ABSTRACT
The COP9 signalosome inhibits the activity of Cullin-RING E3 ubiquitin ligases by removing Nedd8 modifications from their Cullin subunits. Neddylation renders these complexes catalytically active, but deneddylation is also necessary for them to exchange adaptor subunits and avoid auto-ubiquitination. Although deneddylation is thought to be the primary function of the COP9 signalosome, additional activities have been ascribed to some of its subunits. We recently showed that COP9 subunits protect the transcriptional repressor and tumor suppressor Capicua from two distinct modes of degradation. Deneddylation by the COP9 signalosome inactivates a Cullin 1 complex that ubiquitinates Capicua following its phosphorylation by MAP kinase in response to Epidermal Growth Factor Receptor signaling. The CSN1b subunit also stabilizes unphosphorylated Capicua to control its basal level, independently of the deneddylase function of the complex. Here we further examine the importance of deneddylation for COP9 functions in vivo. We use an uncleavable form of Nedd8 to show that preventing deneddylation does not reproduce the effects of loss of COP9. In contrast, in the presence of COP9, conjugation to uncleavable Nedd8 renders Cullins unable to promote the degradation of their substrates. Our results suggest that irreversible neddylation prolongs COP9 binding to and inhibition of Cullin-based ubiquitin ligases.
Introduction
Protein turnover plays a major role in many cellular processes, and deficiencies in protein degradation are associated with disease and aging [Citation1]. Proteins are usually marked for proteasomal degradation by covalent modification with the small protein ubiquitin. One major family of E3 ubiquitin ligases, the Cullin-RING ligases (CRLs), uses Cullin scaffolding subunits to link specific substrate-binding subunits to RING subunits that recruit E2 ubiquitin-conjugating enzymes [Citation2]. Understanding the regulation of CRL activity is vital because many CRL substrates would profoundly disrupt normal physiology if their levels were not kept in check. Cyclical processes such as circadian rhythms and the cell cycle depend on protein degradation initiated by CRL ligases [Citation3,Citation4]. Many intercellular signaling pathways likewise hinge on regulating the levels of specific signaling intermediates. If such proteins are not properly degraded or stabilized, their abnormal level can lead to unregulated growth and metastasis. For example, accumulation of β-catenin in the absence of proteins that mark it for ubiquitination by a CRL complex and subsequent degradation is a common cause of colon cancer [Citation5]. Conversely, the transcriptional repressor Capicua (Cic) acts as a suppressor of tumorigenesis and metastasis [Citation6–8]. We recently showed that Cic is also a substrate for CRL ubiquitination in cells in which the Epidermal Growth Factor Receptor (EGFR) pathway is active. Ubiquitination of phosphorylated Cic by the SkpA-Cul1-Archipelago CRL allows the expression of EGFR target genes [Citation9].
CRLs are themselves modified by the addition of a ubiquitin-like peptide, Nedd8, to their Cullin subunit [Citation10]. Neddylation is essential for CRL activity because it induces a conformational change at the Cullin-RING interface that allows the RING protein more flexibility to bring together the E2 conjugating enzyme and the substrate for ubiquitination [Citation11–13]. Consistent with this requirement, loss of Nedd8 function by mutation or by inhibition of the Nedd8-activating enzyme with the drug MLN4924 causes a failure to degrade CRL substrates [Citation14,Citation15]. Nedd8 is removed from Cullins by the COP9 signalosome (CSN), a complex with at least eight subunits that is evolutionarily related to the lid of the 26S proteasome [Citation16,Citation17] (). Paradoxically, early studies found that in vivo, loss of CSN function impairs the degradation of some CRL substrates [Citation18,Citation19]. These findings led to an appreciation of the importance of cycles of neddylation and deneddylation for CRL function [Citation20]. Such cycles are necessary for adaptor subunit exchange, catalyzed by the exchange factor CAND1[Citation21–25], and to prevent auto-ubiquitination of the CRL complexes in the absence of substrates [Citation26,Citation27]. The consequences of deneddylation may vary for different ligases and substrates. In Drosophila, loss of CSN subunits can result in increased CRL activity and reduced levels of CRL substrates such as Cubitus interruptus (Ci), the transcription factor downstream of Hedgehog signaling, and Armadillo (Arm)/β-catenin [Citation28]. However, other substrates such as Cactus, Timeless and Cyclin E show increased levels [Citation29–31]. It is not clear whether this variability reflects different effects of neddylation on the relevant CRLs, or CSN functions other than deneddylation [Citation32–34].
Figure 1. A Nedd8 mutation designed to prevent deneddylation. (A) The COP9 signalosome deneddylates Cullins, reducing the activity of CRL ubiquitin ligase complexes. We predicted that uncleavable Nedd8 would have the same effect as CSN loss of function, preventing Cullin deneddylation and increasing CRL activity. (B) Diagram of the UAS-Nedd8 transgenes that were either transfected into S2 cells or injected into Drosophila embryos. Uncleavable Nedd8 harbors an amino acid change from leucine to proline at position 73.
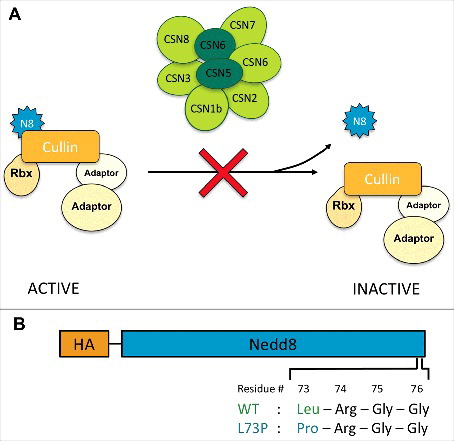
A number of studies have suggested functions for CSN subunits in addition to the deneddylase activity of the complex, which resides in the CSN5 subunit [Citation19,Citation35,Citation36]. Most of the other subunits have primarily structural roles within the holo-complex, although CSN1, CSN2 and CSN4 also contact CRL subunits [Citation37–39]. While it has been proposed that the CSN can inhibit CRLs by binding and occluding their substrate binding sites [Citation32], recent kinetic and structural studies demonstrated that deneddylated Cullins rapidly dissociate from the CSN [Citation38,Citation39]. Removal of certain subunits can modify CSN activity; for instance, sequestration of the CSN4 subunit during ovarian development alters the function of the remainder of the complex [Citation40], and excess CSN6 competes with CSN5 to bind Cullins, preventing their deneddylation [Citation41]. Some CSN subunits also have functions independent of the signalosome, either on their own or within CSN sub-complexes [Citation35]. CSN1, CSN2, CSN4 and CSN8 have been reported to act as direct transcriptional regulators [Citation34,Citation42–47], and the CSN also recruits kinases and de-ubiquitinases [Citation33,Citation48–50]. Interestingly, we found that CSN subunits affected Cic stability by two distinct mechanisms, only one of which was deneddylase-dependent. Loss of CSN5 destabilized Cic that had been phosphorylated by MAPK as a result of EGFR pathway activation, and removing the MAPK interaction domain [Citation51] from Cic could compensate for the absence of CSN5. In contrast, mutations affecting the CSN1b subunit also destabilized the form of Cic that lacked the domain required for phosphorylation by MAPK. We found that CSN1b coimmunoprecipitated with Cic, suggesting the possibility that it stabilizes Cic by direct binding [Citation9].
In order to test which effects of CSN loss result from the failure to deneddylate CRL complexes, we used an alternative approach to prevent this deneddylation. We designed a form of Nedd8 that was conjugated onto Cullins but could not be removed by the CSN [Citation52]. We expected that replacing endogenous Nedd8 with this uncleavable form would resemble the effects of CSN loss, leading to the activation of CRL ubiquitin ligases and increased degradation of substrates such as Cic and Ci [Citation9,Citation28]. Surprisingly, we found that CRL substrates actually accumulated in cells expressing only uncleavable Nedd8, indicating that in the presence of the CSN, preventing deneddylation blocks CRL activity rather than promoting it. We suggest that the CSN remains bound to neddylated CRLs and prevents them from interacting with their substrates.
Results and discussion
A cleavage-resistant form of Nedd8
If deneddylation is the primary function of the CSN, then preventing the removal of Nedd8 from CSN substrates should have the same effect as loss of the CSN (). To test this prediction, we generated a cleavage-resistant form of Nedd8. Mutating leucine 73 to proline in ubiquitin prevents its cleavage by deubiquitinases, while still allowing its conjugation to substrates [Citation53]. The corresponding L73P mutation in mammalian Nedd8 similarly prevents its removal from Cullins by the COP9 signalosome [Citation52]. We constructed transgenes that expressed HA-tagged wild-type Drosophila Nedd8 or Nedd8L73P under the control of UAS sequences and inserted them at the same genomic locus; the coding sequence terminated at glycine 76, before the eight C-terminal amino acids that must be cleaved to allow conjugation to target proteins [Citation54] (). When expressed in S2 cells, HA-Nedd8L73P was more stably conjugated to Cullin 3 (Cul3) than wild-type HA-Nedd8, indicating that it is recognized by the neddylation machinery but not effectively removed (). Similarly, increased conjugation of Nedd8L73P to Cullins was detected when the transgenes were expressed in Nedd8 mutant clones in wing imaginal discs (). We observed no dominant phenotype when either transgene was overexpressed in a wild-type background, either ubiquitously with tubulin (tub)-GAL4 or in the dorsal wing disc with apterous (ap)-GAL4 (, ). This may be because HA-Nedd8 protein expressed from the transgene is unstable in the presence of endogenous Nedd8; higher levels of HA-Nedd8 were detected in Nedd8 mutant cells than in adjacent wild-type cells (). It is possible that the HA tag or the absence of the C-terminus reduces the efficiency of conjugation of transgenic Nedd8, and that unconjugated Nedd8 is degraded.
Figure 2. Nedd8L73P is conjugated onto Cullins and does not dominantly affect development. (A) Lysates from S2 cells transfected with FLAG-Cul3 alone or together with HA-Nedd8WT or HA-Nedd8L73P were immunoprecipitated with anti-HA and blotted with anti-FLAG (upper panel). Lower panels show the input blotted with anti-FLAG, anti-HA, anti-Arm and anti-β-tubulin. More Nedd8L73P than wild-type Nedd8 is present as a Cul3 conjugate. (B) Lysates of wing discs in which HA-Nedd8WT or HA-Nedd8L73P was specifically expressed only in GFP-labeled Nedd8 mutant clones, blotted with anti-HA, anti-Nedd8, anti-GFP and anti- β-tubulin. Both forms of Nedd8 are present in a band at the expected molecular weight of Drosophila Cullins, but Nedd8L73P gives rise to stronger HA and Nedd8 labeling of this band. (C-E) Wing discs stained with anti-HA (C’, D’, E’, green in C, D, blue in E) and anti-Ci (C’’, D’’, E’’, magenta in C, D, red in E). HA-Nedd8WT (C) or HA-Nedd8L73P (D) is driven in the dorsal compartment by ap-GAL4, but has no dominant effect on Ci expression. In (E), HA-Nedd8WT is driven with ap-GAL4 in a wing disc in which Nedd8 mutant clones are marked by the absence of RFP (green). Transgenic tagged Nedd8 rescues Ci accumulation and clone growth in the dorsal compartment, and accumulates to higher levels in clones that lack endogenous Nedd8.
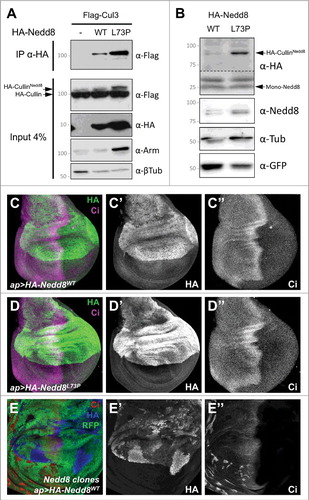
Preventing Nedd8 cleavage inhibits cullin function
We found that the two transgenes differed dramatically in their ability to substitute for endogenous Nedd8. When expressed ubiquitously with tub-GAL4 in a Nedd8 mutant background, wild-type HA-Nedd8 rescued the mutants, which normally die as second instar larvae [Citation14], to the pharate adult stage. HA-Nedd8L73P expression, on the other hand, did not prolong the survival of Nedd8 mutants. We assessed the effect of uncleavable Nedd8 on CRL function by looking at Ci, a transcription factor activated by Hedgehog signaling that can be degraded by Cullin 1 (Cul1) and Cul3-containing CRLs [Citation14,Citation55]. In Nedd8 mutant clones in the wing disc, Ci accumulated at high levels due to the absence of active SkpA-Cul1-Fbox (SCF) complex [Citation14] (). Expression of wild-type HA-Nedd8 within the clones restored Ci to its normal level (). Surprisingly, Nedd8 mutant clones that expressed HA-Nedd8L73P still showed strong accumulation of Ci, indicating that the SCF complex is inactive (). This lack of rescue by Nedd8L73P was not restricted to Cul1, as it was also observed in eye discs, where Ci is ubiquitinated by Cul3 posterior to the morphogenetic furrow [Citation14] (). This phenotype is opposite to that of cells mutant for CSN5 or CSN1b, in which excessive Cul1 neddylation results in reduced levels of Ci and its target gene dpp [Citation9,Citation28].
Figure 3. Nedd8L73P does not rescue CRL function. (A, B, C) Wing discs and (D, E, F) eye discs in which Nedd8 mutant clones are positively labeled with GFP (green) and express no transgene (A, D), HA-Nedd8WT (B, E), or HA-Nedd8L73P (C, F). Discs are stained with anti-Ci (A’, B’, C’, D’, E’, F’, red in A, B, C, magenta in D, E, F) and anti-Nedd8 (A’’, B’’, C’’, blue in A-C). Ci, which is a substrate for a Cul1 ligase in the wing disc and anterior eye disc and for a Cul3 ligase in the posterior eye disc, accumulates in Nedd8 mutant clones and Nedd8 mutant clones rescued with Nedd8L73P, but not in Nedd8 clones rescued with Nedd8WT. Arrows indicate representative clones, and yellow arrowheads mark the morphogenetic furrow in the eye discs.
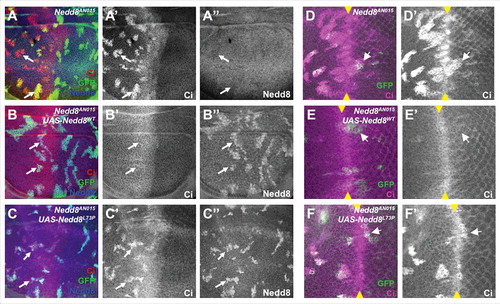
Persistent cullin neddylation may promote prolonged CSN binding
We anticipated that replacing wild-type Nedd8 with Nedd8L73P, which cannot be removed from Cullins, would have the same effect as loss of CSN function, enhancing the ability of CRLs to ubiquitinate substrates such as Ci. However, our results clearly show the opposite: uncleavable Nedd8 strongly inhibits the function of Cul1 and Cul3, leading to Ci stabilization. An important difference between Nedd8L73P substitution and CSN subunit mutation is that in the Nedd8L73P condition, the CSN is still intact. Recent crystallographic and kinetic studies demonstrated that deneddylation dramatically reduces the affinity of CRL complexes for the CSN [Citation17,Citation38,Citation39], implying that Cullins conjugated to Nedd8L73P may remain bound to the CSN. As CSN binding to SCF complexes occludes sites for the E2 ubiquitin conjugating enzyme and the substrate [Citation32,Citation56], constitutive binding to permanently neddylated complexes would sterically inactivate them. Persistent neddylation would also interfere with adaptor subunit exchange by CAND1[Citation23,Citation24], potentially affecting the degradation of specific substrates. Deneddylated Cul1 itself also has an important role in stabilizing adaptor subunits in Neurospora [Citation57], providing another possible explanation for the toxic effects of uncleavable Nedd8.
Alternatively, the effect of Nedd8L73P could be due to its attachment to proteins other than Cullins, which are deneddylated by Deneddylase 1 (Den1) rather than by the CSN [Citation58,Citation59]. In mammalian cells, Nedd8L73P becomes attached to the Nedd8 E2-conjugating enzyme Ubc12 and inhibits its activity, resulting in reduced CRL neddylation and function [Citation52]. However, this hypothesis seems unlikely to explain our results, because Den1 null mutant alleles are viable and fertile with reduced apoptosis [Citation58,Citation60], while Nedd8 mutants expressing Nedd8L73P die early in development and mutant cells survive poorly. In addition, our data show that Cullin neddylation is increased in Nedd8 mutant cells expressing Nedd8L73P (). The accumulation of the Cullin substrate Ci argues that Nedd8L73P reduces Cullin activity, supporting the model that cycles of neddylation and deneddylation are essential for continued Cullin function [Citation57,Citation61].
Outlook
The ubiquitin-proteasome system regulates many proteins that are involved in tumorigenesis and metastasis, such as the human Ci and Cic homologues GLI and CIC, making it a useful target for anti-cancer drugs [Citation8,Citation62]. Although proteasome inhibitors such as MG132 and bortezomib can effectively block the degradation of tumor suppressors, side effects restrict their utility to a small subset of cancers. CRL ligases can be inhibited by MLN4924, a compound that blocks the neddylation of all Cullins and limits the growth of several cancer cell lines [Citation63], and inhibitors of neddylation of specific Cullins are now becoming available [Citation64]. The CSN is also a potential drug target; the potent CSN5i-3, which binds to the active site of CSN5, causes accumulation of neddylated Cullins and promotes ubiquitination and degradation of a subset of F-box subunits, making it selective for specific tumor types [Citation65]. Understanding the interplay between neddylation, CSN binding and CRL activity will improve our ability to design therapeutics that target this system.
Materials and methods
Drosophila genetics and immunohistochemistry
UAS-Nedd8 transgenes were made by synthesizing the Nedd8 sequence, with an N-terminal HA tag and either leucine or proline at position 73, ending at glycine 76 (GenScript), and cloning it into pUASTattB. Transgenic flies were produced by Genetivision. Nedd8 clones were generated by crossing Nedd8AN015, FRT40/SM6-TM6B or Nedd8AN015, FRT40; UAS-Nedd8HA/SM6-TM6B or Nedd8AN015, FRT40; UAS-Nedd8L73PHA/SM6-TM6B males to hs-FLP, UAS-CD8GFP; tub-GAL80, FRT40/CyO; tub-GAL4/TM6c or UbxFLP; Ubi-RFPNLS, FRT40, ap-GAL4/CyO females. Eye and wing discs were stained as described [Citation66]. Antibodies used were chicken anti-GFP (1:300; Aves GFP-1020), rat anti-Ci (1:10) [Citation67], rabbit anti-Nedd8 (1:200; Cell Signaling 19E3), and rat anti-HA (1:100; Roche 3F4). Fluorescent secondary antibodies were from Jackson Immunoresearch (1:200) or Life Technologies (1:1,000) and images were obtained using a Leica SP5 confocal microscope.
Cell culture, immunoprecipitation and Western blotting
S2 cells were maintained in Schneider's medium supplemented with 10% fetal calf serum. 106 cells/well were transfected with 0.9 µg total plasmid DNA using Effectene (QIAGEN). Cells were transfected in 6-cm plates, harvested four days later and lysed in ice-cold lysis buffer (75 mM NaCl, 50 mM Tris-HCl pH 7.5, 1 mM EDTA, 0.2 % NP-40, 5 mM NaF, 1 mM Na3VO4, EDTA-free cOmplete protease inhibitor (Roche)). Lysates were incubated for 1 hour with anti-HA affinity matrix (Roche 3F10). Washes were performed using 75 mM NaCl, 10 mM Tris-HCl pH 7.5, 1 mM EDTA, 5 mM NaF, 1 mM Na3VO [Citation4], EDTA-free cOmplete protease inhibitor (Roche) and precipitates were eluted with 2X Laemmli buffer. To make wing disc protein extracts, 20 discs were lysed in 20 µl RIPA buffer supplemented with EDTA-free cOmplete protease inhibitor (Roche), 5 mM NaF and 1 mM Na3VO4. The lysate was then cleared by centrifugation and an equal volume of 2X Laemmli buffer was added. Protein samples were boiled 5 min at 95°C and loaded on 8% SDS-PAGE gels. The gels were transferred onto nitrocellulose membranes (BIORAD), which were blocked for 30 min in TBST (20 mM Tris pH 7.6, 137 mM NaCl, 0.2% Tween-20) supplemented with 5% low-fat milk, prior to incubation with primary antibodies overnight at 4°C in TBST with 5% milk. Blots were washed with TBST for 30 min and incubated with horseradish peroxidase-conjugated secondary antibodies (1:10,000; Jackson Immunoresearch) for 1 hr in TBST + 5% milk. Blots were developed with enhanced chemiluminescence (Thermo SuperSignal WestPico). Primary antibodies used were rabbit anti-Flag (1:1000; Sigma F7425), rat anti-HA (1:1000; Roche 3F4), mouse anti-Arm (1:100; DSHB N2 7A1), mouse anti-β-tubulin (1:10,000; Sigma T4026), rabbit anti-Nedd8 (1:1000; Cell Signaling 19E3), and chicken anti-GFP (1:10,000; Aves GFP-1020).
Acknowledgments
We thank Cheng-Ting Chien, Qiuling Li and the Bloomington Drosophila Stock Center for fly stocks and reagents. Information obtained from Flybase was essential to this work. We are grateful to DanQing He for technical assistance. This work was supported by the National Institutes of Health (grant EY13777 to J.E.T. and grant ES025166 to T.T.H.).
Additional information
Funding
References
- Hartl FU. Cellular homeostasis and aging. Annu Rev Biochem. 2016;85:1–4. doi:10.1146/annurev-biochem-011116-110806
- Sarikas A, Hartmann T, Pan ZQ. The cullin protein family. Genome Biol. 2011;12:220. doi:10.1186/gb-2011-12-4-220
- Freeman AA, Mandilaras K, Missirlis F, et al. An emerging role for Cullin-3 mediated ubiquitination in sleep and circadian rhythm: insights from Drosophila. Fly. 2013;7:39–43. doi:10.4161/fly.23506
- Zheng N, Wang Z, Wei W. Ubiquitination-mediated degradation of cell cycle-related proteins by F-box proteins. Int J Biochem Cell Biol. 2016;73:99–110. doi:10.1016/j.biocel.2016.02.005
- Krausova M, Korinek V. Wnt signaling in adult intestinal stem cells and cancer. Cell Signal. 2014;26:570–9. doi:10.1016/j.cellsig.2013.11.032
- Bettegowda C, Agrawal N, Jiao Y, et al. Mutations in CIC and FUBP1 contribute to human oligodendroglioma. Science. 2011;333:1453–5. doi:10.1126/science.1210557
- Dissanayake K, Toth R, Blakey J, et al. ERK/p90(RSK)/14–3-3 signalling has an impact on expression of PEA3 Ets transcription factors via the transcriptional repressor Capicua. Biochem J. 2011;433:515–25. doi:10.1042/BJ20101562
- Okimoto RA, Breitenbuecher F, Olivas VR, et al. Inactivation of Capicua drives cancer metastasis. Nat Genet. 2016;49:87–96. doi:10.1038/ng.3728
- Suisse A, He D, Legent K, et al. COP9 signalosome subunits protect Capicua from MAPK-dependent and -independent mechanisms of degradation. Development. 2017;144:2673–82. doi:10.1242/dev.148767
- Merlet J, Burger J, Gomes JE, et al. Regulation of cullin-RING E3 ubiquitin-ligases by neddylation and dimerization. Cell Mol Life Sci. 2009;66:1924–38. doi:10.1007/s00018-009-8712-7
- Boh BK, Smith PG, Hagen T. Neddylation-induced conformational control regulates cullin RING ligase activity in vivo. J Mol Biol. 2011;409:136–45. doi:10.1016/j.jmb.2011.03.023.
- Duda DM, Borg LA, Scott DC, et al. Structural insights into NEDD8 activation of cullin-RING ligases: conformational control of conjugation. Cell. 2008;134:995–1006. doi:10.1016/j.cell.2008.07.022
- Saha A, Deshaies RJ. Multimodal activation of the ubiquitin ligase SCF by Nedd8 conjugation. Mol Cell. 2008;32:21–31. doi:10.1016/j.molcel.2008.08.021
- Ou CY, Lin YF, Chen YJ, et al. Distinct protein degradation mechanisms mediated by Cul1 and Cul3 controlling Ci stability in Drosophila eye development. Genes Dev. 2002;16:2403–14. doi:10.1101/gad.1011402
- Nawrocki ST, Griffin P, Kelly KR, et al. MLN4924: a novel first-in-class inhibitor of NEDD8-activating enzyme for cancer therapy. Expert Opin Investig Drugs. 2012;21:1563–73. doi:10.1517/13543784.2012.707192
- Rozen S, Fuzesi-Levi MG, Ben-Nissan G, et al. CSNAP is a stoichiometric subunit of the COP9 signalosome. Cell Rep. 2015;13:585–98. doi:10.1016/j.celrep.2015.09.021
- Lingaraju GM, Bunker RD, Cavadini S, et al. Crystal structure of the human COP9 signalosome. Nature. 2014;512:161–5. doi:10.1038/nature13566
- Schwechheimer C, Serino G, Callis J, et al. Interactions of the COP9 signalosome with the E3 ubiquitin ligase SCFTIRI in mediating auxin response. Science. 2001;292:1379–82. doi:10.1126/science.1059776.
- Cope GA, Suh GS, Aravind L, et al. Role of predicted metalloprotease motif of Jab1/Csn5 in cleavage of Nedd8 from Cul1. Science. 2002;298:608–11. doi:10.1126/science.1075901
- Wei N, Deng XW. The COP9 signalosome. Annu Rev Cell Dev Biol. 2003;19:261–86. doi:10.1146/annurev.cellbio.19.111301.112449
- Zheng J, Yang X, Harrell JM, et al. CAND1 binds to unneddylated CUL1 and regulates the formation of SCF ubiquitin E3 ligase complex. Mol Cell. 2002;10:1519–26. doi:10.1016/S1097-2765(02)00784-0
- Liu J, Furukawa M, Matsumoto T, et al. NEDD8 modification of CUL1 dissociates p120(CAND1), an inhibitor of CUL1-SKP1 binding and SCF ligases. Mol Cell. 2002;10:1511–8. doi:10.1016/S1097-2765(02)00783-9
- Pierce NW, Lee JE, Liu X, et al. Cand1 promotes assembly of new SCF complexes through dynamic exchange of F box proteins. Cell. 2013;153:206–15. doi:10.1016/j.cell.2013.02.024
- Zemla A, Thomas Y, Kedziora S, et al. CSN- and CAND1-dependent remodelling of the budding yeast SCF complex. Nat Commun. 2013;4:1641. doi:10.1038/ncomms2628
- Wu S, Zhu W, Nhan T, et al. CAND1 controls in vivo dynamics of the cullin 1-RING ubiquitin ligase repertoire. Nat Commun. 2013;4:1642. doi:10.1038/ncomms2636
- Zhou C, Wee S, Rhee E, et al. Fission yeast COP9/signalosome suppresses cullin activity through recruitment of the deubiquitylating enzyme Ubp12p. Mol Cell. 2003;11:927–38. doi:10.1016/S1097-2765(03)00136-9
- Wu JT, Lin HC, Hu YC, et al. Neddylation and deneddylation regulate Cul1 and Cul3 protein accumulation. Nat Cell Biol. 2005;7:1014–20. doi:10.1038/ncb1301
- Wu J-T, Lin W-H, Chen W-Y, et al. CSN-mediated deneddylation differentially modulates Ci(155) proteolysis to promote Hedgehog signalling responses. Nat Commun. 2011;182. doi:10.1038/ncomms1185
- Harari-Steinberg O, Cantera R, Denti S, et al. COP9 signalosome subunit 5 (CSN5/Jab1) regulates the development of the Drosophila immune system: effects on Cactus, Dorsal and hematopoiesis. Genes Cells. 2007;12:183–95. doi:10.1111/j.1365-2443.2007.01049.x
- Knowles A, Koh K, Wu JT, et al. The COP9 signalosome is required for light-dependent Timeless degradation and Drosophila clock resetting. J Neurosci. 2009;29:1152–62. doi:10.1523/JNEUROSCI.0429-08.2009
- Doronkin S, Djagaeva I, Beckendorf SK. CSN5/Jab1 mutations affect axis formation in the Drosophila oocyte by activating a meiotic checkpoint. Development. 2002;129:5053–64.
- Enchev RI, Scott DC, da Fonseca PC, et al. Structural basis for a reciprocal regulation between SCF and CSN. Cell Rep. 2012;2:616–27. doi:10.1016/j.celrep.2012.08.019
- Harari-Steinberg O, Chamovitz DA. The COP9 signalosome: mediating between kinase signaling and protein degradation. Curr Protein Pept Sci. 2004;5:185–9. doi:10.2174/1389203043379792
- Chamovitz DA. Revisiting the COP9 signalosome as a transcriptional regulator. EMBO Rep. 2009;10:352–8. doi:10.1038/embor.2009.33
- Dubiel D, Rockel B, Naumann M, et al. Diversity of COP9 signalosome structures and functional consequences. FEBS Lett. 2015;589:2507–13. doi:10.1016/j.febslet.2015.06.007
- Pick E, Bramasole L. Moonlighting and pleiotropy within two regulators of the degradation machinery: the proteasome lid and the CSN. Biochem Soc Trans. 2014;42:1786–91. doi:10.1042/BST20140227
- Lee JH, Yi L, Li J, et al. Crystal structure and versatile functional roles of the COP9 signalosome subunit 1. Proc Natl Acad Sci U S A. 2013;110:11845–50. doi:10.1073/pnas.1302418110
- Cavadini S, Fischer ES, Bunker RD, et al. Cullin-RING ubiquitin E3 ligase regulation by the COP9 signalosome. Nature. 2016;531:598–603. doi:10.1038/nature17416
- Mosadeghi R, Reichermeier KM, Winkler M, et al. Structural and kinetic analysis of the COP9-Signalosome activation and the cullin-RING ubiquitin ligase deneddylation cycle. Elife. 2016;5:e12102. doi:10.7554/eLife.12102
- Pan L, Wang S, Lu T, et al. Protein competition switches the function of COP9 from self-renewal to differentiation. Nature. 2014;514:233–6. doi:10.1038/nature13562
- Chen J, Shin JH, Zhao R, et al. CSN6 drives carcinogenesis by positively regulating Myc stability. Nat Commun. 2014;5:5384. doi:10.1038/ncomms6384
- Dressel U, Thormeyer D, Altincicek B, et al. Alien, a highly conserved protein with characteristics of a corepressor for members of the nuclear hormone receptor superfamily. Mol Cell Biol. 1999;19:3383–94. doi:10.1128/MCB.19.5.3383
- Hong W, Li J, Wang B, et al. Epigenetic involvement of Alien/ESET complex in thyroid hormone-mediated repression of E2F1 gene expression and cell proliferation. Biochem Biophys Res Commun. 2011;415:650–5. doi:10.1016/j.bbrc.2011.10.130
- Zhang W, Ni P, Mou C, et al. Cops2 promotes pluripotency maintenance by stabilizing nanog protein and repressing transcription. Sci Rep. 2016;6:26804. doi:10.1038/srep26804
- Tsuge T, Matsui M, Wei N. The subunit 1 of the COP9 signalosome suppresses gene expression through its N-terminal domain and incorporates into the complex through the PCI domain. J Mol Biol. 2001;305:1–9. doi:10.1006/jmbi.2000.4288
- Menon S, Chi H, Zhang H, et al. COP9 signalosome subunit 8 is essential for peripheral T cell homeostasis and antigen receptor-induced entry into the cell cycle from quiescence. Nat Immunol. 2007;8:1236–45. doi:10.1038/ni1514.
- Ullah Z, Buckley MS, Arnosti DN, et al. Retinoblastoma protein regulation by the COP9 signalosome. Mol Biol Cell. 2007;18:1179–86. doi:10.1091/mbc.E06-09-0790
- Schweitzer K, Naumann M. CSN-associated USP48 confers stability to nuclear NF-kappaB/RelA by trimming K48-linked Ub-chains. Biochim Biophys Acta. 2015;1853:453–69. doi:10.1016/j.bbamcr.2014.11.028
- Huang X, Langelotz C, Hetfeld-Pechoc BKJ, et al. The COP9 signalosome mediates beta-catenin degradation by deneddylation and blocks adenomatous polyposis coli destruction via USP15. J Mol Biol. 2009;391:691–702. doi:10.1016/j.jmb.2009.06.066
- Schweitzer K, Bozko PM, Dubiel W, et al. CSN controls NF-kappaB by deubiquitinylation of IkappaBalpha. EMBO J. 2007;26:1532–41. doi:10.1038/sj.emboj.7601600
- Astigarraga S, Grossman R, Diaz-Delfin J, et al. A MAPK docking site is critical for downregulation of Capicua by Torso and EGFR RTK signaling. EMBO J. 2007;26:668–77. doi:10.1038/sj.emboj.7601532
- Coleman KE, Bekes M, Chapman JR, et al. SENP8 limits aberrant neddylation of NEDD8 pathway components to promote cullin-RING ubiquitin ligase function. Elife. 2017;6:e24325. doi:10.7554/eLife.24325
- Bekes M, Okamoto K, Crist SB, et al. DUB-resistant ubiquitin to survey ubiquitination switches in mammalian cells. Cell Rep. 2013;5:826–38. doi:10.1016/j.celrep.2013.10.008
- Wada H, Kito K, Caskey LS, et al. Cleavage of the C-terminus of NEDD8 by UCH-L3. Biochem Biophys Res Commun. 1998;251:688–92. doi:10.1006/bbrc.1998.9532
- Jiang J, Struhl G. Regulation of the Hedgehog and Wingless signalling pathways by the F-box/WD40-repeat protein Slimb. Nature. 1998;391:493–6. doi:10.1038/35154
- Fischer ES, Scrima A, Bohm K, et al. The molecular basis of CRL4DDB2/CSA ubiquitin ligase architecture, targeting, and activation. Cell. 2011;147:1024–39. doi:10.1016/j.cell.2011.10.035
- Liu Q, Zhou Y, Tang R, et al. Increasing the un-neddylated Cullin1 portion rescues the csn phenotypes by stabilizing adaptor modules to drive SCF assembly. Mol Cell Biol. 2017;37:e00109–17. doi:10.1128/MCB.00109-17.
- Chan Y, Yoon J, Wu JT, et al. DEN1 deneddylates non-cullin proteins in vivo. J Cell Sci. 2008;121:3218–23. doi:10.1242/jcs.030445
- Kim K, Yoon J, Yim J, et al. Deneddylase 1 regulates deneddylase activity of the Cop9 signalosome in Drosophila melanogaster. Insect Sci. 2015;24:27–34. doi:10.1111/1744-7917.12274
- Broemer M, Tenev T, Rigbolt KT, et al. Systematic in vivo RNAi analysis identifies IAPs as NEDD8-E3 ligases. Mol Cell. 2010;40:810–22. doi:10.1016/j.molcel.2010.11.011
- Bosu DR, Kipreos ET. Cullin-RING ubiquitin ligases: global regulation and activation cycles. Cell Div. 2008;3:7. doi:10.1186/1747-1028-3-7
- Gummlich L, Rabien A, Jung K, et al. Deregulation of the COP9 signalosome-cullin-RING ubiquitin-ligase pathway: mechanisms and roles in urological cancers. Int J Biochem Cell Biol. 2013;45:1327–37. doi:10.1016/j.biocel.2013.03.023
- Tanaka T, Nakatani T, Kamitani T. Negative regulation of NEDD8 conjugation pathway by novel molecules and agents for anticancer therapy. Curr Pharm Des. 2013;19:4131–9. doi:10.2174/1381612811319220017
- Zhou H, Lu J, Liu L, et al. A potent small-molecule inhibitor of the DCN1-UBC12 interaction that selectively blocks Cullin 3 neddylation. Nat Commun. 2017;8:1150. doi:10.1038/s41467-017-01243-7
- Schlierf A, Altmann E, Quancard J, et al. Targeted inhibition of the COP9 signalosome for treatment of cancer. Nat Commun. 2016;7:13166. doi:10.1038/ncomms13166
- Hazelett DJ, Bourouis M, Walldorf U, et al. decapentaplegic and wingless are regulated by eyes absent and eyegone and interact to direct the pattern of retinal differentiation in the eye disc. Development. 1998;125:3741–51.
- Motzny CK, Holmgren R. The Drosophila Cubitus interruptus protein and its role in the wingless and hedgehog signal transduction pathways. Mech Dev. 1995;52:137–50. doi:10.1016/0925-4773(95)00397-J