ABSTRACT
Background: Intrauterine chronic hypoxia (ICH) can lead to pancreatic dysmetabolism in offspring. This study aimed to determine the changes in islet function of offspring through a rat ICH model and detect the factors affecting islet function. Methods: Twenty couples of healthy Sprague – Dawley adult rats were randomly mated, and the pregnant rats were randomly allocated to ICH and normal control (NC) groups. Pregnant rats in the ICH group were placed in a hypoxic chamber with 13% oxygen concentration for hypoxia treatment twice a day for 4 h until delivery at 21 days. NC group is inlet with normal air from beginning to end. After delivery, blood was taken from the heart of pregnant rats for blood gas analysis. The weight of the offspring rats was measured at 12 h after birth and 16 weeks after birth. At 16 weeks, the immunohistochemical results of β-cell total, islet area, insulin (INS), and glucose transporter 2 (GLUT2) proteins were obtained from the islets. The mRNA data of INS and pancreatic and duodenal homeobox 1 (PDX-1) genes were obtained from pancreas. Results: We found the β-cell total, islet area, and the positive cell area of INS and GLUT2 of offspring rats in ICH group were lower than those of NC group, while the levels of INS and PDX-1 genes were higher in ICH group than in NC group. Conclusions: ICH can lead to islet hypoplasia in adult male offspring rats. However, this is within the compensatory range.
GRAPHICAL ABSTRACT
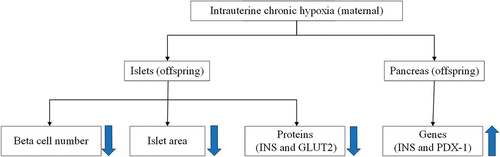
KEYWORDS:
Introduction
Intrauterine chronic hypoxia (ICH) is one of the most common complications of pregnancy, and its direct cause is inadequate perfusion of blood to the placenta.Citation1,Citation2 Indirect risk factors, such as pre-eclampsia, preterm labor, cardiopulmonary disease, obesity, and high altitude, may also cause intrauterine hypoxia.Citation3–5 It is reported that offspring of pregnant rats exposed to hypoxic environment show elevated blood pressure and blood glucose compared to those exposed to normal oxygen level.Citation6,Citation7 In addition, some animal models of mid-to-late-pregnancy hypoxia have shown that ICH leads to lung and nerve damage, reduction in ovarian primordial follicle count, telomere length shortening, and accelerated aging in offspring of rodents.Citation8–11 ICH caused by various factors will induce fetal blood flow to be preferentially supplied to the heart and brain and other vital organs, possibly at the expense of others,Citation12 thus affecting fetal development.Citation13 This mechanism is called a phenomenon of “fetal programming,”Citation14 which uses genetic modifications to automatically adapt to external stimuli.Citation15,Citation16 However, this mechanism increases the offspring’s susceptibility to metabolic disease in adulthood.Citation6,Citation17,Citation18
Islets are the endocrine portion of the pancreas. β-Cells are the most important and the most abundant ones in islets because they secrete insulin (INS) as the only hormone with hypoglycemic effects. An adverse intrauterine environment, such as placental insufficiency,Citation19 may result in reduced β-cell numbers and impaired function.Citation20,Citation21 However, the mechanisms by which maternal hypoxia exerts long-term effects on the offspring and islet function in the offspring affected by ICH remain poorly understood.
Therefore, in this study, we analyzed the functional changes in the islets of the offspring through an ICH rat model. Furthermore, we investigated the mechanisms involved in these changes by detecting the factors affecting islet function.
Materials and methods
Establishment of an ICH animal model, collection of specimens and ethical approval information
Twenty healthy male Sprague – Dawley (SD) rats (2 months old, weighing 350–400 g) and 20 healthy females (2 months old, weighing 260–300 g) with no mating history were obtained from Shanghai Slaughter Laboratory Animal Co. (License No.: SCXK (Shanghai) 2012–0002, Certificate of Conformity No.: 2015000515518). The rats were kept at the Laboratory Animal Center of Quanzhou Medical College (Laboratory Animal Use Permit No.: SYXK (Min) 2016–0001). The animal study protocol was approved by the Ethics Committee of the Second Affiliated Hospital of Fujian Medical University (protocol code 290 and date of approval 2021).
As described by Aiken et al.,Citation9 SD male rats were randomly mated with female rats in the same cage at 1:1, and pregnancy was confirmed by observing the vaginal plug.Citation22 The day the vaginal plugs were detected was considered the first day of pregnancy. A total of 20 pregnant rats were immediately removed from the mating cage. Five pregnant rats were randomly selected to be housed in the same cage (four separate cages for individual rearing). All pregnant rats were randomly allocated to an ICH or NC group of ten rats each. The ICH group was placed in a 13% oxygen concentration hypoxic chamber on day 6 of gestation for hypoxic treatment, with a small fan constantly mixing the air inside the chamber. The oxygen concentration in the chamber was continuously monitored using an oxygen detector, and the flow of oxygen and nitrogen was adjusted in real-time to maintain the oxygen concentration at 13%, while carbon dioxide and water vapor in the chamber were absorbed by calcium chloride and sodium lime, respectively. A small hole in the oxygen chamber was connected to the outside. Thus, the air pressure inside the chamber could be balanced with the atmospheric pressure. The concentration of carbon dioxide in the silo was less than 3%. Inside the laboratory room, the temperature was 19–23°C, and humidity was 58–65%.
The rats were allowed to have natural lighting, ad libitum feeding, and water. The pregnant rats were removed from the room, placed in a normal air cage, and subjected to oxygen deprivation twice a day, in the morning and afternoon, for 4 h each time, until the day before delivery. Subsequently, they were delivered at a normal oxygen concentration. In the NC group, 10 pregnant rats were placed in a normal chamber with 21% oxygen concentration. Air was continuously introduced to maintain the oxygen concentration inside the chamber at the same level as outside the chamber. The other conditions were the same in both groups. After hypoxic treatment, the arterial blood gas analysis of all pregnant rats was obtained from blood collected from the heart. In order to avoid the effects of unequal planes of nutrition, we randomly raised ten offspring rats from each litter. In 12 h after delivery, 20 male offspring rats from 20 pregnant rats (10 for ICH, 10 for NC) were randomly selected (an offspring from a litter) and weighed. After 16 weeks, the 20 offspring rats were weighed and anesthetized with 10% chloral hydrate at a dose of 3.5 mL/kg intraperitoneally. The abdominal cavity was opened, the whole pancreas was isolated. Then the pancreas was partially placed in lyophilized tubes, snap frozen in liquid nitrogen, moved to −80°C refrigerator for storage to extract intact RNA, and partially fixed in 10% formalin for 24–48 h for immunohimstochemical examination.
Immunohistochemical examinations
Formalin-fixed pancreatic tissue was paraffin-embedded and sectioned at 5 μm thickness. The field of view with islets was randomly selected, and an average of five images were taken from each section. Immunohistochemical methods were used to detect the positive cell area of INS and glucose transporter 2 (GLUT2) in islet and the total number of β-cells in islet (expressed as a percentage of the area of β-cells positive at typical sites in the observed pancreatic tissue under low magnification) and the islet area. The antibody concentration for immunohistochemistry was 1 mg/mL, and the dilution ratio was 1:100 (BEIJING BIOSYNTHESIS BIOTECHNOLOGY CO., LTD). The measurements for the results of immunohistochemistry were performed blindly. The NC group was considered negative group. The positive group was none. Positive cells should meet the following criteria: good contrast in section staining, clear background, and accurate localization. The positive cell area of the entire section was observed in the 400× field of view of an Olympus B×53light microscope. The field of view with islets was randomly selected, and an average of five images, under 400× magnification, was taken for each section. After DAB staining and hematoxylin counterstaining, positive cell area was quantified using the Image Pro Plus 6.0 (IPP) image analysis software. The integrated optical density (IOD) of each positive result was measured using the IPP image analysis software.Citation23 The average IOD values of five islets were calculated for quantitative analysis and comparatively analyzed. The IOD value represents the amount of stained material and reflects the relative content of the material.
Detection of INS and PDX-1 mRNA levels using qRT-PCR
The qRT-PCR (quantitative reverse transcription-polymerase chain reaction) was performed after reverse transcription using a high-volume cDNA reverse transcription kit (TransGen Co. Ltd., Beijing). All PCR amplification reactions were carried out in triplicate. Samples were normalized for expression levels and then evaluated using the 2−△△Ct method.
After pancreatic tissue was removed from the −80°C refrigerator, the total RNA sample with eligible quality, purity, and concentration was extracted following the manuals of RNA extraction kit (TransZol Up Plus RNA Kit (Cat. No.: ER501–01), Beijing).
Based on the real-time qPCR primer design principles, the primer sequences are determined as follows ():
Figure 1. Sequential information of (a) β-actin gene, (b) INS gene and (c) PDX-1 gene. INS: Insulin, PDX-1: Pancreatic and duodenal homeobox 1.
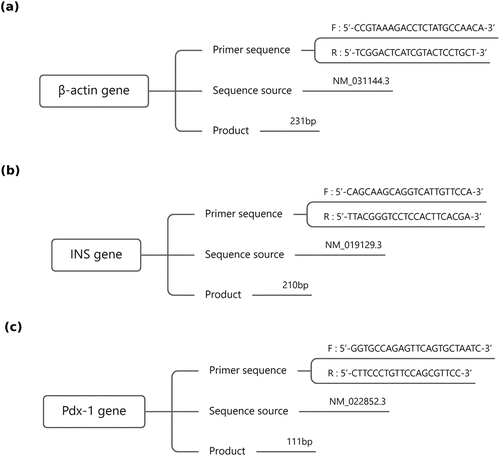
The first-strand cDNA was synthesized following the manuals of reverse transcription kit (TransScriptTM One-Step gDNA Removal and cDNA Synthesis SuperMix (Cat. No.: AT311–01), Beijing).
The fluorescent quantitative PCR kit (2×TransStarTM Top Green qPCR SuperMix (Cat. No.: AQ131–02), Beijing) and QuantStudio (TM) 6 Flex System Fluorescent PCR instrument (ABI Co., America) were used to perform the real-time qPCR experiments following the manuals. The optimal template concentration and annealing temperature were determined to ensure the specificity of PCR primers and the efficiency of PCR amplification. Once the real-time qPCR amplification reaction system and reaction conditions were optimized, the experiments were carried out. Each reaction was repeated three times. The differential gene expression was analyzed. β-Actin was used as the internal reference gene, with the NC group as the control sample and the ICH group as the test sample. The 2−△△Ct method was used to analyze the gene transcription levels, calculate the relative quantity (RQ) of each gene and compare the level of each gene in different samples to obtain the differential transcription analysis results.
Statistical methods
The SPSS 20.0 software was used for statistical analysis. Data conforming to normal or nearly normal distribution were analyzed using the Student’s t-test; otherwise, the nonparametric test was used. The differences were considered statistically significant at P < 0.05.
Results
Arterial blood gas analysis
The partial pressure of carbon dioxide in arterial blood (PaCO2) () of pregnant rats in the ICH group (6.98 ± 1.13 KPa) was not significantly different from that in the NC group (6.01 ± 0.64 KPa) (t = 2.113, P = 0.053). The partial pressure of oxygen in arterial blood (PaO2) () of pregnant rats in the ICH group (5.64 ± 1.78 KPa) was significantly lower than that in the NC group (9.59 ± 0.35 KPa) (t = 6.159, P < 0.001). The potential of hydrogen (pH) () of pregnant rats in the ICH group (7.21 ± 0.12) was significantly lower than that in the NC group (7.42 ± 0.10) (t = 3.803, P = 0.002). And the oxygen saturation of the arterial blood (SaO2) () of pregnant rats in the ICH group (61%±6%) was significantly lower than that in the NC group (89%±11%) (t = 6.321, P < 0.001).
Figure 2. Comparison of blood gas analysis of pregnant rats in ICH group and NC group (n = 8 for ICH and n = 8 for NC). (a) Carbon dioxide partial pressure (PaCO2) and oxygen partial pressure (PaO2); (b) Hydrogen potential (pH); (c) Oxyhemoglobin saturation (SaO2). Data are shown as mean and standard deviation. P-values were calculated by unpaired Student’s t-test. *p < 0.05. ICH: Intrauterine chronic hypoxia, NC: Normal control.
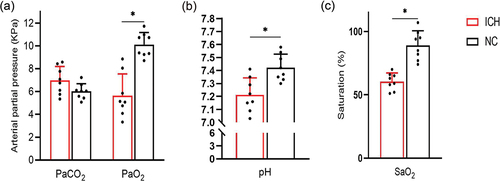
Comparison of body weight after birth
The body weight of the offspring rats at 12 h after birth () was significantly less in the ICH group (5.0 ± 0.1 g) than in the NC group (7.3 ± 0.3 g) (t = 23.0, P < 0.001). The body weight of the offspring rats at 16 weeks after birth () of pregnant rats in the ICH group (410.4 ± 33.3 g) was not significantly different from that in the NC group (425.2 ± 12.7 g) (t = 1.313, P = 0.206).
Figure 3. Body weight of offspring rats at different stages after birth in ICH group and NC group (n = 10 for ICH and n = 10 for NC). (a) 12 h after birth, (b) 16 weeks after birth. Data are shown as mean and standard deviation. P-values were gained by using unpaired Student’s t-test. *p < 0.05. ICH: Intrauterine chronic hypoxia, NC: Normal control.
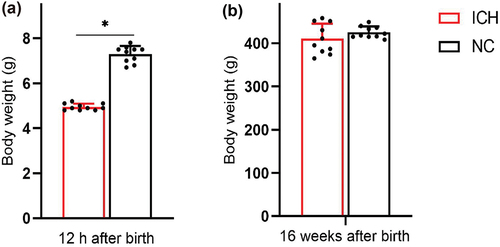
Islet immunohistochemical results
Immunohistochemical staining of the islets of adult male offspring showed significant declines in β-cell total () in the ICH group (0.85% ± 0.10%) compared to the NC group (1.13% ± 0.05%) (t = 7.920, P < 0.001). Islet area () also declined in the ICH group (11,904 ± 1573 μm2) compared to the NC group (15,324 ± 761 μm2) (t = 6.189, P < 0.001).
Figure 4. Immunohistochemical results of islets in offspring rats (DAB × 400) (n = 10 for ICH and n = 10 for NC). (a) Islets in ICH group, (b) islets in NC group. (c) INS positive cell area in ICH group, (d) INS positive cell area in NC group. (e) GLUT2 positive cell area in ICH group, (f) GLUT2 positive cell area in NC group. Comparison of (g) total β-cells and (h) islet area of adult male offspring rats in ICH and NC groups. The β-cell positive area of a typical site at low magnification is expressed as a percentage of the pancreatic tissue area observed. (i) Comparison of positive cell area of INS and GLUT2 of adult male offspring rats in ICH group and NC group. Integrated optical density (IOD) values were used to reflect the relative content of positive cell area of substances. Data are shown as mean and standard deviation. P-values were gained by using unpaired Student’s t-test. *p < 0.05. ICH: Intrauterine chronic hypoxia, NC: Normal control, INS: Insulin, GLUT2: Glucose transporter 2.
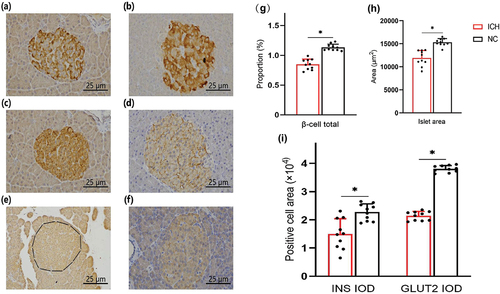
In addition, immunohistochemical staining of the islets of adult male offspring showed significant declines in INS positive cell area () in the ICH group ([(1.50 ± 0.51) × 104]) compared to the NC group ([(2.27 ± 0.28) × 104]) (t = 4.185, P < 0.001). And GLUT2 positive cell area () also declined in the ICH group ([(2.14 ± 0.15) × 104]) compared to the NC group ([(3.81 ± 0.11) × 104) (t = 28.391, P < 0.001).
Gene transcription levels in the pancreas
The level of INS gene () was significantly higher in the ICH group (2.66 ± 1.82) than in the NC group (0.75 ± 0.69) (t = 2.672, P = 0.024). And the level of PDX-1 gene () was significantly higher in the ICH group (3.88 ± 3.31) than in the NC group (0.89 ± 0.85) (t = 2.435, P = 0.040).
Figure 5. Comparison of relative quantity (RQ) of INS and PDX-1 mRNA of adult male offspring rats in ICH and NC groups (n = 8 for ICH and n = 5 for NC). RQ were used to reflect the relative quantity of the expression of INS and PDX-1 gene. Data are shown as mean and standard deviation. P-values were gained by using unpaired Student’s t-test. *p < 0.05. ICH: Intrauterine chronic hypoxia, NC: Normal control, INS: Insulin, PDX-1: Pancreatic and duodenal homeobox 1.
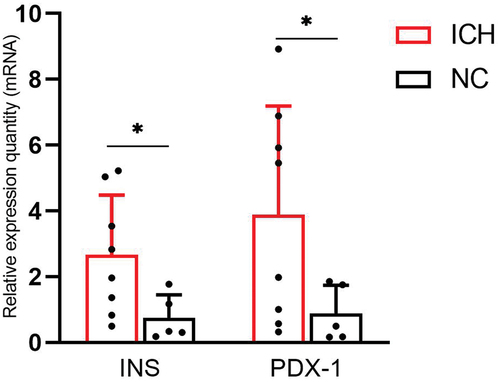
Discussion
In this study, the impairment of islet function was determined in the ICH group by comparing β-cell count and islet area. In ICH group, the positive cell area of INS and GLUT2 decreased significantly, indicating that the promoter factors that stimulate the secretion of insulin by β-cells decreased, which further demonstrated the impairment of islet function. However, the increased expression of INS and PDX-1 genes, at the molecular level, demonstrated impaired β-cell function, requiring it to work harder to maintain euglycemia.
In this study, blood gas analysis of pregnant rats showed that PaO2 and SaO2 in ICH group were significantly lower than those in NC group, which proved that the hypoxia treatment of pregnant rats was successful. The PaCO2 in the ICH group was slightly higher than that in NC group, indicating that we did not cause significant CO2 retention. The pH value of ICH group was significantly lower than that of NC group, which was consistent with SaO2 according to Bohr effect. The weight of offspring rats at different stages after birth showed that the weight of offspring rats in ICH group was significantly lower than that in NC group within 12 h after birth, while the weight of offspring rats in 16 weeks (adult stage) was not significantly different, which was consistent with previous studies.Citation24
In our study, adult male islets in the offspring of the ICH group had reduced islet area and β-cell number compared to those in the NC group. These results suggest that β-cells are damaged functionally and that damaged β-cells may initiate apoptosis, further reducing the number of β-cells. At present, there are mainly two theories,Citation25–27 constant endoplasmic reticulum stress and glucolipotoxicity effect, which suggest that after the damage of β-cells, the apoptosis process is initiated, causing a large number of β-cells to decrease. Our results are consistent with previous studies. In addition, the decreased positive cell area of INS and GLUT2 was further evidence of the decrease of β-cells. Because studies have shown that in maturity-onset diabetes of the young type 3 (MODY3) patients, downregulated GLUT2 levels and mutations in the encoding gene SLC2A2 cause a defect in INS secretion.Citation28
However, increased expression of INS and PDX-1 genes was also observed in the ICH group, suggesting that there may be a “negative feedback” process as a compensatory mechanism for β-cell dysfunction in the body due to the changes in the metabolic environment. PDX-1 is one of the most important regulatory factors related to β-cell development, regeneration, differentiation, and other functions.Citation18 In mature β-cells, PDX-1 can activate INS genes and positively promote the secretion of related factors involved in glucose metabolism, such as GLUT2 protein.Citation29 They can promote insulin secretion together. Besides, in vitro experiments revealed that PDX-1 allows adipose-derived mesenchymal stem cells to differentiate into insulin-secreting cells, with great potential for islet β-cell secretion and regenerative capacity.Citation30
This study had a few limitations. The PDX-1 gene in this study may promote the up-regulation of INS genes, but the extent of its promotion needs to be further studied. The mechanisms of altered glucose and lipids in the offspring of adult rats in the ICH group should be further investigated. In addition, in this study, only quantitative reverse transcription PCR (qRT-PCR) was used to detect the level of INS and PDX-1 mRNA, and the mechanism of the altered islet function in the offspring in the ICH group should be further investigated by using western blot and microarray analyses in future studies.
In conclusion, ICH damages the islet development and β-cell function of adult male offspring rats, whose direct manifestation is a decrease in the β-cell total and islet area.
It is also indirectly reflected by decreased INS and GLUT2 positive cell areas. However, the increased relative expression of INS and PDX-1 genes suggests the existence of a “negative feedback” regulatory mechanism that compensates for the loss of β cell number and function and contributes to β cell stability. In this experiment, the damage of β-cells is still within the compensable range. If irreversible decompensation is reached, the expression of INS and PDX-1 may be reduced.
Ethics committee approval
We conducted this study in full conformance with the Declaration of Helsinki principles and the animal study protocol was approved by the Ethics Committee of the Second Affiliated Hospital of Fujian Medical University (protocol code 290 and date of approval 2021).
Acknowledgments
We would like to thank Editage (www.editage.cn) for English language editing.
Disclosure statement
No potential conflict of interest was reported by the author(s).
Additional information
Funding
References
- Lynch TA, Westen E, Li D, Katzman PJ, Malshe A, Drennan K. Stillbirth in women with diabetes: a retrospective analysis of fetal autopsy reports. J Matern Fetal Neonatal Med. 2020;35(11):2091–9. doi:10.1080/14767058.2020.1779213.
- Zur RL, Kingdom JC, Parks WT, Hobson SR. The placental basis of fetal growth restriction. Obstet Gynecol Clin North Am. 2020;47(1):81–98. doi:10.1016/j.ogc.2019.10.008.
- Moore LG. Hypoxia and reproductive health: reproductive challenges at high altitude: fertility, pregnancy and neonatal well-being. Reproduction. 2021;161(1):F81–90. doi:10.1530/REP-20-0349.
- Bianchi C, Taricco E, Cardellicchio M, Mandò C, Massari M, Savasi V, Cetin I. The role of obesity and gestational diabetes on placental size and fetal oxygenation. Placenta. 2021;103:59–63. doi:10.1016/j.placenta.2020.10.013.
- Liao J, Zhang Z, Huang W, Huang Q, Bi G. Neonatal neuron specific enolase, a sensitive biochemical marker of neuronal damage, is increased in preeclampsia: a retrospective cohort study. Brain Dev. 2020;42(8):564–571. doi:10.1016/j.braindev.2020.04.011.
- Zhang Q, Xiao X, Zheng J, Li M, Yu M, Ping F, Wang T, Wang X. A maternal high-fat diet induces DNA methylation changes that contribute to glucose intolerance in offspring. Front Endocrinol (Lausanne). 2019;10:871. doi:10.3389/fendo.2019.00871.
- Sutovska H, Molcan L, Koprdova R, Piesova M, Mach M, Zeman M. Prenatal hypoxia increases blood pressure in male rat offspring and affects their response to artificial light at night. J Dev Orig Health Dis. 2021;12(4):587–594. doi:10.1017/S2040174420000963.
- Lawrence KM, McGovern PE, Mejaddam A, Rossidis AC, Baumgarten H, Kim A, Grinspan JB, Licht DJ, Didier RA, Vossough A, et al. Chronic intrauterine hypoxia alters neurodevelopment in fetal sheep. J Thorac Cardiovasc Surg. 2019;157(5):1982–1991. doi:10.1016/j.jtcvs.2018.12.093.
- Aiken CE, Tarry-Adkins JL, Spiroski AM, Nuzzo AM, Ashmore TJ, Rolfo A, Sutherland MJ, Camm EJ, Giussani DA, Ozanne SE. Chronic gestational hypoxia accelerates ovarian aging and lowers ovarian reserve in next-generation adult rats. FASEB J. 2019;33(6):7758–7766. doi:10.1096/fj.201802772R.
- Tong Y, Zhang S, Riddle S, Zhang L, Song R, Yue D. Intrauterine hypoxia and epigenetic programming in lung development and disease. Biomedicines. 2021;9(8):944. doi:10.3390/biomedicines9080944.
- Rudloff S, Bileck A, Janker L, Wanner N, Liaukouskaya N, Lundby C, Huber TB, Gerner C, Huynh-Do U. Dichotomous responses to chronic fetal hypoxia lead to a predetermined aging phenotype. Molecular & Cellular Proteomics: MCP. 2022;21(2):100190. doi:10.1016/j.mcpro.2021.100190.
- Fajersztajn L, Veras MM. Hypoxia: from placental development to fetal programming. Birth Defects Res. 2017 Oct 16;109(17):1377–1385. doi:10.1002/bdr2.1142.
- Norvilaitė K, Ramašauskaitė D, Bartkevičienė D, Žaliūnas B, Kurmanavičius J. Doppler ultrasonography of the fetal tibial artery in high-risk pregnancy and its value in predicting and monitoring fetal hypoxia in IUGR fetuses. Medicina (Kaunas). 2021;57(10):1036. doi:10.3390/medicina57101036.
- Barker DJ, Thornburg KL. Placental programming of chronic diseases, cancer and lifespan: a review. Placenta. 2013 Oct;34(10):841–845. doi:10.1016/j.placenta.2013.07.063.
- Cao G, González J, Ortiz Fragola JP, Muller A, Tumarkin M, Moriondo M, Azzato F, Blanco MV, Milei J. Structural changes in endocrine pancreas of male Wistar rats due to chronic cola drink consumption. Role of PDX-1. PLoS One. 2021;16(6):e0243340. doi:10.1371/journal.pone.0243340.
- Liu J, Lang G, Shi J. Epigenetic regulation of PDX-1 in type 2 diabetes mellitus. Diabetes Metab Syndr Obes. 2021;14:431–442. doi:10.2147/DMSO.S291932.
- Munetsuna E, Yamada H, Yamazaki M, Ando Y, Mizuno G, Hattori Y, Kageyama I, Teshigawara A, Nouchi Y, Ishikawa H, et al. Maternal fructose intake predisposes rat offspring to metabolic disorders via abnormal hepatic programming. FASEB J. 2021;35(12):e22030. doi:10.1096/fj.202101276R.
- Christoforou ER, Sferruzzi-Perri AN. Molecular mechanisms governing offspring metabolic programming in rodent models of in utero stress. Cell Mol Life Sci. 2020;77(23):4861–4898. doi:10.1007/s00018-020-03566-z.
- Mohan R, Baumann D, Alejandro EU. Fetal undernutrition, placental insufficiency, and pancreatic β-cell development programming in utero. Am J Physiol Regul Integr Comp Physiol. 2018;315(5):R867–R878. doi:10.1152/ajpregu.00072.2018.
- Boehmer BH, Limesand SW, Rozance PJ. The impact of IUGR on pancreatic islet development and β-cell function. J Endocrinol. 2017;235(2):R63–76. doi:10.1530/JOE-17-0076.
- Marciniak A, Patro-Małysza J, Kimber-Trojnar Ż, Marciniak B, Oleszczuk J, Leszczyńska-Gorzelak B. Fetal programming of the metabolic syndrome. Taiwanese Journal Of Obstetrics And Gynecology query. 2017 Apr;56(2):133–138. doi:10.1016/j.tjog.2017.01.001.
- Azimzadeh M, Jelodar G. Prenatal and early postnatal exposure to radiofrequency waves (900 MHz) adversely affects passive avoidance learning and memory. Toxicol Ind Health. 2020;36(12):1024–1030. doi:10.1177/0748233720973143.
- Sun S, Zheng G, Zhou D, Zhu L, He X, Zhang C, Wang C, Yuan C. Emodin interferes with nitroglycerin-induced migraine in rats through CGMP-PKG pathway. Front Pharmacol. 2021 Oct 20;12:758026. doi:10.3389/fphar.2021.758026.
- Ding H, Luo Y, Hu K, Huang H, Liu P, Xiong M, Zhu L, Yi J, Xu Y. Hypoxia in utero increases the risk of pulmonary hypertension in rat offspring and is associated with vasopressin type‑2 receptor upregulation. Mol Med Rep. 2020;22:4173–4182. doi:10.3892/mmr.2020.11533.
- Zhang IX, Raghavan M, Satin LS. The endoplasmic reticulum and calcium homeostasis in pancreatic beta cells. Endocrinology. 2020;161:bqz028. doi:10.1210/endocr/bqz028.
- Prentki M, Peyot ML, Masiello P, Madiraju SRM. Nutrient-induced metabolic stress, adaptation, detoxification, and toxicity in the pancreatic β-cell. Diabetes. 2020;69(3):279–290. doi:10.2337/dbi19-0014.
- Castro MC, Villagarcía HG, Román CL, Maiztegui B, Flores LE, Schinella GR, Massa ML, Francini F. Chronological appearance of endocrine and metabolic dysfunctions induced by an unhealthy diet in rats. Medicina (Kaunas). 2021;58(1):8. doi:10.3390/medicina58010008.
- Low BSJ, Lim CS, Ding SSL, Tan YS, NHJ N, Krishnan VG. Decreased GLUT2 and glucose uptake contribute to insulin secretion defects in MODY3/HNF1A hiPSC-derived mutant β cells. Nat Commun. 2021;12(1):3133. doi:10.1038/s41467-021-22843-4.
- Dewanjee S, Vallamkondu J, Kalra RS, Chakraborty P, Gangopadhyay M, Sahu R, Medala V, John A, Reddy PH, De Feo V, et al. The emerging role of HDACs: pathology and therapeutic targets in diabetes mellitus. Cells. 2021;10(6):1340. doi:10.3390/cells10061340.
- Takahashi H, Sakata N, Yoshimatsu G, Hasegawa S, Kodama S. Regenerative and transplantation medicine: cellular therapy using adipose tissue-derived mesenchymal stromal cells for type 1 diabetes mellitus. J Clin Med. 2019;8(2):249. doi:10.3390/jcm8020249.