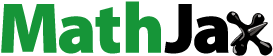
Abstract
Immune thrombocytopenia (ITP) is an autoimmune bleeding disorder characterized by low platelet count and presence of IgG autoantibodies to platelet surface glycoproteins, such as IIbβ3 and GPIb/IX. Our previous work has shown that platelets in ITP patients exist in an activated state. Two different marker-based approaches are used to study the course of platelet activation: (1) binding of PAC-1 antibody, signifying a change in αIIbβ3 conformation, and (2) expression of P-selectin, signifying alpha granule content release from platelets. Here, we describe the development of a new scFv antibody (R38) that, compared with PAC-1, appears to better distinguish between platelets of ITP patients and healthy controls. Notably, R38 was generated using commercially sourced resting-state integrin that was coated on a microtiter plate. Its ability to distinguish between ITP patients and healthy controls thus suggests that inadvertent integrin activation caused by coating involves a conformational change and exposure of a cryptic epitope. This report also describes for the first time the potential use of an scFv antibody in the immunodiagnosis of platelet activation in ITP patients.
Keywords:
Introduction
In this study, we aimed to identify phage display-derived single chain variable fragment (scFv) monoclonal antibodies capable of distinguishing between resting-state and activated-state platelets, as well as immune thrombocytopenic purpura (ITP) patients and healthy controls, based on presumed differences in conformational states of the αIIbβ3 integrin in experimentally-activated platelets and ITP patients, over normal platelets and healthy controls. ITP is an auto-immune disorder in which antibodies to a specific glycoprotein present on the surfaces of platelets and platelet-generating megakaryocytes (the αIIbβ3 or the GPIb/IX integrin) cause the immune destruction of platelets.Citation1-3 The autoantibodies target the integrin on platelet surfaces in ITP patients, and platelet-bound antibodies have also been isolated and studied.Citation4 Additionally, it has been demonstrated that autoantibodies in ITP can also result in impaired proplatelet formation by megakaryocytes.Citation5,6
In terms of platelet physiology and the structural-biochemistry of the integrin, 2 points are relevant to ITP: 1) If the αIIbβ3 integrin is hidden, autoantibodies cannot be generated against any epitopes present on the protein; and 2) If epitopes on the integrin are usually inaccessible by the immune system, then there must be some underlying mechanism that causes a conformational change in the integrin, to expose epitopes that are not normally accessible, for autoantibodies targeting the exposed epitopes to be generated. Therefore, at least 2 prerequisites could conceivably apply to the effector action of autoantibodies upon platelets: 1) inside-out signaling, or some equivalent event leading to greater presence of the integrin on the surfaces of platelets and, 2) conformational alterations within the integrin, leading to exposure of some cryptic epitopes against which antibodies happen to be generated in vivo. Indeed, it is even conceivable that ITP could primarily be a consequence of an overdriven platelet activation mechanism, resulting in the exposure of cryptic epitopes and the generation of autoantibodies, with the further auto-immune consequences of generation, presence and action of autoantibodies as an end result of the trigger. Notably, in this regard, we have recently shown that platelets in ITP patients do exist in an activated state.Citation7 However, our earlier studies were conducted using commercially available PAC-1 and P-selectin antibodies. We decided to attempt a de novo generation of phage display-derived antibodies against integrins and platelets to examine whether such antibodies are capable of distinguishing between healthy controls and patients to a greater degree.
Phage display antibody library-based screening methods were used to identify antibodies binding to ADP-activated as well as thrombin-activated platelets. We also used the same method to select for antibodies binding to commercially obtained integrin (presumed to be in the resting-state, rather than in the activated-state). The scFv monoclonal antibodies thus identified were tested for binding to platelets of ITP patients as well as healthy controls. The most remarkable result obtained during these studies was that the monoclonal scFv antibodies found to be best capable of differentiating between both resting and activated platelets, as well as ITP patients and healthy controls, were derived from phage display library screening against commercially sourced αIIbβ3 integrin coated/immobilized on a microtiter plate. This suggests that what we had believed to be resting-state integrin was probably substantially converted to activated-state integrin through a conformational change caused by binding to microtiter plate surfaces. Notably, others have also shown that conformational change(s) can accompany binding of protein(s) to microtiter plate(s).Citation8
Of all antibodies generated and tested in the current studies, one generated against commercially sourced αIIbβ3 integrin (R38 scFv) showed a two-fold better ability to distinguish between platelets derived from patients and healthy controls compared with commercially sourced antibodies, PAC-1 and P-Selectin.
Results
Generation of scFv antibodies against ADP-activated and thrombin-activated platelets
Following three rounds of biopanning and amplification in each case (ADP or thrombin activated platelets), 96 scFv clones were screened in cell-ELISA assays, to identify clones capable of distinguishing between resting-state and activated-state platelets. Figure S1A shows that only 3 scFv clones generated against ADP-activated platelets (O5, O27 and O52) showed differential binding between resting and activated platelets with fold-increases of 6.25%, 7.75% and 3.29%, respectively. The scFv clone O52 was chosen for further studies, based on the high level of expression of this particular clone. Figure S1B shows the results of screening scFv antibodies generated against thrombin-activated platelets; 6 scFv clones (N8, N13, N34, N56, N87 and N93) showed differential binding between resting-state and activated-state platelets, with fold-increases of 2.1%, 1.65%, 2.1%, 1.26%, 1.95% and 3.97%, respectively. The scFv clone N87 was chosen for further studies. The selection of clones was based upon both the differential capabilities of the scFv antibodies to bind to resting-state and activated-state platelets, as well as levels of expression of the scFv antibodies in soluble form.
Biopanning of antibody library against resting state integrin αIIbβ3
We used commercially sourced αIIbβ3 integrin complex in 3 cycles of biopanning and amplification with the intent of generating antibodies against resting state integrins. Following the third round of panning, 2 scFv clones, R38 and R87, were randomly picked for characterization, assuming that these could be used as antibodies specific to the resting-states of integrins/platelets.
Characterization of the selected clones
The four selected scFv antibodies (O52, N87, R38 and R87) were purified (Fig. S2) and the sequences of the CDR regions of all were determined (). In order to determine the binding partners of each of these scFv antibodies, platelet lysate was either used in native state () or treated with thrombin (for activation) () and immunoprecipitation (IP) was carried out using scFv antibodies immobilized on protein-A agarose beads. In , comparison of lane 3 (platelet lysate alone) with lane 4 (IP done with scFv O52) shows that O52 bound to several of the protein constituents of the platelet lysate. Similar data was obtained for R38 and R87 (). This was to be expected, since the latter 2 antibodies were obtained by biopanning carried out against commercially sourced (pure) αIIbβ3 proteins, and αIIbβ3 could be expected to be complexed with fibrinogen and other proteins when the platelets are activated with thrombin. The red and black arrows in the figure were independently confirmed to be GPIIb and IIIa, respectively, by mass spectrometry and western blotting (Fig. S3 and Fig. S4, respectively). It is important to note that no binding of scFv antibodies was observed when denatured lysate (using 1% SDS and 0.5% deoxycholate) was used for immunoprecipitation instead of native lysate (data not shown), suggesting that these scFv antibodies bind to conformational epitopes only, and not to sequence epitopes.
Figure 1. Immunoprecipitation (IP) of platelet lysates, prepared under native conditions (Panel A) or activated with thrombin (Panel B). Solubilized proteins contained in platelet lysates were subjected to immunoprecipitation with scFv antibodies, immobilized on Protein-A agarose resin. Elution fractions collected were analyzed by SDS-PAGE (10% acrylamide). Panel A: Lane 1: molecular weight marker; Lane 2: scFv alone (no IP); Lane 3: platelet lysate alone (no IP); Lane 4: IP with O52; Lane 5: IP with no scFv (control); Lane 6: IP with R87; Lane 7: IP with N87. It is to be noted that 2 separate gels are presented in Panel A. Panel B: Lane 1: platelet lysate alone (no IP); Lane 2: molecular weight marker; Lane 3: IP with scFv R38; Lane 4: IP with scFv R87.
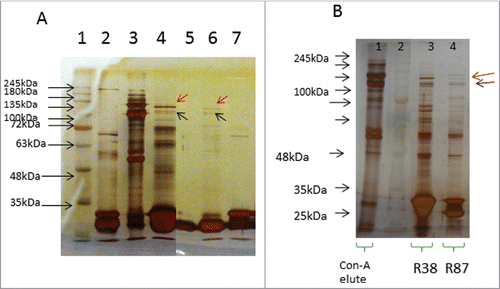
Table 1. Amino acid sequence of CDR regions of heavy chains of the scFv clones
Immunostaining of resting and activated platelets with selected scFv antibodies
shows that all 4 scFv antibodies, i.e., O52, R38, R87, and N87, bind to ADP-activated platelets. The only difference is that N87 also binds to “resting state” platelets, and is therefore not able to differentiate between “resting state” and “activated state” platelets, whereas this is not true of the other 3.
Assessment of scFv antibodies with respect to inhibition of platelet aggregation
Since our data showed that 3 scFv antibodies, O52, R38, and R87, were effective at distinguishing between resting-state and activated-state platelets, we examined the abilities of these antibodies to effect reduction in platelet aggregation. Platelet aggregation was determined by measuring the change in the optical density (i.e., transmittance of light) of stirred platelet-rich plasma (PRP) after addition of the agonist (ADP) to the ‘aggregometer’ cuvette. shows that PAC-1 reduced the extent of platelet aggregation by 85% relative to control, while the scFv antibodies O52, R38 R87, and N87 were effective in reducing the extent of ADP-induced aggregation by 27%, 46%, 41% and 41% respectively, at a concentration of 50 µg/ml. At a concentration of 100 µg/ml, the 4 antibodies reduced platelet aggregation to the extents of 37% (O52), 59% (R38), 37% (R87) and 44% (N87). Statistical evaluation of the extent of platelet aggregation with respect to the control showed that the inhibition of platelet aggregation was significant in the case of PAC-1 starting from a concentration of 10 µg/ml (p value < 0.001). R38 showed statistically significant decrease in platelet aggregation starting from a concentration of 50 µg/ml (p value < 0.01); R87 and N87 showed statistically significant decrease in platelet aggregation at 50 µg/ml (p value < 0.01). O52 resulted in statistically significant decrease in platelet aggregation starting from a concentration of 25 µg/ml (p value < 0.01).
Figure 3. Effect of scFv antibodies on inhibition of ADP-activated platelet aggregation. Platelet-rich plasma was prepared from 4.5 ml of blood sample, collected in 0.5 ml of 3.8% sodium citrate. The platelet count was adjusted to 2 × 108/ml. Aggregation assays were performed at 37°C in siliconized cuvettes at a stirring speed of 900 rpm. 450ul of PRP was taken per tube, and incubated with PAC-1 and scFv antibodies for 20 min. This was followed by addition of 10μM of ADP, and light transmission was recorded till 3 min, and expressed as the percentage of the maximal platelet aggregation in the absence of scFv (PBS). Experiments were conducted using blood drawn from 5 different donors; results were expressed as mean ± SEM.
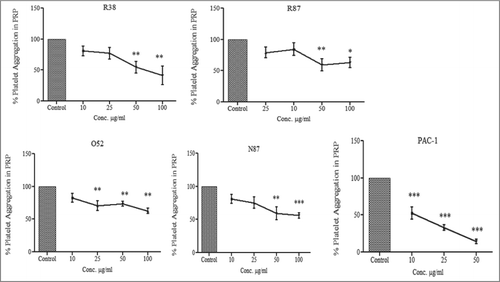
Assessment of scFv antibodies with respect to binding to activated platelets by flow cytometry
We assessed the ability of scFv antibodies to bind to platelets activated by different agonists (ADP, collagen and thrombin) using flow cytometry. The mean fluorescence intensity (MFI) was recorded and in each case the fold activation, i.e., MFI of activated platelets versus MFI of resting platelets was calculated. In these experiments, platelets were incubated with the respective antibodies, before the addition of the individual agonists, and each experiment was carried out with 3 different donors. For statistical comparisons, results were declared significant if P-value < 0.05. Significance was denoted as p < 0.05 (*); < 0.005(**); < 0.0001 (***). shows that when ADP was used for platelet activation, the fold increase in MFI in the case of PAC-1 was significantly greater than the activation fold for any of the other scFv antibodies. However, this was not the case with collagen or thrombin activated platelets (Panels B and C, respectively). When platelets were activated with thrombin (), the activation fold for binding of O52 and R38 was significantly greater than that for PAC-1 (p values: 0.019 and 0.014, respectively); the activation fold was significantly greater than P-selectin for each of the other scFv antibodies (p values: 0.01 for O52; 0.005 for R38; 0.03 for R87 and 0.001 for N87). This suggests that: 1) the scFv antibodies are effective in recognizing the conformation provided by the aggregates being formed upon thrombin activation, and 2) the conformations provided by the aggregates formed by collagen and thrombin are not similar, although both are strong agonists.
Figure 4. Assessment of binding of scFv antibodies to platelets aggregated by different agonists. Platelets were activated by either ADP (Panel A), or collagen (Panel B), or thrombin (Panel C). In each case mean fluorescence intensity (MFI) of binding to activated platelets vs resting platelets was observed and activation fold (of activated vs resting platelets) was determined. The experiment was repeated in each case with 3 different donors; the data was expressed as mean ± SEM.
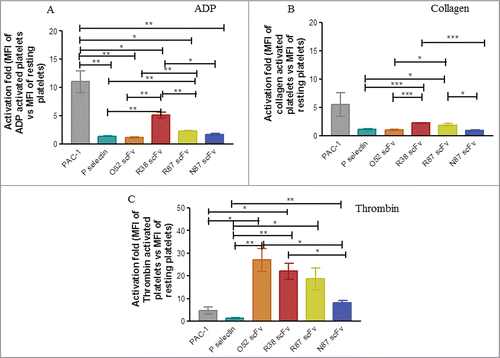
Assessment of status of platelet activation in chronic ITP patients, using the panel of scFv antibodies
We examined the potential of the scFv antibodies in the assessment of activation status of platelets in ITP patients by flow cytometry, using the same protocol described earlier.Citation7 Platelets were gated based on side scatter and forward scatter as described earlier.Citation7 In each case, the fold-activation was determined in ITP patients in terms of mean fluorescent intensity (MFI) obtained for a fluorophore (tagged to the scFv antibody) for platelets of ITP patients vs. the value of the MFI obtained for the same scFv antibody in case of a healthy control (Fig. S5; ). In each patient, PAC-1 and P-selectin MFI values were also similarly determined. As is evident from , R38 scFv was the best, with a 2-fold better ability to distinguish between platelets derived from patients and healthy controls. A comparison of activation fold values obtained with different antibodies showed that R38 antibody is better than PAC-1 (p value: 0.02) as well as P-selectin (p value: 0.0017) in detecting platelet activation in ITP patients with respect to that of healthy controls. The only other significant value obtained was that of O52 compared to P-selectin (p value: 0.015). It was not surprising that N87 antibody is poor in detecting platelet activation because, as seen earlier, it is incapable of distinguishing resting platelets from activated platelets (immunostaining data).
Figure 5. Assessment of platelet activation in healthy controls and ITP patients by flow cytometry. Baseline expression of P-selectin, and binding of PAC-1, and FITC-labeled scFv antibodies was examined. In each case mean fluorescence intensity (MFI) of binding was observed and activation fold was determined in ITP patients in terms of MFI of ITP patients vs. MFI of healthy control for binding of each antibody. Number of patients for PAC-1 and P-selectin = 24. For the scFv antibodies, the number of patients were as follows: scFv R87: n = 14, scFv R38: n = 9, scFv O52: n = 9; scFv N87: n = 14. Data is represented as mean ± SEM.
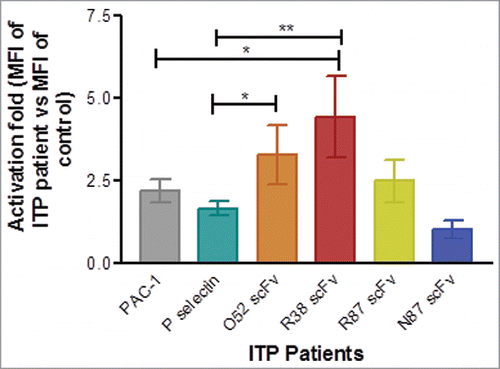
Discussion
Using phage display antibody library screening methods, we identified scFv antibodies capable of binding to integrins/platelets that could be useful in several respects. Firstly, because these antibodies are scFv (and not whole immunoglobulins), they possess the advantages commonly associated with such antibodies for diagnosis [i.e., small size, ease of production in bulk quantities, and economy of production], and therapy [i.e., non-immunogenicity, owing to the sequences in the phage display library being entirely human-derived]. Secondly, apart from the general benefits described above, at least one of these scFv antibodies, (scFv R38), could be useful in immunodiagnosis because it appears to be at least two-fold better at recognizing activated platelets in the specific context of ITP patients compared to the PAC-1 and P-selectin antibodies that are currently widely used for the same purpose.
It is important to note that although N87 was observed to also bind to activated platelets, it is not very useful in the context of distinguishing resting and activated platelets because it binds equally well to resting platelets. This becomes crucial in the context of patients since N87 loses its importance in being able to differentiate between healthy controls (where platelets are not activated) and ITP patients (where platelets are present in an activated state Citation7).
Interestingly, R87 and R38 were obtained through panning the phage display antibody library against commercially sourced αIIbβ3. These antibodies were expected, therefore, to be generated against epitopes on resting state integrins. However, intriguingly, both R87 and R38 were able to bind to conformational epitopes specific to activated-state platelets, rather than to resting-state platelets. Jacobin et al.Citation9 reported that the inactive integrin complex when coated on a plastic surface tends to acquire a high-affinity conformational state, and R38 and R87 could have been inadvertently selected against such states on the integrins; specifically, they could have been selected against cryptic epitopes exposed by the binding during coating of plates. More pertinently, these epitopes seem to be exposed and available in the platelets of ITP patients.
The process of platelet activation/aggregation is very complex and involves multiple protein interactions. The process is initiated by the binding of fibrinogen (Fg) to activated αIIbβ3, thereby bridging adjacent platelets.Citation10-11 Upon activation, the platelets release the contents of alpha granules, which includes many adhesive proteins [e.g., Fg, von Willebrand factor and thrombospodin], plasma proteins [e.g., IgG and albumin], cellular mitogens [e.g., platelet-derived growth factor and transforming growth factor beta], coagulation factors [e.g., factor V] and protease inhibitors [e.g., alpha 2-macroglobulin and alpha 2-antiplasmin].Citation12 The inner lining of the alpha-granule unit membrane has been demonstrated to contain a number of physiologically important receptors, including αIIbβ3 and P-selectin.Citation13 Subsequently, P-selectin, which is progressively expressed on the platelet surface, binds by means of the lectin domain to its binding site on adjacent platelets, stabilizing interactions between already-bridged platelets, thereby allowing the formation of large and stable platelet aggregates. It is not surprising, therefore, that immunoprecipitation of the platelet lysate with the scFv antibodies shows the presence of several proteins in the eluate, which could be in complex with the integrins.
R38, R87 and N87 were effective in significantly reducing the extent of platelet aggregation with respect to the control This decrease in aggregation is considerable, especially in light of the fact that another antibody known to bind specifically to GPIIb, i.e., the Tab antibody,Citation14 does not support inhibition of aggregation at all. It is possible that the site involving the binding of R87 and R38 is important in platelet aggregation, unlike the site of binding implicated in binding to the Tab antibody, resulting in steric blocking of the binding of fibrinogen to platelets. Another antibody known to specifically bind to GPIIIa (AP3),Citation15 also does not support any inhibition of aggregation of platelets. Importantly, both R38 and R87 antibodies do not bind to resting platelets and stain only activated platelets. It would seem, therefore, that these antibodies bind to a region on the integrins that plays an important role in aggregation, in addition to becoming exposed upon activation. Two kinds of ligand binding sites exist on the surface of αIIbβ3: 1) the activation- dependent sites, for binding of ligands such as fibronectin (the RGD type) and fibrinogen (the AGDV type); and 2) the activation-independent sites for binding of small molecule ligands, such as RGD mimetics. R38 and R87 would therefore fall in the category of activation-dependent ligands. The monoclonal antibody 3C7, which is directed against the complex αIIbβ3, is also known to inhibit platelet aggregation.Citation16 However, this antibody was observed to bind to both non-activated and activated platelets, and as such would not be of use simultaneously in the diagnosis of the activation status of platelets in patients.
It is interesting that binding of R38 and R87 to αIIbβ3 on platelet surfaces is dependent on activation, and can effect inhibition of aggregation, since it has been reported that, in most patients with chronic ITP, autoantibodies bind to epitopes localized on αIIb.Citation17 It would seem then that the particular epitope recognized by R38 and R87 could be important in the pathogenesis of ITP on account of the ability of these antibodies to: 1) differentiate between resting-state and ADP-induced activated-state platelets; 2) bind to αIIb, which offers important epitopes for recognition by autoantibodies in ITP patients; and 3) effectively recognize the conformation provided by the aggregates formed upon thrombin activation. Further, because anti-αIIbβ3 autoantibodies from most chronic ITP patients bind to epitopes localized on αIIb, and because their binding to antigen does not occur in the absence of αIIb or in the presence of β3 coupled to alpha v integrin,Citation17 the binding of R87 and R38 to αIIb in an ADP-dependent fashion is particularly interesting both from a fundamental and a therapeutic point of view. Again, this is especially so because the selective binding of these scFv antibodies to activated-state platelets rather than to resting-state platelets will prevent undesired side effects emanating from the binding of such an scFv to αIIbβ3 receptors on circulating platelets and megakaryocytes if they were to be chronically occupied by this antibody.Citation18
In this respect, R87 or R38 could be considered as candidate antithrombotic drugs that can be expected to act upon the receptors at the appropriate site, and only when needed, for example in the setting of percutaneous coronary interventions. The antibodies may also be well suited as immunodiagnostic reagents in the context of ITP.
Materials and Methods
Patient samples
Twenty-four patients diagnosed with chronic ITP in the age range of 22 y to 61 y and 15 age-matched healthy controls with platelet count in the normal range of 150, 000 to 450,000/μl were recruited in this study. Informed consent was taken from each patient and the study was approved by the Institutional Ethics Committee of the Postgraduate Institute of Medical Education and Research. The patients who were on drugs other than steroids, or with other autoimmune disorders, infections and below 15 y of age were considered inappropriate for the study. The indication of treatment was ongoing bleeding in the form of purpuric spots on the body or mucosal bleed (gums, hematuria, menorrhagia) or platelet count less than 30,000/μl. Blood samples were drawn after 4 weeks of steroid therapy, and non-responder (NR) and responder (R) patients (categorized according to the ASH 2011 practice guidelines for ITPCitation19) were selected for the study on the basis of steroid response and platelet count. Based on the guidelines, the ITP patients were classified as non-responders (NR < 30 × 109/l, or less than 2 fold increase in platelet count from baseline) and as responders (R ≥ 30 × 109/l, and greater than 2 fold increase in platelet count from baseline, and absence of bleeding). All non-responders and partial responders (NR and R) were included in the study; the complete responders (CR > 100 × 109/l and absence of bleeding) were excluded.
Clinical evaluation
Clinical history, physical examination (petechia, purpura, ecchymosis, mucosal bleeding, menorrhagia, internal bleeding) and laboratory investigations (hemogram, bone-marrow findings) were recorded. Patients positive for auto-immune markers for other auto-immune disorders were excluded from the study.
Preparation of platelet rich plasma and activation by agonists
Briefly, 5 ml of blood was drawn from normal healthy individuals or ITP patients, by venipuncture using 21-gauge needle, taking precautions by not using the first 2 ml of blood for flow analysis. Samples were mixed with 1/10 vol of the anticoagulant, sodium citrate, and PRP was prepared by centrifugation at 800 rpm for 10 min. 1 × 105 platelets from this preparation (from ITP patients and healthy controls) were added to 50 µl of phosphate-buffered saline (PBS). The platelets were induced for activation by 20 µM ADP, or 2 µg/ml collagen type I or 0.5 U/ml thrombin.
Biopanning of scFv antibodies against activated platelets
Phage-display technology was employed for generating antibodies specific to thrombin- or ADP-activated platelets. The human antibody scFv library (available as Tomlinson I and J, procured from MRC, UK), constructed in fusion with the pIII protein of M13 filamentous phages, was used for generating antibodies specific to activated platelets using the methods described earlier.
To obtain ADP-activated platelets, washed platelets were used. For this purpose, PRP suspension was centrifuged at 1000 × g for 10 min; the pellet was resuspended in Tyrode buffer and centrifuged 2 times to remove the remaining red blood cells. The final platelet pellet was suspended in Tyrode-HEPES buffer (pH 7.4) and platelet count of 2.5 × 108/ml was adjusted. The washed platelets were activated with either 20 µM ADP or 0.5 U/ml), fixed with 0.025% paraformaldehyde, and coated overnight at 4°C in 100 µl of 0.1 M sodium bicarbonate (pH 9.6). To obtain thrombin-activated platelets, subtractive panning was used to remove the population of phages specific to resting-platelets (prepared by using PGE1 in platelet rich plasma or PRP). The phage library was first incubated with resting-state platelets and unbound phages were collected and used for panning against thrombin-activated platelets, using the same protocol as described earlier.Citation20
In both cases, 3 rounds of biopanning and amplification of phages were carried out; an aliquot of phage obtained as eluate from the third biopanning round was infected into exponentially growing HB2151 E. coli cells to produce soluble scFv, and 10-fold dilutions were grown on TYE plates containing ampicillin and 1% glucose. Individual colonies were picked and inoculated into media present in well of a 96-well plate, grown overnight and transferred the next day into individual wells for overnight growth and induction with 1 mM IPTG. The supernatant from these wells, containing the soluble scFv in each case, was then used to check for binding against resting and ADP-activated platelets, in an ELISA assay, using the scFv as the primary antibody and mouse anti-His-HRP conjugated antibody as secondary antibody.
Biopanning of scFv antibodies against resting state αIIbβ3
Biopanning and amplification steps were also carried out to generate scFv antibodies against commercially sourced GPIIb/GPIIIa integrin (Enzyme Research labs), with the intent of generating scFv against resting state integrin.
Screening of scFv clones by Cell-ELISA assay
Resting platelets were prepared by using prostaglandin (PGE1) in PRP; activated platelets were prepared in buffer without PGE1, and activated with thrombin (0.5 U/ml); the final platelet pellet was suspended in Tyrode-Hepes buffer (pH 7.4) and the platelet count was adjusted to 2.5 × 108/ml. In both cases, 107 platelets were added to each well on a 24-well plate, followed by stirring using a rice bead in each well containing activated platelets. After fixation with 0.025% paraformaldehyde, resting-state and activated-state platelets were coated overnight at 4°C in 0.1 M bicarbonate buffer (pH 9.6). During each washing step, plates were centrifuged at 3000 rpm for 5 minutes. After overnight coating of platelets, plates were washed 3 times with 1X PBS; wells containing platelets were blocked with 5% BSA for 2 h at room temperature. Primary antibody (scFv) was added into wells after washing with PBS, and incubated for 2 h at room temperature. Mouse anti-His-HRP conjugated antibody at a dilution of 1:10,000 was added to each well and incubated for 1 h at room temperature. Color was developed with TMB; the reaction was stopped with 1 N sulfuric acid, and absorption measurements on plates were done at 450 nm.
Purification of individual scFv antibodies
Each of the above scFv antibodies was expressed in large scale, and purified; HB2151 E coli cells were induced with IPTG under standard conditions, leading to expression of soluble scFv antibodies through the suppression of amber suppressor stop codon placed between the scFv and the pIII coat protein region of the phage genome. After infection with the phage, cells were grown overnight; next day 1% inoculum was added to fresh 2X TY media containing 100 μg/ml ampicillin and 0.1% glucose, and it was grown for 3 h at 37°C with shaking. The culture was then induced with 2 mM IPTG and induced with shaking at 30°C overnight, for expression of soluble scFv. The soluble scFvs were extracted from the periplasmic extract and purified using Ni-NTA agarose beads as per manufacture's protocol (Qiagen, Germany).
Immunoprecipitation of proteins from the native and denatured platelet lysate
To identify the target of scFv antibodies, immunoprecipitation experiments were performed with soluble scFvs (immobilized on protein-A agarose beads), using proteins extracted from native platelet lysate and denatured platelet lysate. For this purpose, soluble scFv antibodies were dialyzed with Tris NaCl buffer (50 mM Tris and 150 mM NaCl pH 7.5) for 2 h. For immobilization of scFv to Protein-A agarose beads, dialyzed scFv (0.5 mg/ml) was incubated with 50 µl of Protein-A agarose beads (50% slurry) for 2 h on the rocker. The unbound scFv was collected from the Protein-A agarose beads and scFv antibody/protein-A resin was washed with washing buffer (50 mM Tris and 150 mM NaCl pH 7.5) 2–3 times, followed by equilibration buffer (2–3 times). Resting and denatured platelet lysate were then incubated with scFv/Protein-A resin for 2 h on a rocker, respectively. The scFv conjugated protein-A beads were washed with wash buffer 4–6 times, followed by elution with 50 µl of elution buffer (0.1 M glycine buffer pH 2.5). Eluate fractions were analyzed on 10% SDS-PAGE, under reducing conditions.
Western blot
Immunoprecipitated protein fractions of scFv antibodies and platelet lysate (5 µg/well) were run under reducing conditions, and blotted onto nitrocellulose membrane. The presence of platelet integrin (αIIbβ3) was determined by western blotting using CD41a antibody (GPIIb) and CD 61 antibody (GPIIIa) in the respective gels. Briefly, eluted fractions were transferred to nitrocellulose membrane using the electro-transfer method. Nitrocellulose membrane was stained by 0.1% Ponceau S (MERCK Chemicals, USA) in 1% acetic acid for 2 min for visualizing the transferred bands and destained in PBS. The membrane was then washed 2 times with PBS buffer for 2 min and then incubated in 10% skim milk powder (Hi media, India) for 2 h at 37°C, followed by incubation with CD41a (1:2000) and CD61(1:10,000) antibodies (in 1% skim milk powder) overnight at 4°C in respective gels. Gels were then washed and incubated with HRP conjugated secondary antibody (1:20,000 dilution; Sigma, USA). The blot was developed with enhanced chemiluminescence.
LC-MS/MS
LC-MS/MS was carried out on Orbitrap Fusion mass spectrometer (Thermo Fisher Scientific) interfaced with Easy nLC1000 Ultra High Pressure Chromatography (Thermo Scientific, Bremen, Germany). Peptides were analyzed by reversed phase liquid chromatography, with a trap column (C18; 3 μm, 100 Å) and an analytical column (75 μ m × 2 cm, C18, 5 μm, 100 Å). The mobile phases were: phase A - 0.1% formic acid, 5% acetonitrile and phase B - 95% acetonitrile, 0.1% formic acid. Using a spray voltage of 1.7 kV and heated capillary temperature set to 250°C, mass spectrometry spectra were acquired in the m/z range of 400 to 1600. MS and MS/MS scans were acquired in an Orbitrap Fusion mass analyzer and the peptides were fragmented by higher energy collision dissociation. The mass spectrometry data obtained was searched against the Homo sapiens NCBI RefSeq fasta database using Proteome Discoverer, version 1.4 (Thermo Scientific, Bremen, Germany) workflow.
Immunostaining of platelets by scFv antibodies using confocal microscopy
The expression of integrins on the platelet surface was determined by immunostaining. For this, PRP was isolated from the citrated blood; resting or ADP-activated platelets were put on cover slip in 6-well plate, and the plate was centrifuged at 3000 rpm for 3 minutes. Centrifugation helps the platelets to attach firmly on the coverslip. Cells were fixed with 1% paraformaldehyde for 5 min and washed 3 times with PBS. Non-specific binding was reduced by incubating the platelets overnight at 4°C in blocking solution (5% BSA). FITC-conjugated scFv antibody was incubated for 1 h at room temperature (25°C; at concentration of 50 μg/ml). Coverslips were washed with PBS and mounted on slide using DPX Mountant. Slides were observed under the confocal microscope (Olympus; Tokyo, Japan) for expression of markers and staining patterns.
Inhibition of platelet aggregation
Platelet aggregation was determined by measuring the change in the optical density (i.e., light transmittance) of stirred PRP after addition of the agonist, ADP (5 µl, 10 µM) to the aggregometer cuvette, using the previously described protocol.Citation21 Solutions of scFv antibodies were used at a concentration of 0–50 µg/ml, and incubated with PRP for 20 min; ADP (5 µl, 10 µM) was added to the sample, and changes in light transmission were recorded with the light transmission for PPP (platelet-poor plasma) sample in one channel representing 100% light transmittance, and the PRP sample in another channel representing 0% transmittance. Platelet aggregation was measured and maximum deflection was obtained after 3 minutes of curve registration computed as percentage of maximal aggregation. The experiments were done with samples drawn from 5 different donors. Data were expressed as the mean ± SEM; statistical analysis was performed by analysis of variance (ANOVA) by Dunnett's test. The results were declared significant if P-value < 0.05. Significance was denoted as p < 0.05 (*); <0.01(**); <0.001(***).
Preparation of FITC-labeled scFv antibodies
scFv antibodies were labeled with FITC fluorochrome using manufacture's protocol. Briefly, scFv antibody (at concentration 0.5 mg/ml) was dialysed with sodium borate buffer pH 8.5 for 2 h. After dialysis, scFv antibody was collected, centrifuged and incubated with FITC in dark for 1 h at room temperature (added according to the concentration of antibody as described in manufacturer's protocol; Thermo scientific; Rockford, USA). After labeling, FITC conjugated scFv antibody was centrifuged and the excess fluorochrome was removed by a desalting column. The FITC conjugated scFv antibody fractions were collected and absorbance was measured.
Funding
MLG thanks the Department of Biotechnology (DBT), Govt. of India, for funding the study.
Flow cytometric evaluation of platelet activation
The protocol used to determine the status of platelet activation was the same as reported by us earlier;Citation7 platelets (1 × 105) were incubated with antibodies specific for activation markers P-selectin (PE-CD62P) and FITC-PAC-1 (used at a concentration of 1 µg/test each), or FITC-labeled scFv antibodies (used at a concentration of 3.3 µg/test, determined by titration as being the optimal concentration to be used). The suspension was incubated for 45 minutes in dark at 30°C. In each case, mean fluorescence intensity (MFI) of binding was observed and activation fold was determined in terms of MFI of ITP patient vs. MFI of healthy control for binding of each antibody. The number of patients for PAC-1 and P-selectin antibodies was 24. For the scFv antibodies, the number of patients were as follows: scFv R87: n = 14; scFv R38: n = 9; scFv O52: n = 9; scFv N87: n = 14. Data is represented as ±SEM. Since the data was normally distributed and passed the normality test, unpaired t test was used. Comparisons were made between 2 groups at a time, and results were declared significant if P-value < 0.05. Significance was denoted as p < 0.05 (*); < 0.005(**).
Disclosure of Potential Conflicts of Interest
No potential conflicts of interest were disclosed.
Supplemental Material
Supplemental data for this article can be accessed on the publisher's website.
Supplemental_Material.zip
Download Zip (1,008.8 KB)Acknowledgments
PB thanks the UGC for providing fellowship. Thermo Fisher Scientific (Bangalore, India) is thanked for LC-MS MS data and analysis.
References
- McMillan R. The pathogenesis of chronic immune thrombocytopenic purpura. Semin Hematol 2007; 44:S3-S11; PMID:18096470; http://dx.doi.org/10.1053/j.seminhematol.2007.11.002
- McMillan R. Autoantibodies and autoantigens in chronic immune thrombocytopenic purpura. Semin Hematol 2000; 37(3):239-48; PMID:10942218; http://dx.doi.org/10.1016/S0037-1963(00)90102-1
- Beardsley DS, Ertem M. Platelet autoantibodies in immune thrombocytopenic purpura. Transfus Sci 1998; 19:237-44; PMID:10351135; http://dx.doi.org/10.1016/S0955-3886(98)00037-X
- Hou M(1), Stockelberg D, Kutti J, Wadenvik H. Antibodies against platelet GPIb/IX, GPIIb/IIIa, and other platelet antigens in chronic idiopathic thrombocytopenic purpura. Eur J Haematol 1995; 55:307-14; PMID:7493677; http://dx.doi.org/10.1111/j.1600-0609.1995.tb00702.x
- Iraqi M, Perdomo J, Yan F, Choi P, Chong BH. Immune thrombocytopenia: antiplatelet autoantibodies inhibit proplatelet formation by megakaryocytes and impair platelet production in vitro. Haematologica 2015; 100:623-32; PMID:25682608; http://dx.doi.org/10.3324/haematol.2014.115634
- Lev PR, Grodzielski M, Goette NP, Glembotsky AC, Espasandin YR, Pierdominici MS, Contrufo G, Montero VS, Ferrari L, Molinas FC, Heller PG, Marta RF. Impaired proplatelet formation in immune thrombocytopenia: a novel mechanism contributing to decreased platelet count. Br J Haematol 2014; 165:854-64; PMID:24673454; http://dx.doi.org/10.1111/bjh.12832
- Bhoria P, Sharma S, Varma N, Malhotra P, Varma S, Luthra-Guptasarma M. Effect of steroids on the activation status of platelets in patients with Immune thrombocytopenia (ITP). Platelets 2015; 26:119-26; PMID:24617442; http://dx.doi.org/10.3109/09537104.2014.888546
- Schwab C, Bosshard HR. Caveats for the use of surface-adsorbed protein antigen to test the specificity of antibodies. J Immunol Methods 1992; 147:125-34; PMID:1311735; http://dx.doi.org/10.1016/S0022-1759(12)80037-8
- Jacobin MJ, Robert R, Pouns O, Laroche-Traineau J, Nurden A, Peter K, Little M, Clofent-Sanchez G. Improving selection of alphaIIbbeta3-binding phage antibodies with increased reactivity derived from immunized donors. Clin Immunol 2003; 108:199-210; PMID:14499243
- Fullard JF. The role of the platelet glycoprotein IIb/IIIa in thrombosis and haemostasis. Curr Pharm Des 2004; 10:1567-76; http://dx.doi.org/10.2174/1381612043384682
- Bennett JS. Structure and function of the platelet integrin alphaIIbbeta3. J Clin Invest 2005; 115:3363-9; PMID:16322781; http://dx.doi.org/10.1172/JCI26989
- Shattil SJ, Newman PJ. Integrins: dynamic scaffolds for adhesion and signaling in platelets. Blood 2004; 104:1606-15; PMID:15205259; http://dx.doi.org/10.1182/blood-2004-04-1257
- Harrison P, Cramer EM. Platelet alpha-granules. Blood Rev 1993; 7:52-62; PMID:8467233; http://dx.doi.org/10.1016/0268-960X(93)90024-X
- McEver RP, Bennett EM, Martin MN. Identification of two structurally and functionally distinct sites on human platelet membrane glycoprotein IIb-IIIa using monoclonal antibodies. J Biol Chem 1983; 258:5269-75; PMID:6220015
- Newman PJ, Allen RW, Kahn RA, Kunicki TJ. Quantitation of membrane glycoprotein IIIa on intact human platelets using the monoclonal antibody, AP-3. Blood 1985; 65:227-32; PMID:3155488
- Chen P, Sun CX, Liu JN. A novel anti-platelet monoclonal antibody (3C7) specific for the complex of integrin alpha IIb beta3 inhibits platelet aggregation and adhesion. J Biol Chem 2005; 280:25403-8; PMID:15890654; http://dx.doi.org/10.1074/jbc.M500462200
- McMillan R, Lopez-Dee J, Loftus JC. Autoantibodies to alpha(IIb)beta(3) in patients with chronic immune thrombocytopenic purpura bind primarily to epitopes on alpha(IIb). Blood 2001; 97:2171-2; PMID:11264188; http://dx.doi.org/10.1182/blood.V97.7.2171
- Bednar RA, Gaul SL, Hamill TG, Egbertson MS, Shafer JA, Hartman GD, Gould RJ, Bednar B. Identification of low molecular weight GP IIb/IIIa antagonists that bind preferentially to activated platelets. J Pharmacol Exp Ther 1998; 285:1317-26; PMID:9618439
- Neunert C, Lim W, Crowther M, Cohen A, Solberg Jr L, Crowther MA. The American Society of Hematology 2011 evidence-based practice guideline for immune thrombocytopenia. Blood 2011; 117:4190-207; PMID:21325604; http://dx.doi.org/10.1182/blood-2010-08-302984
- Sharma R, Vasishta RK, Sen RK, Luthra-Guptasarma M. Refolding of HLA-B27 heavy chains in the absence of beta2m yields stable high molecular weight (HMW) protein forms displaying native-like as well as non-native-like conformational features: implications for autoimmune disease. BiochimBiophysActa 2007; 1772:1258-69; PMID:18036353
- Schwarz M, Meade G, Stoll P, Ylanne J, Bassler N, Chen YC, Hagemeyer CE, Ahrens I, Moran N, Kenn D. et al. Conformation-specific blockade of the integrin GPIIb/IIIa: a novel antiplatelet strategy that selectively targets activated platelets. Circ Res 2006; 99:25-33; PMID:16778135; http://dx.doi.org/10.1161/01.RES.0000232317.84122.0c