Abstract
This short review discusses pharmacological modulation of the opening/closing properties (gating) of small- and intermediate-conductance Ca2+-activated K+ channels (KCa2 and KCa3.1) with special focus on mechanisms-of-action, selectivity, binding sites, and therapeutic potentials. Despite KCa channel gating-modulation being a relatively novel field in drug discovery, efforts in this area have already revealed a surprising plethora of pharmacological sites-of-actions and channel subtype selectivity exerted by different chemical classes. The currently published positive modulators show that such molecules are potentially useful for the treatment of various neurodegenerative disorders such as ataxia, alcohol dependence, and epilepsy as well as hypertension. The negative KCa2 modulators are very effective agents for atrial fibrillation. The prediction is that further unraveling of the molecular details of gating pharmacology will allow for the design of even more potent and subtype selective KCa modulators entering into drug development for these indications.
Abbreviations
KCa2.x | = | small-conductance Ca2+-activated K+ channel |
KCa3.1 | = | intermediate-conductance Ca2+-activated K+ channel |
ALS | = | amyotropic lateral sclerosis |
Cam | = | calmodulin |
CamBD | = | calmodulin binding domain |
ICARS | = | International Cooperative Ataxia Rating Scale |
KCNN | = | potassium channel gene class N. |
Pharmacological Modulation of Ion Channel Gating
In the current context we define pharmacological modulation of ion channels as modulation of their activation, inactivation or deactivating mechanisms. In other words, gating modulation. Thus, we will not discuss trafficking modulation, which alters channel density on the plasma membrane or classical pore blockers, which simply obstruct the flow of ions through an open channel.Citation1 We will also not consider compounds operating at orthosteric sites (agonists), like endogeneous neurotransmitters on ionotropic receptors. This article will instead focus on allosteric modulation that strengthens (positive modulator) or weakens (negative modulator) the response to the physiological stimuli for channel activation.
Pharmacological gating modulation is historically a well-established principle in drug development. The most famous example is probably the modulation of GABAA receptors by benzodiazepines: Drugs like diazepam bind to an interface pocket between the α and γ subunits and increase the affinity for activation by GABA, an effect which strengthens inhibitory signaling in the brain and is valuable in the treatment of anxiety and seizure disorders. Importantly, the efficacy of different benzodiazepines at this binding site varies from full to partial positive modulators and comprises compounds with no efficacy, and even compounds exerting negative gating modulation.Citation2,3 Another well-known example is the dihydropyridine binding site on L-type Ca2+ channels (Cav1.x): Nifedipine and amlodipine exert complex modulation of voltage-dependent gating parameters resulting in overall decreased Ca2+-currents at physiological membrane potentials.Citation4 These negative gating modulators are valuable drugs for the treatment of essential hypertension by their relaxing effect on vascular smooth muscles. However, other dihydropyridines like Bay-K-8644 act as positive gating modulators via the same binding site by primarily shifting the voltage activation curve toward more negative membrane potentials.Citation5,6
In the following paragraphs we will review the current understanding of positive and negative gating modulation of Ca2+-activated K+ channels of small- and intermediate-conductance, KCa2.x and KCa3.1 (the KCNN channels), which is an emerging field in drug discovery.
Small/Intermediate Conductance Ca2+-Activated K+ Channels
Four KCNN genes exist in mammals.Citation7 KCNN1, KCNN2 and KCNN3 encode the small-conductance Ca2+-activated K+ channels KCa2.1, KCa2.2 and KCa2.3 (a.k.a. SK1, SK2 and SK3), which are prominently expressed in neurons but also found in some peripheral tissues such as cardiomyocytes and liver.Citation8 KCNN4 encodes the intermediate-conductance KCa3.1 (IK, SK4) channel, which is nearly exclusively expressed on non-excitable cells such lymphocytes, erythrocytes, fibroblasts, vascular endothelium and secretory epithelia.Citation9 However, this notion was recently challenged by a paper finding evidence for KCa3.1 expression in hippocampal CA1 neurons, where the channel apparently contributes to the slow afterhyperpolarization.Citation10 All KCNN channels share a common mode-of-activation in that their gating is voltage-independent and depends exclusively on calmodulin (Cam), which is tightly bound to a Cam binding domain (CamBD) in the C-terminus of the channel ().Citation11-15 Upon Ca2+ binding to Cam the channels activate in a highly coordinated fashion following an extremely steep Hill-equation for KCa2 (and a slightly less steep curve for KCa3.1) and with EC50 values estimated by various groups to be in the range of 250 to 900 nM.Citation14,16,17 The Ca2+ sensitivities of KCNN channels are physiologically modulated by closely associated kinases and phosphatases: For KCa2 channels casein kinase 2 (CK2) and protein phosphatase 2A (PP2A) mediate phosphorylation/dephosphorylation of Cam threonine 80, with the phosphorylated state being less Ca2+ sensitive ().Citation8 For KCa3.1 the nucleoside diphosphate kinase B phosphorylates histidine 358 in the C-terminal, which is permissive for Ca2+ activation.Citation18 KCa2 channels expressed in the soma of neurons are functionally coupled to voltage-activated Ca2+ channels (Cav channels) and give rise to Ca2+-dependent afterhyperpolarizations of medium duration (mAHP) following single or trains of action potentials, which is an essential component of early spike frequency modulation in cortical and limbic neurons.Citation19 In pacemaker neurons of the basal ganglia and cerebellum, cyclic activation of KCa2 channels secures highly regular firing, which is essential for muscle coordination and movement.Citation20,21 KCa2 channels expressed postsynaptically in dendritic spines are coupled to NMDA (GluN1-3) receptors and their activation hyperpolarizes the spine and accelerates voltage-dependent Mg2+ block of the NMDA receptor.Citation22 Thus, dendritic KCa2 channels are important components of memory and learning processes at the subcellular level.Citation23-25 In contrast, KCa3.1 channels in endothelial cells and cells of the immune system, such as T-lymphocytes, are activated by more global Ca2+ signals like Ca2+ released from intracellular stores or by the refilling influx through store operated Ca2+ channels like transient receptor potential channels (TRPs) and Ca2+ release activated channels (CRAC).Citation26,27 KCa3.1 is a major component of the endothelial derived hyperpolarizing (EDH) responseCitation28,29 and important for cell proliferation and cytokine release in lymphocytes.Citation27,30,31
Positive Modulation of KCa2/KCa3.1 Channels
Key compounds and drugs
It can be argued that the oldest positive modulator of KCNN channels is 1-EBIO (), which was identified before the channels were cloned.Citation32 1-EBIO activates all 4 KCNN channels. With an EC50 of ∼30 μM KCa3.1 is most sensitive, while the three KCa2 channels are activated at roughly 5–10-fold higher concentrations.Citation33-35 However, 1-EBIO is a tool compound for research purposes only. A pivotal finding for the field therefore was the demonstration that chlorzoxazone and zoxazolamine, centrally acting muscle relaxants used for many years to treat spasticity, also activated KCNN channels.Citation36 Another compound, riluzole, the only drug FDA approved for treatment of amyotropic lateral sclerosis (ALS), was shown to activate KCa3.1/KCa2.x channels even more potently than 1-EBIO.Citation37 Despite having a very “rich” ion channel pharmacology, including block of voltage-dependent Na+ (Nav) channels (but not glutamate receptors even though its therapeutic action is often referred to as being anti-glutamatergic), the activation of KCNN channels is among riluzole's most potent effects.Citation38 Two molecules also belonging to the benzimidazole/benzothiazole prototype KCa activator class are NS309, a very potent molecule (EC50 10–20 nM for KCa3.1 and ∼600 nM for KCa2 channels) and very useful for in vitro mechanistic work, and SKA-31, which was optimized from riluzole with the aim of getting better selectivity (inactive on Nav channels) and maintaining good in vivo properties.Citation38,39 The very close analogs, SKA-111 and SKA-121, exhibit much higher KCa3.1/KCa2 selectivity, and accentuate the primary role of the benzimidazole/benzothiazole series as being KCa3.1 activators.Citation40
Figure 2. Top, chemical structures of positive and negative gating modulators. Bottom, schematic of effect of gating modulators on the channel Ca2+ concentration response curve.
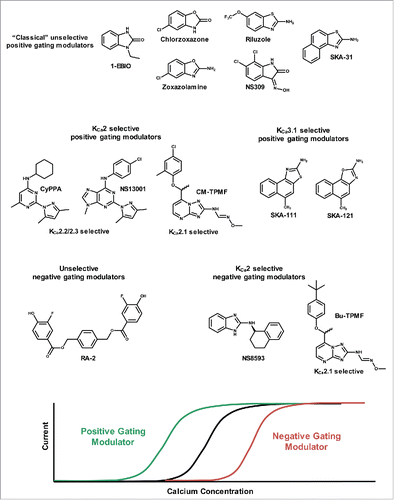
In contrast to the above mentioned molecules, which show little selectivity between the KCa2 family members, CyPPA and its more potent congener NS13001 have fundamentally different structures and also changed selectivity profiles (). Both compounds selectively activate KCa2.3 and KCa2.2 but are completely inactive on KCa2.1 and KCa3.1.Citation16,41 Still other lines of molecules are represented by GW542573X and CM-TPMF, which are selective and potent (CM-TPMF) activators of the human KCa2.1 channel.Citation42,43
Mode(s) of action
Basically, all KCNN activators conform with our definition of a positive gating modulator, since they act by shifting the Ca2+-activation curve toward lower concentrations of Ca2+ in a concentration-dependent way ( bottom). This means that the determined EC50 values for Ca2+, calculated from Ca2+-concentration response curves, decreases as the concentration of modulator increases. Using fast application of different intracellular Ca2+ concentrations to inside-out patches, it has furthermore been shown that 1-EBIO has no effect on activation time constant, whereas it strongly reduces the deactivation constant.Citation44 Applied to a physiological situation this means that KCa2 channels will not activate quicker or to a significantly higher degree during a fast neuronal action potential, but they will stay open longer after the action potential and local Ca2+ transient has ceased. This is exactly what is observed, since positive modulators prolong the mAHP, thereby increasing the time to the firing of the next action potential.Citation34,45 Given these functional data it is therefore an obvious and tempting interpretation that the mode-of-action of positive modulators simply is to decrease the Kd value for Ca2+ binding to Cam by decreasing the koff-value for Ca2+. Intriguingly, however, this is not exactly what happens! Studies of Cam mutants, which make unstable association with the CamBD, have shown that NS309 is able to stabilize the interaction, which points toward an action of NS309 in the transformation of conformational changes between the Cam and the channel.Citation46 Direct measurements of Ca2+ off-rates have confirmed that conclusion.
Site(s) of action
A chimaeric construct where the entire C-terminus () was transferred from KCa3.1 to KCa2.2 demonstrated that the higher 1-EBIO potency on KCa3.1 followed the C-terminus, suggesting that this domain (probably the CamBD) contains the binding site.Citation44 We were able to confirm and refine that conclusion for the more selective CyPPA by a mutation program with KCa2.3 (active) and KCa2.1 (inactive), whereas the equivalent program for the KCa2.1-selective molecules, CM-TPMF and GW542573X, gave the highly surprising result that their sites-of-action resided in transmembrane domain 5 in the pore.Citation42,43 Recently, crystal structures for Cam/CamBD with bound 1-EBIO and NS309, respectively, have been solved.Citation47,48 Both molecules reside in a pocket formed at the interface between Cam/CamBD (), but have different coordination sites on Cam and CamBD. Interestingly, NS309 interacts with an internally disorganized stretch of amino acids (called IDF, shown in red in ), which was not visible in the 1-EBIO crystal. This interaction was speculated to explain the disproportionately high affinity of NS309 compared to other structures in the prototype series. (In this context it is highly interesting that the regulatory membrane lipid, PIP2, which recently has been shown to be an obligatory co-agonist to Ca2+, also binds with high affinity in this region, and also interacts with residues on both Cam and the IDF.Citation49 Detailed analyses are warranted to clarify whether NS309 possibly can substitute for PIP2 or whether it increases its affinity). Based on docking and mutations directed by the crystal structure, it was made plausible that CyPPA binds at the same or an overlapping site as NS309 and 1-EBIO. However, the crystal structure unfortunately did not give any hints as to why CyPPA is completely inactive on KCa2.1. To answer this question CyPPA, or a closely related analog, probably needs to be co-crystallized with the KCa2.2 (or KCa2.3) CamBD and Cam.
Therapeutic indications for positive modulators of KCa2 channels
KCa2 channel expression and/or function has been reported to be down-regulated in limbic brain regions following induction of alcohol dependence and in the hippocampus following pilocarpine induced status epilepticus, suggesting that positive KCa2 channel modulation might be particularly useful for situations where physiological KCa2 channel function has been perturbed.Citation50-53 More generally, due to their ability to prolong the mAHP by increasing the apparent Ca2+ sensitivity of KCa2 channels, positive gating modulators may also have an important role to play in the treatment of neurological diseases characterized by hyperactivity or disorganized firing. An illustrative example is episodic ataxia (EA2) caused by a partial loss-of-function of P/Q type Ca2+ channels (Cav2.1), which make cerebellar Purkinje cell firing irregular due to inefficient cyclic activation of KCa2 channels by action potentials. Local application of 1-EBIO to the cerebellum or systemic administration of chlorzoxazone both reestablished regular pacemaking activity and partially counteracted ataxia.Citation54,55 While this effect is straightforward to rationalize from the mode-of-action of positive modulators, it is less clear why CyPPA, NS13001, and SKA-31 exert positive effects in rodent models of spinocerebellar ataxias (SCA2 and SCA3), which are due to gain-of-function toxicity caused by glutamine prolongation of ataxins.Citation41,56 One possibility is that diseased Purkinje cells - maybe independently of the molecular cause - go through phases where the highly regulated pacemaker firing is lost, for example through changes in climbing fiber input or denervation,Citation57,58 leading to excessive endogeneous burst firing, depolarization block, and Ca2+ overload, which may contribute to accelerated cell death.
Positive modulators of KCa2 channels might terminate bursting by reintroducing mAHPs, reactivate depolarization blocked cells by hyperpolarization, and possibly prevent or delay Purkinje cell degeneration by relieving their metabolic burden. Recently, supportive information for a broader use in ataxia diseases has been obtained from a few smaller clinical trials. Riluzole dosed at 50 mg/kg/day b.i.d. (the treatment regimen used for ALS patients) for 8 weeks was shown to improve symptoms measured by the ICARS scale in ataxia patients of mixed etiology.Citation59 Furthermore, chlorzoxazone specifically improved downbeat nystagmus, an involuntary vertical eye movement, a devastating symptom affecting many ataxia patients.Citation60
A new putative indication for KCa2 positive modulators may be pain disorders. A recent study showed that KCa2 activation with intrathecally administered NS309 can potentiate the analgesic effects of NMDA antagonists in rat pain models.Citation61 This effect may be interpreted in the light of the tight functional coupling between NMDA receptors and KCa2.2 channels (see above) in postsynaptic dendritic spines in pain pathways.
Indications for positive modulators of KCa3.1 channels
Although KCa2 and KCa3.1 channels are usually not present in the same tissues, one place where KCa2.3 and KCa3.1 are co-expressed is the vascular endothelium, where both channels are involved in the endothelium-derived hyperpolarization (EDH) response which regulates the contractile state of the underlying smooth muscle cell layer.Citation62,63 Mice deficient in KCa3.1 and/or KCa2.3 exhibit impaired EDH responses and show a ˜10 mmHg increase in mean arterial blood pressure,Citation28,29 while SKA-31 lowers blood pressure in normotensive and hypertensive mice as well as in conscious, normotensive dogs.Citation29,38,64 Since the blood pressure lowering effect of SKA-31 is absent in KCa3.1−/− mice and higher doses of SKA-31 induce sedation and lower heart rate through central KCa2 channel activation,Citation65 it seems desirable to identify KCa3.1 selective positive gating modulators in order to help investigate whether such compounds could be developed into a new class of endothelial targeted antihypertensives.Citation63 This objective recently seems to have been achieved with the demonstration that the KCa3.1 selective SKA-121 lowers blood pressure in normotensive and hypertensive mice without affecting heart rate.Citation40 However, SKA-121 has a short half-life (at least in rodents) and therefore does not constitute an ideal candidate compound for development.
From a therapeutic perspective an argument against positive gating modulators might be that their continued presence can lead to down-regulation of channel expression, thereby preventing effective treatment in long term dosing regimens. This is a well-founded concern based on the known plasticity of ion channel expression in many situations. Furthermore, it has been observed that classical positive KCNN modulators like 1-EBIO, NS309, and SKA-31 can reduce the expression of KCa3.1 in proliferative vascular smooth muscle cells as well as KCa3.1 blockers or KCa3.1 silencing.Citation66,67 However, the phenomenon has not been reported for KCa2 channels in terminally differentiated cells like neurons and cardiomyocytes, and not described for KCa3.1 and KCa2.3 in vascular endothelium.
Negative Modulation of KCa2 Channels
Key compounds
Negative gating modulation of KCa2 channels is a relatively new concept, in contrast to the rich literature on peptide and small molecule blockers of both KCa2.x and KCa3.1.Citation35 (It should be noted that recent work shows that apamin, the prototypic “blocker” of KCa2.x channels, actually acts by an allosteric mechanism collapsing the outer pore.Citation68,69 However, this is independent of gating, and therefore apamin is not counted as a negative modulator in this context). Detailed mechanistic data are mainly available for the compound that defined the concept, NS8593 (and its closest analogs), which inhibits cloned human and rat isoforms of KCa2 with equal potency, while it is inactive on KCa3.1.Citation70,71 NS8593 () is a non-charged molecule with a very different structure than the small molecule blockers (“apamin mimics”).Citation72,73 Recently, the negative modulator field has been enriched with Bu-TPMF, a KCa2.1 selective negative modulator,Citation42 and with a fluoro-trivanillic ester compound (RA-2), which inhibits both KCa3.1 and KCa2.3 with low nM potencies.Citation74,75
Mode of action
Like all negative-gating modulators NS8593 causes a concentration-dependent shift of the Ca2+-activation curve toward higher Ca2+ concentrations ( bottom). In practical terms, if NS8593 is applied at a relatively low Ca2+ concentration (low degree of channel activation) the inhibitor potency appears high, while it is essentially without effect at higher Ca2+ concentrations (high degree of channel activation).Citation70,76 The distinction between the Ca2+ concentration per se and the degree of activation is essential here, since the effect of NS8593 also disappear at a high degree of channel activation obtained by a combination of a low Ca2+-concentration and a positive modulator like NS309 or SKA-31.Citation45,70,76
Site(s) of action
In contrast to small molecule blockers, NS8593 does not displace apamin in binding studies, and the compound remains active on a channel made apamin-insensitive by specific mutations in the outer pore mouth.Citation71 Another difference is that NS8593 is able to reach its binding site both when applied from the outside and from the inside of the membrane.Citation70 Since the inhibition by NS8593 can easily be “reversed” by positive allosteric modulators and since the right shifting of the Ca2+-activation curve resembles the effect of the physiological negative gating modulation described above, we were initially convinced that the site of action would be at the Cam/CamBD region or maybe at Cam itself. This proved not to be the case! NS8593 interacts deeply in the inner vestibule of the pore, at a site defined by just 2 amino acids, serine 507 and alanine 532 (KCa2.3) positioned close to the inner side of the K+ selectivity filter ().Citation76 Since the physical gate of KCNN channels has - by independent measures - been located at a “deep” position in the channel,Citation77 we speculate that the mechanism behind the negative gating modulation of NS8593, may be due to its interaction with the gate itself. A crystal with bound NS8593 would be very interesting! It is noteworthy that KCa3.1 inhibitors like TRAM-34 act at the equivalently positioned residues in KCa3.1, which questions whether these also act by “shutting the gate” or - as hitherto assumed - by “blocking ion flow.”Citation78 However, the inhibition by TRAM-34 shows no clear dependence on intracellular Ca2+ (or degree of activation) and the effect cannot be “reversed” by positive modulators.Citation76
In contrast to NS8593, Bu-TPMF, the KCa2.1 selective negative gating modulator, interacts with a site lower down in the inner pore vestibule in TM5 (). This site is identical to the site-of-action of the KCa2.1 selective positive modulators, CM-TPMF and GW542573X ().Citation42 The binding site of the fluro-di-benzoate RA-2 is currently unknown,Citation75 but based on the molecules large size and different structure, is not likely to be as high up in the inner pore as NS8593.
Indications for negative gating modulators of KCa2 channels
Much evidence indicates that blocking KCa2 channels by apamin improves learning processes, which is easily comprehended by the close functional association between KCa2.2 and NMDA receptors, as demonstrated in the hippocampus.Citation8 Furthermore, inhibition of KCa2.3 by NS8593 in dopaminergic neurons leads to increased bursting both ex vivo and in vivo, which may influence the balance between tonic and phasic dopaminergic signaling and constitute a principle for treatment of various psychiatric conditions.Citation45,79 However, the therapeutic exploitation for CNS diseases is hitherto hampered by the low therapeutic index toward tremors, ataxia and convulsions, probably induced by Kca2.2 inhibition.
The currently most promising use of negative gating modulators is as atrial selective cardiac anti-arrhythmics.Citation80 NS8593 as well as several small molecule blockers of KCa2 channels have shown efficacy in protecting against and terminating atrial fibrillation both ex vivo and in vivo across a number of different species.Citation81 Intriguingly, this concept also appears to be viable after hypertension induced cardiac remodeling.Citation82
Conclusion
Despite the strong precedence of pharmacological modulation of ion channel gating in the history of drug discovery provided by GABAA receptor modulators, it is an often heard argument that ion channel modulation is too “messy” a principle for rational drug discovery compared to agonist/antagonists or blockers. These reservations may be founded in therapeutic considerations (such as tolerance development as discussed for positive modulators), but we suspect that the attitude is often founded on operational screening considerations, rather than an analysis of the need for specific mode of actions for treatment of various diseases. It is certainly true that the need for a well-defined partial activation of ion channels - a general prerequisite for modulator screens - represents a challenge for quantification, but with the increasingly refined methods for medium-to-high throughput electrophysiology and spectroscopic methods these challenges can usually be handled.Citation83-85 The appearance of both positive and negative gating modulators of KCNN channels from HTS screening programs and classical medicinal chemistry efforts as described in the foregoing paragraphs shows that this is certainly possible. Furthermore, the emerging diversity in terms of mode-of-actions and subtype selectivities suggest to us that the field of KCNN gating modulators offers exciting therapeutic possibilities that currently are only beginning to be realized.
Disclosure of Potential Conflicts of Interest
Palle Christophersen is a full time employee of Saniona A/S. Heike Wulff is named as inventor on a University of California patent claiming SKA-121 and related compounds as antihypertensives.
Acknowledgment
The authors wish to thank Vladimir Varov-Yarovoy in the Department of Physiology & Membrane Biology, University of California, Davis for help with .
Funding
Heike Wulff was supported by the CounterACT Program, National Institutes of Health Office of the Director (NIH OD), and the National Institute of Neurological Disorders and Stroke (NINDS), Grant Numbers U54NS079202 and R21NS072585.
References
- Balut CM, Hamilton KL, Devor DC. Trafficking of intermediate (KCa3.1) and small (KCa2.x) conductance, Ca2+-activated K+ channels: a novel target for medicinal chemistry efforts? Chem Med Chem 2012; 7:1741-55; PMID:22887933; http://dx.doi.org/10.1002/cmdc.201200226
- Changeux JP. The concept of allosteric modulation: an overview. Drug Discov Today Technol 2013; 10:e223-8; PMID:24050272; http://dx.doi.org/10.1016/j.ddtec.2012.07.007
- Sieghart W. Pharmacology of benzodiazepine receptors: an update. J Psychiatry Neurosci 1994; 19:24-9; PMID:8148363
- Wang RX, Jiang WP. Changes of action potential and L-type calcium channel current of Sprague-Dawley rat ventricular myocytes by different amlodipine isomers. Can J Physiol Pharmacol 2008; 86:620-5; PMID:18758511; http://dx.doi.org/10.1139/Y08-065
- Greenberg DA, Cooper EC, Carpenter CL. Calcium channel ‘agonist’ BAY K 8644 inhibits calcium antagonist binding to brain and PC12 cell membranes. Brain Res 1984; 305:365-8; PMID:6204725; http://dx.doi.org/10.1016/0006-8993(84)90444-X
- Schramm M, Thomas G, Towart R, Franckowiak G. Novel dihydropyridines with positive inotropic action through activation of Ca2+ channels. Nature 1983; 303:535-7; PMID:6190088; http://dx.doi.org/10.1038/303535a0
- Wei AD, Gutman GA, Aldrich R, Chandy KG, Grissmer S, Wulff H. International Union of Pharmacology. LII. Nomenclature and molecular relationships of calcium-activated potassium channels. Pharmacol Rev 2005; 57:463-72; PMID:16382103; http://dx.doi.org/10.1124/pr.57.4.9
- Adelman JP, Maylie J, Sah P. Small-conductance Ca2+-activated K+ channels: form and function. Annu Rev Physiol 2012; 74:245-69; PMID:21942705; http://dx.doi.org/10.1146/annurev-physiol-020911-153336
- Wulff H, Castle NA. Therapeutic potential of KCa3.1 blockers: recent advances and promising trends. Expert Rev Clin Pharmacol 2010; 3:385-96; PMID:22111618; http://dx.doi.org/10.1586/ecp.10.11
- King B, Rizwan AP, Asmara H, Heath NC, Engbers JD, Dykstra S, et al. IKCa channels are a critical determinant of the slow AHP in CA1 pyramidal neurons. Cell Rep 2015; 11:175-82; PMID:25865881; http://dx.doi.org/10.1016/j.celrep.2015.03.026
- Adelman JP. SK channels and calmodulin. Channels (Austin) 2015:1-6; PMID:25942650; http://dx.doi.org/10.1080/19336950.2015.1029688
- Schumacher MA, Rivard AF, Bachinger HP, Adelman JP. Structure of the gating domain of a Ca2+-activated K+ channel complexed with Ca2+/calmodulin. Nature 2001; 410:1120-4; PMID:11323678; http://dx.doi.org/10.1038/35074145
- Joiner WJ, Khanna R, Schlichter LC, Kaczmarek LK. Calmodulin regulates assembly and trafficking of SK4/IK1 Ca2+-activated K+ channels. J Biol Chem 2001; 276:37980-5; PMID:11495911
- Xia XM, Fakler B, Rivard A, Wayman G, Johnson-Pais T, Keen JE, Ishii T, Hirschberg B, Bond CT, Lutsenko S, et al. Mechanism of calcium gating in small-conductance calcium-activated potassium channels. Nature 1998; 395:503-7; PMID:9774106; http://dx.doi.org/10.1038/26758
- Fanger CM, Ghanshani S, Logsdon NJ, Rauer H, Kalman K, Zhou J, Beckingham K, Chandy KG, Cahalan MD, Aiyar J. Calmodulin mediates calcium-dependent activation of the intermediate conductance KCa channel, IKCa1. J Biol Chem 1999; 274:5746-54; PMID:10026195; http://dx.doi.org/10.1074/jbc.274.9.5746
- Hougaard C, Eriksen BL, Jorgensen S, Johansen TH, Dyhring T, Madsen LS, Strøbaek D, Christophersen P. Selective positive modulation of the SK3 and SK2 subtypes of small conductance Ca2+-activated K+ channels. Br J Pharmacol 2007; 151:655-65; PMID:17486140; http://dx.doi.org/10.1038/sj.bjp.0707281
- Ishii TM, Silvia C, Hirschberg B, Bond CT, Adelman JP, Maylie J. A human intermediate conductance calcium-activated potassium channel. Proc Natl Acad Sci USA 1997; 94:11651-6; PMID:9326665; http://dx.doi.org/10.1073/pnas.94.21.11651
- Srivastava S, Li Z, Ko K, Choudhury P, Albaqumi M, Johnson AK, Yan Y, Backer JM, Unutmaz D, Coetzee WA, et al. Histidine phosphorylation of the potassium channel KCa3.1 by nucleoside diphosphate kinase B is required for activation of KCa3.1 and CD4 T cells. Mol Cell 2006; 24:665-75; PMID:17157250; http://dx.doi.org/10.1016/j.molcel.2006.11.012
- Stocker M, Krause M, Pedarzani P. An apamin-sensitive Ca2+-activated K+ current in hippocampal pyramidal neurons. Proc Natl Acad Sci USA 1999; 96:4662-7; PMID:10200319; http://dx.doi.org/10.1073/pnas.96.8.4662
- Wolfart J, Roeper J. Selective coupling of T-type calcium channels to SK potassium channels prevents intrinsic bursting in dopaminergic midbrain neurons. J Neurosci 2002; 22:3404-13; PMID:11978817
- Womack MD, Khodakhah K. Somatic and dendritic small-conductance calcium-activated potassium channels regulate the output of cerebellar Purkinje neurons. J Neurosci 2003; 23:2600-7; PMID:12684445
- Ngo-Anh TJ, Bloodgood BL, Lin M, Sabatini BL, Maylie J, Adelman JP. SK channels and NMDA receptors form a Ca2+-mediated feedback loop in dendritic spines. Nat Neurosci 2005; 8:642-9; PMID:15852011; http://dx.doi.org/10.1038/nn1449
- McKay BM, Oh MM, Galvez R, Burgdorf J, Kroes RA, Weiss C, Adelman JP, Moskal JR, Disterhoft JF. Increasing SK2 channel activity impairs associative learning. J Neurophysiol 2012; 108:863-70; PMID:22552186; http://dx.doi.org/10.1152/jn.00025.2012
- Vick KAT, Guidi M, Stackman RW, Jr. In vivo pharmacological manipulation of small conductance Ca2+-activated K+ channels influences motor behavior, object memory and fear conditioning. Neuropharmacology 2010; 58:650-9; PMID:AMBIGUOUS; http://dx.doi.org/10.1016/j.neuropharm.2009.11.008
- van der Staay FJ, Fanelli RJ, Blokland A, Schmidt BH. Behavioral effects of apamin, a selective inhibitor of the SK(Ca)-channel, in mice and rats. Neurosci Biobehav Rev 1999; 23:1087-110; PMID:10643819; http://dx.doi.org/10.1016/S0149-7634(99)00043-3
- Cahalan MD, Chandy KG. The functional network of ion channels in T lymphocytes. Immunol Rev 2009; 231:59-87; PMID:19754890; http://dx.doi.org/10.1111/j.1600-065X.2009.00816.x
- Feske S, Wulff H, Skolnik EY. Ion channels in innate and adaptive immunity. Annu Rev Immunol 2015; 33:291-353; PMID:25861976; http://dx.doi.org/10.1146/annurev-immunol-032414-112212
- Si H, Heyken WT, Wolfle SE, Tysiac M, Schubert R, Grgic I, Vilianovich L, Giebing G, Maier T, Gross V, et al. Impaired endothelium-derived hyperpolarizing factor-mediated dilations and increased blood pressure in mice deficient of the intermediate-conductance Ca2+-activated K+ channel. Circ Res 2006; 99:537-44; PMID:16873714; http://dx.doi.org/10.1161/01.RES.0000238377.08219.0c
- Brahler S, Kaistha A, Schmidt VJ, Wolfle SE, Busch C, Kaistha BP, Kacik M, Hasenau AL, Grgic I, Si H, et al. Genetic deficit of SK3 and IK1 channels disrupts the endothelium-derived hyperpolarizing factor vasodilator pathway and causes hypertension. Circulation 2009; 119:2323-32; PMID:19380617; http://dx.doi.org/10.1161/CIRCULATIONAHA.108.846634
- Ghanshani S, Wulff H, Miller MJ, Rohm H, Neben A, Gutman GA, Cahalan MD, Chandy KG. Up-regulation of the IKCa1 potassium channel during T-cell activation. Molecular mechanism and functional consequences. J Biol Chem 2000; 275:37137-49; PMID:10961988; http://dx.doi.org/10.1074/jbc.M003941200
- Di L, Srivastava S, Zhdanova O, Ding Y, Li Z, Wulff H, Lafaille M, Skolnik EY. Inhibition of the K+ channel KCa3.1 ameliorates T cell-mediated colitis. Proc Natl Acad Sci USA 2010; 107:1541-6; PMID:20080610; http://dx.doi.org/10.1073/pnas.0910133107
- Devor DC, Singh AK, Frizzell RA, Bridges RJ. Modulation of Cl- secretion by benzimidazolones. I. Direct activation of a Ca2+-dependent K+ channel. Am J Physiol 1996; 271:L775-84; PMID:8944721
- Jensen BS, Strobaek D, Christophersen P, Jorgensen TD, Hansen C, Silahtaroglu A, Olesen SP, Ahring PK. Characterization of the cloned human intermediate-conductance Ca2+-activated K+ channel. Am J Physiol 1998; 275:C848-56; PMID:9730970
- Pedarzani P, McCutcheon JE, Rogge G, Jensen BS, Christophersen P, Hougaard C, Strøbaek D, Stocker M. Specific enhancement of SK channel activity selectively potentiates the afterhyperpolarizing current I(AHP) and modulates the firing properties of hippocampal pyramidal neurons. J Biol Chem 2005; 280:41404-11; PMID:16239218; http://dx.doi.org/10.1074/jbc.M509610200
- Wulff H, Kolski-Andreaco A, Sankaranarayanan A, Sabatier JM, Shakkottai V. Modulators of small- and intermediate-conductance calcium-activated potassium channels and their therapeutic indications. Cur Med Chem 2007; 14:1437-57; PMID:17584055; http://dx.doi.org/10.2174/092986707780831186
- Cao Y, Dreixler JC, Roizen JD, Roberts MT, Houamed KM. Modulation of recombinant small-conductance Ca2+-activated K+ channels by the muscle relaxant chlorzoxazone and structurally related compounds. J Pharmacol Exp Ther 2001; 296:683-9; PMID:11181893
- Cao YJ, Dreixler JC, Couey JJ, Houamed KM. Modulation of recombinant and native neuronal SK channels by the neuroprotective drug riluzole. Eur J Pharmacol 2002; 449:47-54; PMID:12163105; http://dx.doi.org/10.1016/S0014-2999(02)01987-8
- Sankaranarayanan A, Raman G, Busch C, Schultz T, Zimin PI, Hoyer J, Köhler R, Wulff H. Naphtho[1,2-d]thiazol-2-ylamine (SKA-31), a new activator of KCa2 and KCa3.1 potassium channels, potentiates the endothelium-derived hyperpolarizing factor response and lowers blood pressure. Mol Pharmacol 2009; 75:281-95; PMID:18955585; http://dx.doi.org/10.1124/mol.108.051425
- Strøbaek D, Teuber L, Jørgensen TD, Ahring PK, Kjaer K, Hansen RS, Olesen SP, Christophersen P, Skaaning-Jensen B. Activation of human IK and SK Ca2+ -activated K+ channels by NS309 (6,7-dichloro-1H-indole-2,3-dione 3-oxime). Biochim Biophys Acta 2004; 1665:1-5; PMID:15471565; http://dx.doi.org/10.1016/j.bbamem.2004.07.006
- Coleman N, Brown BM, Oliván-Viguera A, Singh V, Olmstead MM, Valero MS, Köhler R, Wulff H. New positive Ca2+-activated K+ channel gating modulators with selectivity for KCa3.1. Mol Pharmacol 2014; 86:342-57; PMID:24958817; http://dx.doi.org/10.1124/mol.114.093286
- Kasumu AW, Hougaard C, Rode F, Jacobsen TA, Sabatier JM, Eriksen BL, Strøbæk D, Liang X, Egorova P, Vorontsova D, et al. Selective positive modulator of calcium-activated potassium channels exerts beneficial effects in a mouse model of spinocerebellar ataxia type 2. Chem Biol 2012; 19:1340-53; PMID:23102227; http://dx.doi.org/10.1016/j.chembiol.2012.07.013
- Hougaard C1, Hammami S, Eriksen BL, Sørensen US, Jensen ML, Strøbæk D, Christophersen P. Evidence for a common pharmacological interaction site on K(Ca)2 channels providing both selective activation and selective inhibition of the human K(Ca)2.1 subtype. Mol Pharmacol 2012; 81:210-9; PMID:22046005; http://dx.doi.org/10.1124/mol.111.074252
- Hougaard C1, Jensen ML, Dale TJ, Miller DD, Davies DJ, Eriksen BL, Strøbaek D, Trezise DJ, Christophersen P. Selective activation of the SK1 subtype of human small-conductance Ca2+-activated K+ channels by 4-(2-methoxyphenylcarbamoyloxymethyl)-piperidine-1-carboxylic acid tert-butyl ester (GW542573X) is dependent on serine 293 in the S5 segment. Mol Pharmacol 2009; 76:569-78; PMID:19515965; http://dx.doi.org/10.1124/mol.109.056663
- Pedarzani P, Mosbacher J, Rivard A, Cingolani LA, Oliver D, Stocker M, Adelman JP, Fakler B. Control of electrical activity in central neurons by modulating the gating of small conductance Ca2+-activated K+ channels. J Biol Chem 2001; 276:9762-9; PMID:11134030; http://dx.doi.org/10.1074/jbc.M010001200
- Ji H, Hougaard C, Herrik KF, Strobaek D, Christophersen P, Shepard PD. Tuning the excitability of midbrain dopamine neurons by modulating the Ca2+ sensitivity of SK channels. Eur J Neurosci 2009; 29:1883-95; PMID:19473240; http://dx.doi.org/10.1111/j.1460-9568.2009.06735.x
- Li W, Halling DB, Hall AW, Aldrich RW. EF hands at the N-lobe of calmodulin are required for both SK channel gating and stable SK-calmodulin interaction. J Gen Physiol 2009; 134:281-93; PMID:19752189; http://dx.doi.org/10.1085/jgp.200910295
- Zhang M, Pascal JM, Schumann M, Armen RS, Zhang JF. Identification of the functional binding pocket for compounds targeting small-conductance Ca2+-activated potassium channels. Nat Commun 2012; 3:1021; PMID:22929778; http://dx.doi.org/10.1038/ncomms2017
- Zhang M, Pascal JM, Zhang JF. Unstructured to structured transition of an intrinsically disordered protein peptide in coupling Ca2+-sensing and SK channel activation. Proc Natl Acad Sci USA 2013; 110:4828-33; PMID:23487779; http://dx.doi.org/10.1073/pnas.1220253110
- Zhang M, Meng XY, Cui M, Pascal JM, Logothetis DE, Zhang JF. Selective phosphorylation modulates the PIP2 sensitivity of the CaM-SK channel complex. Nat Chem Biol 2014; 10:753-9; PMID:25108821; http://dx.doi.org/10.1038/nchembio.1592
- Hopf FW, Simms JA, Chang SJ, Seif T, Bartlett SE, Bonci A. Chlorzoxazone, an SK-type potassium channel activator used in humans, reduces excessive alcohol intake in rats. Biol Psychiatry 2011; 69:618-24; PMID:21195386; http://dx.doi.org/10.1016/j.biopsych.2010.11.011
- Padula AE, Griffin WC 3rd, Lopez MF, Nimitvilai S, Cannady R, McGuier NS, Chesler EJ, Miles MF, Williams RW, Randall PK, et al. KCNN genes that encode small-conductance Ca2+-activated K+ channels influence alcohol and drug addiction. Neuropsychopharmacology 2015; 40:1928-39; PMID:25662840; http://dx.doi.org/10.1038/npp.2015.42
- Oliveira MS, Skinner F, Arshadmansab MF, Garcia I, Mello CF, Knaus HG, Ermolinsky BS, Otalora LF, Garrido-Sanabria ER. Altered expression and function of small-conductance (SK) Ca2+-activated K+ channels in pilocarpine-treated epileptic rats. Brain Res 2010; 1348:187-99; PMID:20553876; http://dx.doi.org/10.1016/j.brainres.2010.05.095
- Schulz R, Kirschstein T, Brehme H, Porath K, Mikkat U, Kohling R. Network excitability in a model of chronic temporal lobe epilepsy critically depends on SK channel-mediated AHP currents. Neurobiol Dis 2012; 45:337-47; PMID:21889592; http://dx.doi.org/10.1016/j.nbd.2011.08.019
- Alvina K, Khodakhah K. KCa channels as therapeutic targets in episodic ataxia type-2. J Neurosci 2010; 30:7249-57; PMID:20505091; http://dx.doi.org/10.1523/JNEUROSCI.6341-09.2010
- Walter JT, Alvina K, Womack MD, Chevez C, Khodakhah K. Decreases in the precision of Purkinje cell pacemaking cause cerebellar dysfunction and ataxia. Nat Neurosci 2006; 9:389-97; PMID:16474392; http://dx.doi.org/10.1038/nn1648
- Shakkottai VG, do Carmo Costa M, Dell'Orco JM, Sankaranarayanan A, Wulff H, Paulson HL. Early changes in cerebellar physiology accompany motor dysfunction in the polyglutamine disease spinocerebellar ataxia type 3. J Neurosci 2011; 31:13002-14; PMID:21900579; http://dx.doi.org/10.1523/JNEUROSCI.2789-11.2011
- Cerminara NL, Rawson JA. Evidence that climbing fibers control an intrinsic spike generator in cerebellar Purkinje cells. J Neurosci 2004; 24:4510-7; PMID:15140921; http://dx.doi.org/10.1523/JNEUROSCI.4530-03.2004
- McKay BE, Engbers JD, Mehaffey WH, Gordon GR, Molineux ML, Bains JS, Turner RW. Climbing fiber discharge regulates cerebellar functions by controlling the intrinsic characteristics of Purkinje cell output. J Neurophysiol 2007; 97:2590-604; PMID:17267759; http://dx.doi.org/10.1152/jn.00627.2006
- Ristori G, Romano S, Visconti A, Cannoni S, Spadaro M, Frontali M, Pontieri FE, Vanacore N, Salvetti M. Riluzole in cerebellar ataxia: a randomized, double-blind, placebo-controlled pilot trial. Neurology 2010; 74:839-45; PMID:20211908; http://dx.doi.org/10.1212/WNL.0b013e3181d31e23
- Feil K, Claassen J, Bardins S, Teufel J, Krafczyk S, Schneider E, Schniepp R, Jahn K, Kalla R, Strupp M. Effect of chlorzoxazone in patients with downbeat nystagmus: a pilot trial. Neurology 2013; 81:1152-8; PMID:23975871; http://dx.doi.org/10.1212/WNL.0b013e3182a55f6d
- Hipólito L, Fakira AK, Cabañero D, Blandón R, Carlton SM, Morón JA, Melyan Z. In vivo activation of the SK channel in the spinal cord reduces the NMDA receptor antagonist dose needed to produce antinociception in an inflammatory pain model. Pain. 2015; 156:849-58; PMID:25734988; http://dx.doi.org/10.1097/j.pain.0000000000000124
- Edwards G, Feletou M, Weston AH. Endothelium-derived hyperpolarising factors and associated pathways: a synopsis. Pflugers Arch 2010; 459:863-79; PMID:20383718; http://dx.doi.org/10.1007/s00424-010-0817-1
- Wulff H, Köhler R. Endothelial small-conductance and intermediate-conductance KCa channels: an update on their pharmacology and usefulness as cardiovascular targets. J Cardiovas Pharmacol 2013; 61:102-12; PMID:23107876; http://dx.doi.org/10.1097/FJC.0b013e318279ba20
- Damkjaer M, Nielsen G, Bodendiek S, Staehr M, Gramsbergen JB, de Wit C, Jensen BL, Simonsen U, Bie P, Wulff H, et al. Pharmacological activation of KCa3.1/KCa2.3 channels produces endothelial hyperpolarization and lowers blood pressure in conscious dogs. Br J Pharmacol 2012; 165:223-34; PMID:21699504; http://dx.doi.org/10.1111/j.1476-5381.2011.01546.x
- Radtke J, Schmidt K, Wulff H, Kohler R, de Wit C. Activation of KCa3.1 by SKA-31 induces arteriolar dilatation and lowers blood pressure in normo- and hypertensive connexin40-deficient mice. Br J Pharmacol 2013; 170:293-303; PMID:23734697; http://dx.doi.org/10.1111/bph.12267
- Toyama K, Wulff H, Chandy KG, Azam P, Raman G, Saito T, Fujiwara Y, Mattson DL, Das S, Melvin JE, et al. The intermediate-conductance calcium-activated potassium channel KCa3.1 contributes to atherogenesis in mice and humans. J Clin Invest 2008; 118:3025-37; PMID:18688283; http://dx.doi.org/10.1172/JCI30836
- Bi D, Toyama K, Lemaître V, Takai J, Fan F, Jenkins DP, Wulff H, Gutterman DD, Park F, Miura H. The intermediate conductance calcium-activated potassium channel KCa3.1 regulates vascular smooth muscle cell proliferation via controlling calcium-dependent signaling. J Biol Chem 2013; 288:15843-53; PMID:23609438; http://dx.doi.org/10.1074/jbc.M112.427187
- Lamy C, Goodchild SJ, Weatherall KL, Jane DE, Liégeois JF, Seutin V, Marrion NV. Allosteric block of KCa2 channels by apamin. J Biol Chem 2010; 285:27067-77; PMID:20562108; http://dx.doi.org/10.1074/jbc.M110.110072
- Weatherall KL, Seutin V, Liegeois JF, Marrion NV. Crucial role of a shared extracellular loop in apamin sensitivity and maintenance of pore shape of small-conductance calcium-activated potassium (SK) channels. Proc Natl Acad Sci USA 2011; 108:18494-9; PMID:22025703; http://dx.doi.org/10.1073/pnas.1110724108
- Strøbaek D1, Hougaard C, Johansen TH, Sørensen US, Nielsen EØ, Nielsen KS, Taylor RD, Pedarzani P, Christophersen P. Inhibitory gating modulation of small conductance Ca2+-activated K+ channels by the synthetic compound (R)-N-(benzimidazol-2-yl)-1,2,3,4-tetrahydro-1-naphtylamine (NS8593) reduces afterhyperpolarizing current in hippocampal CA1 neurons. Mol Pharmacol 2006; 70:1771-82; PMID:16926279; http://dx.doi.org/10.1124/mol.106.027110
- Sørensen US1, Strøbaek D, Christophersen P, Hougaard C, Jensen ML, Nielsen EØ, Peters D, Teuber L. Synthesis and structure-activity relationship studies of 2-(N-substituted)-aminobenzimidazoles as potent negative gating modulators of small conductance Ca2+-activated K+ channels. J Med Chem 2008; 51:7625-34; PMID:18998663; http://dx.doi.org/10.1021/jm800809f
- Campos Rosa J, Galanakis D, Piergentili A, Bhandari K, Ganellin CR, Dunn PM, Jenkinson DH. Synthesis, molecular modeling, and pharmacological testing of bis-quinolinium cyclophanes: potent, non-peptidic blockers of the apamin-sensitive Ca2+-activated K+ channel. J Med Chem 2000; 43:420-31; PMID:10669569; http://dx.doi.org/10.1021/jm9902537
- Fletcher DI, Ganellin CR, Piergentili A, Dunn PM, Jenkinson DH. Synthesis and pharmacological testing of polyaminoquinolines as blockers of the apamin-sensitive Ca2+-activated K+ channel (SKCa). Bioorg Med Chem 2007; 15:5457-79; PMID:17560109; http://dx.doi.org/10.1016/j.bmc.2007.05.054
- Oliván-Viguera A1, Valero MS, Murillo MD, Wulff H, García-Otín AL, Arbonés-Mainar JM, Köhler R. Novel phenolic inhibitors of small/intermediate-conductance Ca2+-activated K+ channels, KCa3.1 and KCa2.3. PLoS One 2013; 8:e58614; PMID:23516517; http://dx.doi.org/10.1371/journal.pone.0058614
- Oliván-Viguera A, Valero MS, Coleman N, Brown BM, Laría C, Murillo MD, Gálvez JA, Díaz-de-Villegas MD, Wulff H, Badorrey R, et al. A novel pan-negative-gating modulator of KCa2/3 channels, fluoro-di-benzoate, RA-2, inhibits endothelium-derived hyperpolarization-type relaxation in coronary artery and produces bradycardia in vivo. Mol Pharmacol 2015; 87:338-48; PMID:25468883; http://dx.doi.org/10.1124/mol.114.095745
- Jenkins DP1, Strøbæk D, Hougaard C, Jensen ML, Hummel R, Sørensen US, Christophersen P, Wulff H. Negative gating modulation by (R)-N-(benzimidazol-2-yl)-1,2,3,4-tetrahydro-1-naphthylamine (NS8593) depends on residues in the inner pore vestibule: pharmacological evidence of deep-pore gating of K(Ca)2 channels. Mol Pharmacol 2011; 79:899-909; PMID:21363929; http://dx.doi.org/10.1124/mol.110.069807
- Bruening-Wright A, Lee WS, Adelman JP, Maylie J. Evidence for a deep pore activation gate in small conductance Ca2+-activated K+ channels. J Gen Physiol 2007; 130:601-10; PMID:17998394; http://dx.doi.org/10.1085/jgp.200709828
- Wulff H, Gutman GA, Cahalan MD, Chandy KG. Delineation of the clotrimazole/TRAM-34 binding site on the intermediate conductance calcium-activated potassium channel, IKCa1. J Biol Chem 2001; 276:32040-5; PMID:11425865; http://dx.doi.org/10.1074/jbc.M105231200
- Herrik KF, Christophersen P, Shepard PD. Pharmacological modulation of the gating properties of small conductance Ca2+-activated K+ channels alters the firing pattern of dopamine neurons in vivo. J Neurophysiol 2010; 104:1726-35; PMID:20660424; http://dx.doi.org/10.1152/jn.01126.2009
- Skibsbye L, Wang X, Axelsen LN, Bomholtz SH, Nielsen MS, Grunnet M, Bentzen BH, Jespersen T. Antiarrhythmic Mechanisms of SK Channel Inhibition in the Rat Atrium. J Cardiovasc Pharmacol 2015; PMID:25856531
- Haugaard MM, Hesselkilde EZ, Pehrson S, Carstensen H, Flethøj M, Præstegaard KF, Sørensen US, Diness JG, Grunnet M, Buhl R, et al. Pharmacologic inhibition of small-conductance calcium-activated potassium (SK) channels by NS8593 reveals atrial antiarrhythmic potential in horses. Heart Rhythm 2015; 12:825-35; PMID:25542425; http://dx.doi.org/10.1016/j.hrthm.2014.12.028
- Diness JG, Skibsbye L, Jespersen T, Bartels ED, Sørensen US, Hansen RS, Grunnet M. Effects on atrial fibrillation in aged hypertensive rats by Ca2+-activated K+ channel inhibition. Hypertension 2011; 57:1129-35; PMID:21502564; http://dx.doi.org/10.1161/HYPERTENSIONAHA.111.170613
- Jenkins DP, Yu W, Brown BM, Lojkner LD, Wulff H. Development of a QPatch automated electrophysiology assay for identifying KCa3.1 inhibitors and activators. Assay Drug Dev Technol 2013; 11:551-60; PMID:24351043; http://dx.doi.org/10.1089/adt.2013.543
- Korsgaard MP, Strøbæk D, Christophersen P. Automated planar electrode electrophysiology in drug discovery: examples of the use of QPatch in basic characterization and high content screening on Na(v), K(Ca)2.3, and K(v)11.1 channels. Comb Chem High Throughput Screen 2009; 12:51-63; PMID:19149491; http://dx.doi.org/10.2174/138620709787048037
- Jorgensen S, Dyhring T, Brown DT, Strøbæk D, Christophersen P, Demnitz J. A high-throughput screening campaign for detection of Ca2+-activated K+ channel activators and inhibitors using a fluorometric imaging plate reader-based Tl+-influx assay. Assay Drug Dev Technol 2013; 11:163-72; PMID:23198866; http://dx.doi.org/10.1089/adt.2012.479