Abstract
We investigated N-glycan processing of immunoglobulin G1 using the monoclonal antibody cetuximab (CxMab), which has a glycosite in the Fab domain in addition to the conserved Fc glycosylation, as a reporter. Three GlcNAc (Gn) terminating bi-antennary glycoforms of CxMab differing in core fucosylation (α1,3- and α1,6-linkage) were generated in a plant-based expression platform. These GnGn, GnGnF3, and GnGnF6 CxMab variants were subjected in vivo to further processing toward sialylation and GlcNAc diversification (bisected and branching structures). Mass spectrometry-based glycan analyses revealed efficient processing of Fab glycans toward envisaged structures. By contrast, Fc glycan processing largely depend on the presence of core fucose. A particularly strong support of glycan processing in the presence of plant-specific core α1,3-fucose was observed. Consistently, molecular modeling suggests changes in the interactions of the Fc carbohydrate chain depending on the presence of core fucose, possibly changing the accessibility. Here, we provide data that reveal molecular mechanisms of glycan processing of IgG antibodies, which may have implications for the generation of glycan-engineered therapeutic antibodies with improved efficacies.
Abbreviations
3-FucT | = | Zea maize core α1,3-fucosyltransferase |
3-FucTGnTIV | = | human α1,3-mannosyl-β1,4-N-acetyl-glucosaminyltransferase fused to the CTS region of the Arabidopsis thaliana core α1,3-fucosyltransferase (FUT11) |
6-FucT | = | Mus musculus core α1,6-fucosyltransferase |
CH2 | = | constant domain of an IgG heavy chain |
CTS | = | cytoplasmic tail, transmembrane domain and stem region |
CxMab | = | cetuximab (Erbitux®) |
Fab | = | fragment antigen-binding |
Fc | = | Fragment crystallizable region of immunoglobulin G |
GlcNAc | = | N-acetylglucosamine |
IgG1 | = | Immunoglobulin G subclass 1 |
LC-ESI-MS | = | Liquid chromatography–electrospray ionisation–mass spectrometry |
mAb | = | monoclonal antibody |
SDS-PAGE | = | Sodium dodecyl sulfate polyacrylamide gel electrophoresis |
STGalT | = | β1,4-galactosyltransferase fused to the CTS region of the rat α2,6-sialyltransferase |
6-SiaT | = | α2,6-sialyltransferase |
STGnT-III | = | β1,4-mannosyl-β1,4-N-acetylglucosaminyltransferase fused to the CTS region of the rat α2,6-sialyltransferase |
ΔXT/FT | = | Nicotiana benthamiana glycosylation mutants lacking plant specific core β1,2- xylose and α1,3-fucose residues |
Introduction
It is well known that immunoglobulins (Ig) circulate as a highly heterogeneously glycosylated mixture (microheterogeneity) of an otherwise homogeneous protein backbone, which points to the multiple functions of these proteins. This microheterogeneity may comprise several hundred glycoforms, and it is mainly owed to the presence or absence of sialic acid, galactose, core fucose and bisecting N-acetylglucosamine (GlcNAc). IgG, the simplest Ig isoform, contains a single N-glycosylation site in the constant domain (Fc), representing a conserved site in most Ig classes. The Fc-linked carbohydrates are complex-type bi-antennary N-glycans with high levels of core fucosylation (>80%) and a variable number of galactose residues. A striking difference of serum IgG Fc glycans to other Igs is the low amount of sialylation, 10% vs ∼50%.Citation1,2
Many reports have described variations of IgG Fc glycosylation, especially of the degree of galactosylation, related to diseases and various other physiological changes like age and pregnancy.Citation3 It was found that an absence of sialic acids and low levels of galactosylation might confer important pro-inflammatory properties to IgG.Citation4 Similarly, the absence of core fucose improved the affinity of the Fc to Fcγ receptors (e.g., FcγIII), thereby enhancing antibody-dependent cellular cytotoxicity.Citation5,6 On this basis, glyco-engineered antibodies carrying afucosylated Fc glycans are currently in clinical development.Citation7,8
Besides the conserved N-glycosylation sites on the Fc portion, additional carbohydrate chains can be linked to the hypervariable regions of Ig (“asymmetric antibodies”). For instance, up to 25% of IgG molecules isolated from the serum of healthy human subjects have been reported to carry N-glycans on their variable domains.Citation9,10 The amount of “asymmetric” IgG was found to increase during pregnancy, as well as after the treatment of antibody-producing cells with hormones and cytokines.Citation11,12
Fab-linked glycans from human serum IgG exhibit primarily complex-type bi-antennary N-glycans with high contents of core fucose (80%), bisecting GlcNAc (50%), and sialic acid (80%).Citation9 Depending on their structures and locations, the Fab glycans may influence IgG effector functions by increasing or decreasing the affinity for the antigen.Citation1 One report suggests that Fab glycosylation could modulate antibody half-life.Citation13
A common obstacle that hampers detailed research on the relationship of Ig glycosylation to functional activities is the incomplete knowledge of how glycan structures are generated and the availability of expression platforms that allow the synthesis of targeted glycoforms. Several expression systems, including mono and multicellular organisms, were developed to address this issue.Citation3,14 Plants appear particularly well suited for the generation of human proteins, including antibodies, with a designed glycosylation profile.Citation14,15 Compelling features are speed and flexibility by which monoclonal antibodies (mAbs) with defined glycosylation profiles can be produced.Citation15 Notably, the system is used for the production of clinical grade ZMAPP, a glycan-engineered antibody cocktail for Ebola treatments.Citation8
Here, we aimed to elucidate factors that contribute to the processing of glycan structures in IgG-based antibodies. We chose cetuximab as a model because it carries 2 glycosylation sites, with one in the Fc and one in the Fab region. A plant-based expression system was used to generate different glycoforms. Glyco-variants terminate with GlcNAc residues, but differ in their core fucosylation (no fucose, α1,3- and α1,6-linkage, referred to as GnGn, GnGnF3 and GnGnF6, respectively). These variants were individually used to co-express, in plants, glycosylation enzymes that catalyze sialylation, branching and bisected structures. Mass spectrometry-based glycan analyses revealed efficient processing of Fab glycans by all enzymes. In contrast, Fc glycan processing largely depended on the presence of core fucose. We observed a particularly strong promotion of glycan processing in the presence of plant-specific core α1,3-fucose. Moreover, computer modeling of Fc glycans point to changes in the interactions of Fc carbohydrates upon adding core fucose, corroborating experimental data.
Results
Generation of cetuximab glycoforms in N. benthamiana
The approved mAb cetuximab (CxMab, Erbitux®) was used in a case study for glycan processing of IgG-based antibodies. This mouse-human hybrid mAb is particularly suited because it carries the conserved glycosylation site at the Fc-CH2 domain, and one in the variable region of the heavy chain (Fab). shows a 3-dimensional representation of the antibody based on crystal structures of separate Fc and Fab fragments. The glycan structures were modeled to the Fab structure by superposition of the anchoring asparagine. While glycans connected to the Fab fragment are exposed to the surrounding solvent, the structures located in the Fc fragment are largely shielded by the opposing CH2 fragment. The two glycosites of CxMab produced in mammalian cells (SP2/0 murine myeloma cell) are glycosylated with substantial differences. While Fc carries mainly complex N-glycans terminating with GlcNAc and galactose residues, Fab exhibits predominantly sialylated structures.Citation16,17 Nearly all glycans are core α1,6-fucosylated.
Figure 1. Model of cetuximab constructed from the Fc-fragment with PDB code 4BYH and the Fab-fragments with PDB code 1YY8. The representation of the glycan structure connected to Fab on the variable heavy (VH) chain was modeled using Pymol (Schrödinger, L. L. C. The PyMOL Molecular Graphics System). Heavy chain in dark (Fc) and light (Fab) gray, light chain in gold, glycan structures colored according to Consortium of Functional Glycomics.
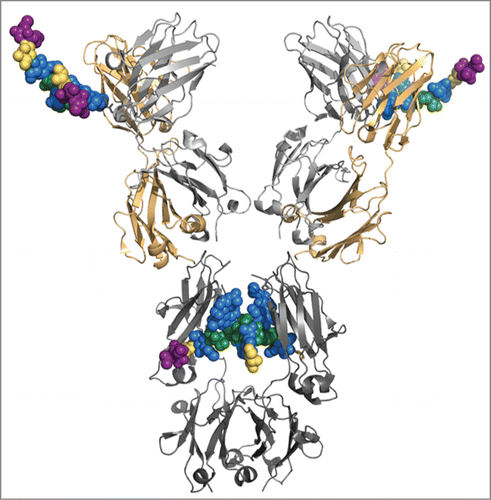
In contrast to mammalian cells that synthesize core fucosylation in α1,6-linkage, plants carry α1,3-linked core fucose. Here, we transiently expressed CxMab in Nicotiana benthamiana, a tobacco-related plant species, widely used for recombinant protein expression.Citation18 In particular, the Nicotiana benthamiana glycosylation mutant ΔXT/FT synthesizing human type bi-antennary complex glycans, i.e., GnGn structures, served as the expression platform.Citation19 In higher eukaryotes, this structure provides the central substrate for further glycan diversifications, including fucosylation, branching and sialylation. Liquid-chromatography-electrospray ionization-mass spectrometry (LC-ESI-MS) was used to determine the N-glycosylation profile of purified CxMab expressed in ΔXT/FT plants. At both glycan sites, a single carbohydrate species, GnGn, was detected (, Fig. S1). Notably, some core α1,3-fucosylated GnGn (∼30%) arose at 88N, pointing to a leaky knock-down of the core α1,3-fucosyltransferase in ΔXT/FT plants. Upon co-expression of CxMab with maize α1,3-fucosyltransferase (3-FucT) and mouse α1,6-fucosyltransferase (6-FucT), GnGnF3 and GnGnF6 structures, respectively, were efficiently generated (, Fig. S1). Both CxMab glycosites were glycosylated in a similar way. In the 6-FucT line, some core α1,3-fucosylated structure (∼20%) were observed in Fab-glycosite due to leaky knock-down of the fucosyltransferase resulting in double core fucosylated glycans (GnGnF3F6). In addition, CxMab was expressed in Nicotiana benthamiana wild type plants (WT), generating plant specific core glycosylation, i.e., β1,2-xylose and α 1,3-fucose (Fig. S1). Plant-typical GnGnXF3 structures were detected at both glycan sites (Fig. S1). In summary, the system allowed the generation of CxMab glycoforms that differed in their core fucosylation.
Figure 2. Relative abundance of cetuximab glycoforms upon co-expression of the mAb with different glycan processing enzymes. (A) Fab and Fc glycans of CxMab expressed in ΔXT/FT (Fab-, Fc-), with α1,3- (Fab-F3, Fc-F3) and α1,6- fucosyltransferase (Fab-F6, Fc-F6). (B) CxMab co-expressed in the 3 platforms described in A with the human sialylation pathway (Sia).Citation20 (C) CxMab co-expressed in the 3 platforms described in A with N-acetylglucosaminyltransferase III (GnT-III). Glycan profiles were determined by LC-ESI-MS. Tryptic glycopeptides of Fab domain (K/M82NSLQSNDTAIYYCAR97) and Fc domain (R/E295EQYNSTYR303) were analyzed. Different shades of blue, green and red represent percentages of non-fucosylated, α1,3- and α1,6-fucosylated structures, respectively. Gray shade represent fraction of non-converted complex glycans (non conv.). Schematic representations of N-glycans structures detected in this study are shown. Nomenclature is in accordance to ProGlycAn (www.proglycan.com). Each glycan analysis was performed at least in 4 independent experiments with no substantial variation (<5%) of the relative abundance of glycoforms. For more detailed information see also Table S1 and Figs. S1–S3.
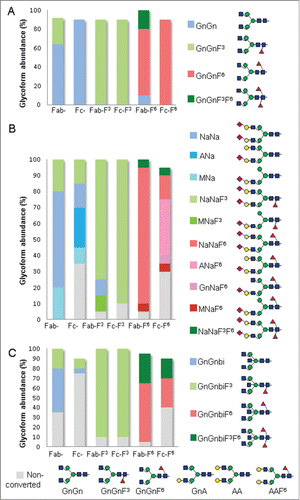
Generation of sialylated cetuximab
We have previously shown that the Nicotiana benthamiana-based expression platform is well suited to produce antibodies with sialylated structures.Citation20 Co-expression of CxMab with the human sialylation (Sia) pathway using ΔXT/FT plants led to differences of the glycan composition between the Fab and the Fc sites. On 88N, virtually all glycans were sialylated, with 80% di-sialo glycans (NaNa, NaNaF3) accompanied by the truncated glycoform MNa, a recurrence of hybrid-type structure (, Table S1 and Fig. S2). Sialylation of Fc was less efficient compared to the Fab counterpart. About 35% complex non-sialylated structures (GnGn, GnA, AA) were present (, Table S1 and Fig. S2). Sialylated structures were detected in a ratio of 50:50% to mono- (MNA, ANa) and di-sialylated (NaNa, NaNaF3) forms.
In contrast to the homogeneous glycan profiles obtained so far, a largely heterogeneous glycosylation of 7–9 structures was generated in the Fc glycosite. A similarly heterogeneous Fc-glycosylation profile was obtained by co-expression of CxMab with the genes for the Sia pathway and 6-FucT. At least 8 fucosylated glycoforms were detected on Fc-glycosite, ∼30% non-sialylated (GnGnF6, GnAF6, AAF6), 45% mono- (MNaF6, GnNaF6 and ANaF6) and 20% di-sialylated (NaNaF6 and NaNaF3F6). By contrast, high amounts of fucosylated di-sialylated structures (90%) were visible on the corresponding Fab-glycosite. Notably, a large amount of fucosylated di-sialylated structures (80–90%, Table S1) was generated by co-expression with 3-FucT (NaNaF3) at both glyco sites. Interestingly, Fab-glycosite carried mainly fucosylated di- (∼80%, NaNaF) and mono-sialylated (5–10%, MNaF) structures, irrespective whether core α1,6- or α1,3-fucose was present (, Table S1 and Fig. S2). Co-expression of CxMab in WT plants with the Sia pathway resulted in homogenous glycosylation with di-sialylated (NaNaXF3) as the dominating form in both glycosites (Fig. S2). Our data point at improved processing of the Fc glycosylation toward di-sialylated structures in the presence of core α 1,3-fucose.
Effect of core fucosylation on the synthesis of other N-glycans
To further analyze possible effects of core fucosylation on glycan processing, we aimed to generate bisecting N-acetylglucosamine, a glycoform present in substantial amounts (∼30%) in serum IgG.Citation16 We co-expressed CxMab with a modified version of human N-acetylglucosaminyltransferase III (STGnT-III) that targets the enzyme to a late Golgi compartment using the expression procedures described above. While structures present on the Fab glycosite (GnGn, GnGnF3, GnGnF6) were efficiently converted into the bisected counterparts (GnGnbi, GnGnF3bi, GnGnF6bi), this process was only partially successful on the Fc glycosite (, Table S1 and Fig. S3). Here, only structures that carried core α1,3-fucosylation (GnGnF3) were quantitatively processed into bisected forms (, Table S1 and Fig. S3). GnGn and GnGnF6 structures on Fc-glycosite were inefficiently processed and substantial amounts remained unaffected (75% and 40%, respectively). The results point to a strong support of core fucosylation, particularly core α1,3-fucosylation, for the generation of bisected glycoforms. Moreover, the process acts in a site-specific manner.
A similar result was obtained upon co-expression of CxMab with a modified version of human N-acetylglucosaminetransferase IV (3-FucTGnT-IV) that generates tri-antennary glycans (Fig. S4). While GnGn, GnGnF,3 GnGnF6 on Fab-glycosite were efficiently converted into tri-antennary structures (Gn[GnGn], Gn[GnGn]F3 and Gn[GnGn]F6) (30–80%), only α1,3-fucose carrying glycans were efficiently processed at Fc-glycosite (90%, Fig. S4).
The support of core fucosylation toward generation of bisected GlcNAc oligosaccharides is particularly interesting since bisected forms on the other hand inhibit the synthesis of central reactions of the biosynthetic pathway such as core α1,6-fucosylation.Citation21,22 Here, we monitored whether this also holds for core α1,3-fucose. Therefore, a GnGnbi glycopeptide was incubated with recombinant core α1,3-FucT. No processing of GnGnbi was observed (Fig. S5A), while in the control experiment the GnGnF3 glycopeptide was efficiently converted into bisected structures upon exposure to recombinant GnT-III (Fig. S5B).
Effect of core fucosylation on in vitro sialylation of galactosylated antibodies
The initial observation that expression of the Sia pathway including rat α2,6-sialyltransferase (6-SiaT) led to sialylated and core α1,3-fucosylated Fc structures in ΔXT/FT could be explained either by genetic interference or by facilitated sialylation as a consequence of Fc-fucosylation. To further investigate the effect of core 3-fucosylation on sialylated Fc glycans, an in vitro setting was chosen. Three glycoforms of human Fc-IgG1 were generated (AA, AAF3 and AAF6) and incubated with recombinant 6-SiaT (r6-SiaT). r6-SiaT was added in a ratio that converts two-thirds of the Fc AA structures into sialylated glycans. MS analysis of the Fc-glycopeptide revealed the presence of similar amounts of mono- and di-sialylated structures on Fc carrying AA and AAF6, both substrates carried about 60% mono- and 10% di-sialylated structures (Fig. S6). By contrast, when AAF3 served as acceptor an increased level of di-sialylated structures (40%) was synthesized.
Structural models of cetuximab
To assess potential conformational differences associated with glycosylation, a molecular model based on previously published Fc structural information was generated. compares 2 crystal structures of the Fc fragment including the glycan structures. The structure with Protein Data Bank (PDB) code 3SGK (magenta) was co-crystallized with FcγRIII;6 the structure with PDB code 4BYH (gray) is sialylated.Citation23 It is clear from this figure that the CH2-domains are closer together in the latter structure. We hypothesize that interactions between the CH2 domains help to orient the glycan structures optimally for processing by the sialic acid transferase, while a weaker interaction between the CH2 domains leads to more diverse conformations of the glycan structures, reducing the accessibility for the appropriate transferase.
Figure 3. Molecular model of Fc glycans. (A) Overlay of crystal structures with PDB code 3SGK (magenta) and 4BYH (gray). (B) Crystal structure with PDB code 4BYH in which the α1,3-fucose moiety (orange) was modeled in using the Molecular Operating Environment (MOE; Chemical Computing Group Inc., 2014). All other carbohydrates are colored as in . (C) Close-up of the α1,3-fucose glycan with 2 hydrogen bonds formed between the 2 opposing strands (arrowed dashed black lines). (D) Close-up of the α1,6-fucose glycan with one hydrogen bond formed between the 2 opposing strands (arrowed dashed black line).
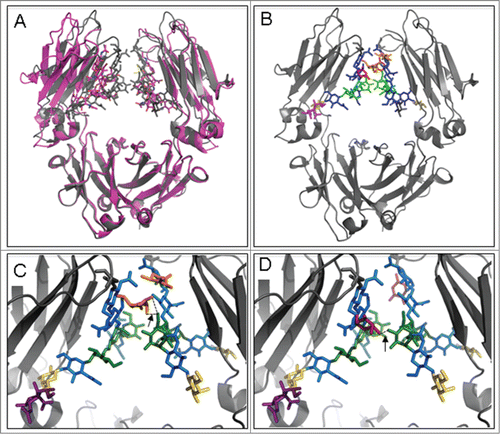
Explicit interactions between the glycan structures connected to the 2 CH2-domains appear to be mediated by the fucose moieties. shows the glycan structures, including α1,6-fucose moieties (in red) as observed in the crystal structureCitation23 and α1,3-fucosyl moieties (in orange) that were modeled using the molecular operating environment (MOE; Chemical Computing Group, Inc. 2014). The close-ups of show how hydrogen bonds between the fucose moieties and the opposing strands may be formed. The interactions seem more pronounced for the fucose in α1,3- linkage (for a top view, see Fig. S7). Note that the 3-dimensional orientation of the glycan structures is not entirely symmetric. The presence of the fucose moieties strengthens the interactions between the Fc strands through the glycan chains and potentially positions the entire glycan chain in a conformation that is optimally suited for sialylation and other glycan processing enzymes. An alternative hypothesis starts from the enhanced stability of the CH2-CH2 interaction, which allows the glycan structure to temporally take up energetically less favorable conformations, thereby facilitating sialylation.
Discussion
Site-specific glycosylation is a feature described for many eukaryotic proteins,Citation24-26 and it is a particularly well pronounced phenomenon in all antibody isotypes. While Fab glycosylation in different isotypes exhibits largely homogeneous glycosylation profiles (mainly bi-antennary, core fucosylated and extensively sialylated structures), Fc domains display considerable heterogeneity in their glycan composition, accounting for their complex effector functions, e.g., inflammation, modulation, immune suppression.
The complex (mostly non-sialylated) largely core fucosylated, galactosylated Fc glycans on IgG are a peculiarity. Even on identical but opposing CH2-domains, glycan variations are observed.Citation27,28 Thus, in an otherwise symmetrical molecule, asymmetry can be introduced by differential N-linked glycosylation at individual Fc sites. It is well known that the N-linked glycans on opposite Fc residues interact, maintaining the conformation of the Fc domain, and changes in Fc glycosylation can alter the Fc conformation and affect the binding to Fc receptors.Citation29–31 Structural analyses reveal protein-carbohydrate and carbohydrate-carbohydrate direct interactions between Fcγ receptors and Fc.Citation6,32 An ongoing debate involves the effect of IgG-Fc sialylation to structural and functional activities of IgG.Citation23,33 For IgG-Fc N-glycans, an unusual feature regarding lectin recognition was recently reported. Sambucus nigra agglutinin only binds to sialic acids on Fc N-glycans if at least 2 sialic acids are present in the CH2 pocket.Citation10 In contrast, no such constraint is seen for Fab glycans. The second sialic acid apparently affects the Fc glycan conformation so that the terminal sugars are no longer shielded by the protein structure.
The work reported here provides an example where the particular spatial orientation of Fc N-glycans or the associated stability of the CH2-CH2 interactions imposes constraints on the action of processing enzymes. Activities of GnT-III, GnT-IV and certainly 6-SiaT are considerably hampered for Fc-glycans as compared to Fab glycans. Curiously, this block can be alleviated by the non-physiological sugar residue core α1,3-fucose. Molecular modeling suggests that both 3- and 6-fucosylation can strengthen the interaction between the 2 homologous Fc domains through glycan chains thus potentially influencing the entire glycan conformation. As a consequence, the glycan structure may be directly positioned favorably for sialylation or the increased stability of the Fc-domain may allow the glycans to become more accessible for glycan processing enzymes.
The results obtained upon expression of the Sia pathway led to re-appearance of core 3-fucose in ΔXT/FT. In addition, overexpressing 3-FucT revealed that core 3-fucose facilitates sialylation. It seems that—on the other hand—sialylation of one glycan in the Fc pocket brings the other glycan in a position favorable for the action of the residual FucT activity in ΔXT/FT.
Core fucose and bisecting GlcNAc, the direct modifiers of the N-glycan core, are frequently observed on serum proteins. We show efficient synthesis of bisected GlcNAc of fucosylated GnGn structures. By contrast, bisecting GlcNAc hampers the transfer of core α1,3-fucose to GnGn, a phenomenon already described for core α1,6-fucosylation.Citation22,34 Our results are in agreement with the observation made for other proteins, where an N-glycan with a bisecting GlcNAc was not the substrate of other glycosyltransferases such as GnT-II, -IV, or -V.Citation21,35 Notably, molecular modeling exhibits that bisected GlcNAc residues may be accommodated without the need for conformational changes in the CxMab-Fc glycan structure (data not shown). Thus, the suppression of further processing and elongation of N-glycans due to bisecting GlcNAc modification may be attributed to a generally improper environment for glycosyltransferases created by this structure.
Recent molecular dynamics simulations showed a wide range of conformations adopted by Fc domains.Citation36 Direct interaction of carbohydrates with protein residues and carbohydrate-carbohydrate interaction of IgG and Fc receptors were shown,Citation37 demonstrating the enormous implication of Fc glycosylation on functional activities of IgGs. In simulations of an Fc fragment involving 6-fucosylated glycan trees, such interactions were also observed, together with a large flexibility of the fucose moiety.Citation38 Our discovery that N-glycan processing in the Fc domain relies on factors more complex than observed with free or Fab-bound N-glycans has repercussions for further studies aiming at the generation of therapeutic antibodies with improved efficacies due to optimized glycosylation. However, despite the recent market entry of a biopharmaceutical from plant cells,Citation39 the present work does not advocate the use of core α1,3-fucosylated antibodies, but rather it adds remarkable detail to our knowledge of Fc structure.
Material and Methods
Cloning cetuximab (Cx)
The amino acid sequence for both the light and heavy chains of cetuximab can be found in the international ImMunoGeneTics information database (IMGT (www.imgt.org/mAb-DB). A 1350-bp fragment containing the cDNA from heavy chain (CxHc, amino acids 20–469) and a 645-bp fragment containing the cDNA from light chain (CxLc, amino acids 20–235) were amplified with primers that introduce flanking BsaI restriction sites, and fragments were cloned into magnICON®-based TMV and PVX vectors (courtesy of Icon Genetics, GmbH)Citation40 to obtain pICHα26211:CxHc and pICHα31150:CxLc (Fig. S8).
Cloning of core α1,3- fucosyltransferase (3-FucT)
The catalytic domain of the Zea mays core α1,3-fucosyltransferase was amplified from pVTBacHis 1-MFucT cloneCitation41 as a BamHI-SalI fragment with the primer pair 5′- tataggatccgaagaacgccgaggcggtcg-3′ and 5′-tatagctgacttacacaaaaattacttcaagctttg-3′. The fragment was cloned into BamHI/SalI sites of the expression vector p3-FucT containing the cytoplasmic tail, transmembrane domain and stem (CTS) region of Arabidopsis thaliana core α1,3-fucosyltransferase.Citation42 Schematic representation of vector features is displayed in Figure S8. Other binary vectors used in this investigation for the modulation of plant glycosylation are described elsewhere: sialic acid pathway,Citation20,43,44 STGnT-III, 3-FucT GnT-IV, 3-FucTGnT-VCitation41 and 6-FucT.Citation45
Cetuximab infiltration and purification
Nicotiana benthamiana WT and ΔXT/FT plantsCitation19 were grown in a growth chamber at 22°C with a 16 h light/ 8h dark photoperiod. Leaves of 4–5 week old plants were used for agro-infiltration experiments. Agrobacteria (strain GV3101 pMP90) transformed with the TMV- (pICHα26211:CxHc) and PVX- (pICHα31150:CxLc) were grown at 29 °C for 24 h. After harvesting by gentle centrifugation (5 min at 3000 x g), the agrobacteria were resuspended in buffer (10 mM MES pH 5.6; 10 mM MgSO4), mixed and diluted to a final OD600 of ∼0.1–0.2. To modulate plant glycosylation, agrobacteria (strain UIA-143) transformed with binary vectors containing the cDNA of specific glycosyltransferases were prepared the same way and co-infiltrated with the viral-based vectors at a final OD600 of ∼0.05. At 3–5 days post-infiltration, 200–250 mg of infiltrated leaves were collected, and the mAb was purified using Protein A Sepharose™ Fast Flow (GE Healthcare) as described previously.Citation45
In vitro sialylation of glycoproteins
A soluble, truncated form of rat α2,6-sialyltransferase (6-SiaT) lacking the first 96 amino acids from the N-terminus and containing instead an N-terminal 6 × His tag was expressed in Sf9 cells with the help of the BaculoGold™ Baculovirus Expression System (BD Biosciences, Schwechat, Austria). Affinity purification was done with Ni-chelate agarose (Thermo Fisher). The enzyme was applied in 50 mM MES buffer (pH 7.0) with 16 mM MnCl2 and 0.2 mM CMP-sialic acid (Kyowa Hakko Europe).
Galactosylated IgG1-Fcs, the acceptor substrate for sialylation, were produced in N. benthamiana ΔXT/FT by co-expressing the STGalTCitation43 with either 3-FucT or 6-FucT to generate different core fucosylation versions. 10 µg each of 3 glycoforms AA, AAF3 and AAF6 (more precisely A4A4, A4A4F3, and A4A4F6) were incubated with amounts of purified recombinant rat 6-SiaT that convert two-thirds of Fc AA glycans into sialylated structures.
Glycopeptide analysis
N-glycan analysis of CxMab was carried out by LC-ESI-MS of tryptic glycopeptides.Citation16,26 Briefly, the heavy chain of SDS-PAGE separated IgGs was excised from the gel, S-alkylated, digested with sequencing grade modified trypsin (Promega) and subsequently analyzed by LC-ESI-MS.Citation16
Discrimination of core α1,3- from core α1,6-linked fucosylated glycans was accomplished by incubating a glycopeptide sample with 75 mU of PNGase F (Roche).
In vitro incubation with GnT-III and 3-FucT
Recombinant core α1,3-fucosyltransferase (3-FucT) was obtained as described by Leiter et al.Citation46 HepG2 cell derived soluble GnT-III (lacking the first 22 amino acids of the N-terminus) was recombinantly expressed in Sf9 cells. Culture medium containing GnT-III was concentrated by ultrafiltration and brought to 50 mM MES buffer (pH 7.0). De-galactosylated pronase glycopeptides of bovine fibrinCitation47 were incubated with either 3-FucT and 1 mM GDP-fucose (Kyowa Hakko Europe) or GnT-III and 1 mM UDP-GlcNAc (Kyowa Hakko). Subsequent to heat inactivation of enzymes, the products were incubated with the respective other enzyme and analyzed by LC-ESI-MS as described above.
Glycan modeling
The Fab glycan in was modeled using Pymol (Schrödinger, L. L. C. The PyMOL Molecular Graphics System) using the Fc-glycan from the structure with Protein Data Base (PDB) code 4BYH and a superposition of the atoms of Fc to Fab. α1,3-fucosylated glycan trees in were modeled using the carbohydrate builder in the Molecular Operating Environment (MOE 2014.09, Chemical Computing Group, Inc. Montreal). The positions of the fucose atoms were optimized using a steepest descent minimization of the modeled groups, applying the Amber99 force field.Citation48
Disclosure of Potential Conflicts of Interest
No potential conflicts of interest were disclosed.
Supplemental_Material.zip
Download Zip (8.5 MB)Acknowledgments
We thank Thomas Hackl and Pia Gattinger, Department of Applied Genetics and Cell Biology, University of Natural Resources and Life Sciences, Vienna, Austria for excellent technical support. magnICON® vectors were kindly supplied by Victor Klimyuk (IconGenetics, GmbH). cDNAs for the CxMab heavy and light chains were kindly supplied by f-star Biotechnologische Forschungs- und Entwicklungsges.m.b.H Vienna, Austria.
Funding
The work was supported by the Austrian Research Promotion Agency (Laura Bassi Center of Expertise “Plant produced BioPharmaceuticals” Grant Nr. 822757) and the Austrian Science Fund (Grant Nr. L575-B13). Andreas Thader was funded by the Austrian Science Fund (grant Nr. P22274).
Supplemental Material
Supplemental data for this article can be accessed on the publisher's website.
References
- Arnold JN, Wormald MR, Sim RB, Rudd PM, Dwek RA. The impact of glycosylation on the biological function and structure of human immunoglobulins. Ann Rev Immunol 2007; 25:21-50; PMID:17029568; http://dx.doi.org/10.1146/annurev.immunol.25.022106.141702
- Zauner G, Selman MH, Bondt A, Rombouts Y, Blank D, Deelder AM, Wuhrer M. Glycoproteomic analysis of antibodies. Mol Cell Prot MCP 2013; 12:856-65; PMID:23325769; http://dx.doi.org/10.1074/mcp.R112.026005
- Jefferis R. Isotype and glycoform selection for antibody therapeutics. Arch Biochem Biophys 2012; 526:159-66; PMID:22465822; http://dx.doi.org/10.1016/j.abb.2012.03.021
- Nimmerjahn F, Ravetch JV. Anti-inflammatory actions of intravenous immunoglobulin. Ann Rev Immunol 2008; 26:513-33; PMID:18370923; http://dx.doi.org/10.1146/annurev.immunol.26.021607.090232
- Shields RL, Lai J, Keck R, O'Connell LY, Hong K, Meng YG, Weikert SH, Presta LG. Lack of fucose on human IgG1 N-linked oligosaccharide improves binding to human Fcgamma RIII and antibody-dependent cellular toxicity. The J Biol Chem 2002; 277:26733-40; PMID:11986321; http://dx.doi.org/10.1074/jbc.M202069200
- Ferrara C, Grau S, Jager C, Sondermann P, Brunker P, Waldhauer I, Hennig M, Ruf A, Rufer AC, Stihle M, et al. Unique carbohydrate-carbohydrate interactions are required for high affinity binding between FcgammaRIII and antibodies lacking core fucose. Proc Nat Acad Sci U S A 2011; 108:12669-74; PMID:21768335; http://dx.doi.org/10.1073/pnas.1108455108
- Cartron G, de Guibert S, Dilhuydy MS, Morschhauser F, Leblond V, Dupuis J, Mahe B, Bouabdallah R, Lei G, Wenger M, et al. Obinutuzumab (GA101) in relapsed/refractory chronic lymphocytic leukemia: final data from the phase 1/2 GAUGUIN study. Blood 2014; 124:2196-202; PMID:25143487; http://dx.doi.org/10.1182/blood-2014-07-586610
- Qiu X, Wong G, Audet J, Bello A, Fernando L, Alimonti JB, Fausther-Bovendo H, Wei H, Aviles J, Hiatt E, Johnson A, et al. Reversion of advanced Ebola virus disease in nonhuman primates with ZMapp. Nature 2014; 514:47-53; PMID:25171469
- Anumula KR. Quantitative glycan profiling of normal human plasma derived immunoglobulin and its fragments Fab and Fc. J Immunol Meth 2012; 382:167-76; PMID:22683540; http://dx.doi.org/10.1016/j.jim.2012.05.022
- Stadlmann J, Pabst M, Altmann F. Analytical and functional aspects of antibody sialylation. J Clin Immunol 2010; 30:15-9; PMID:20390325; http://dx.doi.org/10.1007/s10875-010-9409-2
- Gutierrez G, Malan Borel I, Margni RA. The placental regulatory factor involved in the asymmetric IgG antibody synthesis responds to IL-6 features. J Reprod Immunol 2001; 49:21-32; PMID:11137110; http://dx.doi.org/10.1016/S0165-0378(00)00074-7
- Canellada A, Blois S, Gentile T, Margni Idehu RA. In vitro modulation of protective antibody responses by estrogen, progesterone and interleukin-6. Am J Reprod Immunol 2002; 48:334-43; PMID:12516657; http://dx.doi.org/10.1034/j.1600-0897.2002.01141.x
- Huang L, Biolsi S, Bales KR, Kuchibhotla U. Impact of variable domain glycosylation on antibody clearance: an LC/MS characterization. Anal Biochem 2006; 349:197-207; PMID:16360109; http://dx.doi.org/10.1016/j.ab.2005.11.012
- Loos A, Steinkellner H. IgG-Fc glycoengineering in non-mammalian expression hosts. Arch Biochem Biophys 2012; 526:167-73; PMID:22634260; http://dx.doi.org/10.1016/j.abb.2012.05.011
- Strasser R. Biological significance of complex N-glycans in plants and their impact on plant physiology. Front Plant Sci 2014; 5:363; PMID:25101107; http://dx.doi.org/10.3389/fpls.2014.00363
- Stadlmann J, Pabst M, Kolarich D, Kunert R, Altmann F. Analysis of immunoglobulin glycosylation by LC-ESI-MS of glycopeptides and oligosaccharides. Proteomics 2008; 8:2858-71; PMID:18655055; http://dx.doi.org/10.1002/pmic.200700968
- Ayoub D, Jabs W, Resemann A, Evers W, Evans C, Main L, Baessmann C, Wagner-Rousset E, Suckau D, Beck A. Correct primary structure assessment and extensive glyco-profiling of cetuximab by a combination of intact, middle-up, middle-down and bottom-up ESI and MALDI mass spectrometry techniques. MAbs 2013; 5:699-710; PMID:23924801; http://dx.doi.org/10.4161/mabs.25423
- Stoger E, Fischer R, Moloney M, Ma JK. Plant molecular pharming for the treatment of chronic and infectious diseases. Ann Rev Plant Biol 2014; 65:743-68; PMID:24579993; http://dx.doi.org/10.1146/annurev-arplant-050213-035850
- Strasser R, Stadlmann J, Schähs M, Stiegler G, Quendler H, Mach L, Glössl J, Weterings K, Pabst M, et al. Generation of glyco-engineered Nicotiana benthamiana for the production of monoclonal antibodies with a homogeneous human-like N-glycan structure. Plant Biotechnol J 2008; 6:392-402; PMID:18346095; http://dx.doi.org/10.1111/j.1467-7652.2008.00330.x
- Castilho A, Strasser R, Stadlmann J, Grass J, Jez J, Gattinger P, Kunert R, Quendler H, Pabst M, Leonard R, et al. In planta protein sialylation through overexpression of the respective mammalian pathway. J Biol Chem 2010; 285:15923-30; PMID:20305285; http://dx.doi.org/10.1074/jbc.M109.088401
- Narasimhan S, Freed JC, Schachter H. The effect of a “bisecting” N-acetylglucosaminyl group on the binding of biantennary, complex oligosaccharides to concanavalin A, Phaseolus vulgaris erythroagglutinin (E-PHA), and Ricinus communis agglutinin (RCA-120) immobilized on agarose. Carb Res 1986; 149:65-83; PMID:3731182; http://dx.doi.org/10.1016/S0008-6215(00)90370-7
- Umana P, Jean-Mairet J, Moudry R, Amstutz H, Bailey JE. Engineered glycoforms of an antineuroblastoma IgG1 with optimized antibody-dependent cellular cytotoxic activity. Nature Biotechnol 1999; 17:176-80; PMID:10052355; http://dx.doi.org/10.1038/6179
- Crispin M, Yu X, Bowden TA. Crystal structure of sialylated IgG Fc: implications for the mechanism of intravenous immunoglobulin therapy. Proc Nat Acad Sci U S A 2013; 110:E3544-6; PMID:23929778; http://dx.doi.org/10.1073/pnas.1310657110
- Sturm A, Van Kuik JA, Vliegenthart JF, Chrispeels MJ. Structure, position, and biosynthesis of the high mannose and the complex oligosaccharide side chains of the bean storage protein phaseolin. J Biol Chem 1987; 262:13392-403; PMID:3654619
- Go EP, Chang Q, Liao HX, Sutherland LL, Alam SM, Haynes BF, Desaire H. Glycosylation site-specific analysis of clade C HIV-1 envelope proteins. J Prot Res 2009; 8:4231-42; PMID:19610667; http://dx.doi.org/10.1021/pr9002728
- Pabst M, Chang M, Stadlmann J, Altmann F. Glycan profiles of the 27 N-glycosylation sites of the HIV envelope protein CN54gp140. Biol Chem 2012; 393:719-30; PMID:22944675; http://dx.doi.org/10.1515/hsz-2012-0148
- Jefferis R, Lund J, Mizutani H, Nakagawa H, Kawazoe Y, Arata Y, Takahashi, N. A comparative study of the N-linked oligosaccharide structures of human IgG subclass proteins. Biol Chem 1990; 268:529-37; PMID:2363690
- Masuda K, Yamaguchi Y, Kato K, Takahashi N, Shimada I, Arata Y. Pairing of oligosaccharides in the Fc region of immunoglobulin G. FEBS lett 2000; 473:349-57; PMID:10818239; http://dx.doi.org/10.1016/S0014-5793(00)01557-X
- Jefferis R, Lund J, Pound JD. IgG-Fc-mediated effector functions: molecular definition of interaction sites for effector ligands and the role of glycosylation. Immunol Rev 1998; 163:59-76; PMID:9700502; http://dx.doi.org/10.1111/j.1600-065X.1998.tb01188.x
- Krapp S, Mimura Y, Jefferis R, Huber R, Sondermann P. Structural analysis of human IgG-Fc glycoforms reveals a correlation between glycosylation and structural integrity. J Mol Biol 2003; 325:979-89; PMID:12527303; http://dx.doi.org/10.1016/S0022-2836(02)01250-0
- Radaev S, Sun PD. Recognition of IgG by Fcgamma receptor. The role of Fc glycosylation and the binding of peptide inhibitors. J Biol Chem 2001; 276:16478-83; PMID:11297533; http://dx.doi.org/10.1074/jbc.M100351200
- Lu J, Chu J, Zou Z, Hamacher NB, Rixon MW, Sun PD. Structure of FcgammaRI in complex with Fc reveals the importance of glycan recognition for high-affinity IgG binding. Proc Nat Acad Sci U S A 2015; 112:833-8; PMID:25561553; http://dx.doi.org/10.1073/pnas.1418812112
- Sondermann P, Pincetic A, Maamary J, Lammens K, Ravetch JV. General mechanism for modulating immunoglobulin effector function. Proc Nat Acad Sci U S A 2013; 110:9868-72; PMID:23697368; http://dx.doi.org/10.1073/pnas.1307864110
- Ferrara C, Brunker P, Suter T, Moser S, Puntener U, Umana P. Modulation of therapeutic antibody effector functions by glycosylation engineering: influence of Golgi enzyme localization domain and co-expression of heterologous beta1, 4-N-acetylglucosaminyltransferase III and Golgi alpha-mannosidase II. Biotechnol and Bioeng 2006; 93:851-61; PMID:16435400; http://dx.doi.org/10.1002/bit.20777
- Brockhausen I, Schachter H. Glycosyltransferases involved in N- and O-glycan biosynthesis. In: Glycosciences: status and perspectives, eds. H.-J. Gabius aSG, London-Weinheim: Chapman & Hall; 1997:79-113.
- Frank M, Walker RC, Lanzilotta WN, Prestegard JH, Barb AW. Immunoglobulin G1 Fc domain motions: implications for Fc engineering. J Mol Biol 2014; 426:1799-811; PMID:24522230; http://dx.doi.org/10.1016/j.jmb.2014.01.011
- Ahmed AA, Giddens J, Pincetic A, Lomino JV, Ravetch JV, Wang LX, Bjorkman PJ. Structural characterization of anti-inflammatory immunoglobulin G Fc proteins. J Mol Biol 2014; 426:3166-79; PMID:25036289; http://dx.doi.org/10.1016/j.jmb.2014.07.006
- Lai B, Hasenhindl C, Obinger C, Oostenbrink C. Molecular dynamics simulation of the crystallizable fragment of IgG1-insights for the design of Fcabs. Intern J Mol Sci 2014; 15:438-55; PMID:24451126; http://dx.doi.org/10.3390/ijms15010438
- Pastores GM, Petakov M, Giraldo P, Rosenbaum H, Szer J, Deegan PB, Amato DJ, Mengel E, Tan ES, Chertkoff R, et al. A Phase 3, multicenter, open-label, switchover trial to assess the safety and efficacy of taliglucerase alfa, a plant cell-expressed recombinant human glucocerebrosidase, in adult and pediatric patients with Gaucher disease previously treated with imiglucerase. Blood Cells Mol Dis 2014; 53:253-260; PMID:24950666; http://dx.doi.org/10.1016/j.bcmd.2014.05.004
- Marillonnet S, Thoeringer C, Kandzia R, Klimyuk V, Gleba Y. Systemic Agrobacterium tumefaciens-mediated transfection of viral replicons for efficient transient expression in plants. Nature Biotechnol 2005; 23:718-23; PMID:15883585; http://dx.doi.org/10.1038/nbt1094
- Bondili JS, Castilho A, Mach L, Glossl J, Steinkellner H, Altmann F, Strasser R. Molecular cloning and heterologous expression of beta1,2-xylosyltransferase and core alpha1,3-fucosyltransferase from maize. Phytochemistry 2006; 67:2215-24; PMID:16920165; http://dx.doi.org/10.1016/j.phytochem.2006.07.007
- Castilho A, Gattinger P, Grass J, Jez J, Pabst M, Altmann F, Gorfer M, Strasser R, Steinkellner H. N-glycosylation engineering of plants for the biosynthesis of glycoproteins with bisected and branched complex N-glycans. Glycobiology 2011; 21:813-23; PMID:21317243; http://dx.doi.org/10.1093/glycob/cwr009
- Strasser R, Castilho A, Stadlmann J, Kunert R, Quendler H, Gattinger P, Jez J, Rademacher T, Altmann F, Mach L, et al. Improved virus neutralization by plant-produced anti-HIV antibodies with a homogeneous beta1,4-galactosylated N-glycan profile. J Biol Chem 2009; 284:20479-85; PMID:19478090; http://dx.doi.org/10.1074/jbc.M109.014126
- Castilho A, Pabst M, Leonard R, Veit C, Altmann F, Mach L Glossl J, Strasser R, Steinkellner H. Construction of a functional CMP-sialic acid biosynthesis pathway in Arabidopsis. Plant Physiol 2008; 147:331-9; PMID:18326787; http://dx.doi.org/10.1104/pp.108.117572
- Castilho A, Bohorova N, Grass J, Bohorov O, Zeitlin L, Whaley K, Altmann F, Steinkellner Hl. Rapid high yield production of different glycoforms of Ebola virus monoclonal antibody. PloS one 2011; 6:e26040; PMID:22039433; http://dx.doi.org/10.1371/journal.pone.0026040
- Leiter H, Mucha J, Staudacher E, Grimm R, Glossl J, Altmann F. Purification, cDNA cloning, and expression of GDP-L-Fuc:Asn-linked GlcNAc alpha1,3-fucosyltransferase from mung beans. J Biol Chem 1999; 274:21830-9; PMID:10419500; http://dx.doi.org/10.1074/jbc.274.31.21830
- Fabini G, Freilinger A, Altmann F, Wilson IB. Identification of core alpha 1,3-fucosylated glycans and cloning of the requisite fucosyltransferase cDNA from Drosophila melanogaster. Potential basis of the neural anti-horseadish peroxidase epitope. J Biol Chem 2001; 276:28058-67; PMID:11382750; http://dx.doi.org/10.1074/jbc.M100573200
- Wang JM, Cieplak P, Kollman PA. How well does a restrained electrostatic potential (RESP) model perform in calculating conformational energies of organic and biological molecules? J Comput Chem 2000; 21:1049-74; http://dx.doi.org/10.1002/1096-987X(200009)21:12%3c1049::AID-JCC3%3e3.0.CO;2-F