ABSTRACT
Although dengue virus (DENV) infection severely threatens the health of humans, no specific antiviral drugs are currently approved for clinical use against DENV infection. Attachment and fusion are 2 critical steps for the flavivirus infection, and the corresponding functional epitopes are located at E protein domain III (E-DIII) and domain II (E-DII), respectively. Here, we constructed a bispecific antibody (DVD-1A1D-2A10) based on the 2 well-characterized anti-DENV monoclonal antibodies 1A1D-2 (1A1D) and 2A10G6 (2A10). The 1A1D antibody binds E-DIII and can block the virus attaching to the cell surface, while the 2A10 antibody binds E-DII and is able to prevent the virus from fusing with the endosomal membrane. Our data showed that DVD-1A1D-2A10 retained the antigen-binding activity of both parental antibodies. Importantly, it was demonstrated to be significantly more effective at neutralizing DENV than its parental antibodies both in vitro and in vivo, even better than the combination of them. To eliminate the potential antibody-dependent enhancement (ADE) effect, this bispecific antibody was successfully engineered to prevent Fc-γ-R interaction. Overall, we generated a bispecific anti-DENV antibody targeting both attachment and fusion stages, and this bispecific antibody broadly neutralized all 4 serotypes of DENV without risk of ADE, suggesting that it has great potential as a novel antiviral strategy against DENV.
Introduction
Dengue virus (DENV) is a member of the arthropod-borne Flavivirus family, which also includes the Japanese encephalitis, West Nile, yellow fever and tick-borne encephalitis viruses. Clinically, DENV is the agent of dengue fever (DF), dengue hemorrhagic fever (DHF) and dengue shock syndrome (DSS).Citation1,2 According to the World Health Organization, around half of the world population are living in epidemic areas, over 50 million people are infected each year, and ˜500,000 of those people develop severe DHF and DSS.Citation3 Although DENV infection severely threatens the human health, no specific antiviral drugs are currently approved for the treatment of DENV infection. Additionally, since DENV has 4 serotypes, more severe DHF and DSS frequently occur when the secondary infection is of a serotype different from the first. This problem also occurs via antibody-dependent enhancement (ADE) when a neutralizing antibody at a sub-neutralizing concentration or non-neutralizing antibody binds dengue virus.Citation4
The early stages of DENV infection include 2 critical steps: cell binding and envelop fusion, both of which are closely related to the E protein.Citation5 Firstly, virions attach to the surface of a host cell and enter the cell by receptor-mediated endocytosis.Citation6 Domain III of the E protein, emerging as an immunoglobulin-like fold, is essential for receptor binding and has the most potentially neutralizing epitopes. Therefore, some type-specific neutralizing antibodies recognize this domain specifically.Citation7-9 After entering the cell, acidification of the endosomal vesicle triggers conformational changes in the virion. Then, the fusion peptides are exposed, the viral envelop fuses with the endosomal vesicle, and particle disassembly occurs. The peptides have a quite hydrophobic loop (residences 98–110) at the tip of the DII that is highly conserved in all serotypes even in other flavivirus.Citation10-12 Neutralizing antibodies that recognize DII mostly concentrate in this area, and most of them are characterized by cross-reaction. In addition, because of the function of the DII, antibodies that recognize this domain might have potential for therapy.
Antibody-based therapy represents a promising alternative strategy for flavivirus infection, and previous studies reported that several antibodies are effective in animal models, both as prophylactic and therapeutic agents.Citation13-15 So far, however, no antibody drug, either alone or a cocktail of multiple antibodies, is available for the treatment of DENV infection. The anti-DENV mAb 1A1D-2 (1A1D) binds one β-strand of E-D III with residues 305–312, residues 325, 364, 388 and 390 also contacting the antibody. It could protect mice from the infection of DENV1–3, but did not bind to DENV4 and did not have significant therapeutic effect against DENV4.Citation7,16 In our previous study, we reported a broadly cross-neutralizing antibody 2A10G6 (2A10), which could block the fusion step of all 4 serotypes of dengue virus. 2A10 recognized residues D98, R99 and W101, which were located on the fusion loop of E-D II.Citation12
Previous studies have indicated that the combination of variant monoclonal antibodies (mAbs) is much more efficient than single mAbs.Citation17 Our previous studies have shown that a bispecific antibody engineered from 2 distinct monoclonal antibodies against hepatitis B surface antigen (HBsAg) had superior HBV-neutralizing activity compared with the combination of both parental monoclonal antibodies, possibly through steric hindrance or induction of HBsAg conformational changes.Citation18 In this study, we constructed a bispecific antibody using the anti-DENV mAbs 1A1D and 2A10. This bispecific antibody, denoted as DVD-1A1D-2A10, was confirmed to be more effective at neutralizing DENV than its parental mAbs, and even better than the combination of them. Subsequently, we mutated 9 amino acids on the Fc domain of the antibody to eliminate antibody-dependent enhancement (ADE) of infection. Our data suggest that this bispecific antibody has potential as a treatment for DENV infection.
Results
Construction and characterization of DVD-1A1D-2A10
We engineered a dual-variable domain (DVD) antibody using the anti-DENV monoclonal antibodies 1A1D and 2A10. The structure of this bispecific antibody, DVD-1A1D-2A10, was shown in . The purity and the molecular weight of the purified DVD-1A1D-2A10 were determined by SDS-PAGE (). Under reducing conditions, DVD-1A1D-2A10 yielded 2 protein bands with molecular mass of 64kDa (heavy chain) and 36kDa (light chain), both of which corresponded approximately to the calculated molecular mass of the monomeric polypeptides. Under non-reducing conditions, a single band of ∼200 kDa for DVD-1A1D-2A10 was observed, compared to 150 kDa for the normal bivalent antibody.
Figure 1. Structure and characterization of DVD-Ig. (A) Schematic representation of the structure of DVD-1A1D-2A10. (B) SDS-PAGE analysis of purified antibodies under non-reducing and reducing conditions. Lane 1, molecular weight protein markers; lane 2, DVD-1A1D-2A10; lane 3, bivalent antibody.
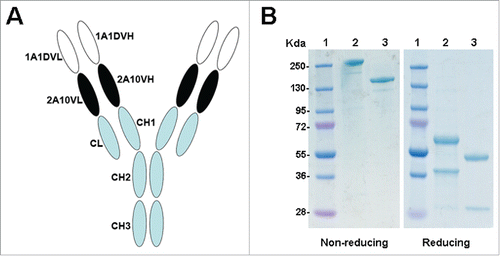
To further determine whether the DVD-1A1D-2A10 could recognize both D I/II and D III, a series of assays, including western blot, ELISA and indirect immunofluorescence assays (IFA), were carried out. Western blot shows that DVD-1A1D-2A10 can recognize recombinant D I/II and DIII proteins simultaneously (). In a competition ELISA, DVD-1A1D-2A10 demonstrated the same dual-specific binding ability (). Subsequently, to confirm whether both of the 2 variable regions of the DVD-1A1D-2A10 engaged virions, a series of indirect IFA were designed to detect dual-specific binding on infected BHK21 cells (). The results showed that DVD-1A1D-2A10 retained binding activity of both 1A1D and 2A10. We then compared the binding affinity of DVD-1A1D-2A10 to single epitope with its parental mAb. DVD-1A1D-2A10 showed almost exactly the same binding affinity as 2A10 on series diluted D I/II of E protein (), and a similar result was detected when D III of E protein was used (). As demonstrated by these results, the binding properties of the DVD-1A1D-2A10 were very similar to the parental mAbs.
Figure 2. Bispecific binding capacity of DVD-1A1D-2A10. (A) Western blot analysis of DVD-1A1D-2A10. 1A1D and 2A10 bind DIII and DI/II, respectively; DVD-Ig binds both D I/II and D III. (B) A competition ELISA was used to confirm DVD-1A1D-2A10 binding D I/II and D III simultaneously. (C) Bispecific binding to virus. BHK21 cells were infected with DENV2-43. Three to 5 days after infection, cells were fixed and analyzed by indirect immunofluorescence analysis. First, cells were blocked by murine antibodies (m1A1D, m2A10 and m1A1D+m2A10, respectively). Then, these cells were incubated with corresponding chimeric antibodies (c1A1D, c2A10 and cDVD-1A1D-2A10) and tested by fluorescein isothiocyanate (FITC)–conjugated anti-human IgG. Green means that chimeric antibody could bind to E proteins on the virus surface. Red represent negative cells. (D and E). Binding activity of DVD-1A1D-2A10 compared to parental antibodies. Increasing concentrations of DVD-1A1D-2A10, 1A1D, and 2A10 were added to 96-well plates coated with DI/II (D) or DIII (E). DVD-1A1D-2A10 retained equal binding activity of 1A1D and 2A10, respectively.
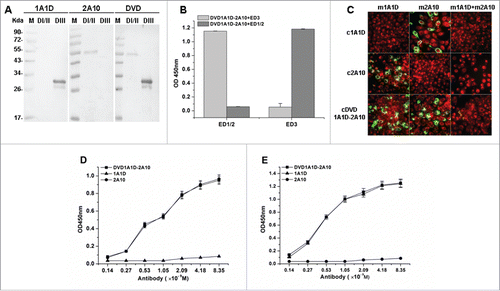
Neutralizing ability in vitro
To investigate the neutralizing potential of the DVD-1A1D-2A10, we used a standard plaque reduction neutralization assay on BHK-21 cells. 1A1D, 2A10 and a combination of them were added as controls. As shown in , the combination of 1A1D and 2A10 significantly increased the neutralizing activity against DENV1-3 compared with either single antibody. DVD-1A1D-2A10 further increased the neutralizing activity against DENV1-3, with plaque reduction neutralization test (PRNT)50 of 2 nM, 0.6 nM and 1.1 nM, respectively, an average of 10 times better than the combination of 1A1D and 2A10 (). However, because 1A1D has no neutralizing effect against DENV4, the DVD-1A1D-2A10 and the pair had quite similar DENV4-neutralizing ability compared to 2A10, with a PRNT50 of ∼30 nM (). Taken together, these results indicate that DVD-1A1D-2A10 has great potential as a neutralizing agent against all serotypes of DENV.
Figure 3. Enhanced neutralizing ability of DVD in vitro. DENV1-4 viruses were incubated with antibodies at different concentrations. Neutralization abilities were evaluated by PRNT assays using BHK21 cells. Results were exhibited by percentage of plaques reduction and shown as mean ± SD of 3 independent experiments.
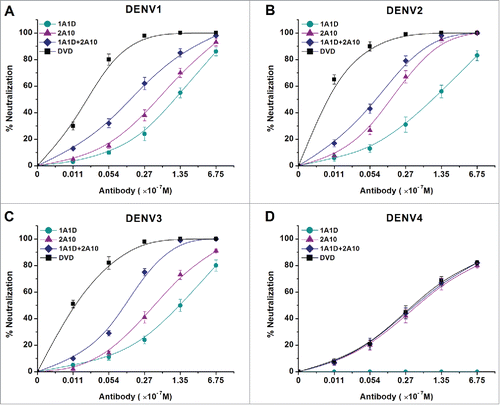
Table 1. PRNT50 values of antibodies against DENV1-4.
Because attachment and fusion are crucial steps of the virus infection, and 1A1D and 2A10 react with the regions that undertake these 2 processes, respectively, DVD-1A1D-2A10 theoretically might inhibit both attachment and fusion. Therefore, we performed a pre- and post-attachment inhibition assay. As expected, regardless of whether exposure was before or after virus attachment, DVD-1A1D-2A10 could achieve complete neutralization at a concentration of 1.33 μM. By contrast, the combination of 2 parental mAbs only achieved ∼70% neutralization at the same concentration (). Additionally, both 1A1D and 2A10 neutralized infection when applied before the attachment of the virus, but 1A1D's neutralizing ability was significant decreased when added after virus attachment to the cell surface. These results indicate that DVD-1A1D-2A10 might be used to prevent or treat the infection of all 4-serotype DENV in vivo.
Figure 4. DVD-1A1D-2A10 could neutralize DENV infection by blocking both viral adsorption and fusion. Regardless of blocking absorption (A) or fusion (B), DVD-1A1D-2A10 was more efficient than antibodies mixture or single mAb. In contrast, simply mixing 2 mAbs was not significantly better than single mAb. Neutralization percentages at the different antibody concentrations are shown as mean ± SD of 3 independent experiments.
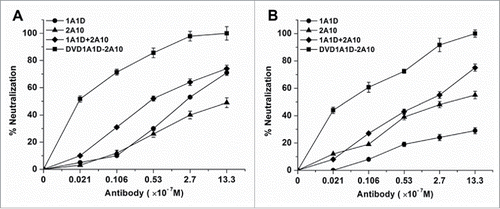
Elimination of ADE in vitro
It has already been confirmed that sub-neutralizing concentrations of neutralizing antibody enhanced DENV infection in Fcγ receptor positive cells, and that deleting 9 amino acids (positions 231–239) at the N terminus of Fc domain could eliminate ADE. Therefore, we deleted these 9 amino acids to construct a high affinity bispecific antibody without ADE. As we expected, both 1A1D and 2A10 had ADE effects at concentrations from 10−5 M to 10−11 M, which reached a peak at concentration of 10−8 M and 10−9 M, respectively. For DVD-1A1D-2A10, it exhibited lower ADE effects at concentrations from 10−7 M to 10−11 M. In contrast, the DVD-Fc9m did not present any ADE effect in all 4 serotypes of DENV ().
In vivo protection studies
A suckling mouse model was established to analyze the protection efficacy of DVD-1A1D-2A10 against lethal DENV 1-4 infection. In in vitro assays, 1A1D, 2A10 and the combination of them were used as references. As demonstrated in , there was no significant difference in protection from DENV4 infection between DVD-1A1D-2A10 and 2A10. However, DVD-1A1D-2A10 was shown to be significantly more effective in protecting mice from DENV 1–3 infection than 1A1D, 2A10, and 1A1D plus 2A10 (). Importantly, DVD-1A1D-2A10 at a concentration of 6.67×10−7 M could confer full protection against lethal DENV 1–3 challenge (). When the concentration of antibodies was reduced to 1.34×10−7 M, DVD-1A1D-2A10 still could protect at least 90% of mice from lethal DENV 1–3 infection, and it was significantly better than other mAbs or the mAb combination at the same concentration (). Statistical analysis in these assays between DVD-1A1D-2A10 and other groups use the log-rank test. These results indicated that there was a significant increase in protection ability of DVD-1A1D-2A10 compared to its parental mAbs or the combination of them in vivo ().
Figure 6. Enhanced neutralizing ability of DVD-1A1D-2A10 against DENV1-4 in vivo. Antibodies were diluted to corresponding concentrations, then pre-mixed with different virus. The mixture was inoculated into suckling mice by intracranial injection. Each group contains 8 to 12 suckling mice. Data only showed the final survival rate in percentage of the Kaplan-Meier survival curves, and the negative control mice (not shown) were dead 6 to 8 days after infection. The log-rank test was used to analyze the differences between the DVD groups and other antibodies treated groups. Significant differences are indicated by asterisks (*** P < 0.001, ** P < 0.01 and * P < 0.05).
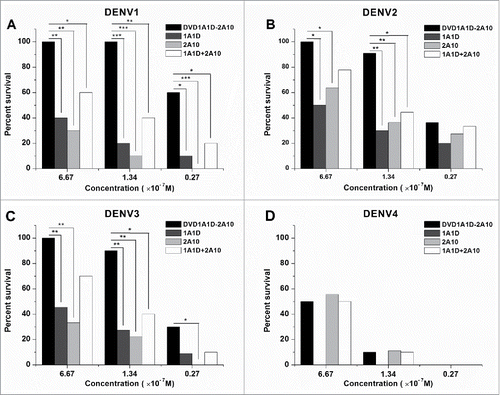
To explore the prophylactic and therapeutic efficacy, antibodies at a single dose (50 μg) were administered before or after lethal DENV challenge (200 PFU of DENV1-4). For prophylaxis, antibodies were injected 24 hours before intracranial virus infection. DVD-1A1D-2A10 did not have a significant improvement against DENV4 compared to 2A10 possibly because of the ineffectiveness of 1A1D against DENV4 (). In contrast, DVD-1A1D-2A10 protected 80%, 90% and 70% of suckling mice from lethal DENV1-3 challenge, respectively, compared to PBS-treated control group (P < 0.001), in which all died 6 to 9 days after infection (), while other antibodies exhibited much lower protection ability, with 20% to 60% survival rates (). To define the therapeutic potential of DVD-1A1D-2A10, we administered antibodies 4 hours later to intracranial virus infection of suckling mice with DENV1-4. As shown in , control groups showed morbidity at the sixth day after virus infection and died in the next 3 days. There was no difference between the 1A1D and PBS groups when mice were infected with DENV4. DVD-1A1D-2A10 could protect 40% mice from DENV4 infection, which was a little better than 2A10, but the result was without statistical significance (). In contrast, DVD-1A1D-2A10 protected above 70% of suckling mice from DENV1-3 infection, especially 100% survival rate on DENV2 (P < 0.001). In the meantime, the combination of 1A1D and 2A10 protected 36.4%, 80% and 60% of suckling mice from DENV1-3 infection respectively whereas single mAb only protected most 30% (). Log-rank test showed that significant differences existed between treated groups and PBS control. These results strongly demonstrated the prophylactic and therapeutic potential of DVD-1A1D-2A10 against severe DENV infections.
Figure 7. Preventive effects in vivo. Groups of one day old suckling mice were administrated with 50 μg of antibodies one day before challenge with 200 PFU of DENV1-4, respectively. PBS replaced antibody in the negative controls. The number of animals for each group ranged from 9 to 12. Kaplan-Meier survival curves were analyzed by the log-rank test and compared to curves of the PBS controls. Significant differences are indicated by asterisks (*** P < 0.001, ** P < 0.01 and * P < 0.05).
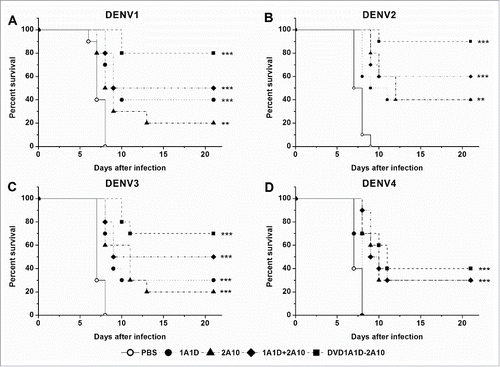
Figure 8. Therapeutic effects in vivo. Groups of one day old suckling mice were administrated with 50 μg of antibodies 4 hours after challenge with 200 PFU of DENV1-4, respectively. PBS replaced antibody in the negative controls. The number of animals for each group ranged from 9 to 12. Kaplan-Meier survival curves were analyzed by the log-rank test and compared to curves of the PBS controls. Significant differences are indicated by asterisks (*** P < 0.001, ** P < 0.01 and * P < 0.05).
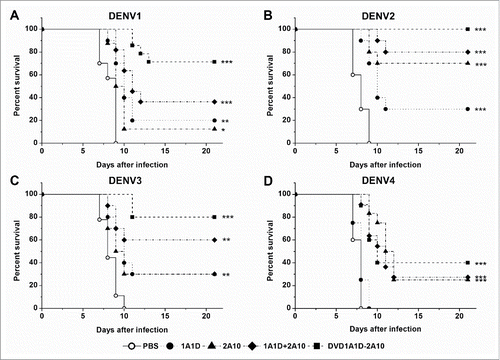
Discussion
Since 1975, when hybridoma was first generated, monoclonal antibodies have been envisioned as landmarks in the treatment of human disease. The past decades have brought several major breakthroughs in monoclonal antibody therapeutics development, including identification of relevant targets, antibody humanization and large-scale production. The number of mAbs under development for disease indications is growing rapidly, with about 440 different antibodies now in clinical trials. In most biologic systems, however, pathogenesis may involve multiple disease mediators or a pathogen acting in several independent steps. The utility of the combination of 2 or 3 mAbs has already been demonstrated in preclinical research, especially in tumor therapeutics,Citation19,20 but clinical use of 2 separate monoclonal antibodies as combination therapy is limited due to regulatory hurdles and cost. In addition, the efficacy and safety problems of the combination of 2 different mAbs have not been completely addressed.Citation21
A novel approach for engineering any 2 mAbs of distinct specificities into one immunoglobulin G-like molecules was recently described, and previous studies have demonstrated that the bispecific antibodies could preserve activities of the parental antibodies.Citation21,22 More importantly, bispecific antibodies can be efficiently produced at levels comparable to a conventional antibody molecule by mammalian expression system as a single species for easy manufacturing and purification.Citation21 Most bispecific antibodies were designed to treat tumor or autoimmune disease. In the present study, we engineered a bispecific antibody using 2 distinct anti-DENV mAbs, 1A1D and 2A10. Previous studies demonstrated that 1A1D could block the virus attaching to the cell surface, while 2A10 inhibited the virus from fusing with the endosomal membrane.Citation12,23 Attachment and fusion are 2 critical steps for the flavivirus infection, and the corresponding functional epitopes are located at E protein domain III and domain II, respectively. Blocking of either of the 2 steps could affect DENV infection.Citation6,7,24 Simultaneously blocking attachment and fusion is predicted to inhibit DENV infection more effectively, which has been demonstrated in our studies.
Multiple serotype DENV infections are very common. If an antibody cannot effectively neutralize 4 serotypes DENV at the same time, it may lead to ADE by facilitating the virus-antibody complex binding to Fc receptor-bearing cells.Citation4,25 High-titer viremia is the main reason for visceral disease such as the liver damage,Citation26 and viremia is generally 10- to 100-fold greater in DHF and DSS than in DF.Citation27,28 Passive transfer of a high dose of serotype-specific antibodies eliminated viremia, but lower doses of these antibodies or cross-reactive antibodies can induce more severe symptoms,Citation4 and poorly neutralizing cross-reactive antibodies raised in response to a previous serotype are believed to contribute to pathogenesis of severe dengue by promoting virus entry via Fcγ receptors, which plays a critical role in ADE and leads to the enhancement of infection.Citation29 Therefore, DVD-1A1D-2A10, the bispecific antibody with potent neutralizing activity against all 4 serotypes of dengue virus, may avoid ADE effects. In addition, we further mutated the Fc of DVD-1A1D-2A10 to prevent its binding to Fcγ receptors. Taken together, our bispecific anti-DENV antibody has been modified to totally eliminate the ADE effect.
In this study, DVD-1A1D-2A10, the bispecific antibody targeting different epitopes of dengue virus E protein, was shown to be significantly more effective in its neutralizing ability than the combination of its parental mAbs. In the binding assay, the bispecific antibody was shown to retain the full binding activities of both parental antibodies. This raises a question of why the bispecific antibody is better in neutralization. Recently, we engineered a bispecific antibody using 2 different mAbs against hepatitis B surface antigen.Citation18 This bispecific antibody showed significantly more potent HBV-neutralizing activity compared with the combination of both parental monoclonal antibodies.Citation18 We also developed a bispecific anti-ErbB2 antibody using trastuzumab and pertuzumab, 2 ErbB2-specific humanized antibodies that bind to different epitopes on ErbB2.Citation30 TPL showed superior ErbB2 heterodimerization-blocking activity over the combination of both parental monoclonal antibodies.Citation30 Further data indicated that TPL potently abrogated ErbB2 signaling in trastuzumab-resistant breast cancer cell lines.Citation30 In addition, we showed that TPL was far more effective than trastuzumab plus pertuzumab in inhibiting the growth of trastuzumab-resistant breast cancer cell lines.Citation30 These bispecific antibodies directed against 2 different epitopes of the same antigen often only retain the full binding activities of both parental antibodies. However, they show better activity than the combination of 2 parental antibodies. The most unexpected finding is the result reported by Lu and colleagues, who developed a bispecific anti-VEGFR-2 antibody.Citation31 The extracellular domain of VEGFR-2 consists of 7 Ig-like domains, and VEGF binds to the second and third Ig-like domains.Citation32 Lu and colleagues engineered a bispecific diabody using 2 anti-VEGFR2 single-chain variable fragments (scFvs).Citation31 One scFv binds to an epitope that is located on VEGFR-2 domain 1, and the other scFv binds to an epitope on domains 6 and 7. Neither of the 2 scFvs blocks VEGFR-2 from binding to VEGF, or has any effect on VEGF-induced receptor activation.Citation31 Surprisingly, the bispecific diabody effectively blocks VEGFR-2-VEGF interactions.Citation31 Taken together, these data suggest that the bispecific antibody against 2 different epitopes of the same antigen might exert unique effects through steric hindrance or by causing major conformational changes of the antigen. Particularly, for the bispecific antibody DVD-1A1D-2A10 against dengue virus, conformational changes of the E protein during the infection process of dengue virus might help explain the reason why the bispecific antibody is more effective in its neutralizing ability than the combination of its parental mAbs. The E protein has different conformational states in different stages of infection: it is a dimer in attachment, but a trimer when fusing with a host cell.Citation33 During the transition, the E protein rearranges and moves about 30° at the hinge between domains I and II.Citation34 The bispecific antibody DVD-1A1D-2A10, which binds DIII and DII of the E protein of dengue virus simultaneously, might hinder the conformational change necessary for the infection process of dengue virus through steric hindrance.
In summary, we generated a bispecific anti-DENV antibody that can neutralize all 4 serotypes of dengue virus without ADE effects. This bispecific antibody may be developed as a promising prophylactic and therapeutic agent against DENV infection.
Materials and methods
Cells and viruses
BHK21 cells were cultured in Dulbecco's Modified Eagle Medium (DMEM) supplemented with 10% fetal bovine serum (FBS). Mosquito cells C6/36 were maintained in RPMI-1640 medium supplemented with 10% FBS. Chinese hamster ovary (CHO) cells were cultured in 16DM (a mixture with 50% DMEM and 50% RPMI-1640) supplemented with 10% FBS. All cells were maintained at 37°C with 5% CO2, except for the C6/36 cells, which were maintained at 28°C. The broadly flavivirus cross-neutralizing antibody 2A10G6 (abbreviated as 2A10 below) was prepared as described previously.Citation12 The nucleotide sequence of the antibody 1A1D-2 (abbreviated as 1A1D below) was synthetized according to the Protein Data Bank (2R69H, 2R69L), and then the antibody was expressed in a mammalian cell expression system.
The flavivirus stains used in this research were DENV1-128, DENV2-43, DENV3-80-2 and DENV4-B5. All viruses were kept at State Key Laboratory of Pathogen and Biosecurity, and were prepared from culture supernatants of infected mosquito C6/36 cells or the suspensions of brains from infected suckling mice.
Construction of bispecific antibody
The anti-DENV bispecific antibody (anti-DENV dual-variable-domain immunoglobulin) was constructed as described previouslyCitation21,22 and denoted as DVD-1A1D-2A10. Briefly, the heavy chain variable region (VH) and light chain variable region (VL) of 1A1D were genetically fused to the 5′ terminus of the heavy chain and light chain of 2A10, respectively, through a short linker. The linker between the 2 variable regions in both the heavy chain (the amino acid sequence of the linker is ASTKGPSVF) and the light chain (the amino acid sequence of the linker is TVAAPSVFI) were selected from the N termini of CH1 and CΚ sequences, respectively. The resulting heavy and light chain genes were respectively cloned into the pCDNA3.1 (+) vector (Invitrogen, San Diego, CA), yielding the heavy and light chain expression vectors for the DVD-1A1D-2A10. Appropriate light and heavy expression vectors were then cotransfected into CHO cells. Stable transfectants producing the highest amount of recombinant protein were selected in normal medium with G418 and cultured in serum-free medium.Citation35 Finally, the bispecific antibody was purified by Protein A affinity chromatography from the serum-free supernatant.
Protein expression
The cDNA of DENV-2 E protein (GeneBank AF119661) were encoded into the pGEX-4T-2 expression plasmids using flanking BamH I and Xho I restriction sites. The recombinants were sequenced and transformed into BL21-DE3 competent E. coli and expression was induced with 0.6 mM isopropyl β-D-1-thiogalactopyranoside at 20°C. All E. coli were collected by centrifuge at 6000 rpm, 4°C. In addition, E protein was expressed with GST-tag, and purified by affinity chromatography. Soluble D III and D I/II of E protein was obtained through the same method.
Western blot
Proteins were separated using 10% SDS-PAGE and transferred to PVDF membranes. The membranes were blocked with PBST containing 5% skim milk and reacted with appropriate antibodies at room temperature (RT) for 1 hour. Then, membranes were washed 3 times with 0.1% Tween 20/Tris-buffered saline (TBST) and incubated with alkaline phosphatase-conjugated goat anti-mouse secondary antibody (1:1000). Subsequently, these membranes were incubated for 1 hour at RT, washed 3 times again and developed with NBT/BCIP (KPL).
Competition ELISA
A competition ELISA was used to confirm whether the DVD-1A1D-2A10 could bind 2 different parts of E protein at the same time. Briefly, 96-well microtiter plates were coated overnight at 4°C with 1 μg/ml recombinant D I/II or DIII protein in PBS. Plates were washed 3 times with PBST and blocked 2 hours at 37°C with 5% skim milk dissolved in PBST. Then plates were washed again as described above. In the meantime, DVD-1A1D-2A10 was incubated with excessive D III or D I/II protein at 37°C for 1 hour, then added into microtiter plate coated with the D III or D I/II protein and incubated for 1 hour at 37°C. Subsequently, plates were washed 5 times and then incubated with horseradish peroxidase-conjugated goat anti-mouse IgG (1:5000) at 37°C for 1 hour. Plates were washed 5 times and then reacted with TMB substrate. The reaction was stopped by adding 2 N H2SO4 to the medium, and emission (450 nm) was read using a microplate reader.
Indirect immunofluorescence assay
IFA were performed to illustrate the binding of DVD-1A1D-2A10 in cells. BHK21 cells were infected with DENV1-4. Cells were harvested 3 days post-infection, and resuspended in RPMI 1640 containing 10% FBS. The resuspended cells were dropped onto slides and incubated for 6 h at 37°C and 5% carbon dioxide. Then, the slides were fixed with acetone for 30 min in -20°C and rinsed twice in PBS for further use. Infected BHK21 cells were first blocked with 20 μg/ml murine-1A1D (m1A1D), m2A10 or m1A1D+m2A10 at 37°C for 1 hour. Then cells were washed 3 times with PBS. Next, the blocked infected cells were incubated with 1 μg/ml chimeric-1A1D (ch1A1D), ch2A10 or chDVD, respectively, for 1 hour at 37°C. After washing again, cells were treated with 200-fold dilution of FITC-conjugated anti-human IgG in 0.02% (w/v) Evans Blue for 30 mins at 37°C and rinsed with PBS. After washing 5 times in PBS, the cells on the glass slides were observed under a fluorescent microscope.
Neutralization assay
A standard plaque reduction neutralization test (PRNT) was adopt to evaluate the neutralizing ability of antibodies.Citation36,37 Serial 5-fold dilutions of antibodies were mixed with the same volume of DENV 1-4 (˜200 PFU) and incubated at 37°C for 1 hour. Then, mixtures were added to monolayers of BHK21 cells in 12-well plates. After 1 hour adsorption at 37°C, the supernatant was removed, and 1 ml of 1% (w/v) low melting point agarose in 2×DMEM with 4% FBS were layered onto the infected cells. After an additional incubation at 37°C for 5 days, the cells were fixed using 4% formaldehyde and stained with 1% crystal violet to visualize the plaques. Percentage of plaque reduction was calculated as described previously.Citation7 PRNT50 values were calculated by doing a non-linear regression analysis using Sigmaplot (Version 10.0, Systat Software, Inc.., CA)
Animal experiments
Suckling mice were used to identify neutralizing ability of the DVD-1A1D-2A10 in vivo. DENV1-4 (1×104 PFU/ml) were mixed with an equal volume of serial dilutions of antibodies and incubated for 1 hour at 37°C. Then the mixture was injected intracerebrally into 1-day-old suckling mice. The control group was only administered PBS buffer. For 3 weeks after injection, mice were monitored daily for clinical signs of infection, including asitia, a hunched back, paralysis and death. Protection differences between the DVD and other groups were evaluated using the log-rank test.
To assess the prophylactic and therapeutic efficacy, single doses (50 μg) of antibodies were administered by intraperitoneal injection 1 day before or 4 hours after mice were challenged with 200 PFU of DENV1-4. The survival status of the suckling mice was observed and calculated as previous described. Protection significance was evaluated using the log-rank test and compared to the PBS controls.
Adsorption and fusion blocking assay
Blocking of DENV2 infection at adsorption or fusion step was performed using 200 PFU of DENV2 and serial 5-fold dilutions of antibodies. For the adsorption blocking assay, antibodies were added to BHK21 cells in 12-well plates and incubated for 1 hour at 4°C before virus infection. For the fusion blocking assay, DENV2 were added to BHK21 cells for 1 hour at 4°C firstly, then the antibodies were added and incubated for additional 1 hour at 4°C. After three washes with PBS, the PRNT protocol was followed as described above.
Measurement of ADE in vitro
K562 cell line is a well-accepted model to study ADE. We determined the ADE of dengue virus infection in K562 cells. Serial 10-fold dilutions of antibodies were mixed with DENV1-4, and incubated for 1 h at 37°C. Mixtures were then added to 2×105 K562 cells at multiplicity of infection of 0.1˜0.25 for 2 h in 12-well plates. The cells were subsequently washed with PBS 3 times. After collecting cells by centrifugation, the cell pellets were resuspended with RPMI-1640 medium containing 2% FBS and added to 12-well plates, then incubated for 4 days at 37°C with 5% CO2. The titer of viruses in the supernatant was then measured by PRNT. The ADE effect was calculated as different viral yields in the supernatant after infection in the presence of the added antibodies.
Disclosure of potential conflicts of interest
No potential conflicts of interest were disclosed.
Funding
This work was supported by grants from the National Key Project for Infectious Diseases (2012ZX10002012-009), National Natural Science Foundation of China (No. 81101243, No.31270974, No.31500755), Shanghai Key Laboratory of Cell Engineering (14DZ2272300) and Shanghai Leading Academic Discipline Project (B905). Huajing Wang was supported by Military Logistic Project (CWS12J086). Chengfeng Qin was supported by Excellent Young Scientist Program of NSFC (NO.81522025).
References
- Mackenzie JS, Gubler DJ, Petersen LR. Emerging flaviviruses: the spread and resurgence of Japanese encephalitis, West Nile and dengue viruses. Nat Med 2004; 10:S98-109; PMID:15577938; http://dx.doi.org/10.1038/nm1144
- Halstead SB. Pathogenesis of dengue: challenges to molecular biology. Science 1988; 239:476-81; PMID:3277268; http://dx.doi.org/10.1126/science.3277268
- Narvaez F, Gutierrez G, Perez MA, Elizondo D, Nunez A, Balmaseda A, Harris E. Evaluation of the traditional and revised WHO classifications of Dengue disease severity. PLoS Negl Trop Dis 2011; 5:e1397; PMID:22087348; http://dx.doi.org/10.1371/journal.pntd.0001397
- Balsitis SJ, Williams KL, Lachica R, Flores D, Kyle JL, Mehlhop E, Johnson S, Diamond MS, Beatty PR, Harris E. Lethal antibody enhancement of dengue disease in mice is prevented by Fc modification. PLoS Pathog 2010; 6:e1000790; PMID:20168989; http://dx.doi.org/10.1371/journal.ppat.1000790
- Li L, Lok SM, Yu IM, Zhang Y, Kuhn RJ, Chen J, Rossmann MG. The flavivirus precursor membrane-envelope protein complex: structure and maturation. Science 2008; 319:1830-4; PMID:18369147; http://dx.doi.org/10.1126/science.1153263
- Mukhopadhyay S, Kuhn RJ, Rossmann MG. A structural perspective of the flavivirus life cycle. Nat Rev Microbiol 2005; 3:13-22; PMID:15608696; http://dx.doi.org/10.1038/nrmicro1067
- Lok SM, Kostyuchenko V, Nybakken GE, Holdaway HA, Battisti AJ, Sukupolvi-Petty S, Sedlak D, Fremont DH, Chipman PR, Roehrig JT, et al. Binding of a neutralizing antibody to dengue virus alters the arrangement of surface glycoproteins. Nat Struct Mol Biol 2008; 15:312-7; PMID:18264114; http://dx.doi.org/10.1038/nsmb.1382
- Oliphant T, Engle M, Nybakken GE, Doane C, Johnson S, Huang L, Gorlatov S, Mehlhop E, Marri A, Chung KM, et al. Development of a humanized monoclonal antibody with therapeutic potential against West Nile virus. Nat Med 2005; 11:522-30; PMID:15852016; http://dx.doi.org/10.1038/nm1240
- Crill WD, Roehrig JT. Monoclonal antibodies that bind to domain III of dengue virus E glycoprotein are the most efficient blockers of virus adsorption to Vero cells. J Virol 2001; 75:7769-73; PMID:11462053; http://dx.doi.org/10.1128/JVI.75.16.7769-7773.2001
- Kuhn RJ, Zhang W, Rossmann MG, Pletnev SV, Corver J, Lenches E, Jones CT, Mukhopadhyay S, Chipman PR, Strauss EG, et al. Structure of dengue virus: implications for flavivirus organization, maturation, and fusion. Cell 2002; 108:717-25; PMID:11893341; http://dx.doi.org/10.1016/S0092-8674(02)00660-8
- Modis Y, Ogata S, Clements D, Harrison SC. Structure of the dengue virus envelope protein after membrane fusion. Nature 2004; 427:313-9; PMID:14737159; http://dx.doi.org/10.1038/nature02165
- Deng YQ, Dai JX, Ji GH, Jiang T, Wang HJ, Yang HO, Tan WL, Liu R, Yu M, Ge BX, et al. A broadly flavivirus cross-neutralizing monoclonal antibody that recognizes a novel epitope within the fusion loop of E protein. PLoS One 2011; 6:e16059; PMID:21264311; http://dx.doi.org/10.1371/journal.pone.0016059
- Roehrig JT, Staudinger LA, Hunt AR, Mathews JH, Blair. Antibody prophylaxis and therapy for flavivirus encephalitis infections. Ann N Y Acad Sci 2001; 951:286-97; PMID:11797785; http://dx.doi.org/10.1111/j.1749-6632.2001.tb02704.x
- Engle MJ, Diamond MS. Antibody prophylaxis and therapy against West Nile virus infection in wild-type and immunodeficient mice. J Virol 2003; 77:12941-9; PMID:14645550; http://dx.doi.org/10.1128/JVI.77.24.12941-12949.2003
- Morrey JD, Siddharthan V, Olsen AL, Roper GY, Wang H, Baldwin TJ, Koenig S, Johnson S, Nordstrom JL, Diamond MS. Humanized monoclonal antibody against West Nile virus envelope protein administered after neuronal infection protects against lethal encephalitis in hamsters. J Infect Dis 2006; 194:1300-8; PMID:17041857; http://dx.doi.org/10.1086/508293
- Rajamanonmani R, Nkenfou C, Clancy P, Yau YH, Shochat SG, Sukupolvi-Petty S, Schul W, Diamond MS, Vasudevan SG, Lescar J. On a mouse monoclonal antibody that neutralizes all four dengue virus serotypes. J Gen Virol 2009; 90:799-809; PMID:19264660; http://dx.doi.org/10.1099/vir.0.006874-0
- Nowakowski A, Wang C, Powers DB, Amersdorfer P, Smith TJ, Montgomery VA, Sheridan R, Blake R, Smith LA, Marks JD. Potent neutralization of botulinum neurotoxin by recombinant oligoclonal antibody. Proc Natl Acad Sci U S A 2002; 99:11346-50; PMID:12177434; http://dx.doi.org/10.1073/pnas.172229899
- Tan W, Meng Y, Li H, Chen Y, Han S, Zeng J, Huang A, Li B, Zhang Y, Guo Y. A bispecific antibody against two different epitopes on hepatitis B surface antigen has potent hepatitis B virus neutralizing activity. MAbs. 2013; 5:946-55; PMID:24492346; http://dx.doi.org/10.4161/mabs.26390
- Arndt M, Krauss J. Bispecific diabodies for cancer therapy. Methods Mol Biol 2003; 207:305-21; PMID:12412482
- Peipp M, Valerius T. Bispecific antibodies targeting cancer cells. Biochem Soc Trans 2002; 30:507-11; PMID:12196124; http://dx.doi.org/10.1042/bst0300507
- Wu C, Ying H, Grinnell C, Bryant S, Miller R, Clabbers A, Bose S, McCarthy D, Zhu RR, Santora L, et al. Simultaneous targeting of multiple disease mediators by a dual-variable-domain immunoglobulin. Nat Biotechnol 2007; 25:1290-7; PMID:17934452; http://dx.doi.org/10.1038/nbt1345
- Kou G, Shi J, Chen L, Zhang D, Hou S, Zhao L, Fang C, Zheng L, Zhang X, Liang P, et al. A bispecific antibody effectively inhibits tumor growth and metastasis by simultaneous blocking vascular endothelial growth factor A and osteopontin. Cancer Lett 2010; 299:130-6; PMID:20826049; http://dx.doi.org/10.1016/j.canlet.2010.08.011
- Nybakken GE, Oliphant T, Johnson S, Burke S, Diamond MS, Fremont DH. Structural basis of West Nile virus neutralization by a therapeutic antibody. Nature 2005; 437:764-9; PMID:16193056; http://dx.doi.org/10.1038/nature03956
- Thompson BS, Moesker B, Smit JM, Wilschut J, Diamond MS, Fremont DH. A therapeutic antibody against west nile virus neutralizes infection by blocking fusion within endosomes. PLoS Pathog 2009; 5:e1000453; PMID:19478866; http://dx.doi.org/10.1371/journal.ppat.1000453
- Halstead SB. Neutralization and antibody-dependent enhancement of dengue viruses. Adv Virus Res 2003; 60:421-67; PMID:14689700; http://dx.doi.org/10.1016/S0065-3527(03)60011-4
- Jessie K, Fong MY, Devi S, Lam SK, Wong KT. Localization of dengue virus in naturally infected human tissues, by immunohistochemistry and in situ hybridization. J Infect Dis 2004; 189:1411-8; PMID:15073678; http://dx.doi.org/10.1086/383043
- Vaughn DW, Green S, Kalayanarooj S, Innis BL, Nimmannitya S, Suntayakorn S, Endy TP, Raengsakulrach B, Rothman AL, Ennis FA,et al. Dengue viremia titer, antibody response pattern, and virus serotype correlate with disease severity. J Infect Dis 2000; 181:2-9; PMID:10608744; http://dx.doi.org/10.1086/315215
- Murgue B, Roche C, Chungue E, Deparis X. Prospective study of the duration and magnitude of viraemia in children hospitalised during the 1996-1997 dengue-2 outbreak in French Polynesia. J Med Virol 2000; 60:432-8; PMID:10686027; http://dx.doi.org/10.1002/(SICI)1096-9071(200004)60:4%3c432::AID-JMV11%3e3.0.CO;2-7
- Halstead SB, Lan NT, Myint TT, Shwe TN, Nisalak A, Kalyanarooj S, Nimmannitya S, Soegijanto S, Vaughn DW, Endy TP. Dengue hemorrhagic fever in infants: research opportunities ignored. Emerg Infect Dis 2002; 8:1474-9; PMID:12498666; http://dx.doi.org/10.3201/eid0812.020170
- Li B, Meng Y, Zheng L, Zhang X, Tong Q, Tan W, Hu S, Li H, Chen Y, Song J, et al. Bispecific antibody to ErbB2 overcomes trastuzumab resistance through comprehensive blockade of ErbB2 heterodimerization. Cancer Res. 2013; 73:6471-83; PMID:24046294; http://dx.doi.org/10.1158/0008-5472.CAN-13-0657
- Lu D, Kotanides H, Jimenez X, Zhou Q, Persaud K, Bohlen P, Witte L, Zhu Z. Acquired antagonistic activity of a bispecific diabody directed against two different epitopes on vascular endothelial growth factor receptor 2. J Immunol Methods 1999; 230:159-71; PMID:10594363; http://dx.doi.org/10.1016/S0022-1759(99)00135-0
- Roskoski R Jr. VEGF receptor protein-tyrosine kinases: structure and regulation. Biochem Biophys Res Commun 2008; 375:287-91; PMID:18680722; http://dx.doi.org/10.1016/j.bbrc.2008.07.121
- Li L, Lok SM, Yu IM, Zhang Y, Kuhn RJ, Chen J, Rossmann MG. The flavivirus precursor membrane-envelope protein complex: structure and maturation. Science 2008; 319:1830-4; PMID:18369147; http://dx.doi.org/10.1126/science.1153263
- Mukhopadhyay S, Kuhn RJ, Rossmann MG. A structural perspective of the flavivirus life cycle. Nat Rev Microbiol 2005; 3:13-22; PMID:15608696; http://dx.doi.org/10.1038/nrmicro1067
- Dai J, Li B, Shi J, Peng L, Zhang D, Qian W, Hou S, Zhao L, Gao J, Cao Z, et al. A humanized anti-osteopontin antibody inhibits breast cancer growth and metastasis in vivo. Cancer Immunol Immunother 2010; 59:355-66; PMID:19690854; http://dx.doi.org/10.1007/s00262-009-0754-z
- Gromowski GD, Barrett AD. Characterization of an antigenic site that contains a dominant, type-specific neutralization determinant on the envelope protein domain III (ED3) of dengue 2 virus. Virology 2007; 366:349-60; PMID:17719070; http://dx.doi.org/10.1016/j.virol.2007.05.042
- Matsui K, Gromowski GD, Li L, Schuh AJ, Lee JC, Barrett AD. Characterization of dengue complex-reactive epitopes on dengue 3 virus envelope protein domain III. Virology 2009; 384:16-20; PMID:19101005; http://dx.doi.org/10.1016/j.virol.2008.11.013