Abstract
The objective of this study was to evaluate the effects of dietary naringin supplementation on rumen fermentation, inflammatory response and bacterial communities in fattening goats fed high-concentrate diet. Twenty four Boer male goats were randomly divided into three groups and assigned to receive dietary treatments. The three dietary treatments were a normal diet, a high-concentrate diet and a high-concentrate diet supplemented with 500 mg of naringin/kg of dry matter intake. Rumen fluid and plasma samples were collected at the end of the animal trial. Rumen fluid were analysed for pH, short-chain fatty acids, endotoxin (lipopolysaccharide, LPS) and microbiota. Illumina Miseq sequencing of the 16S rRNA gene was applied to investigate ruminal bacterial communities. The concentrations of interleukin −6 and tumour necrosis factor alpha as well as LPS-binding protein, haptoglobin, serum amyloid A and the activities of antioxdant enzymes in plasma were analysed. The result showed that feeding the high concentrate diet shifted the ruminal fermentation pattern from acetate towards propionate and valerate, and improved growth performance compared with the control group. However, the high concentrate diet promoted ruminal pH reduction and LPS release, modified bacterial communities and increased systemic inflammatory response. Naringin supplementation increased molar proportions butyrate and decreased valerate. Naringin supplementation decreased inflammatory response and increased the activities of antioxdant enzymes in plasma. Moreover, naringin addition increased the relative abundance of Bacteroidetes at the phylum level and Rikenellaceae_RC9_gut_group at the genus level. Taken together, these results suggest that dietary supplementation with naringin attenuates high grain diet-induced inflammatory response and modifies ruminal fermentation.
Dietary supplementation of naringin decreased inflammatory response and increased the activities of antioxdant enzymes in fattening goats.
Naringin supplementation changed ruminal microbiota of fattening goats.
Naringin has potential for use as feed additive to modify ruminal fermentation in a high-grain intensive ruminant production.
HIGHLIGHTS
Introduction
In current intensive goat production, high-concentrate diet feeding has become common practice to meet the energy demand for rapid-growing meat goats. High-concentrate diet is rich in carbohydrate that can lead to rapid ruminal production of organic acids like short-chain fatty acids which are the major energy sources for the ruminant host. However, the rapid accumulation of SCFAs reduced buffering capacity and ruminal pH, which may result in subacute ruminal acidosis (McCann et al. Citation2016). In the meantime, the bacterial community structure and diversity in the rumen were altered (Mao et al. Citation2016). The lower ruminal pH may enhance the lysis of Gram-negative bacteria which is accompanied with increasing ruminal-free lipopolysaccharide (Plaizier et al. Citation2014). Consequently, the rumen epithelial barrier failed in a low-pH rumen condition, LPS may be translocated from the gastrointestinal tract into the blood circulation and can stimulate the release of pro-inflammatory cytokines (Plaizier et al. Citation2014, 2018), and induce acute phase protein production (Gabay and Kushner Citation1999).
Several dietary strategies, such as sodium bicarbonate buffer (Mao et al. Citation2017), dicarboxylic acids (Vyas et al. Citation2015), monensin (Mutsvangwa et al. Citation2002) and probiotics (Henning et al. Citation2010), have been used to prevent the occurrence of SARA. However, antibiotic usage in food animals has been absolutely banned by the European Union and strictly restricted in other countries due to public concerns about food safety and antibiotic resistance. Removal of antibiotics and to explore some natural substances (Rhodes Citation1996) and secondary plant compounds such as flavonoids have been proposed as an alternative to antibiotic therapies.
Naringin, a bioflavonoid, is an abundant and inexpensive by-product of citrus cultivation. It is a naturally occurring polyphenolic compound widely distributed in the plant kingdom as a secondary metabolite. It has been reported that naringin has many biological activities, such as cholesterol-lowering (Cho et al. Citation2011), anticancer (Aroui et al. Citation2016), anti-inflammatory (Nie et al. Citation2012) and antioxidant (Jeon et al. Citation2001). Furthermore, several studies have shown that flavonoid can suppress the Escherichia coli and Staphylococcus aureus (Tripoli et al. Citation2007) and adjust the microbiota composition and ratio of intestine to improve the intestinal microbiota balance (Hara et al. Citation1995). In addition, dietary naringin supplementation can inhibit inflammatory immune response and improve animal disease resistance (Goliomytis et al. Citation2019). In spite of the excellent aforementioned biological functions, information is extremely scarce regarding dietary naringin supplementation on inflammatory response, antioxidant capacity and ruminal bacterial community in ruminants fed high-concentrate diets. Therefore, this study was undertaken to evaluate the effects of naringin supplementation on the ruminal fermentation, antioxidant status, immune response and ruminal microbial community of goats under high-concentrate diet.
Materials and methods
Animal care
This study was performed in accordance with the approved regulations for the administration of affairs concerning experimental animals. The experimental procedures were approved by the animal welfare and ethics committee of Yangzhou University (YZUDWLL-202001-003).
Animals, diets and experimental design
Twenty four four-month-old Boer male goats with an initial body weight of 29 ± 1.5 kg and similar body condition were used in this study, and all the animals were fed the same diet before the start of the study. The goats were randomly assigned to receive one of three diets: (1) control diet (CON; n = 8; concentrate: forage 35: 65), (2) high-concentrate diet (HC; n = 8; concentrate: forage 65: 35) and (3) high-concentrate diet supplemented with 500 mg of naringin/kg of dry matter intake (THC; n = 8; concentrate: forage 65: 35). The naringin (purity ≥ 95%; Shanghai Macklin Biochemical Technology Co., LTD, Shanghai, China) dose was chosen according to prior dose–response trials in lambs (Bodas et al. Citation2012; Alhidary and Abdelrahman Citation2016) and beef (Seradj et al. Citation2018). Goats were housed in individual pens (1.3 × 1.3 m) and offered a TMR fed twice daily at 0700 and 1700 h, with free access to diet and water. The ingredients and chemical composition of the diet are shown in Table . The animal feeding trial lasted for seven weeks. Daily orts were collected for feed intake measurement.
Table 1. Composition and nutrient levels of diets (Dry matter basis).
Sample collection
Rumen fluid samples were collected 3 h after the morning feeding on day 42 by an oesophageal tube equipped with a strainer and a syringe, as described by Zhou et al. (Citation2014) and Paz et al. (Citation2016). To avoid contamination by saliva, the initial rumen fluid collected was discarded. Next, about 50 mL rumen fluid was collected. About 10 mL of the collected rumen fluid of each goat was transferred into sterile tubes and stored in the liquid nitrogen for later microbial analysis. The rest of the rumen fluid (about 40 mL) was squeezed through four layers of cheesecloth to obtain filtrate, and a portion of strained rumen fluid was immediately used to determine pH values by using a mobile pH metre (FE-20, Mettler Toledo Co., Ltd, Shanghai, China). Another portion of the filtrate was transferred into sterile tubes for SCFA analyses. The last portion of the filtrate was transferred into sterile and pyrogen-free centrifuge tubes for LPS analysis. All filtrate samples were stored at −20 °C. Blood samples from the jugular vein were collected before morning feeding on day 35. Approximately 10 mL of blood was collected into a vacuum tube with sodium heparin, centrifuged at 4 °C for 15 min at 3000 × g, plasma was transferred into 2 mL LPS-free glass tubes. Another 5 mL blood was collected into a vacuum tube without additive, clotted at room temperature for 30 min and then centrifuged at 3000 × g for 15 min to obtain serum. Both plasma and serum were stored at −20 °C for future analyses.
The BW of each goat was measured for two consecutive days at the start and the end of the trial. Dry matter intake was recorded daily for each goat. Data were subsequently used for calculation of average daily gain, average daily DM intake and feed conversion ratio.
The rumen fluid samples were analysed for SCFAs using gas chromatograph (GC-14B, Shimadzu, Japan) with CP-WAX 52 CB capillary columns (30 m × 1.00 μm × 0.53 mm, Agilent) as described in our previous study (Sun et al. Citation2020). Briefly, rumen fluid was thawed (4 °C), centrifuged at 12,000 × g and 4 °C for 10 min, the supernatant was aspirated and mixed with metaphosphoric acid (25%) in a ratio of 5:1, then centrifuged at 12,000 × g and 4 °C for 10 min. The supernatant passed through a 0.45-μm filter and were transferred into a capillary column GC. The aliquots were run at a programmed temperature gradient (100 °C initial temperature, with a 3 °C rise per min until 150 °C).The gas used consisted of O2 (0.4 Mpa), H2 (0.3 Mpa) and N2 (0.7 Mpa). The column flow rate was 1 mL per minute. The temperature was 200 °C for the injector and 200 °C for the detector.
The LPS concentrations of rumen fluid and plasma were detected using the End-point Chromogenic Tachypleus Amoebocyte Lysate kit (Xiamen Bioendo Technology Co., Ltd, Xiamen, China) according to manufacturer’s instructions. The rumen fluid was thawed and centrifuged at 10,000 × g for 15 min, then the supernatant was transferred into another depyrogenated centrifuge tube and recentrifuged at 10,000 × g for 30 min. The supernatant was filtered through a 0.22-μm pyrogen-free filter (Millex; Millipore Corporation, Bedford, MA) and collected into a depyrogenated glass tube. It was then heated at 100 °C for 30 min, cooled at room temperature (about 25 °C) for 10 min and subsequently diluted using pyrogen-free water to attain an LPS concentration range of 0.1 to 1 EU/mL, as suggested by the manufacturer before the assay.
Plasma cytokines including Toll-like receptor 4, interleukin 6 and tumour necrosis factor-alpha were determined using the goat ELISA kit (YIFEIXUE BIO TECH, Nanjing, Jiangsu, China). The acute phase proteins including LPS-binding protein, haptoglobin and serum amyloid A were assayed using the goat ELISA kit (YIFEIXUE BIO TECH, Nanjing, Jiangsu, China). The antioxidant indices of plasma include total antioxidant capacity, malondialdehyde production, superoxide dismutase and glutathione peroxidase activity were analysed using commercial kits (YIFEIXUE BIO TECH, Nanjing, Jiangsu, China) and determined on a microplate reader (MultiskanEX, Thermo Fisher Scientific, Waltham, MA) according to the manufacturers’ procedures.
Microbial DNA was extracted using the FastDNA®soil DNA kit (Omega Bio-tek Norcross, GA, USA) according to the manufacturer’s protocol. The final DNA concentration and purification were determined by NanoDrop 2000 UV-vis spectrophotometer (Thermo Scientific, Wilmington), and DNA quality was checked by 1% agarose gel electrophoresis. The V3-V4 hypervariable regions of the bacteria 16S rRNA gene were amplified with primers 338 F (5′-ACTCCTACGGGAGGCAGCAG-3′) and 806 R (5′-GGACTACHVGGGTWTCTAAT-3′) by thermocycler PCR system (GeneAmp 9700, ABI). The PCR reactions were conducted using the following program: 3 min of denaturation at 95 °C, 27 cycles of 30 s at 95 °C, 30 s for annealing at 55 °C and 45 s for elongation at 72 °C, and a final extension at 72 °C for 10 min. PCR reactions were performed in triplicate 20-μL mixture containing 4 μL of 5 × FastPfu Buffer, 2 μL of 2.5 mM dNTPs, 0.8 μL of each primer (5 μM), 0.4 μL of FastPfu Polymerase and 10 ng of template DNA. The resulting PCR products were extracted from a 2% agarose gel and further purified using the AxyPrep DNA Gel Extraction Kit (Axygen Biosciences, Union City, CA) and quantified using QuantiFluor TM -ST (Promega) according to the manufacturer’s protocol. Purified amplicons were pooled in equimolar and paired-end sequenced (2 × 300) on an Illumina MiSeq platform (Illumina, San Diego) according to the standard protocols by Majorbio Bio-Pharm Technology Co. Ltd (Shanghai, China).Raw fastq files were quality-filtered by Trimmomatic and merged by FLASH with the following criteria: (i) The reads were truncated at any site receiving an average quality score <20 over a 50 bp sliding window. (ii) Sequences whose overlap being longer than 10 bp were merged according to their overlap with mismatch no more than 2 bp. (iii)Sequences of each sample were separated according to barcodes (exactly matching) and Primers (allowing 2 nucleotide mismatching), and reads containing ambiguous bases were removed. Operational taxonomic units (OTUs) were clustered with 97% similarity cut-off using UPARSE(version 7.1 http://drive5.com/uparse/) with a novel ‘greedy’ algorithm that performs chimaera filtering and OTU clustering simultaneously. The taxonomy of each 16S rRNA gene sequence was analysed by RDP Classifier algorithm (http://rdp.cme.msu.edu/) against the Silva (SSU123) 16S rRNA database using confidence threshold of 70% (the resulting community compositions are only useful for comparisons across treatments).
Statistical analysis
Data of this study were analysed by one-way ANOVA using SPSS software (version 23) according to the following model: Yij = μ + Di + Cj + eij, where Yij is the observation of dependent variables; μ is the overall mean; Di represents the fixed effect of treatment; Cj is the random goat effect and eij is the residual error for the observation. Differences among treatment means were classified using Tukey’s post hoc test for multiple comparisons. P ≤ 0.05 was considered statistically significant, whereas a tendency was considered at 0.05 < P ≤ 0.10.
Results
Growth performance
Feed intake and body weight gain as influenced by CON and HC/or naringin supplementation are presented in Table . The results showed that final BW and ADG were greater by feeding high-concentrate diet compared with CON group (p < 0.05), whereas ratio of feed to body weight gain was decreased at HC and THC group compared with CON group (p < 0.05). Howerver, final BW, ADG, ADFI and feed: gain ratio were not affected by dietary supplementation of naringin when compared with HC group (p > 0.05).
Table 2. Effect of naringin supplementation on the performance of goats fed high concentrate diet.
Ruminal fermentation
High-concentrate diets significantly lowered the ruminal pH value (p < 0.05, Table ), whereas dietary naringin supplementation partially alleviated ruminal pH reduction (p < 0.05). Moreover, high-concentrate diets increased (p < 0.05) the concentrations of NH3-N and decreased molar proportions of acetate (p < 0.05). There was no significant difference in the concentration of total volatile fatty acids among groups (P = 0.447). However, higher molar proportions of propionate and valerate were observed in HC group compared with CON group (p < 0.05). The THC group had lower molar proportions of valerate compared with HC group (p < 0.05). Moreover, compared with the HC group, the THC group increased numerically the molar proportions of butyrate, although the difference did not attain a significant level.
Table 3. Ruminal pH, short-chain fatty acid (SCFA) concentrations in rumen fluid and lipopolysaccharide (LPS) concentrations in rumen fluid and plasma in goats feeding different diet.
LPS concentrations, inflammatory response and antioxidant activities
The HC group had higher LPS content in rumen fluid (p < 0.05) and plasma (p < 0.05) than the CON group (Table ). Naringin supplementation decreased ruminal LPS, as compared with the HC group. The plasma LPS of the THC group was numerically lower than that of the HC group, although the difference was not statistically significant.
As shown in Table , the concentrations of SAA in the HC group were significantly increased compared with the CON group (p < 0.05). In addition, compared with the CON group, the THC group increased numerically the concentrations of LBP and Hp.
Table 4. Effects of naringin supplementation on acute phase protein and cytokines in plasma of goats fed high concentrate diet.
Naringin supplementation led to lower concentrations of SAA (p < 0.05) compared with the HC group. With respect to plasma cytokines, the concentrations of IL-6 and TNF-α of the HC group were higher (p < 0.05) compared with the CON group. Supplementation of naringin in the THC group decreased (p < 0.05) the IL-6 levels compared with the HC group.
The antioxidant status of plasma is shown in Figure . Feeding the HC group significantly increased (p < 0.05) the MDA levels of plasma compared with feeding the CON. Naringin supplementation in THC group resulted in lower (p < 0.05) levels MDA and higher (p < 0.05) levels GSH compared with the HC group. Moreover, no significant differences were observed among groups in SOD and T-AOC of plasma.
Ruminal bacterial community
In total, 777,539 high-quality sequences were generated from 12 rumen fluid samples with an average of 64,794 sequences per sample. Sequences were clustered into OTUs according to the sequence similarity level of 97%. As the rarefaction curves of Shannon index reached a plateau (Figure ), it indicated that the sequencing depth in the present study was enough to detect most of the bacteria. Compared with the CON, the HC and THC reduced numerically almost the all alpha indices, although the difference did not attain a significant level.
Figure 2. (A) Rarefaction curves of Shannon index of rumen fluid bacterial community in goats fed different diets. (B) Rarefaction curves of Sobs index on OUT level of rumen fluid bacterial community in goats fed different diets.
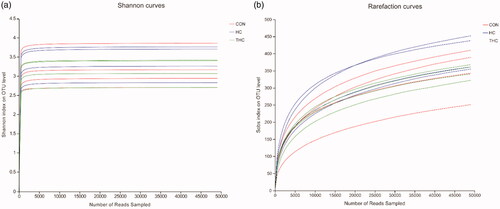
Results of the PCoA based on unweighted UniFrac distances illustrated the variation of rumen fluid microbial communities across the diets (Figure ). The PCoA axis 1 (PC1) accounted for 33.46% of the variation and the axis 2 (PC2) accounted for 20.74% of the variation. There was a clear boundary between the CON and HC clusters, revealing that the bacterial structures were different between these two groups. The THC cluster was not completely separated from that of the HC and had some overlaps.
Figure 3. Principal coordinate analysis of ruminal bacteria in goats fed different diets. PC1, PCoA axis 1; PC2, PCoA axis 2.
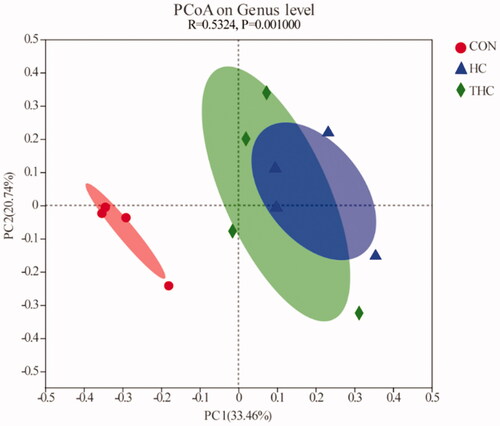
Results of rumen bacteria revealed shifts in relative abundance at phylum and genus levels among experimental groups. At the phylum level (Figure ), the most abundant bacterial phyla in the rumen fluid were Firmicutes, Bacteroidetes and Actinobacteria among the three groups. Compared with the CON, HC increased (p < 0.05) the relative abundance of Firmicutes and decreased (p < 0.05) the proportions of Bacteroidetes. The THC had higher (p < 0.05) Bacteroidetes and lower (p < 0.05) Firmicutes relative abundance compared with the HC. Moreover, the relative abundance of Proteobacteria was higher (p < 0.05) in the HC and THC compared with the CON. There were no significant shifts (p > 0.05) detected in the relative abundance of Desulfobacterota, Patescibacteria, Cyanobacteria, Spirochaetota, Synergistota.
Figure 4. Bacterial composition in rumen fluid at the phylum level when goat was fed different diets.
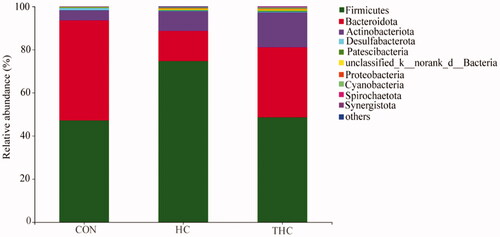
At the genus level as shown in Figure , the dominant genera of the CON, HC and THC groups were Prevotella, Ruminococcus and Rikenellaceae_RC9_gut_group, respectively. Compared with the CON, the HC and THC decreased (p < 0.05) the relative abundance of Prevotella and Veillonellaceae_UCG-001. But the percentages of these two genera were not different between the HC and THC groups (p > 0.05). Naringin supplementation increased (p < 0.05) Rikenellaceae_RC9_gut_group relative abundance and decreased (p < 0.05) that of Lachnospiraceae_ND3007_group compared with the HC.
Discussion
The goats generally are given high concentrate diet to meet higher energy requirement in the fattening stage to achieve higher growth performance. Dietary concentrate is rapidly fermented by ruminal microorganisms to produce SCFAs (Kim et al. Citation2018; Ma et al. Citation2018). Although the ADFI was not affected by feed supplementation, feeding the HC shifted the fermentation pattern to produce a less acetate and more propionate and valerate compared with the CON treatment. This could explain the higher growth performance observed in this study, because propionate and valerate are conducive to enhancing growth performance (Bergman Citation1990).
In this study, feeding the HC promoted ruminal pH reduction and LPS release, as compared with feeding the CON group. LPS originates from cell wall of Gram-negative bacteria, and is released due to cell lysis (Dong et al. Citation2011). High-concentrate diet induced lower ruminal pH, which is consistent with the results of other study (Khafipour et al. Citation2016). The lower ruminal pH can result in more deaths of Gram-negative bacteria and excessive release of LPS. When LPS was translocated from the rumen epithelium into the blood, it ultimately induced body inflammatory responses (Li et al. Citation2012; Eckel and Ametaj Citation2016; Chang et al. Citation2018; Humer et al. Citation2018). It has been previously demostrated that translocation of LPS from the rumen into blood could increase pro-inflammatory cytokines release and APPs production (Dong et al. Citation2011). Similarly, we observed that compared with the CON, the HC significantly increased plasma LPS concentration, and accordingly the higher concentrations of proinflammatory cytokines including IL-6 and TNF-α as well as APPs including SAA were observed in our present study. Moreover, we have observed that dietary supplementation with naringin attenuated host inflammatory responses. It has been reported in previous studies that naringin can reduce inflammation or enhance immune function by regulating pro-inflammatory cytokines, anti-inflammatory cytokines and their gene expression (Dourado et al. Citation2014). This may explain the weakening of inflammatory response in THC group. Evidence from previous studies indicates that high concentrate diets promoted oxidative stress and reduced antioxidant status as a result of an increase in the production of reactive oxygen species, lipid peroxidation or impairment of the antioxidant defense systems, which can result in a marked decrease in blood concentration of the markers of antioxidant capacity, including the activities of antioxidant enzymes, e.g. superoxide dismutase, catalase and glutathione peroxidase. In the current study, antioxidant status in response to naringin supplementation resulted in a significant increase in antioxidant status including decreased malondialdehyde values and increased activities of glutathione peroxidase. This ameliorating effect of naringin on antioxidant status during fattening period in goat is consistent with previous reports, which naringin dietary supplementation at 0.15% rates improved antioxidant status of the liver of lambs (Bodas et al. Citation2012). In addition, Similar results were also reported for naringin in rabbits (Jeon et al. Citation2001), rats (Seo et al. Citation2003) and mice (Kim et al. Citation2004). The mechanism of which naringin improve antioxidant status may related to its containing two phenolic hydroxyl groups with strong activity. Phenolic hydroxytong can improve the activity of superoxide dismutase, glutathione peroxidase and catalase by combining with free radicals, reactive oxygen species and some transition metal ion in the body activity and antioxidant effect.
It has been previously demonstrated that high-concentrate diet feeding increased the relative abundance of Firmicutes and decreased that of Bacteroidetes at the phylum level and that of Prevotella at the genus level compared with low-concentrate diet (Liu et al. Citation2014; Khafipour et al. Citation2016; Hua et al. Citation2017). In accordance with these studies, Firmicutes had surpassed Bacteroidetes to become the most abundant phylum in the rumen fluid of the HC group in this study. Moreover, our observations revealed that dietary supplementation with naringin had improved the abundance of Bacteroidetes, this may be attributed to the increased ruminal pH and the decreased ruminal LPS.
Our results showed that the abundance of the genus Prevotella decreased when goats were fed the HC compared with the CON group, which concords with the results from Mao (Mao et al. Citation2013) and Kim (Kim et al. Citation2018). Although the Prevotella abundance was reduced in the HC group, it still remained one of the predominant genera. In addition, we also observed in this study that naringin supplementation into the THC significantly increased relative abundance of Rikenellaceae_RC9_gut_group. Rikenellaceae_RC9_gut_group is classified in the Rikenellaceae family and members of these families are hydrogen-producing bacteria that selectively neutralise cytotoxic reactive oxygen species (Xie et al. Citation2019). It has been reported that endogenous hydrogen mediates suppression of proinflammatory cytokines, especially, IL-1, TNF-α, IL-6 in inflammatory tissues (Chen et al. Citation2011). This might be one of the possible reasons that the naringin supplementation in high-concentration diet reduced the host’s inflammatory response and oxidative stress. Most genera of Lachnospiraceae have been associated with the production of propionate (Schären et al. Citation2017; San-Juan-Vergara et al. Citation2018). In our study, we observed that the abundance of Lachnospiraceae_ND3007_group was higher in the HC group among the groups, which was consistent with the above results that higher molar proportions of propionate in the HC group. In addition, this study also shows that the relative abundance of Christensenellaceae_R-7_group, NK4A214_group, norank_f__F082, norank_f__Eubacterium_coprostanoligenes_group and norank_f__Bifidobacteriaceae have also changed, which may indicate that these bacteria may also play an important physiological and ecological role in rumen.
Conclusion
In conclusion, feeding the high concentrate diet promoted the pH reduction and the LPS release in the rumen. Furthermore, feeding the high-concentrate diet increased inflammatory response and disturbed the ruminal bacterial stability. Dietary naringin supplementation increased butyrate ratio and modified the ruminal bacterial composition. In addition, naringin supplementation could alleviate the chronic inflammatory response and oxidative stress by suppressing serum SAA, TNF-α, IL-6 and improving the activities of antioxidant enzymes in goats feeding high-concentrate diet. Therefore, Naringin has potential for use as feed additive to alleviate inflammatory response and to modify ruminal fermentation in a high grain intensive ruminant production.
Acknowledgment
The authors gratefully thank Dr Fangfang Zhao, Dr Yaqian Jin, Dr Yi Ma and Xinzhao Zhang, Ying Zhang and Yaotian Fan for assistance with sample collection.
Software and data repository resources: The raw sequencing data were submitted to the Sequence Read Archive (SRA) of National Centre for Biotechnology Information (NCBI) (http://www.ncbi.nlm.nih.gov/sra) under the accession number of PRJNA821647.
Ethical approval
This study was performed in accordance with the approved regulations for the administration of affairs concerning experimental animals. The experimental procedures were approved by the animal welfare and ethics committee of Yangzhou University.
Disclosure statement
No potential conflict of interest was reported by the authors.
Data availability statement
The results and analyses presented in this paper freely available upon request.
Additional information
Funding
References
- Alhidary IA, Abdelrahman MM. 2016. Effects of naringin supplementation on productive performance, antioxidant status and immune response in heat-stressed lambs. Small Ruminant Res. 138:31–36.
- Aroui S, Najlaoui F, Chtourou Y, Meunier A-C, Laajimi A, Kenani A, Fetoui H. 2016. Naringin inhibits the invasion and migration of human glioblastoma cell via downregulation of MMP-2 and MMP-9 expression and inactivation of p38 signaling pathway. Tumour Biol. 37(3):3831–3839.
- Bergman EN. 1990. Energy contributions of volatile fatty acids from the gastrointestinal tract in various species. Physiol Rev. 70(2):567–590.
- Bodas R, Prieto N, Jordán MJ, López-Campos Ó, J. Giráldez F, Morán L, Andrés S. 2012. The liver antioxidant status of fattening lambs is improved by naringin dietary supplementation at 0.15% rates but not meat quality. Animal. 6(5):863–870.
- Chang G, Liu X, Ma N, Yan J, Dai H, Roy AC, Shen X. 2018. Dietary addition of sodium butyrate contributes to attenuated feeding-induced hepatocyte apoptosis in dairy goats. J Agric Food Chem. 66(38):9995–10002.
- Chen X, Zuo Q, Hai Y, Sun XJ. 2011. Lactulose: an indirect antioxidant ameliorating inflammatory bowel disease by increasing hydrogen production. Med Hypotheses. 76(3):325–327.
- Cho KW, Kim YO, Andrade JE, Burgess JR, Kim Y-C. 2011. Dietary naringenin increases hepatic peroxisome proliferators–activated receptor α protein expression and decreases plasma triglyceride and adiposity in rats. Eur J Nutr. 50(2):81–88.
- Dong G, Liu S, Wu Y, Lei C, Zhou J, Zhang S. 2011. Diet-induced bacterial immunogens in the gastrointestinal tract of dairy cows: impacts on immunity and metabolism. Acta Vet Scand. 53(1):47–48.
- Dourado G, Ribeiro L, Carlos IZ, et al. 2014. Orange juice and hesperidin promote differential innate immune response in macrophages ex vivo. Int J Vit Nutr Res. 83(3):162–167.
- Eckel EF, Ametaj BN. 2016. Invited review: Role of bacterial endotoxins in the etiopathogenesis of periparturient diseases of transition dairy cows. J Dairy Sci. 99(8):5967–5990.
- Gabay C, Kushner I. 1999. Acute-phase proteins and other systemic responses to inflammation. N Engl J Med. 340(6):448–454.
- Goliomytis M, Simitzis P, Papalexi A, Veneti N, Hager-Theodorides AL, Charismiadou MA, Deligeorgis SG. 2019. Influence of citrus flavonoids on laying hen performance, inflammatory immune response, egg quality and yolk oxidative stability. Br Poultr Sci. 60(3):272–278.
- Hara H, Orita N, Hatano S, Ichikawa H, Hara Y, Matsumoto N, Kimura Y, Terada A, Mitsuoka T. 1995. Effect of tea polyphenols on fecal flora and fecal metabolic products of pigs. J Vet Med Sci. 57(1):45–49.
- Henning PH, Horn CH, Leeuw K-J, Meissner HH, Hagg FM. 2010. Effect of ruminal administration of the lactate-utilizing strain Megasphaera elsdenii (Me) NCIMB 41125 on abrupt or gradual transition from forage to concentrate diets. Anim Feed Sci Technol. 157(1-2):20–29.
- Hua C, Tian J, Tian P, Cong R, Luo Y, Geng Y, Tao S, Ni Y, Zhao R. 2017. Feeding a high concentration diet induces unhealthy alterations in the composition and metabolism of ruminal microbiota and host response in a goat model. Front Microbiol. 8:138.
- Humer E, Aschenbach JR, Neubauer V, Kröger I, Khiaosa-Ard R, Baumgartner W, Zebeli Q. 2018. Signals for identifying cows at risk of subacute ruminal acidosis in dairy veterinary practice. J Anim Physiol Anim Nutr. 102(2):380–392.
- Jeon S-M, Bok S-H, Jang M-K, Lee M-K, Nam K-T, Park YB, Rhee S-J, Choi M-S. 2001. Antioxidative activity of naringin and lovastatin in high cholesterol-fed rabbits. Life Sci. 69(24):2855–2866.
- Khafipour E, Li S, Tun HM, Derakhshani H, Moossavi S, Plaizier JC. 2016. Effects of grain feeding on microbiota in the digestive tract of cattle. Anim Front. 6(2):13–19.
- Kim H-J, Oh GT, Park YB, Lee M-K, Seo H-J, Choi M-S. 2004. Naringin alters the cholesterol biosynthesis and antioxidant enzyme activities in LDL receptor-knockout mice under cholesterol fed condition. Life Sci. 74(13):1621–1634.
- Kim Y-H, Nagata R, Ohkubo A, Ohtani N, Kushibiki S, Ichijo T, Sato S. 2018. Changes in ruminal and reticular pH and bacterial communities in Holstein cattle fed a high-grain diet. BMC Vet Res. 14(1):1–10.
- Li S, Khafipour E, Krause DO, Kroeker A, Rodriguez-Lecompte JC, Gozho GN, Plaizier JC. 2012. Effects of subacute ruminal acidosis challenges on fermentation and endotoxins in the rumen and hindgut of dairy cows. J Dairy Sci. 95(1):294–303.
- Liu J, Xu T, Zhu W, Mao S. 2014. High-grain feeding alters caecal bacterial microbiota composition and fermentation and results in caecal mucosal injury in goats. Br J Nutr. 112(3):416–427.
- Ma N, Abaker JA, Bilal MS, Dai H, Shen X. 2018. Sodium butyrate improves antioxidant stability in sub-acute ruminal acidosis in dairy goats. BMC Vet Res. 14(1):1–13.
- Mao S, Huo W, Liu J, Zhang R, Zhu W. 2017. In vitro effects of sodium bicarbonate buffer on rumen fermentation, levels of lipopolysaccharide and biogenic amine, and composition of rumen microbiota. J Sci Food Agric. 97(4):1276–1285.
- Mao SY, Huo WJ, Zhu WY. 2016. Microbiome–metabolome analysis reveals unhealthy alterations in the composition and metabolism of ruminal microbiota with increasing dietary grain in a goat model. Environ Microbiol. 18(2):525–541.
- Mao SY, Zhang RY, Wang DS, Zhu WY. 2013. Impact of subacute ruminal acidosis (SARA) adaptation on rumen microbiota in dairy cattle using pyrosequencing. Anaerobe. 24:12–19.
- McCann JC, Luan S, Cardoso FC, Derakhshani H, Khafipour E, Loor JJ. 2016. Induction of subacute ruminal acidosis affects the ruminal microbiome and epithelium. Front Microbiol. 7:701.
- Mutsvangwa T, Walton JP, Plaizier JC, Duffield TF, Bagg R, Dick P, Vessie G, McBride BW. 2002. Effects of a monensin controlled-release capsule or premix on attenuation of subacute ruminal acidosis in dairy cows. J Dairy Sci. 85(12):3454–3461.
- Nie Y-C, Wu H, Li P-B, Luo Y-L, Long K, Xie L-M, Shen J-G, Su W-W. 2012. Anti-inflammatory effects of naringin in chronic pulmonary neutrophilic inflammation in cigarette smoke-exposed rats. J Med Food. 15(10):894–900.
- Paz HA, Anderson CL, Muller MJ, Kononoff PJ, Fernando SC. 2016. Rumen bacterial community composition in Holstein and Jersey cows is different under same dietary condition and is not affected by sampling method. Front Microbiol. 7:1206.
- Plaizier JC, Danesh Mesgaran M, Derakhshani H, Golder H, Khafipour E, Kleen JL, Lean I, Loor J, Penner G, Zebeli Q, et al. 2018. Review: Enhancing gastrointestinal health in dairy cows. Animal. 12(s2):s399–s418.
- Plaizier JC, Li S, Le Sciellour M, Schurmann BL, Górka P, Penner GB. 2014. Effects of duration of moderate increases in grain feeding on endotoxins in the digestive tract and acute phase proteins in peripheral blood of yearling calves. J Dairy Sci. 97(11):7076–7084.
- Rhodes MJC. 1996. Physiologically-active compounds in plant foods: an overview. Proc Nutr Soc. 55(1B):371–384.
- San-Juan-Vergara H, Zurek E, Ajami NJ, Mogollon C, Peña M, Portnoy I, Vélez JI, Cadena-Cruz C, Diaz-Olmos Y, Hurtado-Gómez L, et al. 2018. A Lachnospiraceae-dominated bacterial signature in the fecal microbiota of HIV-infected individuals from Colombia, South America. Sci Rep. 8(1):1–13.
- Schären M, Drong C, Kiri K, Riede S, Gardener M, Meyer U, Hummel J, Urich T, Breves G, Dänicke S, et al. 2017. Differential effects of monensin and a blend of essential oils on rumen microbiota composition of transition dairy cows. J Dairy Sci. 100(4):2765–2783.
- Seo H-J, Jeong K-S, Lee M-K, Park YB, Jung UJ, Kim H-J, Choi M-S. 2003. Role of naringin supplement in regulation of lipid and ethanol metabolism in rats. Life Sci. 73(7):933–946.
- Seradj AR, Gimeno A, Fondevila M, Crespo J, Armengol R, Balcells J. 2018. Effects of the citrus flavonoid extract Bioflavex or its pure components on rumen fermentation of intensively reared beef steers. Anim Prod Sci. 58(3):553–560.
- Sun H, Luo Y, Zhao F, Fan Y, Ma J, Jin Y, Hou Q, Ahmed G, Wang H. 2020. The effect of replacing wildrye hay with mulberry leaves on the growth performance, blood metabolites, and carcass characteristics of sheep. Animals. 10(11):2018.
- Tripoli E, Guardia ML, Giammanco S, Majo DD, Giammanco M. 2007. Citrus flavonoids: Molecular structure, biological activity and nutritional properties: a review. Food Chem. 104(2):466–479.
- Vyas D, Beauchemin KA, Koenig KM. 2015. Using organic acids to control subacute ruminal acidosis and fermentation in feedlot cattle fed a high-grain diet. J Anim Sci. 93(8):3950–3958.
- Xie Y, Li W, Zhu L, Zhai S, Qin S, Du Z. 2019. Effects of phycocyanin in modulating the intestinal microbiota of mice. Microbiologyopen. 8(9):e00825.
- Zhou J, Dong G, Ao C, Zhang S, Qiu M, Wang X, Wu Y, Erdene K, Jin L, Lei C, et al. 2014. Feeding a high-concentrate corn straw diet increased the release of endotoxin in the rumen and pro-inflammatory cytokines in the mammary gland of dairy cows. BMC Vet Res. 10(1):1–10.