in poultry, especially the antioxidant mechanism, have been conducted.
Nuclear factor erythroid 2-related factor 2 (Nrf2) is an important transcription factor and is demonstrated to be responsible for regulating antioxidant and cellular oxidative stress responses (Iranshahy et al. Citation2018). Normally, the inactive Nrf2-Kelch-like ECH-associated protein 1 (Keap-1) complex exists in the cytoplasm. Under oxidative stress or specific chemical reagent induction, Nrf2 dissociates from this complex to translocate into the nucleus, where it induces the expression of antioxidant response elements, such as haem oxygenase-1 (HO-1, Huang et al. Citation2021; Campbell et al. Citation2021). HO-1 has been shown to induce cytoprotective effects against oxidative injury and inflammatory insults in RAW 264.7 macrophages and zebrafish (Ji et al. Citation2021). HO-1 is involved in the degradation of haem and generates carbon monoxide, ferrous Fe, and bilirubin, which are also prominent endogenous antioxidant cytoprotectants (Sugiyama et al. Citation2018). Exogenous 5-ALA administration leads to the upregulation of HO-1 expression in the murine tubular epithelial cells (Liu et al. Citation2019; Ji et al. Citation2021). In addition, Zhao et al. (Citation2015) reported that 5-ALA ameliorated tissue injury and cardiomyocyte damage by activating the Nrf2/HO-1 signalling pathway. Based on this information, we hypothesised that dietary supplementation with 5-ALA would improve the antioxidant capacity of broilers by regulating the Nrf2/HO-1 signalling pathway. In this experiment, we investigated the effect of dietary supplementation with 5-ALA on the growth performance, nutrient digestibility, blood characteristics, iron status, and antioxidant capacity of broilers.
Materials and methods
Animals used in this experiment were approved by the Animal Ethics Committee of the Chinese Academy of Agricultural Sciences. All experimental operations complied with the guidelines for animal experiments set by the National Institute of Animal Health (Statement no. AEC-CAAS-20190120).
Animal management and experimental design
A total of 600 one-day-old Arbour Acres broilers were randomly assigned to five dietary treatments, with six replicates per group and 20 birds per replicate (half male and half female). The temperature was set at 32 °C for the first 3 days and then decreased by 2 °C each successive week until it reached 24 °C. The chicken house was kept under 24 h continuous light for the first 3 days, followed by 18 h continuous light and 6 h darkness from days 4 to 42. The birds were provided feed and water ad libitum and vaccinated according to the local vaccination schedule during the feeding trial.
Four different concentrations of 5-ALA (15, 30, 45, and 60 mg/kg; 5-ALA) were produced by adding 5-ALA to the control diet. The 5-ALA used in this study was provided by Challenge Corporation (Beijing, China). The control group was fed a basal diet (0 mg/kg). Diets were free from antibiotics and coccidiostat but were pelleted before feeding. All nutrients contained in the basal diet met or exceeded the feeding standard of China (NY/T 2004) for broilers. The ingredient and nutrient compositions of the basal diets are shown in Table .
Table 1. Ingredient composition and nutrient content of basal diets (as-fed basis).
Growth performance
After 8 hours of fasting, the body weight (BW) of broilers was measured for each replicate on the morning of d 21 and d 42. Feed intake was recorded on a cage basis. Subsequently, the average daily gain (ADG), average daily feed intake (ADFI), and feed to gain ratio (F/G) were calculated.
Apparent total tract digestibility
Broilers aged 28 to 35 d were fed diets mixed with titanium dioxide (0.4%) as an inert marker to determine the total tract nutrient digestibility coefficients of dry matter (DM), gross energy (GE) and crude protein (CP, Short et al. Citation1996). Fresh excreta samples from each replicate were collected daily for 3 days after a 5-day (d 28–32) adaptation period and then stored at −20 °C. Feathers, scales, and feed were removed from the excreta samples. Before chemical analysis, excreta samples were dried at 65 °C and then ground to a particle size of 0.45 mm. All feed and excreta samples were analysed for DM. The CP content was determined by the Kjeldahl method (KDY-9830, Ketuo, China). The GE content of the samples was determined in a bomb calorimeter (C2000, IKA, Guangzhou, China) using benzoic acid as the standard. The apparent nutrient digestibility coefficients were calculated using the following formula:
(1)
(1)
where NE = nutrient concentration in excreta (%), Nd = nutrient concentration in diet (%), Td = titanium dioxide concentration in diet (%), and TE = titanium dioxide concentration in excreta (%)
Determination of biochemical parameters
At 21 d and 42 d, two birds (one male and one female) with approximately average weight from each replicate group were euthanized by head-only electrical stunning, and then ten-millilitre blood samples were immediately obtained from the left jugular vein. Two-millilitre blood samples were stored in anticoagulant tubes for the analysis of RBCs and haemoglobin concentration. The remaining blood samples (8 mL) were centrifuged at 1500 xg for 10 min at 4 °C. The serum samples were kept at −20 °C until analysis.
RBC counts and HGB levels were analysed by a TEK-II mini automatic blood cell analyser (TEK5000P, Tecom Technologies Co., Jiangxi, China). The concentrations of serum total protein (TP), albumin (ALB), globulin (GLB), uric acid (UA), creatinine (CRE), aspartate aminotransferase (AST), alanine aminotransferase (ALT), and Fe; total Fe-binding capacity (TIBC); and total bilirubin (TBIL) were analysed by an automated system (7600 analyser, Hitachi High Technologies Co., Tokyo, Japan) or ELISA plate reader (Multiskan SkyHigh, Thermo Fisher Scientific, Massachusetts, USA) with standard commercial kits following the protocols recommended by the manufacturer (Nanjing Jiancheng Bioengineering Institute, Nanjing, China).
Liver tissue collection and antioxidants analysis
Liver samples from 12 birds per group (2 subsamples/replicate cage) were taken from the same lobe or side, washed with sterile saline solution to remove blood residue, immediately preserved in liquid nitrogen and then kept at −70 °C for mRNA and antioxidant capacity analysis. A 10% liver homogenate was prepared using phosphate buffer solution at a weight (g)-to-volume (mL) ratio of 1:9. The homogenate supernatant was obtained by centrifugation (3500 xg) for 10 min at 4 °C. The protein concentration of the homogenate was determined with the Bradford method.
The levels of total antioxidant capacity (T-AOC), malondialdehyde (MDA), superoxide dismutase (SOD), and CAT were measured in the homogenate supernatant of the live tissue using their respective commercial assay kits (T-AOC, A015-2-1; MDA, A003-1-2; SOD, A001-1-1; CAT, A007-1-1) purchased from Jiancheng Bioengineering Research Institute (Nanjing, China).
Real-time quantitative PCR analysis
Total RNA was extracted from liver tissue of broilers at 21 days of age, following the TRIzol method protocol. The quality and concentration of RNA samples were measured in a NanoDrop-2000 spectrophotometer (Thermo Fisher Scientific Co., Waltham, MA). The optical density at 260/280 nm was analysed, and RNA gel imaging detection was performed.
The RNA was reverse transcribed into cDNA with a transcription first strand cDNA Synthesis Kit (Tiangen, China) following the procedure recommended by the manufacturer. Primers were designed and checked for specificity using GenBank from the National Centre for Biotechnology Information. The primers were synthesised by Sangon Biotech Co. Ltd. (Shanghai, China). The primer sequences and accession numbers are listed in Table .
Table 2. Specific sequences primers were used for RT-PCR in this study.
The cDNA samples were amplified by real-time quantitative polymerase chain reaction with SuperReal PreMix (Probe) reagents (Tiangen, China). The RT–PCR conditions were as follows: 95 °C for 5 min, followed by 40 cycles of 95 °C for 10 s and 60 °C for 30 s, and a final extension at 72 °C for 5 min.
The specificity of qPCR products was evaluated by melting curve analysis. β-actin was utilised as an internal reference gene, broilers fed the basal diet were used as a calibrator in this study, and the relative expression of the target gene was calculated by the 2−ΔΔCT method (Livak and Schmittgen Citation2001).
Statistics analysis
All data were analysed by one-way analysis of variance (ANOVA) with the SPSS 19.0 software package for Windows (SPSS, Chicago, IL). The replicates were considered the experimental unit. Differences in means among treatments were separated by Tukey's multiple comparisons. P < .05 was used to judge statistical significance. The statistical model is as follows:
(2)
(2)
where Y = the dependent variables, μ = general mean, αi = fixed effect of treatment i, and eij = random error.
Results
Growth performance
As shown in Table , compared with the control group (0 mg/kg 5-ALA), dietary supplementation with 60 mg/kg 5-ALA significantly (P < .05) reduced the F/G at 1–21 and 22–42 d of age. In addition, dietary supplementation with 60 mg/kg 5-ALA increased (P < .05) the BW of 42-d-old broilers. There were no significant effects on ADG, ADFI or mortality with increasing levels of 5-ALA.
Table 3. Effects of dietary supplementation with 5-aminolevulinic acid on growth performance of broiler chickens.
Nutrient utilisation
Table shows the nutrient utilisation of DM, GE and CP measured during d 28 to 35. Compared with the control group, dietary supplementation with 45 and 60 mg/kg 5-ALA significantly (P < .01) increased the digestibility coefficient of CP. No significant differences were observed in DM and GE in response to 5-ALA supplementation in the diets.
Table 4. Effects of dietary supplementation with 5-aminolevulinic acid on nutrient utilisation in broiler chickens.
Haematological iron status
As shown in Table , at the age of 21 d, the HGB concentrations in the 30 mg/kg 5-ALA supplementation group was significantly higher than that of those in the control group; dietary supplementation with 5-ALA showed a tendency to improve serum TIBC concentrations (P = .097) and RBC counts (P = .089). However, 5-ALA supplementation had no effect on RBC counts, HGB, serum Fe, and serum TIBC concentrations at the age of 42 d.
Table 5. Effects of dietary supplementation with 5-aminolevulinic acid on the haematological iron status of the blood of broiler chickens.
Serum biochemical parameters
The serum biochemical characteristics are presented in Table . At the age of 21 d, a significantly (P < .05) lower UA level was observed in the groups fed 45 and 60 mg/kg 5-ALA than in the control; dietary 5-ALA supplementation had no significant effects on AST, ALT, CRE, TP, ALB, and TBIL.
Table 6. Effects of dietary supplementation with 5-aminolevulinic acid on serum biochemical parameters of 42-d-old broiler chickens.
Antioxidant indexes of the liver
As indicated in Table , compared with the control group, dietary supplementation with 60 mg/kg 5-ALA significantly increased (P < .05) T-AOC activities. CAT activity was improved (P < .05) in the 5-ALA-supplementation groups, while MDA concentrations and MDA/T-AOC ratios were reduced (P < .05) in the 45 and 60 mg/kg 5-ALA groups in 21-d-old broilers. There were no significant effects on T-AOC, CAT, SOD activity, and MDA concentrations in 42-d-old broilers, while dietary supplementation with 45 mg/kg 5-ALA significantly reduced (P < .05) the MDA/T-AOC ratio.
Table 7. Effects of dietary supplementation with 5-aminolevulinic acid on antioxidant capacity in the liver of broiler chickens.
Relative mRNA expression in liver
As shown in Figure , compared with the control diet, dietary supplementation with 5-ALA improved (P < .05) the cytochrome P450 family 1 (CYP1A1) mRNA expression levels; dietary supplementation with 30 and 45 mg/kg 5-ALA significantly increased (P < .05) the expression level of ferrochelatase (FECH) gene. The expression levels of the ferritin light chain (FTL) and divalent metal transporter (DMT) genes were upregulated (P < .05) when the diet was supplemented with 45 and 60 mg/kg 5-ALA. The gene expression of Nrf2 and HO-1 was analysed in the liver (Figure ). Dietary supplementation with 45 and 60 mg/kg 5-ALA significantly decreased (P < .05) the expression level of the Keap1 gene but upregulated the expression of the Nrf2 gene. In addition, dietary supplementation with 60 mg/kg 5-ALA significantly increased the expression level of the HO-1 gene.
Figure 1. The effects of 5-aminolevulinic acid (5-ALA) supplementation on the mRNA expression of substances related to Fe status, haem synthesis and metabolism in the liver of broilers. DMT, divalent metal transporter; FTL, ferritin light polypeptide; FECH, ferrochelatase; CYP1A1, cytochrome P450, family 1, subfamily A.
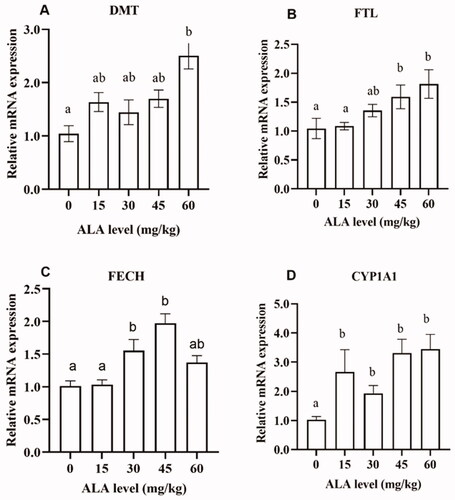
Discussion
Previous studies reported that dietary supplementation with 10 or 15 mg/kg had no significant effect on the growth performance of broilers (Chen et al. Citation2008b; Wang et al. Citation2011a). In our study, dietary supplementation with 15 mg/kg 5-ALA also had no significant effect on growth performance, but dietary supplementation with 60 mg/kg 5-ALA reduced the F/G at 1–21 (3.91%) and 1–42 d (2.86%). Similarly, there was a beneficial effect on BW, ADG, and F/G when weanling pigs were fed a high (1000/kg) level of 5-ALA (Hossain et al. Citation2016), while dietary supplementation with 5, 10, 15 or 50 mg/kg 5-ALA had no significant effect on the growth performance of weanling pigs (Mateo et al. Citation2005; Chen et al. Citation2008a). The above results indicated that dietary supplementation with 5-ALA had a dose-response effect on growth performance, and proper dosage is beneficial to improve growth performance. In addition, dietary supplementation with 60 mg/kg 5-ALA increased the digestibility of CP (2.34%) at 28–35 d, which can be used to explain the positive effect on the growth performance of broilers. Hossain et al. (Citation2016) also demonstrated that weanling pigs fed diets supplemented with 5-ALA (500 or 1000 mg/kg) tended to have increased DM and CP digestibility. However, why CP digestibility was improved by supplementation with 5-ALA could not be clearly elucidated. 5-ALA participates in oxygen metabolism and energy generation via the intermediate product haem, which might explain why CP digestibility was improved by supplementation with 5-ALA.
RBC count and HGB concentration are commonly used to assess iron status because iron concentration is associated with the HGB concentration (Southern and Baker Citation1982). Previous studies have indicated that dietary supplementation with exogenous 5-ALA increased the RBC count, HGB concentration, and TIBC of laying hens and weaned pigs (Yan and Kim Citation2011; Wang et al. Citation2011b). In the current study, dietary supplementation with 30 mg/kg 5-ALA improved HGB concentration at 21 days of age. Similarly, Chen et al. (Citation2008b) reported that the HGB concentration tended to increase with increasing 5-ALA (5, 10, and 15 mg/kg) supplementation in broiler diets. However, a positive correlation did not exist between the HGB concentration and the dosage of 5-ALA in the present study. This may be ascribed to the feedback mechanism of haem synthesis. Haem is essential for the formation of haemoglobin. In turn, haem synthesis is regulated by haem oxidase, ferrochelatase, and 5-ALA synthase, which are reaction limiting enzymes during the formation of HGB (Fujino et al. Citation2016). As expected, dietary supplementation with 45 and 60 mg/kg 5-ALA upregulated the liver mRNA expression of light chain ferritin (FTL), and supplementation with 60 mg/kg 5-ALA upregulated the liver mRNA expression of divalent metal transporters (DMT). Similarly, the serum TIBC tended to increase with 5-ALA supplementation on d 21. Divalent metal transporters (DMT) play a very important role in maintaining Fe homeostasis by mediating Fe absorption and transport in the small intestine (Hentze et al. Citation2010). Ferritin and TIBC are important indicators for Fe storage (Harrison and Arosio Citation1996). These results suggested that 5-ALA, especially at 45 and 60 mg/kg, is beneficial to Fe utilisation of 21-d-old broilers. However, the current results showed that dietary supplementation with 5-ALA had no significant effect on Fe status or haematological profiles of 42-day-old broilers. This discrepancy may be attributed to Fe overload in the grower phase. In the current study, the premix provided 100 mg Fe per kg of basal diets during both feeding phases, while tissue Fe deposition decreases as broilers age. Cao et al. (Citation1996) showed that the Fe deposition in the liver, kidney, spleen and bones of broilers at the third week was less than that in the second week. Chen et al. (Citation2008b) also suggested that 5-ALA supplementation had no significant effect on Fe status when chickens were subjected to Fe overload situations, which is in agreement with the results of the current study.
UA is a metabolite of proteins and nucleic acids. Therefore, serum UA or excreta UA should be viable response variables to determine the efficiency of nitrogen utilisation in broilers (Donsbough et al. Citation2010). In our study, the concentration of UA was significantly lower in the 45 and 60 mg/kg 5-ALA groups than in the control group, which is consistent with the results that dietary supplementation with 60 mg/kg 5-ALA improved protein utilisation in broilers. Under normal physiological conditions in animals, serum ALT and AST are maintained at low levels, but dramatic increases in enzyme activity or levels occur in response to tissue injury and inflammation. In our study, dietary 5-ALA supplementation tended to reduce AST and ALT levels. These results indicate that supplementation with 5-ALA is beneficial for improving liver function, which may be related to the anti-inflammatory effect of 5-ALA (Sugiyama et al. Citation2018). In addition, dietary supplementation with 5-ALA contributed to the upregulation of the expression of CYP1A1, which is one of the cytochrome P450 superfamilies. Cytochrome P450 is generally recognised to have the functions of detoxification, cellular metabolism and homeostasis (Manikandan and Nagini Citation2018). Accordingly, this may be another reason that 5-ALA is beneficial for improving liver function.
Cellular redox homeostasis is mainly maintained by an elaborate endogenous antioxidant defence system, which includes endogenous antioxidant enzymes such as SOD, CAT, FTL, and transferrin (Poljsak et al. Citation2013). In our study, dietary supplementation with 45 and 60 mg/kg 5-ALA improved T-AOC and CAT activities of broilers at 21 d. Meanwhile, dietary supplementation with 45 and 60 mg/kg 5-ALA reduced the MDA content and MDA/T-AOC ratio (an indication of antioxidant balance). This decrease reflected the significant decrease in the antioxidant (T-AOC) for use in the protection of cells from free radicals (Attia et al. Citation2020). In addition, our results showed that dietary supplementation with 45 and 60 mg/kg 5-ALA upregulated the mRNA expression levels of Nrf2 and downregulated the expression of the Keap1 gene. Nrf2 and Keap1 mediate the downstream antioxidant enzyme genes and are considered the most important cellular antioxidant pathways (Nguyen et al. Citation2009; Balogh et al. Citation2021). Among the target genes, HO-1 has a cytoprotective effect against oxidative injury and other cellular stresses (Waza et al. Citation2018; Ishfaq et al. Citation2019). Studies have reported that 5-ALA can regulate redox homeostasis by inducing the upregulation of HO-1 expression. Sugiyama et al. (Citation2018) reported that exogenous addition of 5-ALA induces HO-1 expression and leads to the downregulation of the expression of NO and some proinflammatory cytokines in raw macrophages. Pedrosa-Gerasmio et al. reported that 5-ALA upregulated HO-1 gene expression and protected shrimp against pathogens and environmental stress (Pedrosa-Gerasmio et al. Citation2019). In this study, dietary supplementation with 60 mg/kg 5-ALA upregulated the mRNA expression of HO-1. Our results are consistent with the results of these studies. The results indicate that dietary supplementation with 60 mg/kg 5-ALA enhances antioxidative status by upregulating the Nrf2/HO-1 signalling pathway
Conclusion
In conclusion, 5-ALA supplementation, especially at the level of 60 mg/kg, improved the growth performance, CP digestibility, Fe status and liver function of broiler chickens. Moreover, 5-ALA exerted its antioxidant effect by enhancing the Nrf2/HO-1 signalling pathway at the transcriptional level.
Acknowledgments
The research was supported by the China Agriculture Research System of MOF and MARA (CARS-41). We also thank Challenge Corporation (Beijing, China) for providing 5-aminolevulinic acid for this trial.
Disclosure statement
We declare that no competing interests exist.
References
- Attia YA, Al-Harthi MA, Abo El-Maaty HM. 2020. The effects of different oil sources on performance, digestive enzymes, carcass traits, biochemical, immunological, antioxidant, and morphometric responses of broiler chicks. Front Vet Sci. 7:181.
- Balogh E, Chowdhury A, Ababneh H, Csiki DM, Tóth A, Jeney V. 2021. Heme-mediated activation of the Nrf2/HO-1 axis attenuates calcification of valve interstitial cells. Biomedicines. 9(4):427.
- Campbell NK, Fitzgerald HK, Dunne A. 2021. Regulation of inflammation by the antioxidant haem oxygenase 1. Nat Rev Immunol. 21(7):411–425.
- Cao J, Luo XG, Henry PR, Ammerman CB, Littell RC, Miles RD. 1996. Effect of dietary iron concentration, age, and length of iron feeding on feed intake and tissue iron concentration of broiler chicks for use as a bioassay of supplemental iron sources. Poult Sci. 75(4):495–504.
- Chen YJ, Kim IH, Cho JH, Min BJ, Yoo JS, Wang Q. 2008a. Effect of δ-aminolevulinic acid on growth performance, nutrient digestibility, blood parameters and the immune response of weanling pigs challenged with Escherichia coli lipopolysaccharide. Livest Sci. 114(1):108–116.
- Chen YJ, KIm IH, Cho JH, Yoo JS, Kim HJ, Shin SO. 2008b. Utilization of δ-aminolevulinic acid for livestock: blood characteristics and immune organ weight in broilers. J Anim Feed Sci. 17(2):215–223.
- Donsbough AL, Powell S, Waguespack A, Bidner TD, Southern LL. 2010. Uric acid, urea, and ammonia concentrations in serum and uric acid concentration in excreta as indicators of amino acid utilization in diets for broilers. Poult Sci. 89(2):287–294.
- Fujino M, Nishio Y, Ito H, Tanaka T, Li X. 2016. 5-Aminolevulinic acid regulates the inflammatory response and alloimmune reaction. Int Immunopharmacol. 37:71–78.
- Gozzelino R, Jeney V, Soares MP. 2010. Mechanisms of cell protection by heme oxygenase-1. Annu Rev Pharmacol. 50:323–354.
- Harrison PM, Arosio P. 1996. The ferritins: molecular properties, iron storage function and cellular regulation. Biochim Biophys Acta. 1275(3):161–203.
- Hentze MW, Muckenthaler MU, Galy B, Camaschella C. 2010. Two to tango: regulation of Mammalian iron metabolism. Cell. 142(1):24–38.
- Hossain MM, Park JW, Kim IH. 2016. δ-Aminolevulinic acid, and lactulose supplements in weaned piglets diet: effects on performance, fecal microbiota, and in-vitro noxious gas emissions. Livest Sci. 183:84–91.
- Huang C, Fan Z, Han D, Johnston LJ, Ma X, Wang F. 2021. Pyrroloquinoline quinone regulates the redox status in vitro and in vivo of weaned pigs via the Nrf2/HO-1 pathway. J Anim Sci Biotech. 12(1):77.
- Iranshahy M, Iranshahi M, Abtahi SR, Karimi G. 2018. The role of nuclear factor erythroid 2-related factor 2 in hepatoprotective activity of natural products: a review. Food Chem Toxicol. 120:261–276.
- Ishfaq M, Chen C, Bao J, Zhang W, Wu Z, Wang J, Liu Y, Tian E, Hamid S, Li R, et al. 2019. Baicalin ameliorates oxidative stress and apoptosis by restoring mitochondrial dynamics in the spleen of chickens via the opposite modulation of NF-κB and Nrf2/HO-1 signaling pathway during mycoplasma gallisepticum infection. Poult Sci. 98(12):6296–6310.
- Jang I, Ko Y, Moon Y, Sohn S. 2014. Effects of vitamin c or e on the pro-inflammatory cytokines, heat shock protein 70 and antioxidant status in broiler chicks under summer conditions. Asian Australas J Anim Sci. 27(5):749–756.
- Ji SY, Cha HJ, Molagoda I, Kim MY, Kim SY, Hwangbo H, Lee H, Kim GY, Kim DH, Hyun JW, et al. 2021. Suppression of lipopolysaccharide-induced inflammatory and oxidative response by 5-aminolevulinic acid in RAW 264.7 macrophages and zebrafish larvae. Biomol Ther. 29(6):685–696.
- Jiang XR, Zhang HJ, Wang J, Wu SG, Yue HY, Lü HY, Cui H, Bontempo V, Qi GH. 2016. Effect of dried tangerine peel extract supplementation on the growth performance and antioxidant status of broiler chicks. Ital J Anim Sci. 15(4):642–648.
- Liu C, Fujino M, Zhu S, Isaka Y, Ito H, Takahashi K, Nakajima M, Tanaka T, Zhu P, Li XK. 2019. 5-ALA/SFC enhances HO-1 expression through the MAPK/Nrf2 antioxidant pathway and attenuates murine tubular epithelial cell apoptosis. Febs Open Bio. 9(11):1928–1938.
- Livak KJ, Schmittgen TD. 2001. Analysis of relative gene expression data using real-time quantitative PCR and the 2(-Delta Delta C(T)) Method. Methods. 25(4):402–408.
- Manikandan P, Nagini S. 2018. Cytochrome P450 structure, function and clinical significance: a review. Current Drug Targets. 19(1):38–54.
- Mateo RD, Morrow JL, Dailey JW, Ji F, Kim SW. 2005. Use of delta-aminolevulinic acid in swine diet: effect on growth performance, behavioral characteristics and hematological/immune status in nursery pigs. Asian Australas J Anim Sci. 19(1):97–101.
- Miao ZQ, Dong YY, Qin X, Yuan JM, Han MM, Zhang KK, Shi SR, Song XY, Zhang JZ, Li JH. 2021. Dietary supplementation of methionine mitigates oxidative stress in broilers under high stocking density. Poult Sci. 100(8):101231.
- Nguyen T, Nioi P, Pickett CB. 2009. the Nrf2-antioxidant response element signaling pathway and its activation by oxidative stress. J Biol Chem. 284(20):13291–13295.
- Nishio Y, Fujino M, Zhao M, Ishii T, Ishizuka M, Ito H, Takahashi K, Abe F, Nakajima M, Tanaka T, et al. 2014. 5-Aminolevulinic acid combined with ferrous iron enhances the expression of heme oxygenase-1. Int Immunopharmacol. 19(2):300–307.
- Ogura S-i, Maruyama K, Hagiya Y, Sugiyama Y, Tsuchiya K, Takahashi K, Abe F, Tabata K, Okura I, Nakajima M, et al. 2011. The effect of 5-aminolevulinic acid on cytochrome c oxidase activity in mouse liver. BMC Res Notes. 4(1):66.
- Pedrosa-Gerasmio IR, Kondo H, Hirono I. 2019. Dietary 5-aminolevulinic acid enhances adenosine triphosphate production, ecdysis and immune response in pacific white shrimp, Litopenaeus vannamei (Boone). Aquac Res. 50(4):1131–1141.
- Poljsak B, Šuput D, Milisav I. 2013. Achieving the balance between ros and antioxidants: when to use the synthetic antioxidants. Oxid Med Cell Longev. 2013:1–11.
- Sato K, Matsushita K, Takahashi K, Aoki M, Fuziwara J, Miyanari S, Kamada T. 2012. Dietary supplementation with 5-aminolevulinic acid modulates growth performance and inflammatory responses in broiler chickens. Poult Sci. 91(7):1582–1589.
- Short FJ, Gorton P, Wiseman J, Boorman KN. 1996. Determination of titanium dioxide added as an inert marker in chicken digestibility studies. J Anim Feed Sci. 59(4):215–221.
- Southern LL, Baker DH. 1982. Iron status of the chick as affected by eimeria acervulina infection and by variable iron ingestion. J Nutr. 112(12):2353–2362.
- Sugiyama Y, Hiraiwa Y, Hagiya Y, Nakajima M, Tanaka T, Ogura S. 2018. 5-Aminolevulinic acid regulates the immune response in LPS-stimulated RAW 264.7 macrophages. BMC Immunol. 19(1):41.
- Wang JP, Lee JH, Jang HD, Yan L, Cho JH, Kim IH. 2011b. Effects of δ-aminolevulinic acid and vitamin C supplementation on iron status, production performance, blood characteristics and egg quality of laying hens. J Anim Physiol Anim Nutr. 95(4):417–423.
- Wang JP, Yan L, Lee JH, Zhou TX, Kim IH. 2011a. Effects of dietary delta-aminolevulinic acid and vitamin C on growth performance, immune organ weight and ferrum status in broiler chicks. Livest Sci. 135(2–3):148–152.
- Waza AA, Hamid Z, Ali S, Bhat SA, Bhat MA. 2018. A review on heme oxygenase-1 induction: is it a necessary evil. Inflamm Res. 67(7):579–588.
- Yan L, Kim IH. 2011. Evaluation of dietary supplementation of delta-aminolevulinic acid and chitooligosaccharide on growth performance, nutrient digestibility, blood characteristics, and fecal microbial shedding in weaned pigs. Anim Feed Sci Tech. 169(3–4):275–280.
- Zhao M, Guo H, Chen J, Fujino M, Ito H, Takahashi K, Abe F, Nakajima M, Tanaka T, Wang J. 2015. 5-Aminolevulinic acid combined with sodium ferrous citrate ameliorates H2O2-induced cardiomyocyte hypertrophy via activation of the MAPK/Nrf2/HO-1 pathway. Am J Physiol-Cell Ph. 308(8):C665–C672.