Abstract
The pH-dependent antigen binding antibody, termed a recycling antibody, has recently been reported as an attractive type of second-generation engineered therapeutic antibody. A recycling antibody can dissociate antigen in the acidic endosome, and thus bind to its antigen multiple times. As a consequence, a recycling antibody can neutralize large amounts of antigen in plasma. Because this approach relies on histidine residues to achieve pH-dependent antigen binding, which could limit the epitopes that can be targeted and affect the rate of antigen dissociation in the endosome, we explored an alternative approach for generating recycling antibodies. Since calcium ion concentration is known to be lower in endosome than in plasma, we hypothesized that an antibody with antigen-binding properties that are calcium-dependent could be used as recycling antibody. Here, we report a novel anti-interleukin-6 receptor (IL-6R) antibody, identified from a phage library that binds to IL-6R only in the presence of a calcium ion. Thermal dynamics and a crystal structure study revealed that the calcium ion binds to the heavy chain CDR3 region (HCDR3), which changes and possibly stabilizes the structure of HCDR3 to make it bind to antigen calcium dependently (PDB 5AZE). In vitro and in vivo studies confirmed that this calcium-dependent antigen-binding antibody can dissociate its antigen in the endosome and accelerate antigen clearance from plasma, making it a novel approach for generating recycling antibody.
Abbreviations
Tm | = | denaturing temperature |
SPR | = | surface plasmon resonance |
CDR | = | complementarity-determining region |
HCDR3 | = | complementarity-determining region 3 in heavy chain |
DSC | = | differential scanning calorimetry. |
Introduction
Therapeutic monoclonal antibodies are now an important option for treating various diseases.Citation1 Engineering technologies that improve the half-life by reducing the isoelectric point of an antibodyCitation2 or that increase neonatal Fc receptor (FcRn) bindingCitation3 and improve binding affinity to the antigen by affinity maturationCitation4 were reported to enhance the potential of therapeutic antibodies. However, these technologies are still limited by the fact that a single antibody molecule can bind to the antigen only once, which limits the amount of antigen that can be neutralized by a certain antibody dosage. Consequently, a huge dose of therapeutic antibody would be required to neutralize an antigen that is continuously produced in large amounts in patients.
As we previously reported, a novel antigen-binding modality termed the recycling antibody can overcome this limitation.Citation5,6 A recycling antibody is a pH-dependent antigen-binding antibody that can bind to antigen at neutral pH and dissociate the antigen at acidic pH. In vivo, a pH-dependent antibody binds to the antigen in plasma, and after endocytosis of the antigen–antibody complex, the antibody dissociates from the antigen in the acidic endosome. This enables the antibody that is now free of antigen to be recycled back to plasma by FcRn, while the dissociated antigen is degraded in the lysosome. When a recycling antibody function was applied to IL-6R, clearance mediated by membrane-bound IL-6R was reduced, and clearance of soluble IL-6R was accelerated by dissociating soluble IL-6R within the endosome.Citation5 Other groups also reported successfully applying the recycling antibody approach to other antigens, such as PCSK9 and IL-6.Citation7,8 Recently, to further enhance the therapeutic potency of recycling antibody, we engineered the Fc domain of a recycling antibody to bind to FcRn at neutral pH. This new modality, termed sweeping antibody, further accelerated the soluble antigen clearance compared to recycling antibody.Citation9
A pH-dependent binding antibody takes advantage of pH differences between plasma (pH 7.4) and endosome (pH 5.8) to dissociate the antigen in the endosome. The pH-dependent binding antibodies reported so far have been generated through histidine mutagenesis onto the CDRs of a lead antibody,Citation5,7,Citation8 Because the pKa of the histidine side chain is around 6, histidine residues are charged differently in plasma and in endosome. When histidine residues are introduced in the interface between antigen and antibody, the antibody will have different affinities in plasma and endosome.Citation5,7,Citation8 Although no crystal structures of a pH-dependent binding antibody with its antigen have been reported, histidine protonation in the acidic endosome is assumed to cause an electrostatic repulsion between the histidine paratope of the antibody and the positively charged or proton donor residue(s) in the epitope of the antigen. This feature of histidine-mediated pH-dependent antigen binding means that a negatively charged or proton-acceptor epitope would be a difficult target for a pH-dependent binding antibody.
To overcome this potential limitation of histidine-mediated recycling antibodies, we explored an alternative approach to engineering a recycling antibody. Whereas a pH-dependent antibody utilizes the pH difference — that is, the difference in proton concentration — between plasma and endosome, calcium ion concentration is another factor reported to be different between plasma and endosome. Calcium ion concentration in plasma is 1.2–2 mM and that in the endosome is 3–30 µM; therefore, we hypothesized that a calcium-dependent antibody that dissociates the antigen at low calcium ion concentration may be used as a recycling antibody.Citation10,11 Moreover, since the difference in calcium ion concentration is even greater than the difference in proton concentration, calcium-dependent antibody may achieve more strongly dependent antigen binding than a pH-dependent antibody.Citation10,11
In this report, we first investigated whether an antibody with calcium-dependent antigen binding could be intentionally isolated from an antibody library by phage display. We successfully identified a calcium-dependent antibody that binds to human soluble IL-6 receptor (hsIL-6R) and solved its crystal structure in the presence of a calcium ion to reveal the mechanism of calcium-dependent antigen binding. Second, we confirmed that this antibody dissociated the antigen in the endosome in vitro and accelerated the antigen clearance in vivo over that of a conventional antibody. We demonstrate that a calcium-dependent antibody can be used for endosomal antigen dissociation and, therefore, can be used as an alternative approach for generating a recycling antibody.
Results
Identification of a calcium-dependent antigen-binding antibody from a human antibody library by phage display
In order to identify an antibody that binds to hsIL-6R in a calcium-dependent manner, we screened antibodies from a human antibody library by phage display. Phage-displayed Fab were selected for their ability to bind to hsIL-6R in the presence of a calcium ion and to elute in the absence of a calcium ion (). After several rounds of panning, we tested 1,920 phage clones for calcium-dependent hsIL-6R binding by ELISA using Fab-displaying phages (data not shown), and identified 6 independent clones with different heavy chain CDR3 (HCDR3) sequences that had a calcium-dependent hsIL-6R binding property. From them, we selected 2 clones, 6RL#9-Fab and 6RK#12-Fab, and converted them into human IgG1 antibodies for further characterization.
Figure 1. Scheme of identification of calcium-dependent antigen-binding antibody. A calcium-dependent antigen-binding antibody was obtained from a human naïve antibody library as follows: Fab-expressing phages were bound to the antigen in the presence of a calcium ion and dissociated in the absence of a calcium ion. After several rounds of panning, several phages were cloned. Phage clones with a calcium-dependent antigen-binding property were converted to human IgG1 format.
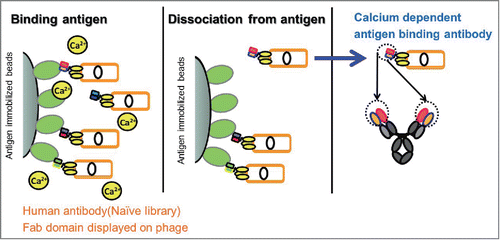
Calcium-dependent hsIL-6R binding kinetics of 6RL#9
To evaluate the antigen-binding properties of these 2 antibodies in the presence of a calcium ion, we tested the antigen binding by surface plasmon resonance (SPR) using purified IgG1. The calcium concentration was set as 2 mM (plasma) or 3 µM (endosome) for SPR analysis to evaluate whether these antibodies bind to the antigen in plasma and dissociate the antigen in the endosome. 6RL#9-hIgG1 and 6RK#12-hIgG1 showed antigen binding in a calcium-dependent manner ().
Figure 2. Calcium-dependent antigen-binding profile of 6RL#9 and 6RK#12. Calcium-dependent antigen binding of 6RL#9 (A) and 6RK#12 (B) was evaluated by SPR analysis.
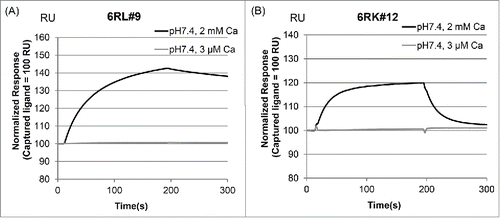
Next, a detailed kinetics analysis was conducted on 6RL#9-hIgG1. compares the hsIL-6R binding affinity of 6RL#9 with that of the conventional anti-hsIL-6R antibody NPH-hIgG1,Citation5,9 which has no calcium-dependent antigen-binding activity. The antigen binding of 6RL#9-hIgG1 at the 3 µM concentration of calcium ions was significantly weaker than that at the 2 mM concentration, while with NPH-hIgG1 the calcium ion concentration had no effect on binding affinity. (Fig. S1)
Table 1. Equilibrium dissociation constants (KD) of the interactions of anti-hsIL-6R antibodies with hsIL-6R at 2 mM CaCl2 and 3 μM CaCl2
Direct binding of the calcium ion to 6RL#9-hIgG1 in its Fab region
To elucidate the mechanism of calcium-dependent hsIL-6R binding to 6RL#9-hIgG1, we tested whether the calcium ion directly binds to 6RL#9-hIgG1 or hsIL-6R by using the differential scanning calorimetry method.Citation12 The thermal denaturation curves are shown in . When the denaturing temperature (Tm) of the Fab domain of 6RL#9-hIgG1 in the absence and presence of a calcium ion was compared with that of NPH-hIgG1, the Tm of the Fab of NPH-hIgG1 was not significantly changed, but the presence of a calcium ion had a stabilizing effect on the Fab of 6RL#9-hIgG1 (). These results demonstrate that the Fab of a calcium ion binds to 6RL#9-hIgG1 but not to hsIL-6R, a difference that presumably contributes to the calcium-dependent hsIL-6R binding of 6RL#9-hIgG1.
Figure 3. Effect of a calcium ion on the thermal stability of 6RL#9 and its antigen hsIL-6R. The thermal stability of hsIL-6R (A) and anti-hsIL-6R antibodies (B: NPH-hIgG1, C: 6RL#9-hIgG1) was determined by differential scanning calorimetry at 2 mM or 3 µM of a calcium ion. Dotted line represents 2 mM CaCl2 condition and solid line shows 3 µM CaCl2 condition.
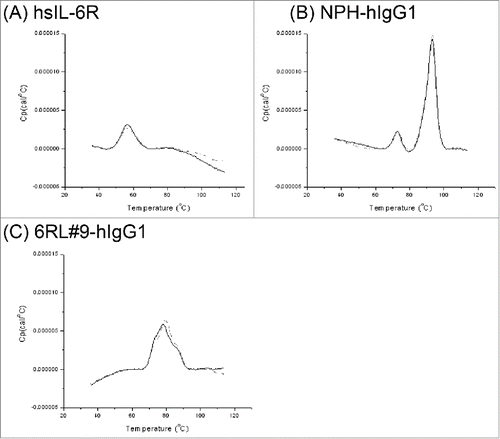
Table 2. Denaturing temperature (Tm) of hsIL-6R and Fab domain of NPH-hIgG1 and 6RL#9-hIgG1 in the presence and absence of a calcium ion
Binding of a calcium ion to HCDR3 of 6RL#9 Fab revealed by X-ray crystal structure analysis
Because the thermal denaturation study suggested that the calcium ion binds to 6RL#9-hIgG1 in its Fab domain, we obtained crystal structures of 6RL#9-Fab with a calcium ion to reveal the specific calcium-binding site and its binding manner (). In this crystal structure, the electron density of CDR1 of the light chain was not clearly observed. As shown in , a large electron density blob that corresponds to a calcium ion was clearly observed, encircled by the HCDR3 loop. The calcium ion was coordinated by the side-chain carboxy oxygen atom of Asp99, and the main-chain carbonyl oxygen atoms of Asp100 and Glu105 (Kabat numbering) in HCDR3 ().
Figure 4. Calcium ion binding into 6RL#9-Fab from X-ray crystal structure. (A) Overall structure of Fv region of 6RL#9-Fab domain in the presence of calcium ion. (B) 2Fo-Fc electron density map of 6RL#9-Fab crystal in calcium ion including condition around HCDR3 is contoured at 1 times rmsd. The structure of 6RL#9-Fab is superimposed. (C) The detailed view of binding between calcium ion and HCDR3 of 6RL#9-Fab. Heavy chain is shown in green. Light chain is shown in magenta. Heavy chain CDR3 residues are indicated with sticks. Calcium ion is shown with a sphere and in yellow. Hydrogen bonds and a salt bridge with calcium ion are shown as dotted lines.
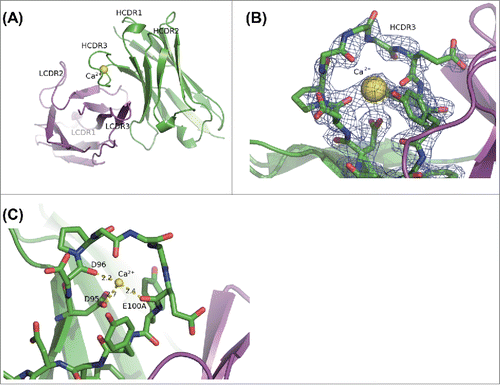
Confirmation of endosomal antigen dissociation by 6RL#9 Fab using confocal microscopy analysis
To test antigen dissociation in the endosome, we performed confocal microscopy analysis by using human FcRn-transfected Madin-Darby canine kidney (MDCK) epithelial cells. MDCK cells were co-transfected with eGFP-tagged human FcRn and human β2 microglobulin. FcRn-transfected cells were treated with a pre-incubated same amount of hsIL-6R and anti-hsIL-6R antibodies or hsIL-6R alone. Treatment of labeled hsIL-6R alone showed slight uptake of hsIL-6R to FcRn-transfected cells (). In this study, the Fc region of anti-hsIL-6R antibodies was mutated (M252Y/N434Y/Y436V; EU numbering) to enhance FcRn binding at neutral pH to increase the sensitivity. The affinity of engineered Fc region to human FcRn was a 75-fold increase compared with hIgG1 at pH7.0 condition (Table S1). When labeled hsIL-6R was incubated with NPH-mutated IgG1, which does not have calcium dependency, hsIL-6R was co-localized with FcRn at the rim of the endosome, indicating that hsIL-6R is not dissociated from an antibody that is bound to FcRn at acidic pH (). On the other hand, in the case of the calcium-dependent binding antibody, labeled hsIL-6R was surrounded by FcRn, indicating that hsIL-6R was dissociated from the antibody (). This data confirmed that a calcium-dependent antigen-binding antibody can dissociate an antigen within the endosome.
Figure 5. Evaluation of endosomal antigen dissociation by confocal microscopy analysis. The calcium-dependent antigen-binding properties of an antibody affects the intracellular location of the antigen. FcRn expressing MDCK cells were pulsed with (A) labeled hsIL-6R alone or labeled hsIL-6R at a same amount of (B) a conventional antibody without calcium dependency (NPH) or (C) an antibody with calcium-dependent antigen binding (6RL#9). In (A), (B) and (C), upper and lower panels show 200 fold and 400 fold magnification, respectively. Cell images are shown with FcRn, hsIL-6R and nuclear in green, red and blue, respectively.
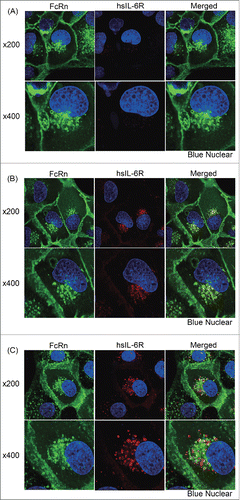
Acceleration of antigen clearance by 6RL#9 in vivo
Recycling antibody with a pH-dependent binding property was previously reported to accelerate the soluble antigen clearance in vivo compared to conventional antibody.Citation5,7,Citation8 To investigate whether a calcium-dependent antigen-binding antibody can be used as an alternative approach to generating recycling antibody, we tested whether 6RL#9-hIgG1 accelerates the clearance of hsIL-6R in vivo. While hsIL-6R co-injected with NPH-hIgG1 was cleared from plasma more slowly than hsIL-6R alone, hsIL-6R co-injected with 6RL#9-hIgG1 was cleared from plasma more rapidly than the conventional antibody, at a rate comparable to that of hsIL-6R alone (). This demonstrates that a calcium-dependent antigen-binding antibody can dissociate the antigen in the endosome in vivo and accelerate the clearance of antigen from plasma.Citation5
Figure 6. Plasma concentration–time profile of hsIL-6R injected alone or in complex with anti-hsIL-6R antibodies. C57BL/6 mice were injected with either hsIL-6R alone or in complex with a conventional antibody without calcium dependency (NPH-hIgG1) or with a calcium-dependent antigen-binding antibody (6RL#9-hIgG1). Plasma concentration of hsIL-6R at the indicated time points was determined by ELISA, and mean values for each mouse group are presented. Error bar represents standard deviation.
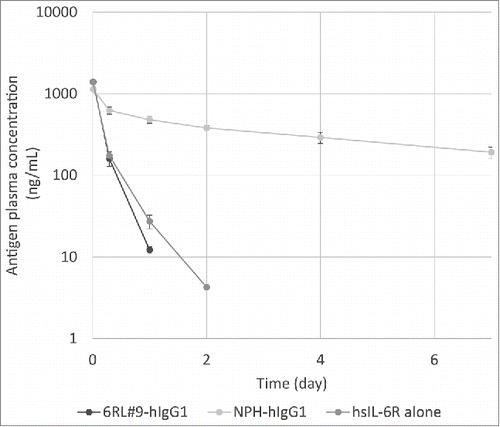
Discussion
Since an anti-IL-6R antibody was used to demonstrate that pH-dependent antigen-binding antibody is an effective approach for overcoming the limitations of conventional antibody,Citation5 several groups have reported that this approach can be applied to different antigens, such as PCSK9 and IL-6.Citation7,8 The advantage of a pH-dependent antigen-binding antibody lies in the difference between the plasma and endosome environments. In this work, because one of the environmental differences between plasma and endosome is the calcium ion concentration, we utilized it as a factor for generating a recycling antibody that dissociates an antigen in the endosome.
We successfully obtained a calcium-dependent antigen-binding antibody from a human antibody library by screening in the absence and presence of calcium ions. Calcium-dependent antibodies are already known — examples are anti-DYKDDDDK antibody clone M1 (also referred to as anti-Flag tag antibody),Citation13 anti-protein C antibody,Citation14 and anti-thrombomodulin antibodyCitation15 — and are obtained from immunized animals. However, these antibodies recognize a structural change in the antigen's epitope that is induced by the calcium ion binding directly to the antigen, not to the antibody. In other words, a calcium-bound form of the antigen is required for these calcium-dependent antigen-binding antibodies. Since most of the therapeutically relevant target antigens do not bind to a calcium ion, this approach is not generally applicable.
To the best of our knowledge, this is the first report to intentionally identify a calcium-dependent antigen-binding antibody against an antigen that does not directly bind to calcium. In contrast to the calcium-dependent antigen-binding antibodies reported previously, 6RL#9-Fab binds directly to the calcium ion. In vitro selection in the presence and absence of calcium using the phage display of an antibody library enabled us to identify such an antibody. Since antibodies obtained by animal immunization are biased toward epitopes that are immunogenic to the host and stronger binding affinity to the antigen (because of the way that the host develops antibody), it would be difficult to obtain calcium-dependent binding antibodies against any antigens efficiently by this method. We believe that in vitro selection using phage display is a powerful approach for identifying calcium-dependent binding antibodies.
Structural analysis revealed that a calcium ion binds 6RL#9-Fab within the HCDR3 region. This coordination of the calcium ion by the HCDR3 loop is a very unique structure and not previously reported. The calcium ion makes 3 coordination linkages with the main chain and side chain atoms of the residues in the HCDR3 loop. As a result of these linkages, the calcium ion may contribute to changing and stabilizing the structure of the HCDR3 loop, which in the original structure has 3 Gly residues (Gly98, 99, and 100) and appears to be flexible. Since the HCDR3 region is known to be an important loop for antigen binding, the structural change and stabilization of the loop induced by calcium-ion binding would be a key feature of the calcium-dependent antigen binding of 6RL#9. This structural feature of 6RL#9 suggests that there is a high probability of eliciting a calcium-dependent antigen-binding property from an antibody with calcium-binding capability. Although we were able to identify calcium-dependent antigen-binding antibodies from a human antibody library, the acquisition rate was very low. This is probably because the calcium-binding motif exists very rarely in the human antibody repertoire. Therefore, if the calcium-binding motif could be incorporated into the antibody library as a scaffold, the resulting rationally designed synthetic library would be an efficient tool for obtaining calcium-dependent antigen-binding antibodies.
In this study, we used both an in vitro confocal microscopic analysis and an in vivo pharmacokinetics study to show that a calcium-dependent antigen-binding antibody can dissociate an antigen within the endosome. In the confocal microscopic analysis, we observed that labeled antigen was located within the FcRn-expressing vacuole. Recently it was reported that a pH-dependent antibody against IL-6 or IL-6R showed dissociation of the antigen in the endosome when marked by eGFP-tagged FcRn.Citation6,8 As in the case of the pH-dependent binding antibody, the calcium-dependent binding antibody we found in this paper also dissociated an antigen within the endosome. For this, the binding constant of the calcium ion must be controlled at the appropriate value between the calcium ion concentrations in plasma and endosome. EF-hand, which is well known as a calcium-binding motif, coordinates a calcium ion in the pentagonal dipyramid fashion,Citation16 and thus can achieve a binding constant in the order of nM.Citation16 On the other hand, 6RL#9 Fab makes only 3 coordination linkages with the calcium ion. This imperfect coordination would be important to keep the binding constant of calcium ions moderate, and to achieve recycling of the antibody from the endosome. Furthermore, the calcium-dependent antibody accelerated the antigen clearance from plasma in vivo, and the pharmacokinetics of the antigen injected together with the calcium-dependent binding antibody was comparable to that of the antigen when injected alone. This also supports the view that a calcium-dependent binding antibody can dissociate the antigen in vivo in the same way as a pH-dependent binding antibody.Citation5,7,Citation8 These results confirm that calcium-dependent antigen-binding antibodies can be used as an alternative approach for generating recycling antibodies.
We and other groups have successfully generated a pH-dependent antigen-binding antibody as a recycling antibody by engineering histidine into a conventional antibody.Citation5,7,Citation8 Our experience implies that, although this approach was effective for all the antibodies we have studied so far, it is sometimes difficult to create a pH-dependent antibody that has strong binding affinity at neutral pH and a rapid dissociation rate at acidic pH. We assume that this is because there is only a 50-fold difference in proton concentration between plasma and endosome, and the antigen–antibody interaction in acidic pH is destabilized by the small difference in neutral histidine and protonated histidine. On the other hand, we assume that a calcium-dependent antigen-binding antibody has advantages over its pH-dependent counterpart in 3 different ways. First, the difference in calcium ion concentration between plasma and endosome is reported to be 650-fold,Citation10,11 which is noticeably larger than the difference in proton concentration. Second, although an antibody with a calcium ion, which is a divalent cation, has positive electrostatic potential in plasma, in an endosome the antibody releases the calcium ion and has a negative—or at least neutral—electrostatic potential, and this much larger difference in electrostatic potential would destabilize the antigen–antibody interaction in endosomes much more than the difference caused by histidine protonation. In addition, the calcium ion can make a complex chelating pattern with the carboxy oxygen atoms of the Asp and Glu side chains or with the carbonyl oxygen atoms of the main chain and Asn and Gln side chains, or with water, etc. Especially, calcium ion can attract carboxy and carbonyl oxygen atoms, which are negatively charged and usually repel each other, and thus can trigger a large conformational change. Therefore, a calcium ion can be used as a switch to activate or inactivate large conformational changes in several natural proteins, such as calmodulin, LDL receptor, and asialoglycoprotein receptor.Citation17–19 Third, since a calcium ion has a larger ionic radius than a proton, it could have an additional steric effect on destabilizing the antigen–antibody interaction in endosome. These features of a calcium-dependent antigen-binding antibody would provide a more efficient and effective approach for generating a recycling antibody.
In conclusion, we report here the use of a phage display of human antibody library to identify calcium-dependent hsIL-6R binding antibodies that bind to the antigen only in the presence of calcium. We confirmed that the calcium binding to HCDR3 contributes to the calcium-dependent antigen-binding property. In vitro and in vivo studies confirmed that a calcium-dependent antigen-binding antibody could dissociate the antigen in the endosome and accelerate antigen clearance from plasma; thus, we demonstrated that a calcium-dependent antigen-binding antibody can be a novel modality for generating a recycling antibody. Moreover, the nature of a calcium-dependent binding antibody could make it a more efficient and effective approach for generating recycling antibodies.
Material and Methods
Preparation of recombinant antibodies
Recombinant antibodies used in these experiments were expressed transiently in FreeStyle™ 293F cells (Invitrogen) transfected with plasmids encoding immunoglobulin heavy chain and light chain and purified from cultured medium using protein A. Site-directed mutagenesis and sub-cloning into mammalian expression vector was performed using an In-Fusion HD Cloning Kit (Clontech).
Antibody screening by phage display
The Fab phage display library used for screening for a calcium-dependent antigen-binding antibody was constructed from human polyA RNA by the general method.Citation20 The phage display library was diluted with TBS (TaKaRa) supplemented with bovine serum albumin (BSA, 4% of final solution) and CaCl2 (1.2 mM in final solution). Next, the library was incubated with biotinylated hsIL-6R (250 pmol) for 60 minutes, and then streptavidin-coated beads were added. After incubation with beads for 15 minutes at room temperature, the beads were washed once with TBS containing 1.2 mM CaCl2. Phages were eluted by re-suspending the beads with TBS containing 2 mM EDTA. The eluted phages were infected with TG1 and rescued by the helper phage. These rescued phages were precipitated with polyethylene glycol, resuspended with TBS supplemented with BSA (4% of final solution) and CaCl2 (1.2 mM in final solution), and used in the next round of panning. In the second and third rounds, the steps in the first round of panning were repeated, using 40 pmol of biotinylated antigen. Biotinylated antigen was prepared as previously described.Citation21
Binding and affinity analysis by SPR
The interaction of antibodies with antigen was monitored using Biacore instruments (GE Healthcare), as previously described.Citation5,9 Antibody was captured on protein A immobilized on a CM4 sensor chip (GE Healthcare), followed by injection of hsIL-6R. During the analysis, 10 mM ACES, 150 mM NaCl, and 2 mM CaCl2 (pH 7.4) with 0.05% Surfactant P20 was used as a running buffer for the high calcium condition, and 10 mM ACES, 150 mM NaCl, and 3 µM CaCl2 (pH 7.4) with 0.05% Surfactant P20 was used for the low calcium condition. Kinetic analysis was performed by global fitting of binding data with a 1:1 Langmuir binding model or a steady-state binding model using Biacore Evaluation Software (GE Healthcare).
Differential scanning calorimetry
All proteins were dialyzed against TBS (TaKaRa) and then diluted to 0.1 mg/ml with or without 2 mM of CaCl2. Forty-two micrograms of protein were loaded into a MicroCal VP-Capillary DSC (MicroCal) and measured at a scanning rate of 4°C/min from 20°C to 115°C. Obtained data were analyzed using Origin 7 software (OriginLab Corporation) and subtracted from the data of the buffer condition.
Crystallization, data collection, and structure determination of calcium-dependent antigenbinding antibodies with a calcium ion
Fab fragments of 6RL#9 were prepared using limited digestion with papain (Roche Diagnostics), cation exchange, and size exclusion chromatography. Crystals of the complex with a calcium ion were obtained by 1:1 mixing of about 12 mg/ml 6RL#9 Fab fragments in 150 mM HEPES (pH 7.8), 50 mM NaCl, and 5 mM CaCl2 with 100 mM HEPES (pH 7.5) with 20%–29% PEG4000 in hanging drop vapor diffusion setups with microseeding at 20°C. For data collection, crystals were flash frozen at 95 K in a cryo solution containing 5 mM CaCl2, 100 mM HEPES (pH 7.5), and 35% PEG4000. Diffraction data to 2.2 Å for the complex with calcium were collected using the Photon Factory beamline BL-17A. Data were processed with Xia2,Citation22 XDS Package,Citation23 and Scala.Citation24 The crystal belonged to the space group P212121, and the asymmetric units contained one 6RL#9 Fab fragment. The structure was determined by molecular replacement with PHASERCitation25 using the VH, CL, and CH1 regions of 1ZA6 and the VL region of 2A9M as search models. A model was built with the program CootCitation26 and refined with the program REFMAC5.Citation27 Data collection and refinement statistics are summarized in . The structure has been deposited in the RCSB Protein Data Bank with PDB code 5AZE. The sequence of Fab domain of 6RL#9 is shown in the supplementary Figure 2. All graphical presentations were prepared with PyMOL.Citation28
Table 3. Data collection and refinement statistics
Establishment of human FcRn transfectant
EGFP-tagged human FcRn (FcRn-EGFP) plasmid was constructed in the pCXND3 vector.Citation29 FcRn-eGFP cDNA was synthesized by directly fusing stop-codon-deleted α-FcRn cDNA to initiating-codon-deleted EGFP cDNA without any linker sequences between the 2 genes. The human β-2 microglobulin cDNA was synthesized and subcloned into the pCXZD1 vector, which is a zeocin-resistant variant of the pCXND3 vector. Each of the plasmids was linearized for transfection at a unique Pvu I site.
MDCK cells (ATCC) were cultured in Minimum Essential Medium Eagle (Sigma-Aldrich) supplemented with 10% fetal bovine serum, 2 mM GlutaMax (Invitrogen), and penicillin/streptomycin (Invitrogen) at 37°C, 5% CO2. To obtain transfectants of MDCK cells that express FcRn-EGFP and β-2 microglobulin, 10 µg of each expression vector was co-transfected by the electroporation method using GenePulser Xcell (Bio-Rad) under conditions of 0.3 kV and 950 µFD. Cells were selected in the presence of 500 µg/ml geneticin (Invitrogen) and 300 μg/ml zeocin (Invitrogen), which select for transfection with the FcRn-eGFP and β-2 microglobulin. Resistant clones were analyzed for expression by immunofluorescence, and clone #B112 was selected for subsequent experiments.
In vitro live-cell imaging study of antigen dissociation
hsIL-6R was prepared as described previously.Citation5,9 hsIL-6R was labeled with Alexa Fluor 555 (Alexa 555) reactive dye (Molecular Probes) according to the methods recommended by the manufacturer. Unincorporated fluorophore was removed by dialysis using EasySep (TOMY).
Antibody–antigen complex was generated by mixing 2.5 µg of antibody and Alexa 555–labeled hsIL-6R in 200 µL of culture medium for 15 min at 37°C. For fluorescence experiments, transfectants were grown on a Poly-D-Lysine Cellware 35-mm Coverslip Bottom Dish (BD Biosciences) without geneticin or zeocin for one day before experiments. To analyze the distribution of Alexa 555–labeled hsIL-6R in cells, cells were incubated in 80 µL of medium containing immune complex for 15 min at 37°C, 5% CO2. After the pulse period, cells were washed with medium, and nuclei were stained with 5 µg/mL of Hoechst33342 (Invitrogen).
A Nikon TE2000 microscope was used for imaging with a 100X 1.40 NA Nikon objective lens. For excitation, a laser excitation system was used consisting of 3 lasers: 405 nm, 488 nm, and 561 nm (Melles Griot, CA). The 405-, 488-, and 561-nm laser lines were used to make an image of nuclear, eGFP, and Alexa 555–labeled hsIL-6R, respectively.
In vivo antigen–antibody co-injection study
All animal experiments in this study were performed in accordance with the Guidelines for the Care and Use of Laboratory Animals at Chugai Pharmaceutical Co., Ltd. In the co-injection model, C57BL/6J mice (Charles River) were given a single intravenous injection of hsIL-6R alone or hsIL-6R pre-mixed with antibody. The first group received 50 µg/kg of hsIL-6R, but the other group additionally received 1 mg/kg of NPH-hIgG1 (which has no calcium-dependent antigen-binding activity) or 100 mg/kg of 6RL#9-hIgG1a, to achieve the same amount of hsIL-6R–antibody complex. The total hsIL-6R concentration in plasma was determined as previously described.Citation5,9
Disclosure of Potential Conflicts of Interest
This work was fully supported by Chugai Pharmaceutical Co., Ltd. Funding to pay the Open Access publication charges for this article was provided by Chugai Pharmaceutical Co., Ltd.
Supplemental Data
Download Zip (213 KB)Acknowledgments
We thank our colleagues at Chugai Research Institute for Medical Science Inc. and Chugai Pharmaceutical Co., Ltd: M. Fujii, A. Ohba, Y. Nakata, A. Maeno, and S. Masujima for antibody generation; T. Sakiyama and M. Wada for preparation of the antibody.
Supplementary Material
Supplemental data for this article can be accessed on the publisher's website.
References
- Reichert JM, Rosensweig CJ, Faden LB, Dewitz MC. Monoclonal antibody successes in the clinic. Nat Biotechnol 2005; 23:1073-8; PMID:16151394; http://dx.doi.org/10.1038/nbt0905-1073
- Igawa T, Tsunoda H, Tachibana T, Maeda A, Mimoto F, Moriyama C, Nanami M, Sekimori Y, Nabuchi Y, Aso Y, et al. Reduced elimination of IgG antibodies by engineering the variable region. Protein Eng Des Sel 2010; 23:385-92; http://dx.doi.org/10.1093/protein/gzq009
- Kuo TT, Aveson VG. Neonatal Fc receptor and IgG-based therapeutics. mAbs 2011; 3:422-30; PMID:22048693; http://dx.doi.org/10.4161/mabs.3.5.16983
- Rajpal A, Beyaz N, Haber L, Cappuccilli G, Yee H, Bhatt RR, Takeuchi T, Lerner RA, Crea R. A general method for greatly improving the affinity of antibodies by using combinatorial libraries. Proc Natl Acad Sci U S A 2005; 102:8466-71; PMID:15939870; http://dx.doi.org/10.1073/pnas.0503543102
- Igawa T, Ishii S, Tachibana T, Maeda A, Higuchi Y, Shimaoka S, Moriyama C, Watanabe T, Takubo R, Doi Y, et al. Antibody recycling by engineered pH-dependent antigen binding improves the duration of antigen neutralization. Nat Biotechnol 2010; 28:1203-7; PMID:20953198; http://dx.doi.org/10.1038/nbt.1691
- Igawa T, Mimoto F, Hattori K. pH-dependent antigen-binding antibodies as a novel therapeutic modality. Biochim Biophys Acta 2014; 1844:1943-50; PMID:25125373; http://dx.doi.org/10.1016/j.bbapap.2014.08.003
- Chaparro-Riggers J, Liang H, DeVay RM, Bai L, Sutton JE, Chen W, Geng T, Lindquist K, Casas MG, Boustany LM, et al. Increasing serum half-life and extending cholesterol lowering in vivo by engineering antibody with pH-sensitive binding to PCSK9. J Biol Chem 2012; 287:11090-7; PMID:22294692; http://dx.doi.org/10.1074/jbc.M111.319764
- Devanaboyina SC, Lynch SM, Ober R, Sripad R, Kim D, Puig-Canto A, Breen S, Kasturirangan S, Fowler S, Peng L, et al. The effect of pH dependence of antibody-antigen interactions on subcellular trafficking dynamics. mAbs 2013; 5:9; http://dx.doi.org/10.4161/mabs.26389
- Igawa T, Maeda A, Haraya K, Tachibana T, Iwayanagi Y, Mimoto F, Higuchi Y, Ishii S, Tamba S, Hironiwa N, et al. Engineered monoclonal antibody with novel antigen-sweeping activity in vivo. PloS One 2013; 8:e63236; PMID:23667591; http://dx.doi.org/10.1371/journal.pone.0063236
- Gerasimenko JV, Tepikin AV, Petersen OH, Gerasimenko OV. Calcium uptake via endocytosis with rapid release from acidifying endosomes. Curr Biol 1998; 8:1335-8; PMID:9843688; http://dx.doi.org/10.1016/S0960-9822(07)00565-9
- Hurwitz S. Homeostatic control of plasma calcium concentration. Crit Rev Biochem Mol Biol 1996; 31:41-100; PMID:8744955; http://dx.doi.org/10.3109/10409239609110575
- Lin YP, Raman R, Sharma Y, Chang YF. Calcium binds to leptospiral immunoglobulin-like protein, LigB, and modulates fibronectin binding. J Biol Chem 2008; 283:25140-9; PMID:18625711; http://dx.doi.org/10.1074/jbc.M801350200
- Slootstra JW, Puijk WC, Ligtvoet GJ, Langeveld JP, Meloen RH. Structural aspects of antibody-antigen interaction revealed through small random peptide libraries. Mol Divers 1996; 1:87-96; PMID:9237197; http://dx.doi.org/10.1007/BF01721323
- Ohlin AK, Stenflo J. Calcium-dependent interaction between the epidermal growth factor precursor-like region of human protein C and a monoclonal antibody. J Biol Chem 1987; 262:13798-804; PMID:2443497
- Dixit VM, Galvin NJ, O'Rourke KM, Frazier WA. Monoclonal antibodies that recognize calcium-dependent structures of human thrombospondin. Characterization and mapping of their epitopes. J Biol Chem 1986; 261:1962-8; PMID:2418018
- Gifford JL, Walsh MP, Vogel HJ. Structures and metal-ion-binding properties of the Ca2+-binding helix-loop-helix EF-hand motifs. Biochem J 2007; 405:199-221; PMID:17590154; http://dx.doi.org/10.1042/BJ20070255
- Gilli R, Lafitte D, Lopez C, Kilhoffer M, Makarov A, Briand C, Haiech J. Thermodynamic analysis of calcium and magnesium binding to calmodulin. Biochemistry 1998; 37:5450-6; PMID:9548926; http://dx.doi.org/10.1021/bi972083a
- Arias-Moreno X, Cuesta-Lopez S, Millet O, Sancho J, Velazquez-Campoy A. Thermodynamics of protein-cation interaction: Ca(+2) and Mg(+2) binding to the fifth binding module of the LDL receptor. Proteins 2010; 78:950-61; PMID:19899171; http://dx.doi.org/10.1002/prot.22619
- Wragg S, Drickamer K. Identification of amino acid residues that determine pH dependence of ligand binding to the asialoglycoprotein receptor during endocytosis. J Biol Chem 1999; 274:35400-6; PMID:10585409; http://dx.doi.org/10.1074/jbc.274.50.35400
- de Haard HJ. Construction of large naive Fab libraries. Methods Mol Biol 2002; 178:87-100; PMID:11968512
- Predonzani A, Arnoldi F, Lopez-Requena A, Burrone OR. In vivo site-specific biotinylation of proteins within the secretory pathway using a single vector system. BMC Biotechnol 2008; 8:41; PMID:18423015; http://dx.doi.org/10.1186/1472-6750-8-41
- G W. xia2: an expert system for macromolecular crystallography data reduction. J Appl Crystallogr 2010; 43:5.
- Kabsch W. XDS. Acta Crystallogr D Biol Crystallogr 2010; D66:8.
- Evans P. Scaling and assessment of data quality. Acta Crystallogr D Biol Crystallogr 2006; 62:72-82; PMID:16369096; http://dx.doi.org/10.1107/S0907444905036693
- McCoy AJ, Grosse-Kunstleve RW, Adams PD, Winn MD, Storoni LC, Read RJ. Phaser crystallographic software. J Appl Crystallogr 2007; 40:17; http://dx.doi.org/10.1107/S0021889807021206
- Emsley P, Lohkamp B, Scott WG, Cowtan K. Features and development of Coot. Acta Crystallogr D Biol Crystallogr 2010; 66:486-501; PMID:20383002; http://dx.doi.org/10.1107/S0907444910007493
- Murshudov GN, Skubak P, Lebedev AA, Pannu NS, Steiner RA, Nicholls RA, Winn MD, Long F, Vagin AA. REFMAC5 for the refinement of macromolecular crystal structures. Acta Crystallogr D Biol Crystallogr 2011; 67:355-67; PMID:21460454; http://dx.doi.org/10.1107/S0907444911001314
- L DW. The PyMOL Molecular Graphics System. Schrodinger, LLC, Palo Alto, CA 2002.
- Orita T, Tsunoda H, Yabuta N, Nakano K, Yoshino T, Hirata Y, Ohtomo T, Nezu J, Sakumoto H, Ono K, et al. A novel therapeutic approach for thrombocytopenia by minibody agonist of the thrombopoietin receptor. Blood 2005; 105:562-6; PMID:15374889; http://dx.doi.org/10.1182/blood-2004-04-1482