ABSTRACT
The prevailing techniques used to generate antibody-drug conjugates (ADCs) involve random conjugation of the linker-drug to multiple lysines or cysteines in the antibody. Engineering natural and non-natural amino acids into an antibody has been demonstrated to be an effective strategy to produce homogeneous ADC products with defined drug-to-antibody ratios. We recently reported an efficient residue-specific conjugation technology (RESPECT) where thiol-reactive payloads can be efficiently conjugated to a native unpaired cysteine in position 80 (C80) of rabbit light chains. Deimmunizing the rabbit variable domains through humanization is necessary to reduce the risk of anti-drug antibody responses in patients. However, we found that first-generation humanized RESPECT ADCs showed high levels of aggregation and low conjugation efficiency. We correlated these negative properties to the phenylalanine at position 83 present in most human variable kappa frameworks. When position 83 was substituted with selected amino acids, conjugation was restored and aggregation was reduced to levels similar to the chimeric ADC. This engineering strategy allows for development of second-generation humanized RESPECT ADCs with desirable biopharmaceutical properties.
Abbreviations
ADC | = | antibody-drug conjugate |
mAb | = | monoclonal antibody |
RESPECT | = | REsidue SPEcific Conjugation Technology |
Vκ | = | variable kappa domain |
Cκ | = | constant kappa domain |
DAR | = | drug to antibody ratio |
CDR | = | complementarity-determining region |
FWR | = | framework |
LC | = | light chain |
HC | = | heavy chain |
Introduction
Most manufacturing processes used for the production of antibody-drug conjugates (ADCs) use random conjugation of cytotoxins to either cysteines or lysines on the monoclonal antibody (mAb). The resultant products are a heterogeneous mixture of molecules with variable drug-to-antibody ratios (DARs) typically ranging from 2 to 6 (For reviews, see refs. Citation1–5). Current efforts to produce homogeneous ADC products utilize site-specific conjugation technologies, including the use of non-natural amino acids with unique orthogonal side-chain chemistry,Citation6 enzymatic modification of an engineered glutamine tag by transglutaminase,Citation7,Citation8 and engineering unpaired cysteines into the mAb, to allow conjugation of thiol-reactive molecules.Citation9
Rabbit antibodies are unique in that the variable kappa (Vκ) domain is often covalently linked to the constant kappa (Cκ) domain through an intrachain disulfide bond between residues 80 (C80, Kabat numberingCitation10) and 171 (C171, EU numberingCitation11).Citation12 During chimerization, the rabbit constant domains are genetically replaced with the human gamma and kappa domains; C80 no longer forms a disulfide bond with C171 and is therefore unpaired. We recently reported the development of REsidue SPEcific Conjugation Technology (RESPECT), which leverages covalent conjugation to the unpaired C80 in rabbit-human chimeric mAbs with thiol-reactive payloads.Citation13 The use of the native C80 as a site of conjugation offers an advantage over cysteine engineering methods whereby this residue is only moderately accessible to solvent due to the disulfide bond naturally occurring with C171. High solvent-exposure of engineered cysteines in antibodies has been shown to lead to the formation of intermolecular disulfides and poor conjugatability.Citation9,Citation14-16 However, moderately-exposed unpaired cysteines have been demonstrated to lead to ADCs having improved serum stability and pharmacokinetic parameters.Citation9,Citation15,Citation17 RESPECT mAbs and resultant ADCs demonstrated high conjugation efficiency, low aggregate levels, excellent target binding specificity, retention of binding affinity, and low off-target cytotoxicity, all desirable properties for therapeutic ADCs.Citation13 Specific tumor targeting was demonstrated by an anti-carbonic anhydrase 9 (CA9) mAb conjugated with maleimido-IRDye 800CW. Further, a non-cleavable maleimido-derivative of auristatin F conjugated at a DAR of 2.0 to anti-mesothelin mAbs showed potent anti-tumor activity in a human xenograft tumor model.
While chimeric antibodies could potentially elicit immunogenic responses in human patients, humanization strategies reduce potential immunogenicity via grafting the non-human complementarity-determining regions (CDRs) onto a human germline framework most similar to the non-human framework. Important objectives of humanization are also the retention of high antigen binding affinity as well as preservation of preferred biophysical properties of the antibody, such as low aggregation and high thermal stability.Citation18-23 In the case of RESPECT antibodies, the humanization process must also preserve high efficiency of cytotoxin conjugation without negatively affecting the biophysical properties of the ADC. The tertiary structure of framework residues adjacent to CDRs have been shown to affect antigen affinity,Citation19,Citation20,Citation22 but it was unknown whether the residues surrounding C80 have an effect on conjugation or antibody stability. Previous reports have found that the location of an engineered unpaired cysteine is essential to efficient conjugation.Citation9 All reported humanization of rabbit mAbs has involved mutating C80 to the residue found in the human equivalent (often a proline or alanine), and therefore the effect of retaining an unpaired C80 during humanization was unknown.Citation24-29
Of note, all 68 rabbit kappa variable genes (IMGT databaseCitation30) are most homologous to the human IGKV1 family, and the grafting of rabbit CDRs onto this human kappa framework has been demonstrated by several groups.Citation24-26,Citation29,Citation31,Citation32 Here, we show that the previously reported humanization strategy is not suitable for RESPECT mAbs because it significantly alters their biophysical properties, resulting in high aggregation levels and lower conjugation efficiency in first-generation humanized RESPECT ADCs. By systematic mutational analysis, as well as engineering and characterization of several humanized RESPECT mAbs, we developed a humanization process that preserves optimal biopharmaceutical properties of second-generation humanized RESPECT ADCs.
Results
Humanization of RESPECT mAbs using conventional CDR grafting leads to ADC with undesired properties
First-generation humanized RESPECT mAbs were engineered by grafting the Kabat-defined CDRs of an anti-human CA9 rabbit antibody 155D5 onto the closest human germline sequence (IGHV3–64*04 and IGKV1–5*03), as determined by IGBLAST (Table S1),Citation33 while retaining the rabbit C80 residue. The resulting antibody, named hu155D5–1, was expressed and purified using protein A affinity purification. Similar to chimeric 155D5 (xi155D513), hu155D5–1 was cysteinylated (146,457 Da, ), and the capping cysteine could be removed using the on-column decysteinylation protocol developed by Albone et al.Citation13 and briefly described in the Material and Methods section (146,224 Da, ). Unexpectedly, and unlike xi155D513 (), no significant conjugation could be observed to hu155D5–1 light chain (LC) when incubated with a 4-fold molar excess of maleimide-PEG2-biotin ().
Figure 1. Analysis of hu155D5–1 cysteinylation and thiol-reactivity. Hu155D5–1 was purified using (A) standard protein A affinity chromatography or (B) a decysteinylation protocol.Citation13 Following deglycosylation by PNGase F, the non-reduced mass was analyzed by ESI-MS. The mass of 146,457 represents a cysteinylated mAb. The additional mass peaks (e.g., 146,777) are likely a result of incomplete deglycosylation before analysis. Decysteinylated xi155D5 and hu155D5–1 before (C and E, respectively) and after (D and F, respectively) incubation with maleimide-PEG2-biotin were reduced with 20 mM DTT and the masses of the LCs were analyzed by ESI-MS.

To rule out that the conjugation inefficiency was specific to the human Vκ framework used (IGKV1–5*03), 155D5 CDRs were subsequently grafted onto other human Vκ families. Because human IGKV families have multiple alleles, the protein sequences for all allelesCitation30 were first aligned within their family and redundant framework alleles were removed, leaving only one framework sequence for each of the IGKV4, −5, −6, −6D, and −7 families. In addition, there are 37, 16, and 15 non-redundant alleles in IGKV1, −2, and −3 families, respectively, and the allele with the closest homology to the consensus amino acid sequence was chosen for each family and used as the framework to humanize 155D5 (Fig. S1). Consequently, the human frameworks IGKV1–39*01, IGKV2–40*01, IGKV3–11*01, IGKV4–1*01, IGKV5–2*01, IGKV6–21*01, IGKV6D-41*01, and IGKV7–3*01 were used for CDR-grafting the 155D5 CDRs, while retaining C80 (). The 155D5 humanized variants were named according to the human germline sequence used, e.g., hu155D5-Vκ1–39. Hu155D5-Vκ5 and -Vκ7 contain N-linked glycosylation sites at residues 20 and 81, respectively. To reduce heterogeneity of the mAb product due to the presence of multiple glycosylation species in the sample, Asn20 in hu155D5-Vκ5 was changed to a Thr as in the sequence of 155D5. However, hu155D5-Vκ5–2-Thr20 was poorly expressed and could not be purified (data not shown). Similarly, Asn81 in hu155D5-Vκ7 was changed to Glu as in all other human Vκ families to remove glycosylation at this position.
Figure 2. Aggregation levels and conjugation efficiency of different variants of zu155D5 generated by using various human Vκ subfamilies. (A) The CDRs of 155D5 were grafted on several human germline frameworks. black: common residues between human and rabbit frameworks, red: unique human framework residues, green: C80, highlighted: residue 83 (B) Humanized 155D5 variants were analyzed for aggregation by SEC-HPLC after protein A (black) and decysteinylation (gray) purification. As in , ESI-MS of non-reduced mAbs was used to determine the percent of decysteinylated C80 (hashed) and percent conjugation (dotted) following incubation with maleimide-PEG2-biotin. * Percent aggregates after protein A purification was not analyzed for xi155D5.
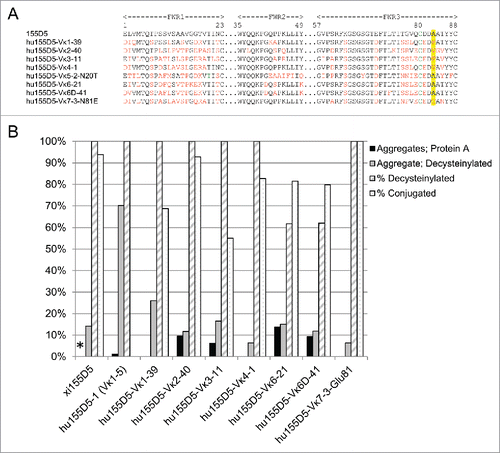
Consistent with chimeric RESPECT mAbs, the first-generation humanized RESPECT mAbs were found to be cysteinylated at C80. The lack of conjugation to hu155D5–1 was not due to inefficient decysteinylation of C80 as the mAb was decysteinylated (). Conjugation efficiencies between 80–100% were observed for hu155D5-Vκ2–40, -Vκ4–1, -Vκ6–21, -Vκ6D-41, and -Vκ7–3-Glu81; in contrast, conjugation efficiency was less than 70% for hu155D5-Vκ1–39 and -Vκ3–11 (), indicating a differential effect of framework sequences on conjugation efficiency.
MAbs were also analyzed by size-exclusion high performance liquid chromatography (SEC-HPLC) to determine aggregation levels before and after decysteinylation. The aggregation levels before decysteinylation varied from low (0–6%) to moderate (9–14%), and increased after decysteinylation (). The aggregation levels in hu155D5-Vκ4–1 and -Vκ7–3-Glu81 remained low at 6.3%, while moderate aggregation (11–15%) was seen in hu155D5-Vκ2–40, -Vκ6–21, and -Vκ6D-41. High levels of aggregation as well as low conjugation efficiency were observed in the remaining mAbs. Hu155D5–1 (huVκ1–5) had the highest amount of aggregation at 70.1%, followed by hu155D5-Vκ1–39 at 26% and hu155D5-Vκ3–11 at 16.5%. Analysis of the Vκ frameworks alignment revealed a common pattern wherein the residue in position 83 was a phenylalanine (F83) in mAbs showing higher aggregation levels or lower conjugation efficiency.
Phenylalanine in position 83 negatively affects conjugation efficiency in first-generation humanized RESPECT mAbs
The potential role of F83 and other amino acids close to C80 in causing increased aggregation and reduced conjugation efficiency was investigated. In silico 3D homology modeling of xi155D5 () and hu155D5–1 variable regions identified residues within an estimated distance of 15 Å from C80. In a series of humanized variants based on the IGKV1–5 framework, the rabbit residues in position 11, 14, 17, 18, 63, 76, 77, 78, 83, 103, or 104 were retained along with C80 during the humanization engineering. As seen in , conjugation and aggregation was highly influenced by residue 83. Mutating residue 83 to alanine (hu155D5–2) was sufficient to increase conjugation efficiency from 0% to 80.1% and decrease aggregation levels from 70.1% to 17.3%. This level of conjugation and aggregation was observed in all mAbs that contained A83 except hu155D5–4 where rabbit residue positions 76–78 appeared to have a negative impact on conjugation and aggregation.
Figure 3. Humanized 155D5 sequences. (A) The structure of 155D5 was modeled in silico using Biovia Discover Studio. The VH is colored orange and the Vκ is colored blue. C80 is shown as a space-fill representation. Residues within 15 Å of C80 and differing between 155D5 and hu155D5–1 are highlighted in yellow. (B) Various rabbit residues in 155D5 were retained during the humanization process throughout framework (FWR)1, FWR3, and FWR4. Black residues (except within CDRs) are present in rabbit (155D5), human germline (IGKV1–5*01) and humanized sequences. Residues changed to human are highlighted in red. Residues changed back to rabbit are highlighted in green. Light chain C80 is highlighted in green. (C) Humanized 155D5 variants were analyzed for aggregation by SEC-HPLC after protein A (black) or decysteinylation (gray) purification. The percent of decysteinylated C80 (hashed) and percent conjugation (dotted) were determined as in .
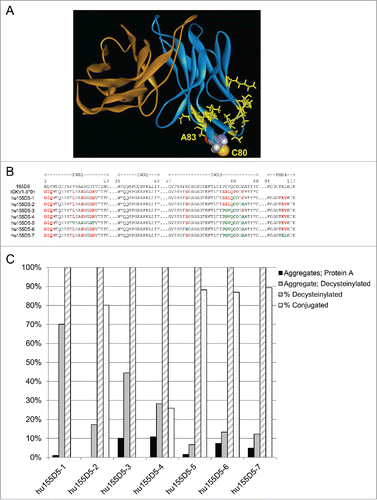
Second-generation humanized RESPECT ADCs via optimization of framework sequence in various mAbs
We then explored whether the effect of residue 83 on aggregation and conjugation was specific to the 155D5 CDRs and if the aforementioned humanization strategy could be applied to other RESPECT mAbs having unique variable sequences. Therefore, rabbit 1E4, 166B3, and 33O11 antibodies were humanized by grafting their CDRs onto the most homologous human frameworks (IGKV1–39*02, IGKV1–39*01, and IGKV1–39*01, respectively) with either phenylalanine (humAb-1) or alanine (humAb-2) at position 83. As seen with hu155D5–1, F83 increased aggregation and lowered conjugation efficiency in humanized 1E4, 166B3, and 33O11 RESPECT mAbs, while A83 restored optimal biophysical properties ().
Figure 4. Aggregation and conjugation efficiency of other humanized rabbit mAbs. Four mAbs were analyzed for the influence of position 83 on aggregate formation, decysteinylation, and conjugation to maleimide-PEG2-biotin. MAbs were analyzed for aggregation by SEC-HPLC after protein A (black) or decysteinylation (gray) purification. The percent of decysteinylated C80 (hashed) and percent conjugation (dotted) were determined as in .
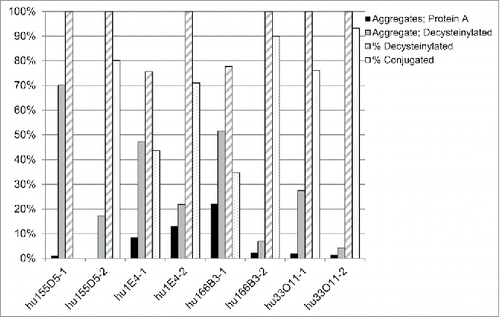
Furthermore, we analyzed the effect of substituting F83 with any of the other amino acids (except cysteine) on aggregation levels and conjugation efficiency using 1E4. Due to low expression, G83 mutant could not be decysteinylated or conjugated and was not analyzed further. The amount of aggregates for all mutants before decysteinylation varied little and was below 15% for all samples, while the F83 variant showed aggregation levels at 22% (). Similarly, aggregation was low for all mutants after decysteinylation (between 12 to 25%), except F83 (47%). Following the decysteinylation protocol, all samples were 100% decysteinylated except F83 (78%) and K83 (50%). Conjugation efficiency ranged from 70 to 97% in all mutants, but was only 44% for both F83 and K83.
Figure 5. Influence of position 83 on aggregation and conjugation. Humanized 1E4 mutants with each amino acid (except cysteine and isoleucine) and humanized 33O11 with isoleucine at position 83 were analyzed for aggregation by SEC-HPLC after protein A (black) or decysteinylation (gray) purification. The percent of decysteinylated C80 (hashed) and percent conjugation (dotted) were determined as in .
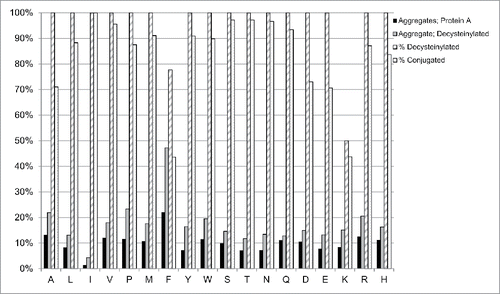
Figure 6. In vitro killing of CA9-expressing cells by an AuF-conjugated C80-containing mAb. Following a 3-day incubation with AuF-conjugated REPECT ADCs, the percent killing was analyzed by staining cells in 0.2% crystal violet followed by lysis in 1% SDS. The absorbance at 570 nm was determined, and percent killing was calculated by dividing the absorbance of the sample by the absorbance of untreated cells and subtracting this value from 1. The EC50s for Mal-PEG2-AuF in HCT116 and HCT116-CA9 cells were 0.27 nM and 0.30 nM, respectively. The EC50s for humanized hu155D5–2 and xi155D5 in HCT116-CA9 cells were 0.57 nM and 0.81 nM EC50, respectively. The control xi1–55–2 ADC showed no significant activity against target-negative or target-positive cells.
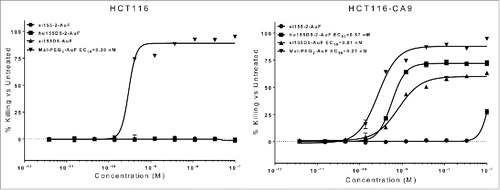
Second-generation humanized RESPECT ADCs possess ideal biophysical and therapeutic properties
To assess whether the humanization method we developed does not alter the therapeutic potential previously seen in a chimeric RESPECT ADC,Citation13 we performed in vitro cytotoxicity assays comparing hu155D5–2 to xi155D5, a rabbit-human chimeric antibody specific to human endosialin, a protein not expressed by human colorectal HCT116 cells (data not shown). MAbs were purified, decysteinylated, and conjugated with maleimide (mal)-PEG2-Auristatin F (AuF) as described previously.Citation13 All ADCs demonstrated low aggregation levels and high conjugation efficiency (DAR >1.9, data not shown). Cells expressing human CA9 (HCT116-CA9) and parental HCT116 cells were treated with xi155D5, hu155D5–2, or xi1–55–2 AuF RESPECT ADCs. Due to the addition of the mal-PEG2 linker to the carboxylic acid of AuF,Citation13 unconjugated mal-PEG2-AuF was cytotoxic to both HCT116 and HCT116-CA9 cells (0.27 nM and 0.30 nM EC50, respectively, ). While no significant cytotoxicity was seen against the target-negative HCT-116 cells, both xi155D5 and hu155D5–2 ADCs showed specific killing of the target-positive HCT116-CA9 cells, with the humanized hu155D5–2 being slightly more potent than xi155D5 (0.57 nM versus 0.81 nM EC50, respectively) despite a slight drop in affinity during humanization (1.5-fold, data not shown). The irrelevant RESPECT ADC showed no significant activity against target-negative or target-positive cells.
Discussion
While the Vκ gene loci in human, mouse, and rat consist of several allotypes (families), rabbit Vκ genes comprise a single Vκ family.Citation30 When aligned for similarity to human Vκ families, the human IGKV1 family has closest homology to rabbit Vκ sequences and is therefore the human family of choice for humanization.Citation29,Citation31,Citation32 Of the 57 human IGKV1 alleles in the IMGT database, 51 contain a phenylalanine at position 83.Citation30 Our data find that the presence of a phenylalanine at this position results in increased aggregation levels and reduced conjugation to C80 in first-generation humanized RESPECT ADCs. Since any other amino acid except lysine decreased aggregation and increased conjugation of C80, use of a valine in alleles IGKV1/OR2–108*01, IGKV1–27*01, IGKV1–37*01, and IGKV1D-37*01 or an isoleucine in alleles IGKV1–33*01 and IGKV1D-33*01 at position 83 is required for optimal engineering of humanized RESPECT ADCs.
Previous reports have described engineering of cysteines into specific sites within antibodies to achieve desirable conjugation properties.Citation9,Citation17 In those studies, cysteines were engineered throughout the antibody, and the sites with moderate solvent exposure, high thiol reactivity, and minimum loss in antigen affinity were selected. Here, in contrast to engineering an optimized site via substitution of a native residue with a cysteine, we engineered residues adjacent to a native unpaired cysteine (C80) that in turn optimized thiol reactivity to linker-cytotoxins and reduced aggregation levels. Our data highlight the unpredictability of introducing residue-specific conjugation sites. The observation that phenylalanine reduces conjugation and increases aggregation would suggest that other bulky residues, such as tyrosine and tryptophan, would have a similar effect. However, this is not the case as the latter 2 residues showed no negative impact on conjugation or aggregation. Since lysine reduced conjugation to C80, one could hypothesize that arginine, due to its charged nature and similar size, would have a similar effect. However, our data did not support such a prediction, emphasizing the point that empirical observations were essential to determine what residues at position 83 affected conjugation at C80.
The increased aggregation and loss of conjugation induced by F83 may be due to increasing solvent exposure of C80. Gomez et al. demonstrated that solvent-exposed unpaired cysteines were conjugated with an additional LC, resulting in triple LC mAbs.Citation34,Citation35 It is possible the aggregation induced by decysteinylation causes LC-LC pairing between 2 different mAb molecules. This hypothesis is consistent with the lack of conjugation in F83 variants. Following our decysteinylation procedure, aggregates were not removed before incubation with maleimide-PEG2-biotin, therefore rendering LC-LC C80 multimers unavailable for conjugation to the maleimide substrate. Prior to electrospray ionization mass spectrometry (ESI-MS) analysis, all HC-LC and LC-LC interchain disulfide bonds were reduced with 10 mM dithiothreitol (DTT). Therefore, F83 may not directly influence formation of the maleimide-thiol bond at C80, but indirectly inhibit conjugation due to LC-LC interchain disulfide-mediated aggregation.
It has been observed that rabbit-derived mAbs exhibit high affinity and antigen specificity, as well as the ability to recognize epitopes conserved between humans and rodents.Citation36-38 Therefore, exploiting humanized ADCs derived from rabbit mAbs may hold potential value in terms of tumor targeting diversification. While the cysteine in position 80 has been typically removed during humanization of rabbit mAbs, we have leveraged its thiol-reactive properties to generate ADCs with high efficiency after proper downstream processing.Citation13 In addition, we identified optimal residue interactions in humanized variable sequences that endow second-generation humanized RESPECT ADCs with the desired biopharmaceutical properties.
Materials and methods
Gene synthesis and cloning
InFusion cloning
Humanized heavy and light variable domains of described previously rabbit mAbsCitation13 were codon optimized for expression in human cells and were synthesized by DNA 2.0. The variable domains were synthesized with a Kozak sequence and an Ig leader sequence, and included 15 base-pairs at the 5′ and 3′ ends homologous to the cloning site within the subcloning vector. Following excision from the DNA 2.0 vector, the fragments were subcloned into an expression plasmid containing a human gamma or kappa constant region using an InFusion HD cloning kit (Clontech). All clones were sequenced to confirm the presence and fidelity of the inserts.
QuikChange
Point mutations were made using Stratagene's QuikChange XL according to the manufacturer's protocol. All clones were sequenced to confirm the presence of the mutation.
Cell culture
Transfections and stable cell line generation
HEK293 cells were transfected using one of 2 methods. Transient transfections and stable cell lines of 293F cells were performed as previously reported.Citation39 Alternatively, Expi293 cells were transfected using ExpiFectamine according to the manufacturer's protocol (ThermoFisher). All cells were maintained in a humidified incubator at 37° C in 8% CO2 with shaking at 125 rpm.
MAb production
Antibody production from stable pools was performed by seeding cells at 0.6 to 1 × 106 cells/mL in 293 FreeStyle medium. Cells were incubated at 37° C, 8% CO2, shaking at 125 rpm. After the culture reached a density of 4 to 6 × 106 cells/mL, cultures were fed as previously reported.Citation39 Cultures were incubated for an additional 7 to 10 d. When the cell viability was less than 50%, cultures were centrifuged for 1 h at 8000 rpm in a Beckman JLA8.1000 rotor. Supernatants were then filtered through a 0.2 μm PES filter and stored at 4° C or −20° C until purification.
mAb purification
Antibody purification by protein A affinity chromatography
Using an ÄKTA Explorer (GE Healthcare), protein A columns (GE Healthcare) were equilibrated with 10 column volumes (CV) of 20 mM sodium phosphate, 10 mM EDTA, pH 7.2. Samples were then loaded followed by washing unbound material with 10 CV of equilibration buffer. Samples were eluted using 5 CV of 0.1 M glycine pH 2.9. Fractions containing antibody were pooled and dialyzed in Dulbecco's phosphate buffer (DPBS) using a MWCO 20K Slide-A-Lyzer (ThermoFisher).
Cysteine decysteinylation
Using an ÄKTA Explorer (GE Healthcare), protein A columns (GE Healthcare) were equilibrated with 10 column volumes (CV) of 20 mM sodium phosphate, 10 mM EDTA, pH 7.2. Samples were then loaded followed by washing unbound material with 10 CV of equilibration buffer. Columns were washed with 16 CV of 20 mM sodium phosphate, 10 mM EDTA, 5 mM cysteine, pH 7.2 at 0.5 mL/min for 16 h. Next, columns were washed with 60 CV of 20 mM Tris, pH 7.5 at 0.5 mL/min for 60 h, and samples were eluted using 5 CV of 0.1 M glycine pH 2.9. Fractions containing antibody were pooled and dialyzed in DPBS using a MWCO 20K Slide-A-Lyzer (ThermoFisher).
SEC-HPLC analysis
Antibody aggregation was analyzed by SEC-HPLC using an Agilent 1100. The mAb was diluted to 1 mg/mL in DPBS. Twenty μL of antibody was injected onto a TSKgel SuperSW guard column (4.6 mm × 3.5 cm, 4 μ), followed by a TSKgel SuperSW3000 column (4.6 mm × 30 cm, 4 μ), eluted from the column with 0.1 M PBS containing 0.15 M NaCl and 0.05% NaN3, at pH 7.4, at a flow rate of 0.3 mL/min for 20 min. All data were analyzed using Agilent ChemStation software.
Conjugation to C80
Decysteinylated antibody (5.0 mg/mL in 1x DPBS, 1 mM EDTA) was mixed with maleimido-PEG2-biotin (ThermoFisher) or maleimido-PEG2-AuF (Concortis Biosystems, Inc.) at a molar ratio of 1:4 (mAb:compound). Conjugation proceeded for 3.5 to 4 h at room temperature. For the maleimido-PEG2-AuF conjugation reaction, 25% propylene glycol was included. Conjugated antibody was purified using HiTrap desalting column(s) (GE Healthcare) with chromatography performed on an FPLC (GE Healthcare) using 1x DPBS as running buffer, to remove maleimido compounds and propylene glycol.
UPLC/ESI-MS analysis of malemide-biotin:mAb conjugation
Purified antibodies were diluted to 1 mg/mL in DPBS. Maleimide-PEG2-biotin (ThermoFisher) was dissolved in DPBS to yield a 20 mM stock solution, followed by dilution to 1 mM in DPBS. Maleimide-PEG2-biotin was added to 1 mL of decysteinylated mAb at a 5:1 conjugation ratio and incubated at room temperature with gentle rotation for 2 h. The reaction was desalted using a Zeba spin desalting column. The mAbs were then deglycosylated using PNGase F (NEB). G7 buffer (10 μL) and PNGase F (2 μL) were added to the mAb (90 μL). The reaction was incubated in a Discover microwave (CEM) for 2 cycles: 1) microwave power 10 W, 37 °C, 10 min, and then wait for 3–5 min; 2) microwave power 2 W, 37 °C, 10 min. A portion of the sample was reduced by adding DTT to a final concentration of 20 mM, followed by incubation at 60 °C for 3 min.
Samples were then analyzed using a Waters Acquity UPLC and Q-ToF Premier mass spectrometer. Samples (0.5–2 μg each) were injected onto a MassPrep micro desalting column at 65 °C, eluted from the column with a 5 min equilibration in 95% of mobile phase A, a 10 min gradient (5–90% B), and a 10 min re-equilibration in 95% of mobile phase A, at 0.05 mL/min. Mobile phase A was 0.1% formic acid in water. Mobile phase B was 0.1% formic acid in acetonitrile. The Q-ToF mass spectrometer was run in positive ion, V-mode with detection in the range of 500–4000 m/z. The source parameters were as follows: capillary voltage, 2.25 kV (intact antibody)-2.50 kV (reduced antibody); sampling cone voltage, 65.0 V (intact antibody) or 50.0 V (reduced antibody); source temperature, 100 °C; desolvation temperature, 250 °C; desolvation gas flow, 550 L/h. The protein peak was deconvoluted using the MassLynx MaxEnt 1 function.
Antibody homology modeling
Homology models of xi155D5 and hu155D5–1 were generated using Biovia Discovery Studio. The most homologous published crystal structures were identified using the BLAST search function. 2X7L was the template for xi155D5 and 1DFB, 3DVG, and 3NCJ were used as templates for hu155D5–1. Homology models were built using the “Create Homology Models” function, followed by energy minimizations.
BIAcore analysis of mAb:antigen affinity
Antibody concentrations were adjusted to generate 30–40 RU signal when bound to the antigen. Humanized mAbs purified by standard protein A affinity chromatography or by the decysteinylation method were injected over an anti-human IgG sensor for 1 min at a flow rate of 10 μL/min. The sensor surface was washed by injecting HBS-P buffer for 1 min at a flow rate of 50 μL/min. To record the antigen association to the captured mAb, a series of increasing concentrations of antigen was injected for 60 sec at a flow rate of 50 μL/min. The dissociation of antigen was monitored for 30 min at the same flow rate. The sensor surface was regenerated by injecting 3 M MgCl2 for 1 min and then 30 sec at a flow rate of 30 μL/min. Sensograms were analyzed with BIAcore T100 Evaluation Software using a 1:1 binding model.
In vitro cytotoxicity assay
HCT116 cells were stably transfected with a pEF-based plasmid encoding human CA9 to generate HCT116-CA9 constitutively expressing human CA9 on the cell surface. HCT116 or HCT116-CA9 cells were seeded in 96-well plates and incubated overnight at 37° C, 5% CO2. Serial dilutions of ADCs were added to the plated cells. After incubation for 3 d at 37° C, 5% CO2, cells were stained with 0.2% crystal violet as follows. The medium was removed and wells were washed with 200 µL of DPBS. Fifty µL of 0.2% crystal violet was added to each well and incubated at room temperature for 15 min. The crystal violet stain was removed and wells were washed 7 times with tap water and air-dried for 30 min. The stained cells were dissolved in 200 µL of 1% SDS solution for 1 h. The plates were then mixed on a vortex mixer for 10 sec. The absorbance at 570 nm was analyzed.
Disclosure of potential conflicts of Interest
All authors are employees of Morphotek, Inc.
Supplementary_Material.zip
Download Zip (79.2 KB)References
- Sochaj AM, Swiderska KW, Otlewski J. Current methods for the synthesis of homogeneous antibody-drug conjugates. Biotechnol Adv 2015; 33(6 Pt 1):775-84; PMID:25981886; https://doi.org/10.1016/j.biotechadv.2015.05.001
- Bakhtiar R. Antibody drug conjugates. Biotechnol Lett 2016; 38(10):1655-64; PMID:27334710; https://doi.org/10.1007/s10529-016-2160-x
- Deonarain MP, Yahioglu G, Stamati I, Marklew J. Emerging formats for next-generation antibody drug conjugates. Expert Opin Drug Discov 2015; 10(5):463-81; PMID:25797303; https://doi.org/10.1517/17460441.2015.1025049
- de Goeij BE, Lambert, JM. New developments for antibody-drug conjugate-based therapeutic approaches. Curr Opin Immunol 2016; 40:14-23; PMID:26963132; https://doi.org/10.1016/j.coi.2016.02.008
- Hu QY, Berti F, Adamo R. Towards the next generation of biomedicines by site-selective conjugation. Chem Soc Rev 2016; 45(6):1691-719; PMID:26796469; https://doi.org/10.1039/C4CS00388H
- Zimmerman ES, Heibeck TH, Gill A, Li X, Murray CJ, Madlansacay MR, Tran C, Uter NT, Yin G, Rivers PJ, et al. Production of site-specific antibody-drug conjugates using optimized non-natural amino acids in a cell-free expression system. Bioconjug Chem 2014; 25(2):351-61; PMID:24437342; https://doi.org/10.1021/bc400490z
- Jeger S, Zimmermann K, Blanc A, Grunberg J, Honer M, Hunziker P, Struthers H, Schibli R. Site-specific and stoichiometric modification of antibodies by bacterial transglutaminase. Angew Chem Int Ed Engl 2010; 49(51):9995-7; PMID:21110357; https://doi.org/10.1002/anie.201004243
- Strop P, Liu SH, Dorywalska M, Delaria K, Dushin RG, Tran TT, Ho WH, Farias S, Casas MG, Abdiche Y et al. Location matters: site of conjugation modulates stability and pharmacokinetics of antibody drug conjugates. Chem Biol 2013; 20(2):161-7; PMID:23438745; https://doi.org/10.1016/j.chembiol.2013.01.010
- Junutula JR, Raab H, Clark S, Bhakta S, Leipold DD, Weir S, Chen Y, Simpson M, Tsai SP, Dennis MS et al. Site-specific conjugation of a cytotoxic drug to an antibody improves the therapeutic index. Nat Biotechnol 2008; 26(8):925-32; PMID:18641636; https://doi.org/10.1038/nbt.1480
- Kabat EA, Wu TT, Perry H, Foeller C. Sequences of Proteins of Immunological Interest. Bethesda (MD): National Institute of Health Publication No. 91-3242; 1991
- Edelman GM, Cunningham BA, Gall WE, Gottlieb PD, Rutishauser U, Waxdal MJ. The covalent structure of an entire gammaG immunoglobulin molecule. Proc Natl Acad Sci U S A 1969; 63(1):78-85; PMID:5257969; https://doi.org/10.1073/pnas.63.1.78
- Strosberg AD, Margolies MN, Haber E. The interdomain disulfide bond of a homogeneous rabbit pneumococcal antibody light chain. J Immunol 1975; 115(5):1422-24; PMID:240892
- Albone EF, Spidel JL, Cheng X, Park YC, Jacob S, Milinichik AZ, Vassen B, Butler J, Kline JB, Grasso L. Generation of therapeutic immunoconjugates via Residue-Specific Conjugation Technology (RESPECT) utilizing a native cysteine in the light chain framework of Oryctolagus cuniculus. In Press. Cancer Biol Ther 2017; PMID:28394698; https://doi.org/10.1080/15384047.2017.1312232
- Shopes B. A genetically engineered human IgG mutant with enhanced cytolytic activity. J Immunol 1992; 148(9):2918-22; PMID:1573276
- Shen BQ, Xu K, Liu L, Raab H, Bhakta S, Kenrick M, Parsons-Reponte KL, Tien J, Yu SF, Mai E, et al. Conjugation site modulates the in vivo stability and therapeutic activity of antibody-drug conjugates. Nat Biotechnol 2012; 30(2):184-89; PMID:22267010; https://doi.org/10.1038/nbt.2108
- Junutula JR, Bhakta S, Raab H, Ervin KE, Eigenbrot C, Vandlen, R, Scheller RH, Lowman HB. Rapid identification of reactive cysteine residues for site-specific labeling of antibody-Fabs. J Immunol Methods 2008; 332(1-2):41-52; PMID:18230399; https://doi.org/10.1016/j.jim.2007.12.011
- Shinmi D, Taguchi E, Iwano, J, Yamaguchi, T, Masuda, K, Enokizono, J, Shiraishi, Y. One-Step Conjugation Method for Site-Specific Antibody-Drug Conjugates through Reactive Cysteine-Engineered Antibodies. Bioconjug Chem 2016; 27(5):1324-31; PMID:27074832; https://doi.org/10.1021/acs.bioconjchem.6b00133
- Igawa T, Tsunoda H, Kuramochi T, Sampei Z, Ishii S, Hattori K. Engineering the variable region of therapeutic IgG antibodies. MAbs 2011; 3(3):243-52; PMID:21406966; https://doi.org/10.4161/mabs.3.3.15234
- Hwang WY, Almagro JC, Buss TN, Tan P, Foote J. Use of human germline genes in a CDR homology-based approach to antibody humanization. Methods 2005; 36(1):35-42; PMID:15848073; https://doi.org/10.1016/j.ymeth.2005.01.004
- Foote J, Winter G. Antibody framework residues affecting the conformation of the hypervariable loops. J Mol Biol 1992; 224(2):487-99; PMID:1560463; https://doi.org/10.1016/0022-2836(92)91010-M
- Almagro JC, Fransson J. Humanization of antibodies. Front Biosci 2008; 13:1619-33; PMID:17981654
- Kim JH, Hong HJ. Humanization by CDR grafting and specificity-determining residue grafting. Methods Mol Biol 2012; 90:7237-245; PMID:22907355; https://doi.org/10.1007/978-1-61779-974-7_13
- Safdari Y, Farajnia S, Asgharzadeh M, Khalili M. Antibody humanization methods - a review and update. Biotechnol Genet Eng Rev 2013; 29:175-86; PMID:24568279; https://doi.org/10.1080/02648725.2013.801235
- Zhang YF, Ho M. Humanization of rabbit monoclonal antibodies via grafting combined Kabat/IMGT/Paratome complementarity-determining regions: Rationale and examples. MAbs 2017; 9(3):419-429. 1-11; PMID:28165915; https://doi.org/10.1080/19420862.2017.1289302
- Zhang YF, Phung Y, Gao W, Kawa S, Hassan R, Pastan I, Ho M. New high affinity monoclonal antibodies recognize non-overlapping epitopes on mesothelin for monitoring and treating mesothelioma. Sci Rep 2015; 5:9928; PMID:25996440; https://doi.org/10.1038/srep09928
- Yu Y, Lee P, Ke Y, Zhang Y, Yu Q, Lee J, Li M, Song J, Chen J, Dai J, et al. A humanized anti-VEGF rabbit monoclonal antibody inhibits angiogenesis and blocks tumor growth in xenograft models. PLoS One 2010; 5(2):e9072; PMID:20140208; https://doi.org/10.1371/journal.pone.0009072
- Popkov M, Mage RG, Alexander CB, Thundivalappil S, Barbas CF, III, Rader C. Rabbit immune repertoires as sources for therapeutic monoclonal antibodies: the impact of kappa allotype-correlated variation in cysteine content on antibody libraries selected by phage display. J Mol Biol 2003; 325(2):325-35; PMID:12488098; https://doi.org/10.1016/S0022-2836(02)01232-9
- Steinberger P, Sutton JK, Rader C, Elia M, Barbas CF, III. Generation and characterization of a recombinant human CCR5-specific antibody. A phage display approach for rabbit antibody humanization. J Biol Chem 2000; 275(46):36073-8; PMID:10969070; https://doi.org/10.1074/jbc.M002765200
- Borras L, Gunde T, Tietz J, Bauer U, Hulmann-Cottier V, Grimshaw JP, Urech DM. Generic approach for the generation of stable humanized single-chain Fv fragments from rabbit monoclonal antibodies. J Biol Chem 2010; 285(12):9054-66; PMID:20056614; https://doi.org/10.1074/jbc.M109.072876
- Lefranc MP. IMGT, the international ImMunoGeneTics database. Nucleic Acids Res 2001; 29(1):207-209; PMID:11125093; https://doi.org/10.1093/nar/29.1.207
- Rader C, Ritter G, Nathan S, Elia M, Gout I, Jungbluth AA, Cohen LS, Welt S, Old LJ, Barbas CF, III. The rabbit antibody repertoire as a novel source for the generation of therapeutic human antibodies. J Biol Chem 2000; 275(18):13668-76; PMID:10788485; https://doi.org/10.1074/jbc.275.18.13668
- Kodangattil S, Huard C, Ross C, Li J, Gao H, Mascioni A, Hodawadekar S, Naik S, Min-debartolo J, Visintin A, et al. The functional repertoire of rabbit antibodies and antibody discovery via next-generation sequencing. MAbs 2014; 6(3):628-36; PMID:24481222; https://doi.org/10.4161/mabs.28059
- Ye J, Ma N, Madden TL, Ostell JM. IgBLAST: an immunoglobulin variable domain sequence analysis tool. Nucleic Acids Res 2013; 41(Web Server issue):W34-W40; PMID:23671333; https://doi.org/10.1093/nar/gkt382
- Gomez N, Vinson AR, Ouyang J, Nguyen MD, Chen XN, Sharma VK, Yuk IH. Triple light chain antibodies: factors that influence its formation in cell culture. Biotechnol Bioeng 2010; 105(4):748-60; PMID:19845001
- Gomez N, Ouyang J, Nguyen MD, Vinson AR, Lin AA, Yuk IH. Effect of temperature, pH, dissolved oxygen, and hydrolysate on the formation of triple light chain antibodies in cell culture. Biotechnol Prog 2010; 26(5):1438-45; PMID:20568280; https://doi.org/10.1002/btpr.465
- Rossi S, Laurino L, Furlanetto A, Chinellato S, Orvieto E, Canal F, Facchetti F, Dei Tos AP. Rabbit monoclonal antibodies: a comparative study between a novel category of immunoreagents and the corresponding mouse monoclonal antibodies. Am J Clin Pathol 2005; 124(2):295-302; PMID:16040303; https://doi.org/10.1309/NR8HN08GDPVEMU08
- Rocha R, Nunes C, Rocha G, Oliveira F, Sanches F, Gobbi H. Rabbit monoclonal antibodies show higher sensitivity than mouse monoclonals for estrogen and progesterone receptor evaluation in breast cancer by immunohistochemistry. Pathol Res Pract 2008; 204(9):655-62; PMID:18565685; https://doi.org/10.1016/j.prp.2008.03.010
- Weller A, Meek J, Adamson ED. Preparation and properties of monoclonal and polyclonal antibodies to mouse epidermal growth factor (EGF) receptors: evidence for cryptic EGF receptors in embryonal carcinoma cells. Development 1987; 100(2):351-63; PMID:3308402
- Spidel JL, Vaessen B, Chan YY, Grasso L, Kline JB. Rapid high-throughput cloning and stable expression of antibodies in HEK293 cells. J Immunol Methods 2016; 439:50-8; PMID:27677581; https://doi.org/10.1016/j.jim.2016.09.007