Abstract
X-ray structure analysis of 4 antibody Fab fragments, each in complex with human granulocyte macrophage colony stimulating factor (GM-CSF), was performed to investigate the changes at the protein-protein binding interface during the course of in vitro affinity maturation by phage display selection. The parental antibody MOR03929 was compared to its derivatives MOR04252 (CDR-H2 optimized), MOR04302 (CDR-L3 optimized) and MOR04357 (CDR-H2 and CDR-L3 optimized). All antibodies bind to a conformational epitope that can be divided into 3 sub-epitopes. Specifically, MOR04357 binds to a region close to the GM-CSF N-terminus (residues 11–24), a short second sub-epitope (residues 83–89) and a third at the C-terminus (residues 112–123). Modifications introduced during affinity maturation in CDR-H2 and CDR-L3 led to the establishment of additional hydrogen bonds and van der Waals contacts, respectively, providing a rationale for the observed improvement in binding affinity and neutralization potency. Once GM-CSF is complexed to the antibodies, modeling predicts a sterical clash with GM-CSF binding to GM-CSF receptor α and β chain. This predicted mutually exclusive binding was confirmed by a GM-CSF receptor α chain ligand binding inhibition assay. Finally, high throughput sequencing of clones obtained after affinity maturation phage display pannings revealed highly selected consensus sequences for CDR-H2 as well for CDR-L3, which are in accordance with the sequence of the highest affinity antibody MOR04357. The resolved crystal structures highlight the criticality of these strongly selected residues for high affinity interaction with GM-CSF.
Abbreviations
GM-CSF | = | granulocyte macrophage colony stimulating factor |
GM-CSFR | = | granulocyte macrophage colony stimulating factor receptor |
Fab | = | fragment, antigen binding |
ASA | = | accessible solvent area |
SC | = | surface complementarity |
CDR | = | complementarity-determining region. |
Introduction
Granulocyte macrophage colony-stimulating factor (GM-CSF) was originally identified as a hematopoietic growth factor based on its property to promote macrophage and granulocyte colony formation in vitro.Citation1 GM-CSF-/- mice, however, show unperturbed steady-state cell counts in peripheral blood and bone marrow progenitor cells, indicating that GM-CSF is not an essential growth factor for basal hematopoiesis.Citation2,3 Increasingly, GM-CSF is recognized to act on mature cells of the myeloid linage to promote differentiation, activation and survival of monocytes/macrophages, granulocytes and dendritic cells.Citation4 GM-CSF depletion by means of neutralizing antibodies resulted in anti-inflammatory effects in several animal models of autoimmune diseases like rheumatoid arthritis and multiple sclerosis.Citation5-8 Thus, GM-CSF is considered as a promising target in inflammatory diseases.Citation4,9-11
GM-CSF is a monomerCitation12 and interacts with a receptor complex consisting of 2 transmembrane glycoprotein subunits, known as GM-CSFR α and β. GM-CSFR α initially associates with GM-CSF with nanomolar affinity.Citation13,14 Subsequently the β chain is recruited to form a ternary complex binding GM-CSF with sub-nanomolar affinityCitation15 and signaling via the β chain is initiated. Crystal structure analysis of the human ternary GM-CSF:GM-CSFR complex demonstrated assembly into a higher order dodecameric structure.Citation16,17
Derived from the HuCAL GOLD phage display library, anti-GM-CSF MOR04357, also known as MOR103, is a human monoclonal antibody that has been engineered by in vitro affinity maturation.Citation18 In order to achieve very high affinity and increased functional activity, the library-derived parental antibody MOR03929 was modified in CDR-L3 and parallel in CDR-H2 using trinucleotide consensus cassettes. The immunoglobulin Ig light and Ig heavy chains of the obtained affinity-optimized variants MOR04252 (CDR-H2 optimized) and MOR04302 (CDR-L3 optimized) were finally combined to generate MOR04357. This antibody showed a 5,000 fold improved affinity compared to the parental clone, reaching a KD of 400 fM.Citation17
In the present study, we sought to retrace the changes in the antibody-GM-CSF binding interface during the course of this in vitro affinity maturation process. To this end, protein crystals of each of the 4 antibodies in complex with GM-CSF were generated and analyzed using X-ray crystallography. The crystal structures of the MOR04357 Fab by itself and in complex with GM-CSF, as well as its predecessors MOR03929, MOR04252 and MOR04302 in complex with GM-CSF, were determined between 1.88 Å and 2.95 Å resolution. The information we obtained on contact residues and sterical orientation of the antibody and GM-CSF molecule provides an explanation for why binding of MOR04357 precludes binding of the cytokine to its receptor, which allows the antibody to act as a potent neutralizing molecule.
Results
Crystal structure of MOR03929, MOR04252, MOR04302 and MOR04357 in complex with human GM-CSF
The high affinity GM-CSF-specific antibody MOR04357 was generated by in vitro affinity maturation process using a phage display approach and cross cloning.Citation17 provides a schematic overview of the affinity maturation, the resulting antibodies and the amino acid modifications introduced in each step of the process.
Figure 1: (a) Schematic representation of the in vitro affinity maturation steps applied in the selection of high affinity GM-CSF antibodies. KD values are indicated. Citation17 (b) Sequence overview of CDR-H2 and CDR-L3 of anti-GM-CSF antibodies MOR03929, MOR04302, MOR04252 and MOR04357. Kabat Citation27 numbering scheme is shown.
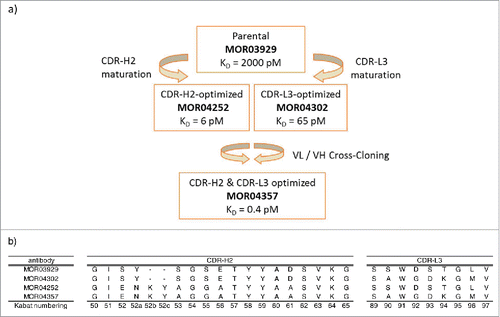
We have now solved crystal structures of GM-CSF in complex with the parental Fab fragment (MOR03929), 2 affinity maturated variants thereof (MOR04252 and MOR04302) that vary in the CDR-H2 or CDR-L3, respectively, and the combined (“cross-cloned”) product of the affinity matured VL and VH variants (MOR04357). These crystal structures represent snapshots of a stepwise 5,000 fold increase of affinity during an in vitro maturation process.Citation17
By crystallization, we obtained protein crystals belonging to 2 different crystal forms, in which MOR03929 and MOR04302 in complex with GM-CSF belong to crystal form I (space group I 21 3) and MOR04252 and MOR04357 in complex with GM-CSF belong to crystal form II (space group P 21 21 21) and contain one or 2 GM-CSF:Fab-complexes, respectively, in the asymmetric unit (ASU). X-ray data collection statistics are given in Table S1 (supplement). The structure of uncomplexed MOR04357 had been solved previously (data not shown).
Structure of GM-CSF
Human GM-CSF is a monomeric, globular cytokine comprising an antiparallel, left-handed 4 α-helix bundle. These helices are interconnected via 2 overhand loops, one of which contains a 2-stranded β-sheet.Citation31 The structure is well resolved in all 4 complexes analyzed between 2.06 Å and 2.95 Å resolution after refinement (Table S2, supplement). shows the complex structure of MOR04357:GM-CSF. Superposition of all 4 structures (according to Cα positions of GM-CSF) reveal highly similar conformations of GM-CSF molecules when bound to the 4 different Fab molecules (rms GM-CSF Cα = 0.24 – 0.37 Å) as depicted in . Furthermore, they are also highly comparable to the X-ray structure of uncomplexed recombinantly expressed human GM-CSF (rms Cα = 0.48 − 0.51 Å) (PDB ID: 1CSG; ref. 31), which gives an estimate of the accuracy of the models. Only two slight shifts in non-epitope regions of GM-CSF molecules of 2.3 Å (rms Cα = 1.29 Å) of the loop regions between helix A and ß-sheet 1 in structures with MOR04252 and MOR04357 compared to MOR03929 and MOR04302, as well as the loop region connecting helix B and C with a shift of 3.5 Å (rms Cα = 1.65 Å), could be observed, which are potentially products of crystal packing forces.
Figure 2. Overall structure and superposition of all 4 Fab:GM-CSF complexes. (a) Structure of MOR04357:GM-CSF as cartoon presentation. Helices are numbered as letters A-D and β-sheet as numbers 1 and 2. N- and C-termini are depicted as N and C. MOR04357 Ig light chain is colored in green, the Ig heavy chain in blue. CDR regions are shown as L1-L3 and H1-H3 with respect to the corresponding chain. (b) GM-CSF and the variable domain of Fab fragment as ribbon presentation. Complex of MOR03929:GM-CSF is presented in yellow, MOR04252:GM-CSF in green, MOR04302:GM-CSF in blue and MOR04357:GM-CSF in red.
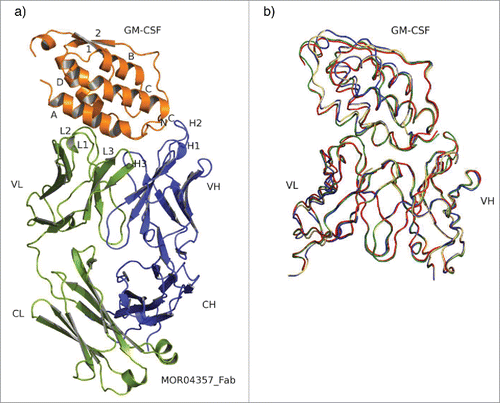
Structure of Fab molecules
The anti-GM-CSF Fabs are structured in 2 antiparallel β-sheets packed tightly against each other. A comparison of Fab MOR04357 free and in complex with GM-CSF yields an rmsd-value of 0.32 Å for all Cα atoms, regarding non-complementarity-determining region (CDR) residues in the variable (Fv-) domain. This indicates that upon complex formation the variable domain-interface does not change significantly with respect to the light and heavy chain orientation. In addition, the 4 different Fab:GM-CSF complexes were superimposed (Cα of non-CDR residues of Fv) to reveal structural changes introduced in the maturation process. Rmsd values of 0.68 Å, 0.24 Å and 0.65 Å were determined between MOR04357 and MOR04302, MOR04252 and MOR03929, respectively, which indicates a good superposition of all 4 complexes. This result indicates that only minor conformational differences between framework regions of all 4 complexes result from maturation process. The interfaces between Ig light and Ig heavy chains of all 4 Fabs were structurally not influenced by affinity maturation regarding their orientation to each other.
Remarkably, there is a rotation of about 39° between residues V47 and F62 in the CDR-L2 loop toward and along GM-CSF in the complex structure of MOR04357:GM-CSF (bound state) compared to the free MOR04357 structure. This rotation is introduced by flipping the psi angle of V47 from 64.5° to 154.4° (). Interestingly, in crystal form I (MOR03929:GM-CSF and MOR04302:GM-CSF), this loop is not fully resolved, while in crystal form II (MOR04252 and MOR04357) it is resolved, which is an indication for general high flexibility within this loop structure. Therefore, this backbone flipping of V47 in VL resulting in interaction of CDR-L2 with GM-CSF gives evidence for an induced fit mechanism for this particular loop.
Figure 3. Cartoon representation of free MOR04357 (pale colors) and MOR04357 in complex with GM-CSF (dark colors). GM-CSF is presented in orange, the Ig light chain in green colors and the Ig heavy chain in blue colors. Respective CDR-L2 loops are presented in yellow and light yellow. Distances are given in angstrom and shown in red, angles are given in degree [°] and presented in black. Val-47 of both structures are shown as stick presentation. Val-47 undergoes a backbone flip from −64.5° to 154.4° when MOR04357 is bound to GM-CSF compared to the uncomplexed MOR04357. This backbone flip results in a shift of 8.2 Å of this loop toward GM-CSF along with a rotation by 39°.
![Figure 3. Cartoon representation of free MOR04357 (pale colors) and MOR04357 in complex with GM-CSF (dark colors). GM-CSF is presented in orange, the Ig light chain in green colors and the Ig heavy chain in blue colors. Respective CDR-L2 loops are presented in yellow and light yellow. Distances are given in angstrom and shown in red, angles are given in degree [°] and presented in black. Val-47 of both structures are shown as stick presentation. Val-47 undergoes a backbone flip from −64.5° to 154.4° when MOR04357 is bound to GM-CSF compared to the uncomplexed MOR04357. This backbone flip results in a shift of 8.2 Å of this loop toward GM-CSF along with a rotation by 39°.](/cms/asset/e6e74296-597a-4b1d-962d-04d0225316e2/kmab_a_1099774_f0003_c.gif)
No other dominant changes in free versus bound MOR04357 were detected, including the matured CDR-H2 and CDR-L3, which indicates that the binding in the remaining epitope primarily follows a lock-and-key mechanism.
Epitope description
All four Fab molecules bind to a discontinuous epitope of a contiguous patch on the convex-shaped surface of GM-CSF involving all 6 CDR loops. This epitope, based on a 4.0 Å distance cut-off,Citation28 is similar between all 4 Fab molecules as shown by superposing the complex structures (). The epitope of MOR04357 can be divided into 3 sub-epitopes: a first region close to the N-terminus of GM-CSF comprising residues 11–24 of helix A, secondly a short sub-epitope comprising residues of helix C and the loop region connecting helix C with β-strand 2 (residues 83–89), as well as a third region of helix D and further residues belonging to the C-terminal tail of GM-CSF (residues 112–123). Overall, the epitope recognized by MOR04357 involves 23 residues, 13 interact with the VL domain, 8 interact with the VH domain and 2 are recognized by both domains of the Fab molecule ().
Table 1. Overview table listing contact sites of MOR04357 to GM-CSF.
A sequence alignment of human, rhesus and rat GM-CSF was performed to identify which of these 23 residues are conserved in those species in which cross-reactivity has been reported (). All 23 of the 23 interacting residues are conserved in rhesus GM-CSF and 12 of 23 are conserved in rat GM-CSF. This result is consistent with the finding that MOR04357 shows equal potency in neutralizing human and rhesus GM-CSF, while rat GM-CSF is neutralized less potently by 3 orders of magnitude.Citation17
Figure 4. Alignment (http://www.jalview.org Citation49) of human, rhesus and rat GM-CSF amino acid sequences. Contacted residues of MOR04357 Fab on human GM-CSF are highlighted in black, supplemented by secondary structure information. Sequence identity to rhesus and rat GM-CSF in the respective regions, is depicted in gray.
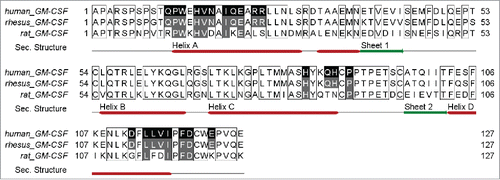
Defined consensus sequences were selected for CDR-H2 and CDR-L3 during in vitro affinity maturation
As described above, engineering of the parental antibody MOR03929 was carried out using parallel CDR-H2 and CDR-L3 diversified antibody libraries. After three rounds of panning, individual clones were screened for improvement in antigen binding.Citation17 In addition, we utilized high throughput sequencing to characterize the sequence diversity that was recovered after 3 rounds of phage display selection in more detail. In total, 4,761 and 2,606 sequences were analyzed after the third round panning of the CDR-L3 and CDR-H2 phage libraries, and 2,381 and 555 unique sequences, respectively, were found. Of these, 38 unique CDR-H2 sequences and 70 unique CDR-L3 sequences were found ≥5x in the sequenced pool, thus representing the most strongly selected variants. Sequence alignments of those selected variants revealed one 19 amino acid major consensus sequence () for CDR-H2, while for CDR-L3 one major consensus sequence of 8 amino acids () was strongly selected.
Figure 5. Consensus sequences are depicted by using the Sequence Logo tool, weighing for frequency of amino acids (http://genome.tugraz.at/Logo/ Citation50). The residue numbering shown is according to Kabat. Citation27(a) CDR-H2 consensus representing 38 unique sequences. (b) CDR-L3 consensus representing 70 unique sequences.
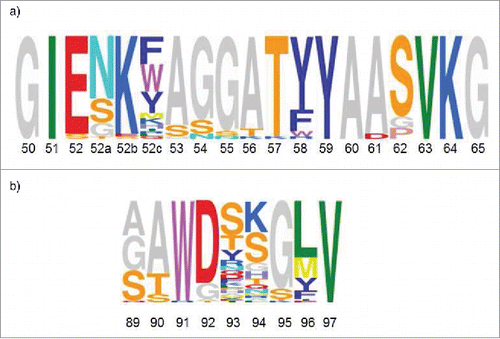
CDR-H2 (GIENKYAGGATYYAASVKG) and CDR-L3 (SAWGDKGMV) sequences of MOR04357 are concordant with the selected consensus sequences. Compared to the respective CDR-H2 (GISYSGSETYYADSVKG) and CDR-L3 (SSWDSTGLV) of the parental antibody MOR03929, it was evident that the CDR-H2 consensus sequence after affinity maturation was extended by 2 amino acids, while the selected CDR-L3 consensus kept the original length.
Altered protein interaction introduced by affinity maturation
As a result of the maturation process in CDR-H2, 2 additional amino acid residues were introduced (positions 52b and 52c) and several interactions to GM-CSF were additionally formed, affecting affinity and potency of MOR04252 (). The affinity-matured CDR-H2 loop of MOR04252 is depicted in superposition to its parental of MOR03929 in . As a new interaction, H87 of GM-CSF gets in contact via hydrogen bond by its backbone carbonyl to the δ-amino group of N52a. The interaction of the imidazole ring of GM-CSF H87 with the hydroxyl group of E56 present in MOR03929 is lost due to the maturation at this position (E56A) with strong consensus. E52 forms intra-loop hydrogen bonds to Y58 and A56 that appear to force the loop stability, which confirms the importance of this positional maturation. Surprisingly, Y58 lost the hydrogen bond interaction to T10 of GM-CSF due to this strong intra-chain interaction to E52. An additional rotation of the psi angle of N52a from −38.3 to −178.9° compared to the parental Y52a in MOR03929 results in an intra-loop hydrogen bond with the backbone amines of G54 and G55 of CDR-H2, and furthermore enables an intra-chain hydrogen bond of K52b backbone amine to the CDR-H1 S30 backbone carbonyl (not shown). These additional interactions in consequence force the stabilization of the loop itself. The rotation of the N52a psi angle leads also to a shift of the loop-tip toward the GM-CSF molecule. Due to this shift of the loop-tip and the additional introduction of 2 amino acids in the CDR-H2 loop in maturation, Y52c is now in an orientation where it sandwiches hydrophobically P89 of GM-CSF, shielding its apolar surface from solvent. Moreover the backbone carbonyl of A53 forms an additional hydrogen bond with the ε-amino group of GM-CSF Q86. A rather small amino acid at position 53 seems required due to a restricted space for the side chain, orienting inside the CDR-H2 loop. The maturation of E56 in MOR03929 to A56 in MOR04252 might be a reason for electrostatic repulsion based on the matured E52 and Y58 which are in close proximity to A56 in MOR04252. Interestingly, in all of 38 most abundant CDR-H2 matured sequences (), a glycine is present at position 50. This is likely due to restricted space toward any potential side chain facing W33 and W47 of the VH.
Figure 6. Stick and cartoon presentation of CDR-H2 of MOR03929 (pale blue) and MOR04252 (green) as well as GM-CSF (orange) from MOR04252:GM-CSF structure only. GM-CSF T10 from MOR03929:GM-CSF structure is shown as lines. Oxygen atoms are depicted in red, nitrogen atoms in blue. Distances are given in angstrom and depicted as dashed lines. The antibody residue numbering is according to Kabat. Citation27
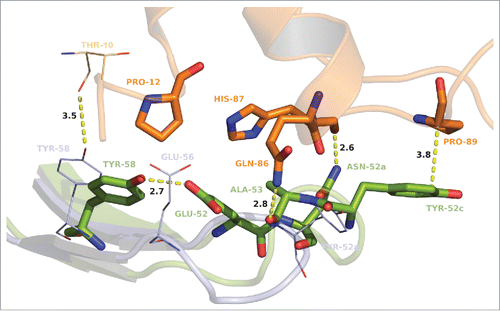
In the MOR04302:GM-CSF complex harboring a matured CDR-L3, the interactions between Fab and GM-CSF are overall comparable to the parental molecule. Only subtle changes in atom positions were observed. The affinity matured CDR-L3 loop of MOR04302 is depicted in superposition to its parental of MOR03929 in . Changes in intra-domain orientation might be an explanation for the increased affinity of MOR04302. This assumption is supported by the maturation of S90A. A small hydrophobic residue leads to less electrostatic repulsion forced by its hydrophobic surrounding (I2, L4, I28, K31) than S90 in MOR03929. W91, which remained unaltered after maturation, enables sandwich formation with W13 of GM-CSF and fits into the hydrophobic pocket arranged by VH chain residues (W33, W47 and F96). Additionally, the side chain of W91 forms a hydrogen bond to the backbone carbonyl of VH F96 and hydroxide of VL Y34. As seen from the obtained CDR-L3 consensus sequence (), an aspartate at position 92 seems to be preferred. However, in the case of MOR04302, this position was presumably context dependent of the surrounding residues matured to a glycine, which might lead to a beneficial orientation in terms of affinity of the backbone carbonyl of G92 to the indole ring of W13 of GM-CSF, forming a hydrogen bond.
Figure 7. Stick and cartoon presentation of CDR-L3 of MOR03929 (pale blue) and MOR04302 (green) as well as GM-CSF (orange) from MOR04302:GM-CSF structure only. Oxygen atoms are depicted in red, nitrogen atoms in blue. Distances are given in angstrom and depicted as dashed lines. The antibody residue numbering is according to Kabat. Citation27
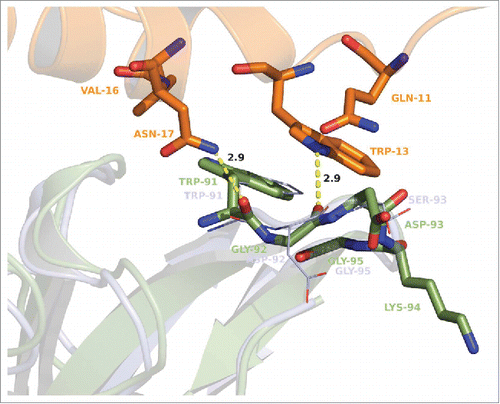
At position 95 in the VL chain, glycine is highly favored as it was not altered during affinity maturation. A perfect fitting into the hydrophobic pocket formed by W33, W47 and F96 of the VH chain and W91 of the same loop and W13 of GM-CSF forces a glycine at this position. At this position, any larger residue would lead to steric hindrance and a loss of affinity. In summary, amino acid changes introduced into CDR-L3 appear to provide several optimizations that lead to an increase of affinity and potency of the molecule compared to its parental.
MOR04357 combines the optimized protein-protein interactions of the single matured CDR-L3 and CDR-H2 of MOR04302 and MOR04252, which leads to an additional improvement in affinity. The combination of both matured VH and VL does not lead to steric interference with each other, as seen from superposition of all 4 complexes (). As a consequence of this cross cloning approach, the formation of the salt bridge between D61 in CDR-H2 and K94 in CDR-L3 as observed for the matured MOR04302 is no longer possible in the cross clone MOR04357 because D61 is matured to alanine in MOR04252. Notably, the loss of this particular salt bridge appears to facilitate a favored orientation of the MOR04357 CDR-L3 backbone, resulting in a higher-affinity interaction with GM-CSF, mainly triggered by W13.
This illustrates that, despite independent selection by phage display, a successive combination of individually matured VH and VL can preserve the individual molecular CDR interactions, and beyond that can yield additional improvement in affinity and potency.
Antigen binding is influenced by physico-chemical parameters like hydrophobicity, surface complementarity (SC) and charge.Citation32-34 To gain insight into the cause of the increase in affinity, we calculated the change of buried accessible solvent area after complexation and maturation (ΔΔASA) for both apolar and polar areas using the POPS algorithm,Citation35 as well as the SC.Citation36
The maturation of MOR04252 leads to an increase of buried apolar accessible solvent area (compared to the MOR03929 complex) after complexation (ΔΔASAapolar) of 32.7 Å2 and ΔΔASApolar of 2.7 Å2. For the MOR04302:GM-CSF complex, we could observe only a slight increase of 5.8 Å2 of ΔΔASAapolar. The increase of ΔΔASApolar was slightly higher than in the matured MOR04252 complex, namely 4.3 Å2. Interestingly, in the cross-clone Fab complex MOR04357:GM-CSF, the increase of ΔΔASAapolar was calculated to be 43 Å2, which is a slightly higher value than the sum of ΔΔASAapolar of the single matured Fabs and its complexes. It seems that there is a mutual influence of CDR-H2 and CDR-L3 concerning their paratope and its accessible solvent areas.
The SC in the parental complex MOR03929:GM-CSF is already very high, with a calculated value of 0.774 (calculated with default parameters), compared with the average SC value of 0.64 – 0.68 for antibody–antigen complexes reported by Lawrence and co-workers,Citation36 and it is also high compared to oligomeric proteins and proteinase-protein inhibitor complexes, which commonly exhibit SC values of 0.70 to 0.76.Citation37 After maturation of the CDR-H2 in MOR04252, the complex with GM-CSF exhibits a reduced SC value of 0.743. A similar reduction, to a value of 0.758, can be seen after maturation of CDR-L3 in MOR04302. After cross-cloning of both matured CDRs to resulting MOR04357, the complex reached a SC value of 0.750.
Molecular Basis of GM-CSF neutralization
Loop residues 241 to 251 and 299 to 305 of GM-CSFR α make contacts to residues 11 to 23 (helix A) and residues 112 to 118 (helix D) of GM-CSF.Citation16 Both stretches are fully covered by the contacts made by MOR04357 (). Superposition of MOR04357:GM-CSF and the published GMCSF:GMCSFR α:GMCSFR β structureCitation16 predicts mutually exclusive binding of GM-CSF to either the antibody or the receptor (). This superposition also indicates that antibody binding prohibits GM-CSF – GM-CSFR α as well as GM-CSFR β contacts. However, already blocking the first, low affinity interaction step between GM-CSF and GM-CSFR α chain is expected to prevent subsequent recruitment of the GM-CSFR β chain, and thus formation of the active, ternary receptor complex.
Figure 8. Superposition of GM-CSF:MOR04357 complex and GM-CSFR α/β:GM-CSF complex structure Citation16 on the basis of GM-CSF atom positions. GM-CSFR β chain is depicted in cartoon presentation as physiological dimer colored in green and violet. Incomplete GM-CSFR α chain is depicted in cartoon presentation colored in red. MOR04357 Fab molecule is presented in pale blue (Ig light chain) and dark blue (Ig heavy chain) shown as semi-transparent molecular surface. GM-CSF of MOR04357:GM-CSF complex structure is shown as cartoon presentation in orange.
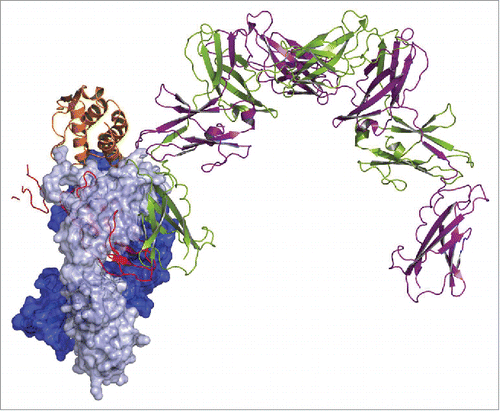
We sought to test whether the predicted mutually exclusive binding of GM-CSF to the 4 anti-GM-CSF Fab antibodies and the GM-CSFR α chain could be confirmed experimentally. To this end, a receptor inhibition ELISA detecting the binding of GM-CSF to immobilized recombinant GM-CSFR α-Fc was employed. Addition of GMCSF antibodies decreased the detectable receptor-associated GM-CSF (), showing that the antibodies bind to GM-CSF in solution and thereby subsequently prevent binding of the cytokine to its receptor. Consistent with the lower affinity to GM-CSF, parental MOR03929 showed less potent receptor inhibition compared to the 3 affinity matured variants, which reached the apparent detection limit of the assay (IC50 ∼50 pM).
Discussion
Comparison of 4 related Fab antibodies in complex with GM-CSF allowed us to retrace the structural changes introduced during in vitro affinity maturation using diversified CDR-H2 or CDR-L3 libraries. The CDR-H2 loop of the matured variant MOR04252 was extended by 2 residues and showed additional changes compared to the parental CDR-H2 sequence (). This completely remodeled CDR enabled formation of 2 new strong hydrogen bonds (N52a, A53) instead of a single weak one, and furthermore highly favored van der Waals contacts via Y52c. This improvement in interactions, along with an increased content of buried hydrophobic surface area from solvent (32.7 Å2), provides a rationale for the observed increase in affinity. This finding is in good agreement with the important contribution of hydrophobic effects in high affinity protein-protein interactions.Citation38-41
In contrast, interactions made by the affinity-matured CDR-L3 of MOR04302 introduced more subtle changes when compared to the parental antibody MOR03929. Despite the same number of hydrogen bonds involved in antigen binding, an increase in affinity was noted. The slight increase in apolar buried accessible surface area (5.8 Å2) is at least partially an explanation for the 30-fold affinity increase compared to the parental molecule. An additional contribution seems to be mediated by matured S90A, which decreases the electrostatic repulsion and enables an energetically beneficial interaction with GM-CSF due to a decreased torsional strain in the CDR-L3 loop.
The combination of both individually affinity matured VL and VH domains in MOR04357 joins the gain of both affinity improvements, resulting in a further increase in binding affinity.
It is described that up to a certain level of affinity, a gain in SC can be the driving force.Citation41 Further affinity improvement, however, can be the result of additional burying of hydrophobic area from solvent, even overcompensating a subsequent loss of SC. Here, we propose that the parental molecule MOR03929 has already reached an optimum level of SC (0.774), whereas further affinity gain is most probably the result of burying an increased hydrophobic area from solvent (ΔΔASAapolar = 43 Å2) actually accompanied by a slight decrease in SC as observed for MOR04357 (SC = 0.750).
High throughput sequencing of pools of antibody clones after 3 rounds of phage display selection shows that the sequences found in CDR-H2 of MOR04252 and CDR-L3 of MOR04302 are in accordance with the respective identified enriched consensus sequences. These consensus sequences represent structurally very similar variants that comply with a common structural theme each. The selection by phage display of similar amino acids at certain positions that follow these structural themes indicates that, within the applied library design, the structural sequence space was covered, enabling identification of the highest affinity members of the consensus families.
Important CDR-H2 interactions of MOR04357 are made by E52, N52a, Y52c, A53 and Y58 (). Residues E52, N52a, A53 and Y58 are the most dominantly selected amino acids at the respective positions, stressing their important contribution to high affinity binding (). For positions 52c and 58, predominantly aromatic amino acids are found in the consensus sequence. It is conceivable that the hydrophobic interactions by both tyrosine residues of MOR04357 at these positions could be replaced by other aromatic residues making similar intermolecular contacts.
In CDR-L3 the tryptophan sandwich mediated by W91 provides a lock-and-key mechanism, which was already present in the parental antibody MOR03929 and which was preserved after affinity maturation. The importance of this residue is confirmed by a very strong consensus selection at this position ().
The mechanism of interaction of the 4 related antibodies follows mainly a lock-and-key mechanism as can be deduced from the highly similar conformations of GMCSF molecules when bound to the 4 different Fab molecules and the high structural comparability of uncomplexed and complexed MOR04357. Due to the conformational difference in CDR-L2 in the bound and unbound state of MOR04357, we conclude that this particular loop follows an induced-fit mechanism. It is unclear whether the CDR-L2 loop initially binds to GM-CSF followed by a lock-and-key interaction of the remaining antibody, or whether the antibody interacts in a first step via lock-and-key to GM-CSF without the highly dynamic CDR-L2 loop, which in a second step associates with GM-CSF by an induced-fit mechanism.
In a previous study investigating structural changes during anti-VEGF antibody affinity maturation, Chen and coworkers observed, comparable to our findings, formation of additional van der Waals contacts and hydrogen bonds.Citation42 A strong contribution of additional hydrogen bonding and salt bridge formation was reported for affinity maturation of anti-botulinum neurotoxin antibodies; however, different to our findings, additional elimination of an electrostatic clash contributes to the binding affinity.Citation43 Also, in contrast to our findings, Li and co-workers did not identify additional hydrogen bonds and van der Waals contacts but an increase in SC as a contributor to affinity improvements in anti-hen egg white lysozyme antibodies. Otherwise, in accordance to our findings, additional burying of hydrophobic surface was identified as an important driver of antibody antigen interaction.Citation44 These studies collectively underline that affinity improvements can be achieved by multiple molecular mechanisms, which appear to be quite specific for each paratope-epitope interaction.
In a recent report, the crystal structure of another anti-GM-CSF Fab fragment was used to predict an Fab:GM-CSF structure by modeling as attempts to obtain Fab:GM-CSF co-crystals were not successful. Superposition of this modeled complex structure with the human GM-CSF:ternary GM-CSF receptor complex revealed only little overlap between receptor and Fab when bound to GM-CSF; this finding is in line with the modest neutralizing potency of this antibody.Citation45 The discontinuous epitope bound by MOR04357 includes amino acids I19, E21 and D112 of GM-CSF, which have been found to be critical for interaction with the GM-CSF receptor by mutational analyses.Citation46 In line with this, crystal structure analysis showed that residues 11 to 23 and 112 to 118 of GM-CSF interact with GM-CSFR α, with E21 additionally making contacts to the receptor β chain.Citation16 Both stretches are contacted by MOR04357 (). Thus, the resolved GM-CSF:MOR04357 complex explains the neutralizing function of this antibody, since interaction of both GM-CSF receptor subunits α and β are precluded after GM-CSF is complexed to MOR04357 (). While non-glycosylated GM-CSF was used to facilitate co-crystallization, glycosylated GM-CSF was used for testing the Fab antibodies for blocking of GM-CSF:GM-CSFR α binding (). The potent receptor blocking function observed in this ELISA assay is in line with previous findings that MOR04357 blocks the biological activity of glycosylated and un-glycosylated GM-CSF with very similar potency in a TF-1 proliferation assay.Citation17
Eberhardt and coworkers used a panel of monoclonal antibodies targeting GM-CSF to characterize their epitope specificities.Citation47 To identify discontinuous epitopes, they used combinatorial hexapeptide libraries and peptide scans. They could identify the conformation-dependent epitopes from helix A and the C-terminal tail of GM-CSF A18-E21-R23-R24-F119 and R23-E21-N17-W13 being bound by a moderately neutralizing monoclonal antibody called CC5B5. The epitope recognized by CC5B5 contains residues that are also recognized by MOR04357, namely W13, N17, E21, R23, R24 and F119, indicating that the N-terminal amino acid stretch in essence is identically contacted by both antibodies.
The high affinity and potent neutralizing properties of the GM-CSF antibody MOR04357 have been utilized to validate GM-CSF as a drug target for the treatment of inflammatory autoimmune diseases. In a Phase 1b/2a clinical trial in rheumatoid arthritis patients, MOR103 (i.e., MOR04357 in IgG1 format) was well tolerated and showed preliminary evidence of efficacy in patients with active disease.Citation48 Elucidating the binding mode by co-crystallization with GM-CSF reveals the molecular mode of action of this therapeutic drug candidate.
Materials & Methods
Protein purification and complex formation
Lyophilized recombinant human GM-CSF (Gentaur, Belgium) was reconstituted in ddH2O to a final concentration of 1.2 mg/mL. To remove the stabilizer HSA, GM-CSF was purified using size-exclusion chromatography. Therefore, a Superdex 75 16/60 (GE Healthcare) column was used with 10 mM Tris pH 7.1 and 150 mM NaCl as running buffer at a flow-rate of 0.5 mL/min. Peak-fractions were analyzed by non-reducing SDS-PAGE using 4–12% gradient gels (Invitrogen). Fractions containing GM-CSF were pooled and dialyzed twice against 2 L of 10 mM Tris pH 7.1 and 150 mM NaCl at 4°C overnight using Slide-A-Lyzer dialysis cassettes (MWCO = 10 kDa, Thermo Fisher Scientific). Fab fragments of MOR04357, MOR03929, MOR04302 and MOR04252 were generated as described previouslyCitation17,18 and dialyzed against the same buffer. Concentrations of GM-CSF and Fab fragments were determined using UV-Vis spectroscopy (Shimadzu, Duisburg, Germany) at 280 nm. Complexes of GM-CSF with the respective Fab fragment were assembled by mixing both proteins in a stoichiometric ratio of 1:1.
Crystallization and structure determination
Crystals of MOR04357 were grown by vapor diffusion hanging drop method by mixing 0.5 µL of protein at 10.5 mg/mL and 0.5 µL of reservoir solution (26% PEG3350, 0.2 M NaCl and 0.1 M Tris, pH 8.25) and incubation at 20°C for 10 days. Crystals were cryo-protected in reservoir solution containing 15% 2,3-butanediol and flash-frozen in liquid nitrogen. Crystallization of Fab:GM-CSF complex structures was set up using vapor-diffusion hanging-drop method at 20°C by mixing 0.1–0.5 µL of complex solution with the same volume of reservoir solution. Best conditions for crystallization could be identified using 100 mM Tris, pH 8.75, 200 mM LiSO4, 25–28% (w/v) PEG 3350 (crystal form I) or 100 mM sodium acetate, pH 4.0–4.3, 39–42% (w/v) PEG 400 (crystal form II) and a protein concentration of 28 mg/mL (MOR03929), 30 mg/mL (MOR04252), 35 mg/mL (MOR04302) or 40 mg/mL (MOR04357). For cryo-protection, crystals were either taken directly out of the drop (crystal form II) or transferred to reservoir solution supplemented with 2025% (v/v) PEG200 (crystal form I) and flash-cooled in liquid nitrogen. X-ray diffraction data were collected from single crystals at the SWISS LIGHT SOURCE (SLS, Villigen, Switzerland) using cryogenic conditions. Data were processed using the programs XDS and XSCALE.Citation19,20 Data collection statistics are presented in Table S1 (supplement).
The structures were solved by molecular replacement using MOLREP.Citation21 As a search model, the coordinates from high-resolution structure data of an anti-tetanus toxoid Fab (Protein Data Bank entry: 1AQKCitation22) were used for the uncomplexed MOR04357 structure. Complex structures were solved with the previously solved structure of the MOR04357 Fab fragment and human GM-CSF (Protein Data Bank entry: 2GMFCitation23) as search models. Model building and refinement were performed with the software suite CCP4Citation24 and COOT.Citation25 Water molecules were added to 3 σ contoured Fo – Fc electron density map and the model was refined using REFMAC5.Citation26 Refinement statistics are given in Table S2 (supplement). Figures of structural details were generated using PyMOL (The PyMOL Molecular Graphics System, Version 1.4.1, Schrödinger, LLC). The amino acid sequence numbering system of all Fab fragments is in accordance to Kabat.Citation27
Analysis of the structures
Accessible surface areas were calculated using SURFACECitation24 with a 1.4 Å probe radius. Van der Waals contacts are determined to be in a distance of ≤ 4 Å distance between 2 atoms of 2 neighboring residues.Citation28 Hydrogen bonds and salt bridges were determined using program PyMOL (The PyMOL Molecular Graphics System, Version 1.4.1, Schrödinger, LLC) in which superpositions (upon Cα positions), rms calculations and elbow angle determinationsCitation29 were also performed. Due to poor or non-existent electron density, in the atomic model of complex structures a few, mostly terminal residues are missing (Table S3, supplement).
Expression and Purification of recombinant human GM-CSFR α-Fc
The extracellular part of the GM-CSFR α chain cDNA was fused c-terminally to a human Fc-tag and cloned into expression plasmid pcDNA3.1 (Invitrogen). Human GM-CSFR α-Fc protein was purified post-transfection from eukaryotic cell-culture supernatant by Protein A affinity chromatography (mAb Select SuRe® - GE-Healthcare).
Receptor inhibition ELISA
Recombinant human GM-CSF (Leukine ®, Genzyme, USA) and chicken Avidin (Sigma-Aldrich, Germany) were either biotinylated (ECL Protein Biotinylation Module, GE Healthcare, Germany) or Sulfo-TAG labeled (MSD- Sulfo-TAG NHS Ester, Meso Scale Discovery, USA). A MSD Multi-Array® plate (Meso Scale Discovery, USA) was coated with 2 µg/mL recombinant GM-CSFR α-Fc protein. In parallel, Fabs were incubated with biotinylated GM-CSF (100 pM) for 30 min. The Fab:GM-CSF mixture was transferred to the assay plate for incubation of 1 h and washed, followed by addition of Sulfo-TAG labeled Avidin for the detection of bound biotinylated GM-CSF using a SECTOR® Imager 6000 (Meso Scale Discovery, USA). Data analysis and IC50 calculations were based on a 4 parametric non-linear curve fit using GraphPad Prism 5.04 (GraphPad Software, Inc., USA).
Sequencing of affinity maturation output pools
Trinucleotide-diversified CDR-H2 and CDR-L3 libraries of MOR03929 were generated and affinity maturation pannings were performed using phage display as described.Citation17 Plasmid DNA from E.coli glycerol stocks obtained after the third panning round was subjected to high throughput 454 sequencing using the GS Junior system (Roche, Switzerland) as described.Citation30
Protein Data Bank submission statement
Coordinates and structure factors have been deposited in the Protein Data Bank under accession numbers 5C7X, 5D70, 5D71, 5D72 and 5D7S.
Potential Conflicts of Interest
RE, DW, SH, RS, RO and SS are employees of MorphoSys AG and hold stock or stock options in MorphoSys AG.
Eylenstein etal Supplemental Data
Download PDF (100.2 KB)Acknowledgments
The authors like to thank Katrin Döhn, Anja Unzeitig and Martin Hessling for excellent technical assistance, as well as Jane Hughes and Thomas Tiller for scientific discussions on the manuscript.
Financial support by the German Federal Ministry of Education and Research and support by the Munich Biotech Cluster m4 initiative is gratefully acknowledged. HuCAL and HuCAL GOLD, are registered trademarks of MorphoSys AG.
Supplemental Material
Supplemental data for this article can be accessed on the publisher's website.
References
- Burgess AW, Metcalf D. The nature and action of granulocyte-macrophage colony stimulating factors. Blood 1980; 56:947-58; PMID:7002232
- Stanley E, Lieschke GJ, Grail D, Metcalf D, Hodgson G, Gall JA, Maher DW, Cebon J, Sinickas V, Dunn AR. Granulocyte/macrophage colony-stimulating factor-deficient mice show no major perturbation of hematopoiesis but develop a characteristic pulmonary pathology. Proc Natl Acad Sci USA 1994; 91:5592-6; PMID:8202532; http://dx.doi.org/10.1073/pnas.91.12.5592
- Dranoff G, Mulligan RC. Activities of granulocyte-macrophage colony-stimulating factor revealed by gene transfer and gene knockout studies. Stem Cells 1994; 12 Suppl 1:173-82; PMID:7696961
- Hamilton JA. Colony-stimulating factors in inflammation and autoimmunity. Nat Rev Immunol 2008; 8:533-44; PMID:18551128; http://dx.doi.org/10.1038/nri2356
- Cook AD, Braine EL, Campbell IK, Rich MJ, Hamilton JA. Blockade of collagen-induced arthritis post-onset by antibody to granulocyte-macrophage colony-stimulating factor (GM-CSF): requirement for GM-CSF in the effector phase of disease. Arthritis Res 2001; 3:293-8; PMID:11549370
- Plater-Zyberk C, Joosten LA, Helsen MM, Hepp J, Baeuerle PA, van den Berg WB. GM-CSF neutralisation suppresses inflammation and protects cartilage in acute streptococcal cell wall arthritis of mice. Ann Rheum Dis 2007; 66:452-7; PMID:17020908; http://dx.doi.org/10.1136/ard.2006.057182
- McQualter JL, Darwiche R, Ewing C, Onuki M, Kay TW, Hamilton JA, Reid HH, Bernard CC. Granulocyte macrophage colony-stimulating factor: a new putative therapeutic target in multiple sclerosis. J Exp Med 2001; 194:873-82; PMID:11581310; http://dx.doi.org/10.1084/jem.194.7.873
- Codarri L, Gyulveszi G, Tosevski V, Hesske L, Fontana A, Magnenat L, Suter T, Becher B. RORgammat drives production of the cytokine GM-CSF in helper T cells, which is essential for the effector phase of autoimmune neuroinflammation. Nat Immunol 2011; 12:560-7; PMID:21516112; http://dx.doi.org/10.1038/ni.2027
- Cornish AL, Campbell IK, McKenzie BS, Chatfield S, Wicks IP. G-CSF and GM-CSF as therapeutic targets in rheumatoid arthritis. Nat Rev Rheumatol 2009; 5:554-9; PMID:19798030; http://dx.doi.org/10.1038/nrrheum.2009.178
- Hamilton JA, Tak PP. The dynamics of macrophage lineage populations in inflammatory and autoimmune diseases. Arthritis Rheum. 2009; 60:1210-21; PMID:19404968; http://dx.doi.org/10.1002/art.24505
- Greven DE, Cohen ES, Gerlag DM, Campbell J, Woods J, Davis N, van Nieuwenhuijze A, Lewis A, Heasmen S, McCourt M, et al. Preclinical characterisation of the GM-CSF receptor as a therapeutic target in rheumatoid arthritis. Ann Rheum Dis 2015; 74:1924-30.
- Pandit J, Bohm A, Jancarik J, Halenbeck R, Koths K, Kim SH. Three-dimensional structure of dimeric human recombinant macrophage colony-stimulating factor. Science 1992; 258:1358-62; PMID:1455231; http://dx.doi.org/10.1126/science.1455231
- Gasson JC, Kaufman SE, Weisbart RH, Tomonaga M, Golde DW. High-affinity binding of granulocyte-macrophage colony-stimulating factor to normal and leukemic human myeloid cells. Proc Natl Acad Sci USA 1986; 83:669-73; PMID:3484823; http://dx.doi.org/10.1073/pnas.83.3.669
- Gearing DP, King JA, Gough NM, Nicola NA. Expression cloning of a receptor for human granulocyte-macrophage colony-stimulating factor. EMBO J. 1989; 8:3667-76; PMID:2555171
- Hoang T, De LA, Haman A, Beauchemin V, Kitamura T, Clark SC. The structure and dynamics of the granulocyte macrophage colony-stimulating factor receptor defined by the ternary complex model. J Biol Chem 1993; 268:11881-7; PMID:8505314
- Hansen G, Hercus TR, McClure BJ, Stomski FC, Dottore M, Powell J, Ramshaw H, Woodcock JM, Xu Y, Guthridge M, et al. The structure of the GM-CSF receptor complex reveals a distinct mode of cytokine receptor activation. Cell 2008; 134:496-507; PMID:18692472; http://dx.doi.org/10.1016/j.cell.2008.05.053
- Steidl S, Ratsch O, Brocks B, Durr M, Thomassen-Wolf E. In vitro affinity maturation of human GM-CSF antibodies by targeted CDR-diversification. Mol Immunol 2008; 46:135-44; PMID:18722015; http://dx.doi.org/10.1016/j.molimm.2008.07.013
- Rothe C, Urlinger S, Lohning C, Prassler J, Stark Y, Jager U, Hubner B, Bardroff M, Pradel I, Boss M, et al. The human combinatorial antibody library HuCAL GOLD combines diversification of all six CDRs according to the natural immune system with a novel display method for efficient selection of high-affinity antibodies. J Mol Biol 2008; 376:1182-200; PMID:18191144; http://dx.doi.org/10.1016/j.jmb.2007.12.018
- Kabsch W. Integration, scaling, space-group assignment and post-refinement. Acta Crystallogr D Biol Crystallogr 2010; 66:133-44; PMID:20124693; http://dx.doi.org/10.1107/S0907444909047374
- Kabsch W. XDS. Acta Crystallogr D Biol Crystallogr 2010; 66:125-32; PMID:20124692; http://dx.doi.org/10.1107/S0907444909047337
- Vagin A, Teplyakov A. Molecular replacement with MOLREP. Acta Crystallogr D Biol Crystallogr 2010; 66:22-5; PMID:20057045; http://dx.doi.org/10.1107/S0907444909042589
- Faber C, Shan L, Fan Z, Guddat LW, Furebring C, Ohlin M, Borrebaeck CA, Edmundson AB. Three-dimensional structure of a human Fab with high affinity for tetanus toxoid. Immunotechnology. 1998; 3:253-70; PMID:9530559; http://dx.doi.org/10.1016/S1380-2933(97)10003-3
- Rozwarski DA, Diederichs K, Hecht R, Boone T, Karplus PA. Refined crystal structure and mutagenesis of human granulocyte-macrophage colony-stimulating factor. Proteins 1996; 26:304-13; PMID:8953651; http://dx.doi.org/10.1002/(SICI)1097-0134(199611)26:3%3c304::AID-PROT6%3e3.0.CO;2-D
- Winn MD, Ballard CC, Cowtan KD, Dodson EJ, Emsley P, Evans PR, Keegan RM, Krissinel EB, Leslie AG, McCoy A, et al. Overview of the CCP4 suite and current developments. Acta Crystallogr D Biol Crystallogr 2011; 67:235-42; PMID:21460441; http://dx.doi.org/10.1107/S0907444910045749
- Emsley P, Cowtan K. Coot: model-building tools for molecular graphics. Acta Crystallogr D Biol Crystallogr 2004; 60:2126-32; PMID:15572765; http://dx.doi.org/10.1107/S0907444904019158
- Murshudov GN, Vagin AA, Dodson EJ. Refinement of macromolecular structures by the maximum-likelihood method. Acta Crystallogr D Biol Crystallogr 1997; 53:240-55; PMID:15299926; http://dx.doi.org/10.1107/S0907444996012255
- Wu TT, Kabat EA. An analysis of the sequences of the variable regions of Bence Jones proteins and myeloma light chains and their implications for antibody complementarity. J Exp Med 1970; 132:211-50; PMID:5508247; http://dx.doi.org/10.1084/jem.132.2.211
- Narciso JE, Uy ID, Cabang AB, Chavez JF, Pablo JL, Padilla-Concepcion GP, Padlan EA. Analysis of the antibody structure based on high-resolution crystallographic studies. N Biotechnol 2011; 28:435-47; PMID:21477671; http://dx.doi.org/10.1016/j.nbt.2011.03.012
- Stanfield RL, Gorny MK, Zolla-Pazner S, Wilson IA. Crystal structures of human immunodeficiency virus type 1 (HIV-1) neutralizing antibody 2219 in complex with three different V3 peptides reveal a new binding mode for HIV-1 cross-reactivity. J Virol 2006; 80:6093-105; PMID:16731948; http://dx.doi.org/10.1128/JVI.00205-06
- Tiller T, Schuster I, Deppe D, Siegers K, Strohner R, Herrmann T, Berenguer M, Poujol D, Stehle J, Stark Y, et al. A fully synthetic human Fab antibody library based on fixed VH/VL framework pairings with favorable biophysical properties. MAbs. 2013; 5:445-70; PMID:23571156; http://dx.doi.org/10.4161/mabs.24218
- Walter MR, Cook WJ, Ealick SE, Nagabhushan TL, Trotta PP, Bugg CE. Three-dimensional structure of recombinant human granulocyte-macrophage colony-stimulating factor. J Mol Biol 1992; 224:1075-85; PMID:1569568; http://dx.doi.org/10.1016/0022-2836(92)90470-5
- Sundberg EJ, Urrutia M, Braden BC, Isern J, Tsuchiya D, Fields BA, Malchiodi EL, Tormo J, Schwarz FP, Mariuzza RA. Estimation of the hydrophobic effect in an antigen-antibody protein-protein interface. Biochemistry 2000; 39:15375-87; PMID:11112523; http://dx.doi.org/10.1021/bi000704l
- Sundberg EJ, Mariuzza RA. Luxury accommodations: the expanding role of structural plasticity in protein-protein interactions. Structure. 2000; 8: R137-R142; PMID:10903952; http://dx.doi.org/10.1016/S0969-2126(00)00167-2
- Reichmann D, Phillip Y, Carmi A, Schreiber G. On the contribution of water-mediated interactions to protein-complex stability. Biochemistry 2008; 47:1051-60; PMID:18161993; http://dx.doi.org/10.1021/bi7019639
- Cavallo L, Kleinjung J, Fraternali F. POPS: A fast algorithm for solvent accessible surface areas at atomic and residue level. Nucleic Acids Res. 2003; 31:3364-6; PMID:12824328; http://dx.doi.org/10.1093/nar/gkg601
- Lawrence MC, Colman PM. Shape complementarity at protein/protein interfaces. J Mol Biol 1993; 234:946-50; PMID:8263940; http://dx.doi.org/10.1006/jmbi.1993.1648
- Jones S, Thornton JM. Principles of protein-protein interactions. Proc Natl Acad Sci USA 1996; 93:13-20; PMID:8552589; http://dx.doi.org/10.1073/pnas.93.1.13
- Janin J, Wodak SJ. Protein modules and protein-protein interaction. Introduction. Adv Protein Chem 2002; 61:1-8; PMID:12461819; http://dx.doi.org/10.1016/S0065-3233(02)61000-9
- Wodak SJ, Janin J. Structural basis of macromolecular recognition. Adv Protein Chem 2002; 61:9-73; PMID:12461820; http://dx.doi.org/10.1016/S0065-3233(02)61001-0
- Orengo CA, Bray JE, Hubbard T, LoConte L, Sillitoe I. Analysis and assessment of ab initio three-dimensional prediction, secondary structure, and contacts prediction. Proteins 1999; Suppl 3:149-70; PMID:10526364; http://dx.doi.org/10.1002/(SICI)1097-0134(1999)37:3+%3c149::AID-PROT20%3e3.0.CO;2-H
- Sundberg EJ, Andersen PS, Schlievert PM, Karjalainen K, Mariuzza RA. Structural, energetic, and functional analysis of a protein-protein interface at distinct stages of affinity maturation. Structure. 2003; 11:1151-61; PMID:12962633; http://dx.doi.org/10.1016/S0969-2126(03)00187-4
- Chen Y, Wiesmann C, Fuh G, Li B, Christinger HW, McKay P, de Vos AM, Lowman HB. Selection and analysis of an optimized anti-VEGF antibody: crystal structure of an affinity-matured Fab in complex with antigen. J Mol Biol 1999; 293:865-81; PMID:10543973; http://dx.doi.org/10.1006/jmbi.1999.3192
- Garcia-Rodriguez C, Levy R, Arndt JW, Forsyth CM, Razai A, Lou J, Geren I, Stevens RC, Marks JD. Molecular evolution of antibody cross-reactivity for two subtypes of type A botulinum neurotoxin. Nat Biotechnol 2007; 25:107-16; PMID:17173035; http://dx.doi.org/10.1038/nbt1269
- Li Y, Li H, Yang F, Smith-Gill SJ, Mariuzza RA. X-ray snapshots of the maturation of an antibody response to a protein antigen. Nat Struct Biol 2003; 10:482-8; PMID:12740607; http://dx.doi.org/10.1038/nsb930
- Blech M, Seeliger D, Kistler B, Bauer MM, Hafner M, Horer S, Zeeb M, Nar H, Park JE. Molecular structure of human GM-CSF in complex with a disease-associated anti-human GM-CSF autoantibody and its potential biological implications. Biochem J 2012; 447:205-15; PMID:22839360; http://dx.doi.org/10.1042/BJ20120884
- Hercus TR, Cambareri B, Dottore M, Woodcock J, Bagley CJ, Vadas MA, Shannon MF, Lopez AF. Identification of residues in the first and fourth helices of human granulocyte-macrophage colony-stimulating factor involved in biologic activity and in binding to the alpha- and beta-chains of its receptor. Blood 1994; 83:3500-8; PMID:8204877
- Eberhardt MO, Frank R, Kratje R, Etcheverrigaray M. Identification of two potential receptor-binding sites for hGM-CSF. Brazilian Journal of Chemical Engineering 2003; 20:15-20; http://dx.doi.org/10.1590/S0104-66322003000100004
- Behrens F, Tak PP, Ostergaard M, Stoilov R, Wiland P, Huizinga TW, Berenfus VY, Vladeva S, Rech J, Rubbert-Roth A, et al. MOR103, a human monoclonal antibody to granulocyte-macrophage colony-stimulating factor, in the treatment of patients with moderate rheumatoid arthritis: results of a phase Ib/IIa randomised, double-blind, placebo-controlled, dose-escalation trial. Ann Rheum Dis 2015; 74:1058-64.
- Waterhouse AM, Procter JB, Martin DM, Clamp M, Barton GJ. Jalview Version 2–a multiple sequence alignment editor and analysis workbench. Bioinformatics. 2009; 25:1189-91; PMID:19151095; http://dx.doi.org/10.1093/bioinformatics/btp033
- Crooks GE, Hon G, Chandonia JM, Brenner SE. WebLogo: a sequence logo generator. Genome Res. 2004; 14:1188-90; PMID:15173120; http://dx.doi.org/10.1101/gr.849004
- Kleywegt GJ. Dictionaries for heteros. CCP4/ESF-EACBM. Newsletter on Protein Crystallography 1995; 31:45-55
- Laskowski RA, Rullmannn JA, MacArthur MW, Kaptein R, Thornton JM. AQUA and PROCHECK-NMR: programs for checking the quality of protein structures solved by NMR. J Biomol NMR 1996; 8:477-86; PMID:9008363; http://dx.doi.org/10.1007/BF00228148