ABSTRACT
The placenta works as a selective barrier, protecting the fetus from potential infections that may affect the maternal organism during pregnancy. In this review, we will discuss several challenging infections that are common within Latin American countries and that may affect the maternal-fetal interface and pose risks to fetal development. Specifically, we will focus on emerging infectious diseases including the arboviruses, malaria, leishmaniasis, and the bacterial foodborne disease caused by Shiga toxin-producing Escherichia coli. We will also highlight some topics of interest currently being studied by research groups that comprise an international effort aimed at filling the knowledge gaps in this field. These topics address the relationship between exposure to microorganisms and placental abnormalities, congenital anomalies, and complications of pregnancy.
Abbreviations
ADE: antibody-dependent enhancement; CCL2: monocyte chemoattractant protein-1; CCL3: macrophage inflammatory protein-1 α; CCL5: chemokine (C-C motif) ligand 5; CHIKV: chikungunya virus; DCL: diffuse cutaneous leishmaniasis; DENV: dengue virus; Gb3: glycolipid globotriaosylceramyde; HIF: hypoxia-inducible factor; HUS: hemolytic uremic syndrome; IFN: interferon; Ig: immunoglobulins; IL: interleukin; IUGR: intrauterine growth restriction; LCL: localized cutaneous leishmaniasis; LPS: lipopolysaccharid; MCL: mucocutaneous leishmaniasis; NO: nitric oxide; PCR: polymerase chain reaction; PGF: placental growth factor; PM: placental malaria; RIVATREM: Red Iberoamericana de Alteraciones Vasculares em transtornos del Embarazo; sVEGFR: soluble vascular endothelial growth factor receptor; STEC: shiga toxin-producing Escherichia coli; stx: shiga toxin protein; TNF: tumor necrosis factor; TOAS: T cell original antigenic sin; Var2CSA: variant surface antigen 2-CSA; VEGF: vascular endothelial growth factor; VL: visceral leishmaniasis; WHO: world health organization; YFV: yellow fever virus; ZIKV: Zika virus
Introduction
The placenta has many vital functions, such as transport of nutrients and respiratory gases and secretion of hormones, among other essential roles that allow healthy embryonic/fetal development. Another important placental role is to serve as a selective barrier, protecting the fetus of potentially deleterious infections that may affect the maternal organism during pregnancy (Burton et al. Citation2016). These infections affecting the fetus during pregnancy may result either from intra-uterine vertical transmission or from unexpected shifts within the intra-uterine microbiome.
While the maternal organism tolerates the allogeneic fetus, it must also protect itself against invading agents. The new balance between placental tolerance and systemic protection is achieved via complex immune system modulating mechanisms, including depression of cell-mediated immunity with maintenance of normal humoral immunity. Some pathogens systemically present in the mother can take advantage of these circumstances, colonize the placenta, break the maternal-fetal barrier, and infect the fetus, placing the pregnant women as a population group at risk, due to their susceptibility to infectious diseases. Several different cell layers constitute the placental-blood barrier, including syncytiotrophoblast, discontinuous inner cytotrophoblast layer, basal lamina of the trophoblast, mesenchymal tissue of the villus, basal lamina of the endothelium, and endothelium of the fetal placental capillary. Accordingly, these layers are arranged in a physical fence to selectively prevent pathogens and cells crossing from the maternal circulation from entering the fetal environment.
On the top placental-blood barrier, acting as a defensive mechanism, are the Hofbauer cells, representing the first-line immune response in the placenta. These cells are placental resident macrophages with the ability to protect the placenta against pathogenic infection, preventing intra-uterine vertical transmission (Zulu et al. Citation2019). Although maternal immune cells do not cross this barrier, maternal immunoglobulins (Ig), including IgG, do further contribute to intra-uterine immune defense (Schneider and Miller Citation2010).
Pregnant women constitute a population group at risk due to their higher susceptibility to infectious diseases. Although not yet clarified, ‘susceptibility’ to infections in pregnant women is more often, but not exclusively, described for pathogens that have optional or mandatory intracellular life cycles, such as viruses (e.g., cytomegalovirus), bacteria (e.g., Listeria monocytogenes) and protozoan parasites (e.g., Toxoplasma gondii) (Medawar Citation1952; Robbins et al. Citation2010, Citation2012; Duaso et al. Citation2011; Zeldovich and Bakardjiev Citation2012; Berger et al. Citation2017).
The World Health Organization (WHO) listed some relevant neglected tropical diseases from around the world (WHO Citation2017). Among them, leishmaniasis and dengue, as well as several arbovirus-related diseases, are recognized to be vertically transmitted during intra-uterine life. The Latin America region reports the highest number of dengue cases, with more than 2 million cases per year. Also, Latin America is among the regions that display the highest distribution of leishmaniasis, and Brazil has the highest incidence around the world.
Another vertically transmitted disease affecting Latin America is malaria. The impact of this condition is so wide-spread that the WHO supports the Global Malaria Programme, where 37 countries, including some Latin American countries, join efforts to control and eliminate malaria. While Paraguay and Argentina received the WHO certification as malaria-free countries; others, including Equator, Mexico, Costa Rica, and Suriname still report the occurrence of this infection (WHO Citation2019).
Another recent topic that has caught the attention of public health officials at WHO is bacterial foodborne diseases, and among them, infection with Shiga toxin-producing Escherichia coli (STEC). Of interest, it seems that STEC infection can also cause adverse pregnancy outcomes through vertical transmission (Sacerdoti et al. Citation2018). This type of foodborne infection is easily preventable by simple hygiene and food safety measures. Even so, the incidence of this disease is extremely high in Latin America, and Peru, Bolivia, and the Equator, are among the countries with most notable infection (Nations Citation2018).
In this review, we will analyze the emerging infections affecting the mother, which may impose deleterious effects on the placenta and, consequently, on fetal growth/development. We focus on infectious diseases that are considered public health challenges within Latin America countries, and that are currently being studied from a maternal-fetal perspective by research groups associated to RIVATREM (Red Iberoamericana de Alteraciones Vasculares em transtornos del Embarazo), as part of an international effort to fill the knowledge gaps in this field. This investigative network seeks to strengthen the exchange of knowledge and scientific collaboration for the study of vascular problems associated with pregnancy disorders, with an emphasis on Latin America.
Emerging and reemerging infections in Latin America
Arbovirus infections
Arbovirus (arthropod-borne virus) infections are a current public health problem caused by viruses that are transmitted by arthropod vectors and are pathogenic to human beings. They belong to six leading main RNA virus families (Togaviridiae, Flaviviridae, Bunyaviridae, Reoviridae, Rhabdoviridae, and Orthomyxoviridae) with high genetic variability (Charlier et al. Citation2017). Most arboviruses that cause disease in human beings display high similarity between the clinical aspects or even the absence of them, which can complicate their early diagnosis (Liang et al. Citation2015). The most common manifestations are fever, possibly associated with cutaneous, joint, neurological, or hemorrhagic symptoms and signs, although several arboviruses infections can be asymptomatic (Charlier et al. Citation2017).
Several arboviruses are currently associated with maternal and fetal pathologies, such as Chikungunya virus (CHIKV), Western equine encephalitis virus, Venezuelan equine encephalitis virus, Ross River virus, Dengue virus (DENV), Japanese encephalitis virus, West Nile virus, Yellow fever virus (YFV), Crimean-Congo hemorrhagic fever virus, La Crosse virus, and Rift Valley fever virus (Charlier et al. Citation2017). However, the arbovirus most studied in recent years due to the 2015–2016 outbreak in the Americas is the Zika virus (ZIKV), which received great worldwide attention due to the link with maternal infection and fetal microcephaly and other central nervous system abnormalities (Mlakar et al. Citation2016; de Araújo et al. Citation2018).
More recent studies demonstrated an association of other arboviruses, such as DENV and CHIKV, with poor neonatal outcomes such as meningoencephalitis, myocarditis, bullous skin lesions, and other clinical manifestations (Gerardin et al. Citation2014; Contopoulos-Ioannidis et al. Citation2018; Paixao, et al. Citation2018a). Although other infections associated with congenital disorders are included in prenatal screening, the lack of adherence to the prenatal care program or the incomplete screening for these infections, remain relevant health problems for the mothers and their babies in Latin America (Neu et al. Citation2015; Carvalho et al. Citation2019). In Brazil, for example, the Unified Health System still does not require ZIKV, DENV, or CHIKV serology or molecular biology tests in their prenatal screening tests, even after the recent outbreaks. The lack of standardized screening protocols leaves to the physician the decision whether to order arboviruses tests, in cases where patients present fever and rash during pregnancy.
Although many arbovirus infections result in adverse effects during pregnancy for both mother and child, their current impact during pregnancy is still being studied, and many questions remain to be answered, along with an accurate description of the incidence of adverse fetal outcomes and placental involvement. Furthermore, the pathophysiological mechanisms allowing ZIKV, DENV, and CHIKV to cross the placental barrier remain unclear.
Zika virus (ZIKV)
The ZIKV is a member of the Flaviviridae family, which was initially isolated in Nigeria in 1954 and has been detected since 2015 in the Americas, where it has been causing a worrisome number of cases of congenital brain abnormalities. Although the ZIKV epidemic in the Americas has ceased at present, the infection still constitutes a significant cause of concern, especially in pregnant women living in eco-epidemiologically prone areas (Rodriguez-Morales et al. Citation2018).
Maternal symptoms are generally low-grade fever, headache, and cutaneous rashes. ZIKV infection can also cause myalgia, arthralgia, and vomiting, all of which are also common symptoms of other arbovirus diseases (Mlakar et al. Citation2016; de Araújo et al. Citation2018). Moreover, ZIKV can also cause autoimmune neurologic complications such as Guillain-Barré syndrome, as well as acute disseminated encephalomyelitis in rare occasions (Franca et al. Citation2016; Suwanmanee and Luplertlop Citation2017).
During pregnancy, ZIKV transmission may occur vertically, by crossing the placental barrier and infecting the fetus, which will have a variety of symptoms and malformations depending on gestational age. ZIKV infections during the first trimester can cause the congenital Zika syndrome, which is characterized by stillbirth/miscarriage, intrauterine growth restriction (IUGR), as well as microcephaly, ventriculomegaly, and other brain malformations (Brasil et al. Citation2016; Franca et al. Citation2016). These conditions are caused indirectly by placental inflammation and directly by ZIKV replication in the fetal brain. Thus, several studies have detected ZIKV in fetal or neonatal brains, amniotic fluid, and placenta (Calvet et al. Citation2016; Driggers et al. Citation2016; Mlakar et al. Citation2016), meaning that this virus can be vertically transmitted during intra-uterine life, increasing both intra-uterine and new-born mortality.
Regarding the histopathological findings in ZIKV infected placentas, these vary from patient to patient, as well as according to the gestational trimester of infection. Among others, the most common findings were: chronic inflammation (TORCH type) with villitis, villous immaturity with the persistence of the cytotrophoblast layer and thickening of their basement membrane, edema and hyperplasia of Hofbauer cells, as well as some degree of hypervascularity and stromal fibrosis, and deciduitis (Noronha et al. Citation2016; de Noronha et al. Citation2018). Nevertheless, other studies reported that placentas infected with ZIKV did not have villitis, TORCH type inflammation, necrosis, or scarring, although confirmed the villous immaturity and Hofbauer cells hyperplasia (Rosenberg et al. Citation2017; Schwartz Citation2017). Moreover, the absence or significantly lesser amount of pathological changes in placentas from patients infected in the third trimester of pregnancy strongly suggests that the time of infection is relevant to finding histopathological alterations in the placentas.
Many studies using mouse models to address ZIKV infection in pregnancy have been published recently (Adibi et al. Citation2016; Aliota et al. Citation2016; Miner et al. Citation2016; Cao et al. Citation2017; Kam et al. Citation2017; Xavier-Neto et al. Citation2017; Regla-Nava et al. Citation2018). Those mouse models have successfully demonstrated that ZIKV infection during early pregnancy resulted in infection of the placenta and the fetal brain, showing similar histopathological findings as ZIKV-infected human placentas (Paes et al. Citation2018). Also, ZIKV-infected mice placentas presented poor pregnancy outcomes such as IUGR, miscarriage, and fetal demise (Adibi et al. Citation2016; Aliota et al. Citation2016; Miner et al. Citation2016; Sheridan et al. Citation2018), as well as neural tube defects, hydrocephalus, and arthrogryposis (Xavier-Neto et al. Citation2017), confirming the association between ZIKV infection and those complications observed in human epidemiological studies.
Other experimental approaches using in vitro human placental explants, and bi- and tri-dimensional culture models have confirmed ZIKV infection and replication in the placenta (Petitt et al. Citation2017; Weisblum et al. Citation2017). These studies focused on elucidating which kind of cells are the main placental reservoirs and how those cells would help ZIKV cross the placental barrier and spread into the developing fetus. Thus, first trimester placentas are prone to be infected, and particularly Hofbauer cells, mesenchymal cells, fibroblasts, and cytotrophoblast cells (both villous and extravillous), as wells as decidual cells and decidual macrophages were infected by ZIKV. Replication of ZIKV has been found mainly in Hofbauer cells (Costa MA Citation2016; Tabata et al. Citation2016; Aagaard et al. Citation2017; Weisblum et al. Citation2017). In comparison, at term, both syncytiotrophoblasts and cytotrophoblasts are more resistant to ZIKV infection, which could be due to a decrease in receptors used for viral entry, such as TYRO3 and AXL (Bayer et al. Citation2016; Jurado et al. Citation2016; Quicke et al. Citation2016; Aagaard et al. Citation2017; Sheridan et al. Citation2017). However, ZIKV replicates in placentas from all trimesters, with viral RNA load levels slightly higher in first trimester placentas than third-trimester placentas (Bhatnagar et al. Citation2017). Several trophoblast cell lines were also successfully proven to be infected by ZIKV (Aldo et al. Citation2016; Cao et al. Citation2017; Arumugasaamy et al. Citation2018; Luo et al. Citation2018), modulating pro-apoptotic (Aldo et al. Citation2016) and pro-inflammatory (Ribeiro et al. Citation2018; Ma et al. Citation2018) pathways.
Since Jak/STAT and AKT-mTOR are also involved in brain pathogenesis and microcephaly development due to increased autophagy and suppression of the IFN response pathways (Ojha et al. Citation2018), some studies attempted to find therapeutic targets. Therefore, inhibiting autophagy by suppressing the Atg16L1 gene or by administering hydroxychloroquine can reduce vertical transmission of ZIKV in pregnant mice (Cao et al. Citation2017). Some studies also proposed administration of interferon (IFN)-ɣ during mid-pregnancy to promote host defense and reduce disease severity, as this cytokine was able to decrease ZIKV replication in JEG-3 cells, first-trimester human cytotrophoblast cells, and mouse models, as well as decreasing fetal malformations and demise (Chen et al. Citation2017; Corry et al. Citation2017).
The immunological cross-reactivity between ZIKV and other flaviviruses was recognized in the past years by independent research groups. Interestingly, some of the flaviviruses antibodies are capable of cross-neutralization, but also to elicit antibody-dependent enhancement (ADE) of ZIKV infection (Priyamvada et al. Citation2016; Bardina et al. Citation2017; Fernandez et al. Citation2017; Kam et al. Citation2017; Willis and Hensley Citation2017; Hermanns et al. Citation2018; Brown et al. Citation2019). Thus, previous exposition to DENV is related to increased pathogenesis of ZIKV due to ADE, a phenomenon initially described between DENV serotypes (Bardina et al. Citation2017). Using Stat2 knockout mice, Brown and collaborators (Citation2019) showed DENV antibodies increase ZIKV replication and pathogenesis during pregnancy, as well as inflammation in the placenta, regardless of gestational age, through FcγR in Hofbauer and trophoblast cells (Brown et al. Citation2019). Nonetheless, other studies showed antibodies against DENV prevented severe ZIKV infection and death in mouse models (Kam et al. Citation2017) or even presented therapeutic activity against ZIKV infection (Fernandez et al. Citation2017). Another phenomenon to be considered is DENV-specific CD8+ T cells, found to protect pregnant mice from ZIKV infection through a phenomenon known as T cell original antigenic sin (TOAS) (Regla-Nava et al. Citation2018).
The ZIKV epidemic began in 2007 in the western Pacific islands, followed in 2013–2014 by a larger outbreak in French Polynesia in the South Pacific. In Latin America, ZIKV emerged in 2015, mostly in Brazil, launching a public health emergency. Later, this infection spread to other countries in the Americas and the Pacific (Baud et al. Citation2017). Despite multiple studies in Brazil and other countries within the Americas, there are still many questions that should be addressed regarding the impact of ZIKV during pregnancy as well as on newborns and children exposed to this arboviral infection. For instance, an unsolved issue is the duration of viral persistence in mother and child (Villamil-Gomez et al. Citation2017), since viral RNA was detected in placentas after several weeks of maternal infection, indicating that the virus can persist in the placenta for extended periods following the onset of maternal symptoms (Rosenberg et al. Citation2017).
Dengue virus (DENV)
Dengue fever is a vector-borne disease caused by four distinct serotypes of the Dengue virus (DENV1, 2, 3, and 4) from the Flaviviridae family (Gubler Citation2002). Dengue has recently been one of the most critical public health issues in tropical and subtropical regions. Over the past 50 years, dengue fever has progressively achieved the status of a pandemic, with approximately 4 billion people at risk of infection, an estimated 390 million cases and 20,000 deaths occurring annually, and both pregnant women and infants belonging to the high-risk group (Messina et al. Citation2015). Nevertheless, few reports exist on DENV vertical transmission, fetal abnormalities, and placental infection (Basurko et al. Citation2009; Ribeiro et al. Citation2013; Nunes et al. Citation2016; Ribeiro et al. Citation2017; Paixao et al. Citation2018a; Kallur et al. Citation2019). Among the most common adverse outcomes of maternal DENV infection are preterm birth, IUGR, and classical dengue fever symptoms in newborns (Basurko et al. Citation2009; Ribeiro et al. Citation2018; Kallur et al. Citation2019), also definitely associated with increased maternal and fetal morbidity and mortality (Kallur et al. Citation2019).
In a case report, DENV infection in a Brazilian woman at 12 weeks of gestation resulted in spontaneous abortion eight days after the start of Dengue fever symptoms. After examination, the presence of DENV was confirmed in several tissues of the aborted fetus: kidneys, lung epithelial cells, and ependymal ventricular cells in the brain (Ribeiro et al. Citation2013). Moreover, before the recent epidemic linking ZIKV with congenital neurological abnormalities, flaviviruses were not considered to be associated with congenital malformations in humans, until a recent report by Paixao and collaborators (Citation2018a) that showed DENV was also associated with several brain malformations, increasing the odds of neurological abnormalities by 50% (Paixao et al. Citation2018a). Increased incidence of malformations in the fetal corpus callosum and holoprosencephaly were observed if Dengue fever occurred in the first trimester of pregnancy, as well as other alterations, such as congenital neurologic anomalies, anencephaly, encephalocele, microcephaly, congenital hydrocephalus, and other malformations of the brain and spinal cord (Paixao et al. Citation2018a). Interestingly, the hypothesis of an increased risk for neural tube defects after a Dengue fever outbreak was initially formulated in 1988 in India (Sharma and Gulati Citation1992), thirty years before the study from Paixao and collaborators. Also, since we now know DENV can also be vertically transmitted, some studies have shown placental alterations (Ribeiro et al. Citation2013, Citation2018; Nunes et al. Citation2016), such as cholangitis, villous hyper maturation, and edema, as well as general decidual and placental inflammation (Ribeiro et al. Citation2018).
Nunes and collaborators (Citation2016) reported a case where DENV2 RNA was detected in umbilical cord blood and both NS1 and NS3 viral proteins were found in the placenta and umbilical cord from a 32–33 weeks pregnancy that ended in maternal and fetal death (Nunes et al. Citation2016). The NS3 protein was also found inside Hofbauer cells, as well as cytotrophoblast cells (Aagaard et al. Citation2017). Interestingly, using the HTR-8 SV/neo cells, Luo and collaborators (Citation2018) showed DENV was able to infect them, and the viral RNA time-dependently increased in culture. Also, DENV infected cells produced cytokines such as IFN-ɣ, interleukin-6 (IL-6), tumor necrosis factor alfa (TNF-α), IL-8, monocyte chemoattractant protein-1 (CCL2), macrophage inflammatory protein-1 α (CCL3) and chemokine (C-C motif) ligand 5 (CCL5) (Luo et al. Citation2018). The authors also reported that compared to ZIKV, DENV was able to replicate as efficiently as ZIKV, and to induce similarly high IFN responses, but lower inflammatory cytokine and chemokine levels. Using placental term explants, it was shown that a strain of DENV2 was able to infect placentas similarly to ZIKV, mainly increasing the production of IFN-ɣ and 4, IL-6, IL-1β, and IL-10, although no increase in cell death was observed, which means DENV might act through different pathways than ZIKV (Ribeiro et al. Citation2018).
DENV infection affected approximately 23 million Brazilians in the past four decades (Salles et al. Citation2018). Despite the high incidence of DENV infection in Latin America, studies investigating the impact of this infection during pregnancy on placental function are still largely lacking. A recent case report of a pregnant woman who presented complications due to DEVN infection, leading to maternal and fetus death in the 29th gestational week, indicated the presence of DENV protein in the maternal side of the placenta and revealed fetal organs abnormalities including inflammation (Nunes et al. Citation2019).
The case reports discussed above highlight recent maternal mortality statistics data showing that DENV infection increases the risk of maternal death three times, and hemorrhagic fever caused by DENV increases this risk 450 times (Paixao et al. Citation2018). This is a clear indication that DENV infection should be further studied in the context of pregnancy.
Another essential point will be the evaluation of future vaccines being developed against DENV infection and whether they are effective and safe during pregnancy, given that the sole vaccine currently commercially available is only indicated to seropositive subjects to prevent reinfection in endemic areas (Wilder-Smith Citation2019).
Chikungunya virus (CHIKV)
The CHIKV was initially isolated in the 1950 s during an outbreak in Tanzania. Unlike DENV or ZIKV, CHIKV belongs to the Togaviridae family (Robinson Citation1955). Since its first isolation, three different genotypes were classified: the Asian, the East-Central South African, and the West African, according to genomic comparisons with phylogenetic analysis from originally reported regions (Wahid et al. Citation2017). The first vertical transmission was reported during the massive 2005–2006 outbreak on La Réunion Island, France (Economopoulou et al. Citation2009). The first Asian genotype case detected in the Americas was in the Caribbean island of Saint Martin in 2013 (Leparc-Goffart et al. Citation2014), whereas the first East-Central South African genotype was first isolated in Bahia state, Brazil in 2014 (Costa-da-Silva et al. Citation2017), where both strains started outbreaks through several American countries.
Regarding vertical transmission, the risk and impact of mother-to-child transmission of CHIKV are under review. Most of the knowledge derives from the experience of the massive outbreak caused by the East-Central South African genotype in La Réunion Island, where perinatal infection and several complications of neonatal illness were described (Ramful et al. Citation2007; Fritel et al. Citation2010). However, the Thailand 2009–2010 CHIKV outbreak is not related to maternal-vertical transmission or fetal alterations (Laoprasopwattana et al. Citation2016), which might be explained by different CHIKV strains.
Several studies indicated that CHIKV was able to cross the placental barrier, since maternal CHIKV infection in all trimesters resulted in fetal transmission in 15 to 50% of pregnancies (Senanayake et al. Citation2009; Gerardin et al. Citation2014; Torres et al. Citation2016), and was related to poor pregnancy outcomes, such as preterm births, IUGR, and spontaneous abortion (Senanayake et al. Citation2009; Gerardin et al. Citation2014; Dotters-Katz et al. Citation2015; Ramos et al. Citation2018). Fetal malformations are not frequent, but the newborn may display mild to severe infection symptoms, such as fever, irritability, rash, generalized edema, hyperalgesia, stiff neck, hemodynamic instability, exanthema, hemorrhagic disturbances, diarrhea, distal cyanosis, diffuse abdominal pain, hyperpigmentation, and bullous dermatosis (Senanayake et al. Citation2009; Torres et al. Citation2016; Escobar et al. Citation2017), but also meningoencephalitis, sepsis, respiratory failure and myocarditis in rare cases (Lenglet et al. Citation2006; Ramful et al. Citation2007; Torres et al. Citation2016). Another reason for concern is that CHIKV intrauterine infection is associated with postnatal-onset microcephaly, cerebral palsy, and seizures, as well as a long-term neurodevelopmental delay (Gerardin et al. Citation2014; Contopoulos-Ioannidis et al. Citation2018; Ramos et al. Citation2018).
Unfortunately, observations on changes in placental histopathology are not available, and only a few in vivo or in vitro studies are available regarding how CHIKV infected placental cells and crossed the placental barrier. A study conducted in mice showed that CHIKV was unable to surpass the placental barrier without breaches that allowed transfer of maternal blood to fetal circulation (Couderc et al. Citation2008), while another study found that CHIKV struggled to replicate in human decidua or villi explants, but still could infect cells and replicate, although slower than other related arboviruses (Platt et al. Citation2018). The limited number of studies available does not allow forming a conclusion on whether CHIKV causes substantive placental or fetal damage, despite all the evidence of vertical transmission in several countries, including in Latin America.
Malaria
Over 219 million cases of malaria were reported globally in 2017, resulting in nearly 435,000 deaths (Malária Citation2019), with sub-Saharan Africa being the most affected region. Although some Latin America countries are currently in a pre-elimination phase, malaria is still present in specific regions within Latin America (Alimi et al. Citation2015).
Among people affected by malaria, pregnant women are one of the populations at highest risk, considering their altered cell-mediated immune response. So far, what we have learned is that the hormonal status induced by pregnancy modulates the immune response, and parasitemia tends to be ten times higher within pregnant women, promoting poor pregnancy outcomes and obstetric complications (Rogerson Citation2017). Thus, new insights about the pathophysiology of placental malaria are necessary to explore biomarkers in the early stages of the plasmodial infection during pregnancy, in places showing a high incidence of this infection, including some Latin American countries.
The occurrence of malaria infection in pregnant women is well-described (Nosten et al. Citation1991). Indeed, the occurrence of malaria infection is increased up to 10-fold in primigravidae living under holoendemic conditions (Brabin Citation1983). Malaria is caused by Plasmodium falciparum infection, considered as the central parasite infection associated with low birth weight in first pregnancies in Africa (Walker et al. Citation2014). In Latin American countries such as Colombia, gestational malaria occurs due to both P. falciparum and Plasmodium vivax (Arango et al. Citation2013). Moreover, malaria infection correlates with maternal mortality in endemic zones due to obstetric complications (GBD 2015 Mortality and Causes of Death Collaborators. Citation2016).
Malarial infection in the placenta, mostly known as placental malaria (PM), is characterized by sequestration of infected erythrocytes in the intervillous spaces, initiating a local inflammatory response (Rogerson et al. Citation2003). During PM, the histopathological changes observed include peri villous fibrinoid deposits, augmented thickness of the placental barrier (Souza et al. Citation2013), syncytial knotting, and leukocyte infiltration (Muehlenbachs et al. Citation2010; Carmona-Fonseca et al. Citation2013; Souza et al. Citation2013).
Indeed, these structural modifications, along with vascular alterations within the placenta, directly contribute to the high incidence of low birth weight and fetal death observed in pregnancies committed by PM (Ismail et al. Citation2000). (Machado Filho et al. Citation2014; Lopez-Perez et al. Citation2016)
During P. falciparum infection, erythrocytes express Var2CSA, a variant of P. falciparum erythrocyte membrane protein 1 (Fried and Duffy Citation1996). This protein enables erythrocytes to bind to chondroitin sulfate A, expressed on the cytotrophoblast surface (Fried et al. Citation2006). Var2CSA and other ligands promote erythrocyte sequestering and secretion of CCL2 and CCL-3 (Suguitan et al. Citation2003), favoring monocyte recruitment and placental macrophage activation, actions which amplify the immune response initiated against P. falciparum. Simultaneously, pro-inflammatory cytokines such as TNF-α and IFN-ɣ are released, enhancing the placental inflammation. In the case of P. vivax, the search for specific proteins that promote adherence to placental tissue is still unresolved (Carvalho et al. Citation2010).
Furthermore, infiltration of leukocyte populations results in augmented oxygen consumption, which, combined with the reduced placental blood perfusion, culminates in increased expression of several factors including hypoxia inducible factor-1 (HIF-1α), vascular endothelial growth factor (VEGF), as well as its receptors (VEGFR-1 and sVEGFR-1) (Boeuf et al. Citation2008). Conversely, decreased placental growth factor (PGF) expression is observed. As a consequence, this inflammatory environment favors adverse gestational outcomes, resulting in placental insufficiency and therefore, intrauterine growth restriction (Fried et al. Citation1998; Boeuf et al. Citation2008). (Agudelo et al. Citation2014)
Other immune system components are also involved in PM. For example, in acute malaria infections, an increase of component 5a from the complement system was found (Conroy et al. Citation2009), eliciting angiogenic disturbances in the placenta (Conroy et al. Citation2011, Citation2013; McDonald et al. Citation2015).
Leishmaniasis
Leishmaniasis is a chronic disease caused by several species of protozoa from the Leishmania genre, mainly transmitted through the bite of infected vectors. Leishmania sp. are unicellular organisms belonging to the class Kinetoplastida and the family Trypanosomatidae, that infect humans and multiple other mammals. The leishmaniasis infectious process results in a broad spectrum of clinical manifestations or disease forms, classified as localized cutaneous (LCL), diffuse cutaneous (DCL), mucocutaneous (MCL), and visceral (VL) manifestations. These forms also differ from each other according to the species of the parasite involved, genetic predisposition, and the host’s immune system vulnerability (Desjeux Citation1996; Bastos Citation2012; Gurung and Kanneganti Citation2015).
Although classically leishmaniasis occurs via phlebotomines transmission, the occurrence of leishmaniasis in several regions where the presence of phlebotomines has not been reported suggests alternative routes of transmission, including sexual (venereal) and transplacental (vertical) transmission, with the latter already shown to be responsible for causing VL in mice (Nuwayri-Salti N Citation1985), dogs (Riera and Valladares Citation1996; Rosypal et al. Citation2005; Silva et al. Citation2009; Naucke and Lorentz Citation2012; Ben Slimane et al. Citation2014), and humans (Symmers Citation1960; Meinecke et al. Citation1999; Pagliano et al. Citation2005; Boehme Citation2006; Zinchuk and Nadraga Citation2010).
The first described case of congenital transmission of leishmaniasis occurred in England (Low Citation1926). Even though the pregnant woman remained infected throughout the gestational period, gestation was not compromised and there were no unfavorable symptoms. However, after birth, the child began to show clinical manifestations such as anemia and splenomegaly, and necropsy demonstrated the presence of Leishmania donovani, observed in spleen aspirates. A few years later, another case report described the presence of the parasite responsible for the visceral form of the disease in both the mother and the preterm newborn, arguing that congenital transmission contributed to premature childbirth (Nyakundi et al. Citation1988).
Immunocompromised patients, mostly HIV-infected, are currently subjects of study because of the increased risk of VL co-infection. This fact is of particular interest in developing countries, since the simultaneous presence of these two infections changes the natural course of the clinical manifestations, making it difficult, among other issues, to diagnose and treat both diseases. Similar to these patients, pregnant women are also becoming increasingly targeted by intracellular parasites such as those of the genus Leishmania, due to their immune response adaptation, which indeed confers a predisposition to reactivation of these parasites, leading to the aggravation of cutaneous lesions and fetal complications such as premature births and stillbirths (Morgan et al. Citation2007; Pagliano et al. Citation2016). Another likely form of transplacental transmission is the parasite-mediated degradation of the trophoblast, as occurs with Trypanosoma cruzi and Toxoplasma gondii (Medawar Citation1952; Robbins et al. Citation2010, Citation2012; Duaso et al. Citation2011; Zeldovich and Bakardjiev Citation2012; Berger et al. Citation2017).
Although there are some speculative comments on placenta being adapted to resist infection by pathogens, especially from maternal blood (Robbins et al. Citation2010), placental findings such as late maturation, chronic infarction, and ischemic changes in placental villi were described in a woman with VL, even in the absence of the parasite in this organ. Also, it has been considered that the disease may be associated with placental development and efficacy of the pregnancy (Caldas et al. Citation2003).
The non-classical cutaneous leishmaniasis transmission is less studied, because in this case, the parasites are predominantly found in cells of the phagocytic system and externally in the skin or in mucosal tissues, where the clinical symptoms of the disease occur. However, this does not make it impossible for the parasite to colonize other organs, such as the placenta and it is possible for parasites to reach the Hofbauer cells, considered as the central lineage of Leishmania target cells in this organ (Costa et al. Citation2005; Reithinger and Dujardin Citation2007; Romero et al. Citation2010).
In this sense, congenital transmission and invasion of the placenta by Leishmania parasite, reported in animals studies and humans placenta (Eltoum et al. Citation1992; Avila-Garcia et al. Citation2013; Mescouto-Borges et al. Citation2013), may change the placental structure and lead to villous vessel thrombosis and trophoblastic degeneration of the placenta (Eltoum et al. Citation1992; Baergen Citation2011), which are associated with outcomes such as severe maternal disease, preterm labor, intrauterine growth restriction, and fetal demise (Baergen Citation2011; Agrawal and Hirsch Citation2012; Robbins and Bakardjiev Citation2012; Kemp Citation2014).
Moreover, Leishmania chagasi DNA was detected by polymerase chain reaction (PCR) in 49% of placentas of symptomatic and asymptomatic dogs, indicating that although the placenta is probably not a target tissue for Leishmania infection, it serves as a transmission route for the fetuses, mainly because the placenta is highly vascularized. Leishmania mexicana is transplacentally transmitted and causes fetal death, implantation loss, and reduction of the bodyweight of offspring, demonstrating the importance of identifying the species that cause the cutaneous form of the disease as potentially able to cross the placental barrier and affect fetal health (Pangrazio et al. Citation2009; Avila-Garcia et al. Citation2013).
The capacity of Leishmania for placental invasion and congenital transmission via the transplacental route is also associated with the maternal immunological environment (Maspi et al. Citation2016; Berger et al. Citation2017). On one hand, the production of inflammatory cytokines, such as IL-12 (Park et al. Citation2002), IL-1, IFN-γ, TNF-α (D’Oliveira et al. Citation2002), and IL-2 contributes to activation of macrophages and parasite elimination. On the other hand, parasite replication and persistence are related to the development and production of IL-4, IL-5 and IL-13, leading to susceptibility to the Leishmania infection (Sacks and Noben-Trauth Citation2002; Von Stebut et al. Citation2003; Bailey and Lockwood Citation2007; Scott and Novais Citation2016).
Besides, infection in a murine model resistant to cutaneous leishmaniasis caused by Leishmania major increased the frequency of fetal resorptions and failures in fetal implantation in vivo, and this was associated with decreased IL-10 production and increased IFN-γ by the spleen and popliteal lymph node cells in vitro (Krishnan et al. Citation1996). Therefore, upon parasite infection during pregnancy, an expected Th1 lymphocyte response is changed in direction to Th2 lymphocyte activation.
Currently, leishmaniasis has a wide geographic distribution, being present in the Americas, Europe, Africa and Asia, and is generally associated with poverty, malnutrition, population displacement, and climatic and environmental changes. Worldwide prevalence of 12 million cases is estimated, with an annual incidence of around 50,000 to 90,000 new cases of VL, and between 600,000 and 1 million new cases of LCL. In the Americas, cutaneous leishmaniasis is endemic in 18 countries and 892,846 new cases have been reported in the period 2001–2016 (Mitropoulos et al. Citation2010; PAHO Citation2019).
Although leishmaniasis is a neglected disease, probably due to its low economic appeal, and although experimental and clinical evidence on leishmaniasis is even scarcer in pregnant women, this population should not be neglected, as they are vulnerable to the sequelae of leishmaniasis, which may even contribute to fetal outcomes. Although of significant prevalence, the scarcity of data on this topic shows that much needs to be studied, both by scientists and public health authorities, to be able to characterize the impact that the different types of parasites of the genus Leishmania exert during the whole process of pregnancy, in the short and possibly long term, in both mothers and fetuses.
Shiga toxin-producing escherichia coli
Shiga toxin-producing Escherichia coli (STEC) is a significant public health problem due to the contamination of food and water supplies. STEC is present in the intestinal tract of healthy cattle, and transmission to humans that occurs by consumption of undercooked ground beef, manure-contaminated water, vegetables, and direct transmission from person to person (Hussein Citation2007).
STEC produces a gastrointestinal infection that may manifest as diarrhea and hemorrhagic colitis. STEC can produce two antigenically diverse forms of Shiga toxin proteins (Stx1 and Stx2) and their variants, where Stx2 is more virulent and epidemiologically more relevant than Stx1 (Palermo et al. Citation2009). Once ingested, STEC colonizes the human intestine and releases Stx. This toxin crosses the epithelial barrier, reaching the systemic circulation and the target organs, mainly kidney and brain, and causing, among others, hemolytic uremic syndrome (HUS) (Karmali et al. Citation1983). HUS is characterized by thrombocytopenia, hemolytic anemia, acute renal failure, and central nervous system alterations. The massive HUS outbreak in 2011 in central Europe caused by a novel Stx2-producing STEC affected more adults than children (Frank et al. Citation2011).
Up to now, there is no specific treatment for HUS patients. The prevention of STEC infections and development of HUS principally depends on the appropriate cooking of food, avoiding cross-contamination, and the consumption of pasteurized dairy products (Thomas and Elliott Citation2013).
Regardless of the mechanism of Stx action, Stx may bind to glycolipid globotriaosylceramyde (Gb3) receptors on the endothelial cell surface, undergoing endocytosis through receptor-mediated internalization, followed by retrograde transport to the Golgi apparatus, and finally, inducing cell death by inhibition of protein synthesis and consequent cellular apoptosis (Raa et al. Citation2009).
Infections during pregnancy have been associated with a higher incidence of spontaneous abortion, preterm birth, or placental dysfunction (Romero RC, T. Citation2002; Baud and Greub Citation2011). However, the risks of spontaneous abortion or preterm birth in humans related to STEC infection are unknown or poorly understood. There are scarce reports of neonatal HUS caused by STEC transmission from mother to the newborn during delivery (Steele et al. Citation1984; Ulinski et al. Citation2005; Stritt et al. Citation2013) and a few cases of STEC-mediated HUS during pregnancy (Tanaka et al. Citation2000; Chart et al. Citation2002).
In vitro studies using the WISH cell line derived from human amniotic tissue showed that both Stx1 and Stx2 induce apoptosis (Yoshimura et al. Citation2002). Additionally, Stx2 impairs cell migration, invasion, and endovascular differentiation in extravillous trophoblast cells (Scalise et al. Citation2019).
Furthermore, it has been demonstrated that intraperitoneal injection of Stx2 in pregnant rats during early gestation leads to spontaneous abortion and perinatal complications, possibly linked with direct action of the toxin on placental tissues, which finally results in maternal morbidity and pregnancy loss (Sacerdoti et al. Citation2014). Stx2 binds to the placental microvasculature and decidual cells through GB3 receptors, eliciting placental hypoxia, and IUGR (Sacerdoti et al. Citation2015). As in other target organs, Stx2 also enhances placental inflammation, making the cells more sensitive to the toxin (Ibarra et al. Citation2013). Additionally, the injection of Stx2 and lipopolysaccharide (LPS) in pregnant rats increases nitric oxide (NO) and prostaglandin production in the placenta, leading to preterm delivery of dead fetuses (Ogando et al. Citation2003; Aisemberg et al. Citation2007; Burdet et al. Citation2010). Also, Stx2 increased circulating levels of TNF-α, altering the balance of cytokines at the maternal-fetal interface required for the proper placentation process (Burdet et al. Citation2013). Moreover, Stx2 can also cause spontaneous abortion in mice by damaging trophoblasts and producing intrauterine hemorrhage, fibrin deposition, and neutrophil infiltration (Yoshimura et al. Citation2000).
HUS is widely distributed around the world, with a low incidence rate (1–3 cases/100,000 children <5 years) (Nataro and Kaper Citation1998; Varela et al. Citation2008; Torres et al. Citation2018). In contrast, in South America, HUS is endemic, with an incidence 10-fold higher than in the rest of the world. In Chile and Uruguay, less than 10 cases per 100,000 children of 5 years of age were reported annually (Cordovez et al. Citation1992; Torres et al. Citation2018). In Argentina, HUS is highly prevalent, with an incidence of 10–17 cases per 100,000 children less than five years of age, and lethality of 1 to 4% (Rivas et al. Citation2014). This illness is recognized as the most common cause of acute renal failure in children and the second leading cause of chronic renal failure (Repetto Citation1997, Citation2005).
During pregnancy, several forms of thrombotic microangiopathy may occur because of HUS. In these patients, HUS drastically compromises the maternal kidney function, and this condition is worsened by the absence of specific and efficient treatments (Bruel et al. Citation2017). Since many of the causes of spontaneous abortion and preterm delivery are still unknown, it is reasonable to presume that STEC infections during pregnancy, either symptomatic or asymptomatic, maybe one of the causes for those perinatal complications. It is important to remark that in pathological processes such as fetal growth restriction, spontaneous abortion, preeclampsia, and preterm delivery are associated with inflammation and hypoxia. Thus, asymptomatic STEC infections might be involved in the etiology of these pregnancy disorders.
Concluding remarks
The Sustainable Development Goals of the United Nations organization include ‘to reduce the global maternal mortality ratio to less than 70 per 100,000 live births and end preventable deaths of newborns and children under five years of age, with all countries aiming to reduce neonatal mortality to at least as low as 12 per 1,000 live births and under-5 mortality to at least as low as 25 per 1,000 live births’ (The United Nations Development Program (UNDP) Citation2019). Over the past 20 years, substantial efforts were made to drastically reduce both maternal and neonatal mortality rates within most Latin America countries. Besides cardiovascular complications, which are among the most frequent causes of maternal and fetal mortality all over the world, neglected microorganisms represent significant contributors to disturbances observed during and after pregnancy in Latin America and other low-income countries. However, these conditions are frequently not addressed to the level that they require.
Arbovirosis: The recent events linking ZIKV infection to the occurrence of newborn microencephaly resulted in a tremendous effort from Latin America research groups to reveal the mechanisms involved in the deleterious effect of ZIKV infection during pregnancy. Even the international scientific community embraced the cause seeking out answers, considering the gravity of this epidemic. These joint efforts were imperative to elucidate basic knowledge regarding congenital ZIKV infection, in order to assist with the development of actions aimed at transmission prevention, patient education and communication, as well as public health policy development. However, additional work targeting essential issues related to ZIKV infection during pregnancy are necessary, including the construction of a network to support children with microencephaly and their families; the elucidation of therapies that could limit fetal damage upon infection; educational campaigns for the population, aiming reduction of ZIKV and other arbovirosis’ transmission and early recognition of symptoms; the development of tools for fast diagnostic; limiting the spread of these viruses around the world; creation of collaborative scientific networks, favoring multicentric studies; prioritization of financial resources to increase the knowledge in this field; among others actions is required.
Malaria: the target set by the United Nations organization is to reduce case incidence by at least 90% until 2030 and to eliminate malaria in at least 35 countries. The delay of several countries to pay attention to this disease and its occurrence in areas with difficult access has made the battle against malaria even harder. Efforts are necessary to diagnose asymptomatic infected subjects, considering that only 10-20% of infected people are currently diagnosed. Early diagnostic associated with appropriate treatment can limit malaria transmission. Additionally, it is necessary to improve the search for new therapies and address drug-resistance.
Leishmaniasis: Economically, leishmaniasis can be incredibly debilitating, since injuries can be mutilating, creating a social stigma. Therefore, combatting poverty is another critical point that should be addressed to prevent a disease that affects people with limited financial resources. The development of more effective vaccines and new therapies should be encouraged. Indeed, currently available treatments elicit severe side effects, and most of the time, require hospitalization for intravenous administration. The accuracy and speed of diagnosis are also aspects that challenge the reduction of leishmaniasis around the world. The adaptative capacity of these parasites represents a critical obstacle for addressing leishmaniasis adequately. Collaborative networks, such as the Kladrug-R project between Europe and India, represent important initiatives aiming to gain understanding in these fields.
STEC: Several domestic and wild animals are STEC reservoirs. However, ruminants are the major STEC reservoir, and in most cases, they are asymptomatic. Considering that some Latin American countries are prominent meat exporters, good practices both from producers and consumers are required to avoid food contamination. Special populations, including children, pregnant, and older people are more susceptible to the deleterious and permanent effects of this infection. Although the incidence of STEC is relatively low, some Latin American countries, including Argentina, Chile, and Uruguay, display a high incidence of this infection, and the severity of symptoms associated with sequelae justify further research on this topic.
A common point linking these topics is how these conditions affect the placenta. A brief overview of the data reviewed is presented on and . In this regard, it has been commonly considered that the human placenta fulfills multiple functions including a physical and immunological barrier that prevents the exposure of the embryo/fetus to microorganisms that could compromise its wellbeing and life. Likewise, the presence of pathogenic microorganisms in the placenta has been associated with congenital anomalies and complications of pregnancy, such as IUGR and preterm delivery. Therefore, considering the placenta as a critical protective element during pregnancy, further studies should directly address this organ during the occurrence of these and other infections.
Table 1. In vitro and in vivo studies in a placental model.
Figure 1. Main placental alterations associated with infection. In orange, the description of placental alterations caused by Arbovirosis, including chronic inflammation, deciduitis and villus disruption. In blue, the description of placental alterations caused by Malaria, including placental insufficiency, fibrinoid deposits and inflammation. In green, the description of placental alterations caused by Leishmaniasis, including late maturation, chronic infarction and ischemic changes. In yellow, the description of placental alterations caused by Shiga toxin-producing Escherichia coli, including placental dysfunction, intrauterine hemorrhage, fibrin deposition and neutrophil infiltration.
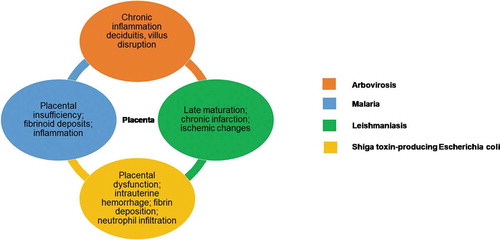
Some points of interests should be further addressed for the cited diseases including, but not only: how these infections affect the placental structure, disrupting the protective barrier conferred by this organ; the occurrence of genetic polymorphisms of drug placental transporters within Latin America population, within transporters used by the drugs currently used to treat these conditions; to elaborate or standardize clinical protocols, considering the classification of drugs used to treat these conditions according to the risk during pregnancy, and also the pharmacokinetics and pharmacodynamic alterations observed throughout the pregnancy; the development of alternative pharmacological treatments to replace drugs that display high rate of resistance or risk of use during pregnancy; the development of point of care tests, to rapidly diagnose these conditions in a noninvasive way, to include the serological screen for significant congenital infections, including arboviruses, during prenatal care.
Considering that the conditions addressed here represent emerging health conditions, which in most cases do not attract the attention of pharmaceutical industry or consolidated research groups, the establishment of cooperative networks addressing common points of interest may be innovative, as a strategy to unite expertise and resources to accomplish more robust investigative work in Latin America. These networks may also favor fund-raising strategies and strengthen research proposals, in an international effort to fill some knowledge gaps observed in the Latin American population. These diseases have significant research potential and joint research actions need to be extended to these and other emerging conditions.
Authors contributions
Manuscript writing (Leishmania): IMR, PCSS; manuscript writing (Arboviruses infection): AUB, ELLT; manuscript writing (Placental Dysbiosis and Microbiota): AC, AMA; manuscript writing (Malaria): JB, OA; manuscript writing (Introduction and Conclusion remarks): RGR, PA-R; manuscript writing (Shiga toxin-producing Escherichia coli): FS, AED, CI; project development, conceptualization, writing – review & editing: CE, TS, FRG; manuscript writing (Leishmania) and project administration: VVL.
Disclosure statement
The authors declare no conflict of interest.
Additional information
Funding
References
- Aagaard KM, Lahon A, Suter MA, Arya RP, Seferovic MD, Vogt MB, Hu M, Stossi F, Mancini MA, Harris RA, et al. eng. 2017. Primary human placental trophoblasts are permissive for Zika Virus (ZIKV) replication. Sci Rep. 7:41389. doi:10.1038/srep41389.
- Adibi JJ, Zhao Y, Cartus AR, Gupta P, Davidson LA. 2016. Placental mechanics in the Zika-microcephaly relationship. Cell Host Microbe. 20(1):9–11. eng. doi:10.1016/j.chom.2016.06.013
- Agrawal V, Hirsch E. 2012. Intrauterine infection and preterm labor. Semin Fetal Neonatal Med. 17(1):12–19. eng. doi:10.1016/j.siny.2011.09.001
- Agudelo OM, Aristizabal BH, Yanow SK, Arango E, Carmona-Fonseca J, Maestre A. 2014. Submicroscopic infection of placenta by plasmodium produces Th1/Th2 cytokine imbalance, inflammation and hypoxia in women from north-west Colombia. Malar J. 13:122. eng. doi:10.1186/1475-2875-13-122.
- Aisemberg J, Vercelli C, Billi S, Ribeiro ML, Ogando D, Meiss R, McCann SM, Rettori V, Franchi AM. 2007. Nitric oxide mediates prostaglandins’ deleterious effect on lipopolysaccharide-triggered murine fetal resorption. Proc Natl Acad Sci U S A. 104(18):7534–7539. eng. doi:10.1073/pnas.0702279104
- Aldo P, You Y, Szigeti K, Horvath TL, Lindenbach B, Mor G. 2016. HSV-2 enhances ZIKV infection of the placenta and induces apoptosis in first-trimester trophoblast cells. Am J Reprod Immunol. 76(5):348–357. eng. doi:10.1111/aji.12578
- Alimi TO, Fuller DO, Quinones ML, Xue RD, Herrera SV, Arevalo-Herrera M, Ulrich JN, Qualls WA, Beier JC. 2015. Prospects and recommendations for risk mapping to improve strategies for effective malaria vector control interventions in Latin America. Malar J. 14:519. eng. doi:10.1186/s12936-015-1052-1.
- Aliota MT, Caine EA, Walker EC, Larkin KE, Camacho E, Osorio JE. 2016. Characterization of lethal Zika virus infection in AG129 mice. PLoS Negl Trop Dis. 10(4):e0004682. eng. doi:10.1371/journal.pntd.0004682
- Arango EM, Samuel R, Agudelo OM, Carmona-Fonseca J, Maestre A, Yanow SK. 2013. Molecular detection of malaria at delivery reveals a high frequency of submicroscopic infections and associated placental damage in pregnant women from northwest Colombia. Am J Trop Med Hyg. 89(1):178–183. eng. doi:10.4269/ajtmh.12-0669
- Arumugasaamy N, Ettehadieh LE, Kuo CY, Paquin-Proulx D, Kitchen SM, Santoro M, Placone JK, Silveira PP, Aguiar RS, Nixon DF, et al. 2018. Biomimetic placenta-fetus model demonstrating maternal-fetal transmission and fetal neural toxicity of Zika virus. Ann Biomed Eng. 46(12):1963–1974. eng. doi:10.1007/s10439-018-2090-y.
- Avila-Garcia M, Mancilla-Ramirez J, Segura-Cervantes E, Farfan-Labonne B, Ramirez-Ramirez A, Galindo-Sevilla N. 2013. Transplacental transmission of cutaneous Leishmania mexicana strain in BALB/c mice. Am J Trop Med Hyg. 89(2):354–358. eng. doi:10.4269/ajtmh.12-0716
- Baergen RN. 2011. Manual of pathology of the human placenta. Boston, MA: Springer. doi:10.1007/978-1-4419-7494-5
- Bailey MS, Lockwood DN. 2007. Cutaneous leishmaniasis. Clin Dermatol. 25(2):203–211. eng. doi:10.1016/j.clindermatol.2006.05.008
- Bardina SV, Bunduc P, Tripathi S, Duehr J, Frere JJ, Brown JA, Nachbagauer R, Foster GA, Krysztof D, Tortorella D, et al. 2017. Enhancement of Zika virus pathogenesis by preexisting antiflavivirus immunity. Science. 356(6334):175–180. eng. doi:10.1126/science.aal4365.
- Bastos MM, Boechat N, Gomes ATPC, Neves MGPMS, Cavaleiro JAS. 2012. O uso de porfirinas em terapia fotodinâmica no tratamento da leishmaniose cutânea. Rev Virtual Quim. 4(3):257–267.
- Basurko C, Carles G, Youssef M, Guindi WE. 2009. Maternal and fetal consequences of dengue fever during pregnancy. Eur J Obstet Gynecol Reprod Biol. 147(1):29–32. eng. doi:10.1016/j.ejogrb.2009.06.028
- Baud D, Greub G. 2011. Intracellular bacteria and adverse pregnancy outcomes. Clin Microbiol infect. 17(9):1312–1322, eng.
- Baud D, Gubler DJ, Schaub B, Lanteri MC, Musso D. 2017. An update on Zika virus infection. Lancet. 390(10107):2099–2109. eng. doi:10.1016/S0140-6736(17)31450-2
- Bayer A, Lennemann NJ, Ouyang Y, Bramley JC, Morosky S, Marques ET Jr., Cherry S, Sadovsky Y, Coyne CB. 2016. Type III interferons produced by human placental trophoblasts confer protection against Zika virus infection. Cell Host Microbe. 19(5):705–712. eng. doi:10.1016/j.chom.2016.03.008
- Ben Slimane T, Chouihi E, Ben Hadj Ahmed S, Chelbi I, Barhoumi W, Cherni S, Zoghlami Z, Gharbi M, Zhioua E. 2014. An investigation on vertical transmission of Leishmania infantum in experimentally infected dogs and assessment of offspring’s infectiousness potential by xenodiagnosis. Vet Parasitol. 206(3–4):282–286. eng. doi:10.1016/j.vetpar.2014.10.020
- Berger BA, Bartlett AH, Saravia NG, Galindo Sevilla N. 2017. Pathophysiology of leishmania infection during pregnancy. Trends Parasitol. 33(12):935–946. eng. doi:10.1016/j.pt.2017.08.012
- Bhatnagar J, Rabeneck DB, Martines RB, Reagan-Steiner S, Ermias Y, Estetter LB, Suzuki T, Ritter J, Keating MK, Hale G, et al. 2017. Zika virus RNA replication and persistence in brain and placental tissue. Emerg Infect Dis. 23(3):405–414. eng. doi:10.3201/eid2303.161499.
- Boeuf P, Tan A, Romagosa C, Radford J, Mwapasa V, Molyneux ME, Meshnick SR, Hunt NH, Rogerson SJ. 2008. Placental hypoxia during placental malaria. J Infect Dis. 197(5):757–765. eng. doi:10.1086/526521
- Boehme CC, Novosel A, Eichenlaub S, Fleischmann E, Loscher T. 2006. Congenital visceral Leishmaniasis. Emerg Infect Dis. 12(2):359–360. doi:10.3201/eid1202.050449d.
- Brabin BJ. 1983. An analysis of malaria in pregnancy in Africa. Bull World Health Organ. 61(6):1005–1016.
- Brasil P, Pereira JP Jr., Moreira ME, Ribeiro Nogueira RM, Damasceno L, Wakimoto M, Rabello RS, Valderramos SG, Halai UA, Salles TS, et al. 2016. Zika virus infection in pregnant women in Rio de Janeiro. N Engl J Med. 375(24):2321–2334. eng. doi:10.1056/NEJMoa1602412.
- Brown JA, Singh G, Acklin JA, Lee S, Duehr JE, Chokola AN, Frere JJ, Hoffman KW, Foster GA, Krysztof D, et al. 2019. Dengue virus immunity increases Zika virus-induced damage during pregnancy. Immunity. 50(3):751–762e755. eng. doi:10.1016/j.immuni.2019.01.005.
- Bruel A, Kavanagh D, Noris M, Delmas Y, Wong EKS, Bresin E, Provot F, Brocklebank V, Mele C, Remuzzi G, et al. 2017. Hemolytic uremic syndrome in pregnancy and postpartum. Clinical Journal of the American Society of Nephrology: CJASN. 12(8):1237–1247. eng. doi:10.2215/CJN.00280117.
- Burdet J, Sacerdoti F, Cella M, Franchi AM, Ibarra C. 2013. Role of TNF-alpha in the mechanisms responsible for preterm delivery induced by Stx2 in rats. Br J Pharmacol. 168(4):946–953. eng. doi:10.1111/j.1476-5381.2012.02239.x
- Burdet J, Zotta E, Cella M, Franchi AM, Ibarra C. 2010. Role of nitric oxide in shiga toxin-2-induced premature delivery of dead fetuses in rats. PLoS One. 5(12):e15127. eng. doi:10.1371/journal.pone.0015127
- Burton GJ, Fowden AL, Thornburg KL. 2016. Placental origins of chronic disease. Physiol Rev. 96(4):1509–1565. eng. doi:10.1152/physrev.00029.2015
- Caldas AJ, Costa JM, Gama ME, Ramos EA, Barral A. 2003. Visceral leishmaniasis in pregnancy: a case report. Acta Trop. 88(1):39–43. eng. doi:10.1016/S0001-706X(03)00160-8
- Calvet G, Aguiar RS, Melo ASO, Sampaio SA, de Filippis I, Fabri A, Araujo ESM, de Sequeira PC, de Mendonca MCL, de Oliveira L, et al. 2016. Detection and sequencing of Zika virus from amniotic fluid of fetuses with microcephaly in Brazil: a case study. Lancet Infect Dis. 16(6):653–660. eng. doi:10.1016/S1473-3099(16)00095-5.
- Cao B, Parnell LA, Diamond MS, Mysorekar IU. 2017. Inhibition of autophagy limits vertical transmission of Zika virus in pregnant mice. J Exp Med. 214(8):2303–2313. eng. doi:10.1084/jem.20170957
- Carmona-Fonseca J, Arango E, Maestre A. 2013. Placental malaria in Colombia: histopathologic findings in Plasmodium vivax and P. falciparum infections. Am J Trop Med Hyg. 88(6):1093–1101. eng. doi:10.4269/ajtmh.12-0363
- Carvalho BO, Lopes SC, Nogueira PA, Orlandi PP, Bargieri DY, Blanco YC, Mamoni R, Leite JA, Rodrigues MM, Soares IS, et al. 2010. On the cytoadhesion of plasmodium vivax-infected erythrocytes. J Infect Dis. 202(4):638–647. eng. doi:10.1086/654815.
- Carvalho FR, Medeiros T, Vianna RAO, Douglass-Jaimes G, Nunes PCG, Quintans MDS, Souza CF, Cavalcanti SMB, Dos Santos FB, Oliveira SA, et al. eng. 2019. Simultaneous circulation of arboviruses and other congenital infections in pregnant women in Rio de Janeiro, Brazil. Acta Trop. 192:49–54. doi:10.1016/j.actatropica.2019.01.020.
- Charlier C, Beaudoin MC, Couderc T, Lortholary O, Lecuit M. 2017. Arboviruses and pregnancy: maternal, fetal, and neonatal effects. Lancet Child Adolesc Health. 1(2):134–146. eng. doi:10.1016/S2352-4642(17)30021-4
- Chart H, Perry NT, Cheasty T, Wright PA. 2002. The kinetics of antibody production to antigens of Escherichia coli O157 in a pregnant woman with haemolytic uraemic syndrome. J Med Microbiol. 51(6):522–525. eng. doi:10.1099/0022-1317-51-6-522
- Chen J, Liang Y, Yi P, Xu L, Hawkins HK, Rossi SL, Soong L, Cai J, Menon R, Sun J. 2017. Outcomes of Congenital Zika disease depend on timing of infection and maternal-fetal interferon action. Cell Rep. 21(6):1588–1599. eng. doi:10.1016/j.celrep.2017.10.059
- Conroy A, Serghides L, Finney C, Owino SO, Kumar S, Gowda DC, Liles WC, Moore JM, Kain KC. 2009. C5a enhances dysregulated inflammatory and angiogenic responses to malaria in vitro: potential implications for placental malaria. PLoS One. 4(3):e4953. eng. doi:10.1371/journal.pone.0004953
- Conroy AL, McDonald CR, Silver KL, Liles WC, Kain KC. 2011. Complement activation: a critical mediator of adverse fetal outcomes in placental malaria? Trends Parasitol. 27(7):294–299. eng. doi:10.1016/j.pt.2011.02.005
- Conroy AL, Silver KL, Zhong K, Rennie M, Ward P, Sarma JV, Molyneux ME, Sled J, Fletcher JF, Rogerson S, et al. 2013. Complement activation and the resulting placental vascular insufficiency drives fetal growth restriction associated with placental malaria. Cell Host Microbe. 13(2):215–226. eng. doi:10.1016/j.chom.2013.01.010.
- Contopoulos-Ioannidis D, Newman-Lindsay S, Chow C, LaBeaud AD. 2018. Mother-to-child transmission of Chikungunya virus: A systematic review and meta-analysis. PLoS Negl Trop Dis. 12(6):e0006510. eng. doi:10.1371/journal.pntd.0006510
- Cordovez A, Prado V, Maggi L, Cordero J, Martinez J, Misraji A, Rios R, Soza G, Ojeda A, Levine MM. 1992. Enterohemorrhagic Escherichia coli associated with hemolytic-uremic syndrome in Chilean children. J Clin Microbiol. 30(8):2153–2157. eng. doi:10.1128/JCM.30.8.2153-2157.1992
- Corry J, Arora N, Good CA, Sadovsky Y, Coyne CB. 2017. Organotypic models of type III interferon-mediated protection from Zika virus infections at the maternal-fetal interface. Proc Natl Acad Sci U S A. 114(35):9433–9438. eng. doi:10.1073/pnas.1707513114
- Costa AA, Saldanha AC, Leite BM, Ramos B, Junior IA, Noronha AL, Bafica A, Barral A, Corbett CE, Costa JM. 2005. Imaging exams of bone lesions in patients with diffuse cutaneous leishmaniasis (DCL). Acta Trop. 96(1):9–15. eng. doi:10.1016/j.actatropica.2005.06.016
- Costa MA. 2016. The endocrine function of human placenta: an overview. Reprod Biomed Online. 32(1):14–43. eng. doi:10.1016/j.rbmo.2015.10.005
- Costa-da-Silva AL, Ioshino RS, Petersen V, Lima AF, Cunha MDP, Wiley MR, Ladner JT, Prieto K, Palacios G, Costa DD, et al. 2017. First report of naturally infected Aedes aegypti with chikungunya virus genotype ECSA in the Americas. PLoS Negl Trop Dis. 11(6):e0005630. eng. doi:10.1371/journal.pntd.0005630.
- Couderc T, Chretien F, Schilte C, Disson O, Brigitte M, Guivel-Benhassine F, Touret Y, Barau G, Cayet N, Schuffenecker I, et al. 2008. A mouse model for Chikungunya: young age and inefficient type-I interferon signaling are risk factors for severe disease. PLoS Pathog. 4(2):e29. eng. doi:10.1371/journal.ppat.0040029.
- D’Oliveira A Jr., Machado P, Bacellar O, Cheng LH, Almeida RP, Carvalho EM. 2002. Evaluation of IFN-gamma and TNF-alpha as immunological markers of clinical outcome in cutaneous leishmaniasis. Rev Soc Bras Med Trop. 35(1):7–10. eng. doi:10.1590/S0037-86822002000100002
- de Araújo TVB, Ximenes RAA, Miranda-Filho DB, Souza WV, Montarroyos UR, de Melo APL, Valongueiro S, de Albuquerque MFPM, Braga C, Filho SPB, 2018. Association between microcephaly, Zika virus infection, and other risk factors in Brazil: final report of a case-control study. Lancet Infect Dis. 18(3):328–336. eng. doi:10.1016/S1473-3099(17)30727-2.
- de Noronha L, Zanluca C, Burger M, Suzukawa AA, Azevedo M, Rebutini PZ, Novadzki IM, Tanabe LS, Presibella MM, CN DDS. 2018. Zika Virus Infection at different pregnancy stages: anatomopathological findings, target cells and viral persistence in placental tissues. Front Microbiol. 9:2266. eng. doi:10.3389/fmicb.2018.02266.
- Desjeux P. 1996. Leishmaniasis. Public Health Aspects and Control Clin Dermatol. 14(5):417–423, eng.
- Dotters-Katz SK, Grace MR, Strauss RA, Chescheir N, Kuller JA. 2015. Chikungunya fever: obstetric considerations on an emerging virus. Obstet Gynecol Surv. 70(7):453–457. eng. doi:10.1097/OGX.0000000000000184
- Driggers RW, Ho CY, Korhonen EM, Kuivanen S, Jaaskelainen AJ, Smura T, Rosenberg A, Hill DA, DeBiasi RL, Vezina G, et al. 2016. Zika Virus infection with prolonged maternal viremia and fetal brain abnormalities. N Engl J Med. 374(22):2142–2151. eng. doi:10.1056/NEJMoa1601824.
- Duaso J, Rojo G, Jana F, Galanti N, Cabrera G, Bosco C, Lopez-Munoz R, Maya JD, Ferreira J, Kemmerling U. 2011. Trypanosoma cruzi induces apoptosis in ex vivo infected human chorionic villi. Placenta. 32(5):356–361. eng. doi:10.1016/j.placenta.2011.02.005
- Economopoulou A, Dominguez M, Helynck B, Sissoko D, Wichmann O, Quenel P, Germonneau P, Quatresous I. 2009. Atypical Chikungunya virus infections: clinical manifestations, mortality and risk factors for severe disease during the 2005-2006 outbreak on Reunion. Epidemiol Infect. 137(4):534–541. eng. doi:10.1017/S0950268808001167
- Eltoum IA, Zijlstra EE, Ali MS, Ghalib HW, Satti MM, Eltoum B, AM E-H. 1992. Congenital kala-azar and leishmaniasis in the placenta. Am J Trop Med Hyg. 46(1):57–62. eng. doi:10.4269/ajtmh.1992.46.57
- Escobar M, Nieto AJ, Loaiza-Osorio S, Barona JS, Rosso F. 2017. Pregnant women hospitalized with chikungunya virus infection, Colombia, 2015. Emerg Infect Dis. 2311: eng. DOI:10.3201/eid2311.170480.
- Fernandez E, Dejnirattisai W, Cao B, Scheaffer SM, Supasa P, Wongwiwat W, Esakky P, Drury A, Mongkolsapaya J, Moley KH, et al. 2017. Human antibodies to the dengue virus E-dimer epitope have therapeutic activity against Zika virus infection. Nat Immunol. 18(11):1261–1269. eng. doi:10.1038/ni.3849.
- Franca GV, Schuler-Faccini L, Oliveira WK, Henriques CM, Carmo EH, Pedi VD, Nunes ML, Castro MC, Serruya S, Silveira MF, et al. 2016. Congenital Zika virus syndrome in Brazil: a case series of the first 1501 livebirths with complete investigation. Lancet. 388(10047):891–897. eng. doi:10.1016/S0140-6736(16)30902-3.
- Frank C, Werber D, Cramer JP, Askar M, Faber M, An der Heiden M, Bernard H, Fruth A, Prager R, Spode A, et al. 2011. Epidemic profile of Shiga-toxin-producing Escherichia coli O104: h4outbreak in Germany. N Engl J Med. 365(19):1771–1780. eng. doi:10.1056/NEJMoa1106483.
- Fried M, Domingo GJ, Gowda CD, Mutabingwa TK, Duffy PE. 2006. Plasmodium falciparum: chondroitin sulfate A is the major receptor for adhesion of parasitized erythrocytes in the placenta. Exp Parasitol. 113(1):36–42. eng. doi:10.1016/j.exppara.2005.12.003
- Fried M, Duffy PE. 1996. Adherence of Plasmodium falciparum to chondroitin sulfate A in the human placenta. Science. 272(5267):1502–1504. eng. doi:10.1126/science.272.5267.1502
- Fried M, Muga RO, Misore AO, Duffy PE. 1998. Malaria elicits type 1 cytokines in the human placenta: IFN-gamma and TNF-alpha associated with pregnancy outcomes. J Immunol. 160(5):2523–2530, eng.
- Fritel X, Rollot O, Gerardin P, Gauzere BA, Bideault J, Lagarde L, Dhuime B, Orvain E, Cuillier F, Ramful D, et al. 2010. Chikungunya virus infection during pregnancy, Reunion, France, 2006. Emerg Infect Dis. 16(3):418–425. eng. doi:10.3201/eid1604.091403.
- Gerardin P, Samperiz S, Ramful D, Boumahni B, Bintner M, Alessandri JL, Carbonnier M, Tiran-Rajaoefera I, Beullier G, Boya I, et al. 2014. Neurocognitive outcome of children exposed to perinatal mother-to-child Chikungunya virus infection: the CHIMERE cohort study on Reunion Island. PLoS Negl Trop Dis. 8(7):e2996. eng. doi:10.1371/journal.pntd.0002996.
- GBD 2015 Mortality and Causes of Death Collaborators. 2016.
- Global, regional, and national life expectancy, all-cause mortality, and cause-specific mortality for 249 causes of death, 1980-2015: a systematic analysis for the Global Burden of Disease Study 2015. Lancet. 388(10053):1459–1544. eng. doi:10.1016/S0140-6736(16)31012-1
- Gubler DJ. 2002. Epidemic dengue/dengue hemorrhagic fever as a public health, social and economic problem in the 21st century. Trends Microbiol. 10(2):100–103. eng. doi:10.1016/S0966-842X(01)02288-0
- Gurung P, Kanneganti TD. 2015. Innate immunity against Leishmania infections. Cell Microbiol. 17(9):1286–1294. eng. doi:10.1111/cmi.12484
- Hermanns K, Gohner C, Kopp A, Schmidt A, Merz WM, Markert UR, Junglen S, Drosten C. 2018. Zika virus infection in human placental tissue explants is enhanced in the presence of dengue virus antibodies in-vitro. Emerg Microbes Infect. 7(1):198. eng. doi:10.1038/s41426-018-0199-6
- Hussein HS. 2007. Prevalence and pathogenicity of Shiga toxin-producing Escherichia coli in beef cattle and their products. J Anim Sci. 85(13 Suppl):E63–72. eng. doi:10.2527/jas.2006-421
- Ibarra C, Amaral MM, Palermo MS. 2013. Advances in pathogenesis and therapy of hemolytic uremic syndrome caused by Shiga toxin-2. IUBMB Life. 65(10):827–835. eng. doi:10.1002/iub.1206
- Ismail MR, Ordi J, Menendez C, Ventura PJ, Aponte JJ, Kahigwa E, Hirt R, Cardesa A, Alonso PL. 2000. Placental pathology in malaria: a histological, immunohistochemical, and quantitative study. Hum Pathol. 31(1):85–93. eng. doi:10.1016/S0046-8177(00)80203-8
- Jurado KA, Simoni MK, Tang Z, Uraki R, Hwang J, Householder S, Wu M, Lindenbach BD, Abrahams VM, Guller S, et al. 2016. Zika virus productively infects primary human placenta-specific macrophages. JCI Insight. 1(13). eng. doi:10.1172/jci.insight.88461
- Kallur SD, Surapaneni T, Boorugu HK, Aziz N, Gala AR, Donnuri S. 2019. Need for guidelines for the combined management of pregnancy and dengue: a retrospective study from an Indian tertiary care maternity hospital. Trop Doct. 49(1):7–9. eng. doi:10.1177/0049475518800638
- Kam YW, Lee CY, Teo TH, Howland SW, Amrun SN, Lum FM, See P, Kng NQ, Huber RG, Xu MH, et al. 2017. Cross-reactive dengue human monoclonal antibody prevents severe pathologies and death from Zika virus infections. JCI Insight. 2(8). eng. doi:10.1172/jci.insight.92428
- Karmali MA, Petric M, Lim C, Fleming PC, Steele BT. 1983. Escherichia coli cytotoxin, haemolytic-uraemic syndrome, and haemorrhagic colitis. Lancet. 322(8362):1299–1300. eng. doi:10.1016/S0140-6736(83)91167-4
- Kemp MW. 2014. Preterm birth, intrauterine infection, and fetal inflammation. Front Immunol. 5:574. eng. doi:10.3389/fimmu.2014.00574.
- Krishnan L, Guilbert LJ, Russell AS, Wegmann TG, Mosmann TR, Belosevic M. 1996. Pregnancy impairs resistance of C57BL/6 mice to Leishmania major infection and causes decreased antigen-specific IFN-gamma response and increased production of T helper 2 cytokines. J Immunol. 156(2):644–652, eng.
- Laoprasopwattana K, Suntharasaj T, Petmanee P, Suddeaugrai O, Geater A. 2016. Chikungunya and dengue virus infections during pregnancy: seroprevalence, seroincidence and maternal-fetal transmission, southern Thailand, 2009-2010. Epidemiol Infect. 144(2):381–388. eng. doi:10.1017/S0950268815001065
- Lenglet Y, Barau G, Robillard PY, Randrianaivo H, Michault A, Bouveret A, Gerardin P, Boumahni B, Touret Y, Kauffmann E, et al. 2006. [Chikungunya infection in pregnancy: evidence for intrauterine infection in pregnant women and vertical transmission in the parturient. Survey of the Reunion Island outbreak]. J Gynecol Obstet Biol Reprod (Paris). 35(6):578–583. fre. doi:10.1016/S0368-2315(06)76447-X.
- Leparc-Goffart I, Nougairede A, Cassadou S, Prat C, de Lamballerie X. 2014. Chikungunya in the Americas. Lancet. 383(9916):514. eng. doi:10.1016/S0140-6736(14)60185-9
- Liang G, Gao X, Gould EA. 2015. Factors responsible for the emergence of arboviruses; strategies, challenges and limitations for their control. Emerg Microbes Infect. 4(1):e18. eng. doi:10.1038/emi.2015.18
- Lopez-Perez M, Pacheco MA, Buritica L, Escalante AA, Herrera S, Arevalo-Herrera M. 2016. Malaria in pregnancy: a passive surveillance study of pregnant women in low transmission areas of Colombia, Latin America. Malar J. 15:66. eng. doi:10.1186/s12936-016-1125-9.
- Low GC, Cooke WE. 1926. A congenital case of Kala-Azar. The Lancet. 208(5389):1209–1211. doi:10.1016/S0140-6736(01)05214-X.
- Luo H, Winkelmann ER, Fernandez-Salas I, Li L, Mayer SV, Danis-Lozano R, Sanchez-Casas RM, Vasilakis N, Tesh R, Barrett AD, et al. eng. 2018. Zika, dengue and yellow fever viruses induce differential anti-viral immune responses in human monocytic and first trimester trophoblast cells. Antiviral Res. 151:55–62. doi:10.1016/j.antiviral.2018.01.003.
- Ma J, Ketkar H, Geng T, Lo E, Wang L, Xi J, Sun Q, Zhu Z, Cui Y, Yang L, et al. eng. 2018. Zika virus non-structural protein 4A blocks the RLR-MAVS signaling. Front Microbiol. 9:1350. doi:10.3389/fmicb.2018.01350.
- Machado Filho AC, da Costa EP, Reis IS, Fernandes EA, Paim BV, Martinez-Espinosa FE. 2014. Effects of vivax malaria acquired before 20 weeks of pregnancy on subsequent changes in fetal growth. Am J Trop Med Hyg. 90(2):371–376. eng. doi:10.4269/ajtmh.13-0285
- Malária. 2019. The Global Found; [accessed 2019 Jul 24th]. https://www.theglobalfund.org/en/malaria/.
- Maspi N, Abdoli A, Ghaffarifar F. 2016. Pro- and anti-inflammatory cytokines in cutaneous leishmaniasis: a review. Pathog Glob Health. 110(6):247–260. eng. doi:10.1080/20477724.2016.1232042
- McDonald CR, Tran V, Kain KC. 2015. Complement activation in placental malaria. Front Microbiol. 6:1460. eng. doi:10.3389/fmicb.2015.01460.
- Medawar P. 1952. Some immunological and endocrinological problems raised by the evolution of viviparity in vertebrates. Symp Soc Exp Biol. 7:320–338.
- Meinecke CK, Schottelius J, Oskam L, Fleischer B. 1999. Congenital transmission of visceral leishmaniasis (Kala Azar) from an asymptomatic mother to her child. Pediatrics. 104(5):e65. eng. doi:10.1542/peds.104.5.e65
- Mescouto-Borges MR, Maues E, Costa DL, Pranchevicius MC, Romero GA. 2013. Congenitally transmitted visceral leishmaniasis: report of two Brazilian human cases. The Brazilian Journal of Infectious Diseases: An Official Publication of the Brazilian Society of Infectious Diseases. 17(2):263–266. eng. doi:10.1016/j.bjid.2012.10.017
- Messina JP, Brady OJ, Pigott DM, Golding N, Kraemer MU, Scott TW, Wint GR, Smith DL, Hay SI. 2015. The many projected futures of dengue. Nat Rev Microbiol. 13(4):230–239. eng. doi:10.1038/nrmicro3430
- Miner JJ, Cao B, Govero J, Smith AM, Fernandez E, Cabrera OH, Garber C, Noll M, Klein RS, Noguchi KK, et al. 2016. Zika Virus Infection during Pregnancy in Mice Causes Placental Damage and Fetal Demise. Cell. 165(5):1081–1091. eng. doi:10.1016/j.cell.2016.05.008.
- Mitropoulos P, Konidas P, Durkin-Konidas M. 2010. New World cutaneous leishmaniasis: updated review of current and future diagnosis and treatment. J Am Acad Dermatol. 63(2):309–322. eng. doi:10.1016/j.jaad.2009.06.088
- Mlakar J, Korva M, Tul N, Popovic M, Poljsak-Prijatelj M, Mraz J, Kolenc M, Resman Rus K, Vesnaver Vipotnik T, Fabjan Vodusek V, et al. 2016. Zika virus associated with microcephaly. N Engl J Med. 374(10):951–958. eng. doi:10.1056/NEJMoa1600651.
- Morgan DJ, Guimaraes LH, Machado PR, D’Oliveira A Jr., Almeida RP, Lago EL, Faria DR, Tafuri WL, Dutra WO, Carvalho EM. 2007. Cutaneous leishmaniasis during pregnancy: exuberant lesions and potential fetal complications. Clin Infect Dis. 45(4):478–482. eng. doi:10.1086/520017
- Muehlenbachs A, Fried M, McGready R, Harrington WE, Mutabingwa TK, Nosten F, Duffy PE. 2010. A novel histological grading scheme for placental malaria applied in areas of high and low malaria transmission. J Infect Dis. 202(10):1608–1616. eng. doi:10.1086/656723
- Nataro JP, Kaper JB. 1998. Diarrheagenic Escherichia coli. Clin Microbiol Rev. 11(1):142–201, eng.
- Nations WHOFaAOotU. 2018. Shiga toxin-producing Escherichia coli (STEC) and food: attribution, characterization, and monitoring: report.
- Naucke TJ, Lorentz S. 2012. First report of venereal and vertical transmission of canine leishmaniosis from naturally infected dogs in Germany. Parasit Vectors. 5:67. eng. doi:10.1186/1756-3305-5-67.
- Neu N, Duchon J, Zachariah P. 2015. TORCH infections. Clin Perinatol. 42(1):77–103. viii. eng. doi:10.1016/j.clp.2014.11.001
- Noronha L, Zanluca C, Azevedo ML, Luz KG, Santos CN. 2016. Zika virus damages the human placental barrier and presents marked fetal neurotropism. Mem Inst Oswaldo Cruz. 111(5):287–293. eng. doi:10.1590/0074-02760160085
- Nosten F, Ter Kuile F, Maelankirri L, Decludt B, White NJ. 1991. Malaria during pregnancy in an area of unstable endemicity. Trans R Soc Trop Med Hyg. 85(4):424–429. eng. doi:10.1016/0035-9203(91)90205-D
- Nunes P, Nogueira R, Coelho J, Rodrigues F, Salomao N, Jose C, de Carvalho J, Rabelo K. 2019. A stillborn multiple organs’ investigation from a maternal DENV-4 Infection: histopathological and inflammatory mediators characterization. Viruses. 11. 4.
- Nunes PC, Paes MV, de Oliveira CA, Soares AC, de Filippis AM, Lima Mda R, de Barcelos Alves AM, da Silva JF, de Oliveira Coelho JM, de Carvalho Rodrigues F, et al. 2016. Detection of dengue NS1 and NS3 proteins in placenta and umbilical cord in fetal and maternal death. J Med Virol. 88(8):1448–1452. eng. doi:10.1002/jmv.24479.
- Nuwayri-Salti NFKH. 1985. Direct non-insect-vector transmission of Leishmania parasites in mice. Int J Parasitol. 15(2):497–500. doi:10.1016/0020-7519(85)90043-8.
- Nyakundi PM, Muigai R, Were JB, Oster CN, Gachihi GS, Kirigi G. 1988. Congenital visceral leishmaniasis: case report. Trans R Soc Trop Med Hyg. 82(4):564. eng. doi:10.1016/0035-9203(88)90508-1
- Ogando DG, Paz D, Cella M, Franchi AM. 2003. The fundamental role of increased production of nitric oxide in lipopolysaccharide-induced embryonic resorption in mice. Reproduction. 125(1):95–110. eng. doi:10.1530/rep.0.1250095
- Ojha CR, Rodriguez M, Lapierre J, Muthu Karuppan MK, Branscome H, Kashanchi F, El-Hage N. 2018. Complementary mechanisms potentially involved in the pathology of Zika virus. Front Immunol. 92340: eng. DOI:10.3389/fimmu.2018.02340.
- Paes M, de Noronha L, Zanluca C, Burger M, Suzukawa AA, Azevedo M, Rebutini PZ, Novadzki IM, Tanabe LS, Presibella MM, et al. 2018. Zika virus infection at different pregnancy stages: anatomopathological findings, target cells and viral persistence in placental tissues. Viruses. 9:2266. eng.
- Pagliano P, Ascione T, Di Flumeri G, Boccia G, De Caro F. 2016. Visceral leishmaniasis in immunocompromised: diagnostic and therapeutic approach and evaluation of the recently released IDSA guidelines. Infez Med. 24(4):265–271, eng.
- Pagliano P, Carannante N, Rossi M, Gramiccia M, Gradoni L, Faella FS, Gaeta GB. 2005. Visceral leishmaniasis in pregnancy: a case series and a systematic review of the literature. J Antimicrob Chemother. 55(2):229–233. eng. doi:10.1093/jac/dkh538
- PAHO. 2019. Epidemiological Report of the Americas. World Health Organization; [accessed 2019 May 22]. http://iris.paho.org/xmlui/bitstream/handle/123456789/50505/Leishreport2019_eng.pdf?sequence=9&isAllowed=y.
- Paixao E, Teixeira M, Costa M, Barreto M, Rodrigues L. 2018a. Symptomatic Dengue during Pregnancy and Congenital Neurologic Malformations. Emerg Infect Dis. 24:1748–1750. doi:10.3201/eid2409.170361.
- Paixao ES, Harron K, Campbell O, Teixeira MG, Costa M, Barreto ML, Rodrigues LC. 2018. Dengue in pregnancy and maternal mortality: a cohort analysis using routine data. Sci Rep. 8(1):9938. eng. doi:10.1038/s41598-018-28387-w
- Palermo MS, Exeni RA, Fernandez GC. 2009. Hemolytic uremic syndrome: pathogenesis and update of interventions. Expert Rev Anti Infect Ther. 7(6):697–707. eng. doi:10.1586/eri.09.49
- Pangrazio KK, Costa EA, Amarilla SP, Cino AG, Silva TM, Paixao TA, Costa LF, Dengues EG, Diaz AA, Santos RL. 2009. Tissue distribution of Leishmania chagasi and lesions in transplacentally infected fetuses from symptomatic and asymptomatic naturally infected bitches. Vet Parasitol. 165(3–4):327–331. eng. doi:10.1016/j.vetpar.2009.07.013
- Park AY, Hondowicz B, Kopf M, Scott P. 2002. The role of IL-12 in maintaining resistance to Leishmania major. J Immunol. 168(11):5771–5777. eng. doi:10.4049/jimmunol.168.11.5771
- Petitt M, Tabata T, Puerta-Guardo H, Harris E, Pereira L. 2017. Zika virus infection of first-trimester human placentas: utility of an explant model of replication to evaluate correlates of immune protection ex vivo. Curr Opin Virol. 27:48–56. eng. doi:10.1016/j.coviro.2017.11.008.
- Platt DJ, Smith AM, Arora N, Diamond MS, Coyne CB, Miner JJ. 2018. Zika virus-related neurotropic flaviviruses infect human placental explants and cause fetal demise in mice. Sci Transl Med. 10(426): eng. DOI:10.1126/scitranslmed.aao7090.
- Priyamvada L, Quicke KM, Hudson WH, Onlamoon N, Sewatanon J, Edupuganti S, Pattanapanyasat K, Chokephaibulkit K, Mulligan MJ, Wilson PC, et al. 2016. Human antibody responses after dengue virus infection are highly cross-reactive to Zika virus. Proc Natl Acad Sci U S A. 113(28):7852–7857. eng. doi:10.1073/pnas.1607931113.
- Quicke KM, Bowen JR, Johnson EL, McDonald CE, Ma H, O’Neal JT, Rajakumar A, Wrammert J, Rimawi BH, Pulendran B, et al. 2016. Zika Virus Infects Human Placental Macrophages. Cell Host Microbe. 20(1):83–90. eng. doi:10.1016/j.chom.2016.05.015.
- Raa H, Grimmer S, Schwudke D, Bergan J, Walchli S, Skotland T, Shevchenko A, Sandvig K. 2009. Glycosphingolipid requirements for endosome-to-Golgi transport of Shiga toxin. Traffic. 10(7):868–882. eng. doi:10.1111/j.1600-0854.2009.00919.x
- Ramful D, Carbonnier M, Pasquet M, Bouhmani B, Ghazouani J, Noormahomed T, Beullier G, Attali T, Samperiz S, Fourmaintraux A, et al. 2007. Mother-to-child transmission of Chikungunya virus infection. Pediatr Infect Dis J. 26(9):811–815. eng. doi:10.1097/INF.0b013e3180616d4f.
- Ramos R, Viana R, Brainer-Lima A, FloreAncio T, Carvalho MD, van der Linden V, Amorim A, Rocha MA, Medeiros F. 2018. Perinatal Chikungunya Virus-associated Encephalitis Leading to Postnatal-Onset Microcephaly and Optic Atrophy. Pediatr Infect Dis J. 37(1):94–95. eng. doi:10.1097/INF.0000000000001690
- Regla-Nava JA, Elong Ngono A, Viramontes KM, Huynh AT, Wang YT, Nguyen AT, Salgado R, Mamidi A, Kim K, Diamond MS, et al. 2018. Cross-reactive Dengue virus-specific CD8(+) T cells protect against Zika virus during pregnancy. Nat Commun. 9(1):3042. eng. doi:10.1038/s41467-018-05458-0.
- Reithinger R, Dujardin JC. 2007. Molecular diagnosis of leishmaniasis: current status and future applications. J Clin Microbiol. 45(1):21–25. eng. doi:10.1128/JCM.02029-06
- Repetto HA. 1997. Epidemic hemolytic-uremic syndrome in children. Kidney Int. 52(6):1708–1719. eng. doi:10.1038/ki.1997.508
- Repetto HA. 2005. Long-term course and mechanisms of progression of renal disease in hemolytic uremic syndrome. Kidney Int Suppl. 68(97):S102–106. eng. doi:10.1111/j.1523-1755.2005.09717.x
- Ribeiro CF, Lopes VG, Brasil P, Coelho J, Muniz AG, Nogueira RM. 2013. Perinatal transmission of dengue: a report of 7 cases. J Pediatr. 163(5):1514–1516. eng. doi:10.1016/j.jpeds.2013.06.040
- Ribeiro CF, Lopes VGS, Brasil P, Pires ARC, Rohloff R, Nogueira RMR. 2017. Dengue infection in pregnancy and its impact on the placenta. Int J Infect Dis. 55:109–112. eng. doi:10.1016/j.ijid.2017.01.002.
- Riera C, Valladares JE. 1996. Viable Leishmania infantum in urine and semen in experimentally infected dogs. Parasitol Today. 12(10):412. eng. doi:10.1016/0169-4758(96)90062-9
- Rivas M, Chinen I, Miliwebsky E, Masana M. 2014. Risk Factors for Shiga Toxin-Producing Escherichia coli-Associated Human Diseases. Microbiol Spectr. 25: eng. DOI:10.1128/microbiolspec.EHEC-0002-2013.
- Robbins JR, Bakardjiev AI. 2012. Pathogens and the placental fortress. Curr Opin Microbiol. 15(1):36–43. eng. doi:10.1016/j.mib.2011.11.006
- Robbins JR, Skrzypczynska KM, Zeldovich VB, Kapidzic M, Bakardjiev AI. 2010. Placental syncytiotrophoblast constitutes a major barrier to vertical transmission of Listeria monocytogenes. PLoS Pathog. 6(1):e1000732, eng.
- Robbins JR, Zeldovich VB, Poukchanski A, Boothroyd JC, Bakardjiev AI. 2012. Tissue barriers of the human placenta to infection with Toxoplasma gondii. Infect Immun. 80(1):418–428. eng. doi:10.1128/IAI.05899-11
- Robinson MC. 1955. An epidemic of virus disease in Southern Province, Tanganyika Territory, in 1952-53. I. Clinical features. Trans R Soc Trop Med Hyg. 49(1):28–32. eng. doi:10.1016/0035-9203(55)90080-8
- Rodriguez-Morales AJ, Cardona-Ospina JA, Ramirez-Jaramillo V, Gaviria JA, Gonzalez-Moreno GM, Castrillon-Spitia JD, Lopez-Villegas A, Morales-Jimenez E, Ramirez-Zapata V, Rueda-Merchan GE, et al. 2018. Diagnosis and outcomes of pregnant women with Zika virus infection in two municipalities of Risaralda, Colombia: second report of the ZIKERNCOL study. Travel Med Infect Dis. 25:20–25. eng.
- Rogerson SJ. 2017. Management of malaria in pregnancy. Indian J Med Res. 146(3):328–333. eng. doi:10.4103/ijmr.IJMR_1304_17
- Rogerson SJ, Pollina E, Getachew A, Tadesse E, Lema VM, Molyneux ME. 2003. Placental monocyte infiltrates in response to Plasmodium falciparum malaria infection and their association with adverse pregnancy outcomes. Am J Trop Med Hyg. 68(1):115–119. eng. doi:10.4269/ajtmh.2003.68.1.0680115
- Romero I, Tellez J, Suarez Y, Cardona M, Figueroa R, Zelazny A, Gore Saravia N. 2010. Viability and burden of Leishmania in extralesional sites during human dermal leishmaniasis. PLoS Negl Trop Dis. 49: eng. DOI:10.1371/journal.pntd.0000819.
- Romero RCT. 2002. Preterm labor, intrauterine infection, and the fetal inflammatory response syndrome. NeoReviews. 3:5. doi:10.1542/neo.3-5-e73.
- Rosenberg AZ, Yu W, Hill DA, Reyes CA, Schwartz DA. 2017. Placental Pathology of Zika Virus: viral Infection of the Placenta Induces Villous Stromal Macrophage (Hofbauer Cell) Proliferation and Hyperplasia. Arch Pathol Lab Med. 141(1):43–48. eng. doi:10.5858/arpa.2016-0401-OA
- Rosypal AC, Troy GC, Zajac AM, Frank G, Lindsay DS. 2005. Transplacental transmission of a North American isolate of Leishmania infantum in an experimentally infected beagle. J Parasitol. 91(4):970–972. eng. doi:10.1645/GE-483R.1
- Sacerdoti F, Amaral MM, Aisemberg J, Cymeryng CB, Franchi AM, Ibarra C. 2015. Involvement of hypoxia and inflammation in early pregnancy loss mediated by Shiga toxin type 2. Placenta. 36(6):674–680. eng. doi:10.1016/j.placenta.2015.03.005
- Sacerdoti F, Amaral MM, Zotta E, Franchi AM, Ibarra C. 2014. Effects of Shiga toxin type 2 on maternal and fetal status in rats in the early stage of pregnancy. Biomed Res Int. 2014:384645. eng. doi:10.1155/2014/384645.
- Sacerdoti F, Scalise ML, Burdet J, Amaral MM, Franchi AM, Ibarra C. 2018. Shiga Toxin-Producing Escherichia coli Infections during Pregnancy. Microorganisms. 64: eng. DOI:10.3390/microorganisms6040111.
- Sacks D, Noben-Trauth N. 2002. The immunology of susceptibility and resistance to Leishmania major in mice. Nat Rev Immunol. 2(11):845–858. eng. doi:10.1038/nri933
- Salles TS, da Encarnacao Sa-guimaraes T, ESL DA, Guimaraes-Ribeiro V, MDF DM, de Castro-salles PF, Dos Santos CR, Do Amaral Melo AC, Soares MR, Ferreira DF, et al. 2018. History, epidemiology and diagnostics of dengue in the American and Brazilian contexts: a review. Parasit Vectors. 11(1):264. eng. doi:10.1186/s13071-018-2830-8.
- Scalise ML, Amaral MM, Reppetti J, Damiano AE, Ibarra C, Sacerdoti F. 2019. Cytotoxic effects of Shiga toxin-2 on human extravillous trophoblast cell lines. Reproduction. 157(3):297–304. eng. doi:10.1530/REP-18-0581
- Schneider H, Miller RK. 2010. Receptor-mediated uptake and transport of macromolecules in the human placenta. Int J Dev Biol. 54(2–3):367–375. eng. doi:10.1387/ijdb.082773hs
- Schwartz DA. 2017. Viral infection, proliferation, and hyperplasia of Hofbauer cells and absence of inflammation characterize the placental pathology of fetuses with congenital Zika virus infection. Arch Gynecol Obstet. 295(6):1361–1368. eng. doi:10.1007/s00404-017-4361-5
- Scott P, Novais FO. 2016. Cutaneous leishmaniasis: immune responses in protection and pathogenesis. Nat Rev Immunol. 16(9):581–592. eng. doi:10.1038/nri.2016.72
- Senanayake MP, Senanayake SM, Vidanage KK, Gunasena S, Lamabadusuriya SP. 2009. Vertical transmission in chikungunya infection. Ceylon Med J. 18(3):47–50. eng. doi:10.4038/cmj.v54i2.865
- Sharma JB, Gulati N. 1992. Potential relationship between dengue fever and neural tube defects in a northern district of India. Int J Gynaecol Obstet. 39(4):291–295. eng. doi:10.1016/0020-7292(92)90260-P
- Sheridan MA, Balaraman V, Schust DJ, Ezashi T, Roberts RM, Franz AWE. 2018. African and Asian strains of Zika virus differ in their ability to infect and lyse primitive human placental trophoblast. PLoS One. 13(7):e0200086. eng. doi:10.1371/journal.pone.0200086
- Sheridan MA, Yunusov D, Balaraman V, Alexenko AP, Yabe S, Verjovski-Almeida S, Schust DJ, Franz AW, Sadovsky Y, Ezashi T, et al. 2017. Vulnerability of primitive human placental trophoblast to Zika virus. Proc Natl Acad Sci U S A. 114(9):E1587–E1596. eng. doi:10.1073/pnas.1616097114.
- Silva FL, Oliveira RG, Silva TM, Xavier MN, Nascimento EF, Santos RL. 2009. Venereal transmission of canine visceral leishmaniasis. Vet Parasitol. 160(1–2):55–59. eng. doi:10.1016/j.vetpar.2008.10.079
- Souza RM, Ataide R, Dombrowski JG, Ippolito V, Aitken EH, Valle SN, Alvarez JM, Epiphanio S, Marinho CR. 2013. Placental histopathological changes associated with Plasmodium vivax infection during pregnancy. PLoS Negl Trop Dis. 7(2):e2071. eng. doi:10.1371/journal.pntd.0002071
- Steele BT, Goldie J, Alexopoulou I, Shimizu A. 1984. Post-partum haemolytic-uremic syndrome and verotoxin-producing Escherichia coli. Lancet. 323(8375):511. eng. doi:10.1016/S0140-6736(84)92877-0
- Stritt A, Tschumi S, Kottanattu L, Bucher BS, Steinmann M, von Steiger N, Stephan R, Hachler H, Simonetti GD. 2013. Neonatal hemolytic uremic syndrome after mother-to-child transmission of a low-pathogenic stx2b harboring shiga toxin-producing Escherichia coli. Clin Infect Dis. 56(1):114–116. eng. doi:10.1093/cid/cis851
- Suguitan AL Jr., Leke RG, Fouda G, Zhou A, Thuita L, Metenou S, Fogako J, Megnekou R, Taylor DW. 2003. Changes in the levels of chemokines and cytokines in the placentas of women with Plasmodium falciparum malaria. J Infect Dis. 188(7):1074–1082. eng. doi:10.1086/378500
- Suwanmanee S, Luplertlop N. 2017. Dengue and Zika viruses: lessons learned from the similarities between these Aedes mosquito-vectored arboviruses. J Microbiol. 55(2):81–89. eng. doi:10.1007/s12275-017-6494-4
- Symmers WS. 1960. Leishmaniasis acquired by contagion: a case of marital infection in Britain. Lancet. 1(7116):127–132. eng. doi:10.1016/S0140-6736(60)90052-0
- Tabata T, Petitt M, Puerta-Guardo H, Michlmayr D, Wang C, Fang-Hoover J, Harris E, Pereira L. 2016. Zika Virus Targets Different Primary Human Placental Cells, Suggesting Two Routes for Vertical Transmission. Cell Host Microbe. 20(2):155–166. eng. doi:10.1016/j.chom.2016.07.002
- Tanaka H, Toyoda N, Adachi E, Takeda T. 2000. Immunologic evaluation of an Escherichia coli O157-infected pregnant woman. A case report. J Reprod Med. 45(5):442–444, eng.
- The United Nations Development Program (UNDP). 2019. [accessed 2019 May 15th]. https://www.undp.org/content/undp/en/home/sustainable-development-goals/goal-3-good-health-and-well-being.html.
- Thomas DE, Elliott EJ. 2013. Interventions for preventing diarrhea-associated hemolytic uremic syndrome: systematic review. BMC Public Health. 13:799. eng. doi:10.1186/1471-2458-13-799.
- Torres AG, Amaral MM, Bentancor L, Galli L, Goldstein J, Kruger A, Rojas-Lopez M. 2018. Recent Advances in Shiga Toxin-Producing Escherichia coli Research in Latin America. Microorganisms. 6(4):100. eng. doi:10.3390/microorganisms6040100
- Torres JR, Falleiros-Arlant LH, Duenas L, Pleitez-Navarrete J, Salgado DM, Castillo JB. 2016. Congenital and perinatal complications of chikungunya fever: a Latin American experience. Int J Infect Dis. 51:85–88. eng. doi:10.1016/j.ijid.2016.09.009.
- Ulinski T, Lervat C, Ranchin B, Gillet Y, Floret D, Cochat P. 2005. Neonatal hemolytic uremic syndrome after mother-to-child transmission of Escherichia coli O157. Pediatr Nephrol. 20(9):1334–1335. eng. doi:10.1007/s00467-005-1871-3
- Varela G, Chinen I, Gadea P, Miliwebsky E, MI M, Gonzalez S, Gonzalez G, MJ G, CC C, Algorta G, et al. 2008. [Detection and characterization of Shiga toxin-producing Escherichia coli from clinical cases and food in Uruguay]. Rev Argent Microbiol. 40(2):93–100. spa.
- Villamil-Gomez WE, Guijarro E, Castellanos J, Rodriguez-Morales AJ. 2017. Congenital Zika syndrome with prolonged detection of Zika virus RNA. J Clin Virol. 95:52–54. eng. doi:10.1016/j.jcv.2017.08.010.
- Von Stebut E, Ehrchen JM, Belkaid Y, Kostka SL, Molle K, Knop J, Sunderkotter C, Udey MC. 2003. Interleukin 1alpha promotes Th1 differentiation and inhibits disease progression in Leishmania major-susceptible BALB/c mice. J Exp Med. 198(2):191–199. eng. doi:10.1084/jem.20030159
- Wahid B, Ali A, Rafique S, Idrees M. 2017. Global expansion of chikungunya virus: mapping the 64-year history. Int J Infect Dis. 58:69–76. eng. doi:10.1016/j.ijid.2017.03.006.
- Walker PG, Ter Kuile FO, Garske T, Menendez C, Ghani AC. 2014. Estimated risk of placental infection and low birthweight attributable to Plasmodium falciparum malaria in Africa in 2010: a modelling study. The Lancet Global Health. 2(8):e460–467. eng. doi:10.1016/S2214-109X(14)70256-6
- Weisblum Y, Oiknine-Djian E, Vorontsov OM, Haimov-Kochman R, Zakay-Rones Z, Meir K, Shveiky D, Elgavish S, Nevo Y, Roseman M, et al. 2017. Zika Virus Infects Early- and Midgestation Human Maternal Decidual Tissues, Inducing Distinct Innate Tissue Responses in the Maternal-Fetal Interface. J Virol. 91(4). eng. doi:10.1128/JVI.01905-16
- WHO. 2019. Update on the e-2020 initiative of 21 malaria-eliminating countries. Geneva, Switzerland: World Health Organization. (WHO/CDS/GMP/2018.10).
- WHO. 2017. Integrating neglected tropical diseases into global health and development: fourth WHO report on neglected tropical diseases. Geneva, Switzerland: World Health Organization. (Licence: CC BY-NC-SA 3.0 IGO.).
- Wilder-Smith A. 2019. The first licensed dengue vaccine: can it be used in travelers? Curr Opin Infect Dis. 32(5):394–400. eng. doi:10.1097/QCO.0000000000000573
- Willis E, Hensley SE. 2017. Characterization of Zika virus binding and enhancement potential of a large panel of flavivirus murine monoclonal antibodies. Virology. 508:1–6. eng. doi:10.1016/j.virol.2017.04.031.
- Xavier-Neto J, Carvalho M, Pascoalino BD, Cardoso AC, Costa AM, Pereira AH, Santos LN, Saito A, Marques RE, Smetana JH, et al. 2017. Hydrocephalus and arthrogryposis in an immunocompetent mouse model of ZIKA teratogeny: A developmental study. PLoS Negl Trop Dis. 11(2):e0005363. eng. doi:10.1371/journal.pntd.0005363.
- Yoshimura K, Fujii J, Tanimoto A, Yutsudo T, Kashimura M, Yoshida S. 2000. Effects of Shiga toxin 2 on lethality, fetuses, delivery, and puerperal behavior in pregnant mice. Infect Immun. 68(4):2254–2258. eng. doi:10.1128/IAI.68.4.2254-2258.2000
- Yoshimura K, Tanimoto A, Abe T, Ogawa M, Yutsudo T, Kashimura M, Yoshida S. 2002. Shiga toxin 1 and 2 induce apoptosis in the amniotic cell line WISH. J Soc Gynecol Investig. 9(1):22–26. eng. doi:10.1177/107155760200900105
- Zeldovich VB, Bakardjiev AI. 2012. Host defense and tolerance: unique challenges in the placenta. PLoS Pathog. 8(8):e1002804. eng. doi:10.1371/journal.ppat.1002804
- Zinchuk A, Nadraga A. 2010. Congenital visceral leishmaniasis in Ukraine: case report. Ann Trop Paediatr. 30(2):161–164. eng. doi:10.1179/146532810X12703902516400
- Zulu MZ, Martinez FO, Gordon S, Gray CM. 2019. The Elusive Role of Placental Macrophages: the Hofbauer Cell. J Innate Immun. 11(6):447–456. eng. doi:10.1159/000497416