ABSTRACT
To rapidly find “best-in-class” antibody therapeutics, it has become essential to develop high throughput (HTP) processes that allow rapid assessment of antibodies for functional and molecular properties. Consequently, it is critical to have access to sufficient amounts of high quality antibody, to carry out accurate and quantitative characterization. We have developed automated workflows using liquid handling systems to conduct affinity-based purification either in batch or tip column mode. Here, we demonstrate the capability to purify >2000 antibodies per day from microscale (1 mL) cultures. Our optimized, automated process for human IgG1 purification using MabSelect SuRe resin achieves ∼70% recovery over a wide range of antibody loads, up to 500 µg. This HTP process works well for hybridoma-derived antibodies that can be purified by MabSelect SuRe resin. For rat IgG2a, which is often encountered in hybridoma cultures and is challenging to purify via an HTP process, we established automated purification with GammaBind Plus resin. Using these HTP purification processes, we can efficiently recover sufficient amounts of antibodies from mammalian transient or hybridoma cultures with quality comparable to conventional column purification.
Introduction
Over the past two decades, monoclonal antibodies (mAbs) have revolutionized the treatment of cancer, as well as immune-mediated, infectious, neurological and respiratory diseases.Citation1 By 2020, the number of marketed therapeutic mAbs is projected to grow to ∼70 molecules.Citation2 Faced with more challenging and complex therapeutic targets, it has become necessary to embark on large antibody discovery campaigns to find the rare antibodies that possess desirable functional and molecular characteristics for therapeutic development. In recent years, advances in antibody discovery technologies in hybridoma, synthetic display libraries and B-cell cloning, coupled with innovations in next generation DNA sequencing and high content analysis, have enabled the generation of thousands of mAbs in antibody discovery campaigns. By using HTP processes to generate large numbers of antibodies within a fast turnaround time for screening and selection, we can eliminate a bottleneck for industrial scale antibody drug discovery. To make such large numbers of antibodies available for assays, additional HTP processes are required to support expressionCitation3,Citation4 and purification. In comparison to ion exchange, reversed phase, size exclusion and other chromatography methods, Protein A- or Protein G- affinity chromatography provides fast one-step purification of antibodies to generate material suitable for screening and primary characterization.Citation5 With the development of many well-tested commercial resins and liquid handling platforms, it is feasible to develop HTP purification workflows that provide fast turnaround time with minimal manual intervention. Previously published reviewsCitation6 have discussed several microscale approaches for HTP chromatography development that included three main chromatography approaches: 1) pipette tip columns, in which resin is packed between frits; 2) batch mode, where small amount of resin slurry is added to supernatant; and 3) miniaturized column, a scaled-down version of a traditional column.Citation7 Spooner et alCitation8 discussed similar chromatography approaches to HTP purification, and indicated batch mode and tip column chromatography were favorable for automation and HTP. However, challenges remain for establishing reliable HTP purification processes due to automation complexity and cost of goods.
During early stages of antibody screening, there is a need for rapid purification of thousands of antibody variants with a wide range of expression titers. Our collaborators previously demonstrated miniaturized transient expression of a human IgG1 antibody at high titers (>360 µg per mL of mammalian cell culture).Citation4 The miniaturization enabled reliable automated HTP production with short turnaround time and reduced costs in consumables per sample. In the previous work, a tip column purification process was used, which could recover approximately 78% of human IgG1 expressed at high titers in 1 mL human embryonic kidney 293 (HEK293) transient cultures. In this work, we provide details of the processes that made it possible to achieve a throughput of ∼2000 antibodies per day using automated HTP antibody purification workflows. We describe the steps taken to identify optimized purification parameters and implementation of full automation using a tip column purification process, with consistent recovery for wide range of expression titers across multiple reuses of the tip columns. Since the tip column mode has less flexibility if a different type of resin or different amount of resin is needed and lower throughput for culture volumes between 1 – 4 mL, we developed a batch mode HTP purification workflow. Using these processes, we demonstrate rapid screening of resins and purification parameters that can maximize recovery not only for human IgGs, but also many other IgG species, particularly for rat IgG2a, which was difficult to purify previously and often encountered in large rat hybridoma antibody discovery campaigns. We also provide key analyses illustrating similarity in the quality of IgGs purified either by the HTP purification workflows described here or by commonly used conventional column-based purification workflows. Finally, to meet the need to provide HTP purification of large antibody collections, we describe our adaption of these workflows to automation using Tecan EVO 200 and Dynamic Devices Lynx LM1200 liquid handling systems. Combined, these HTP purification workflow platforms enhance all aspects of antibody discovery and engineering by providing high quality purified antibodies for functional screening at a daily capacity of at least 20 plates of 96 samples (up to 4× 1 mL replicate cultures per source plate).
Results
Tip column mode and batch mode purification on liquid handling systems
We initially developed tip column mode purification using a customized Tecan Freedom EVO 200 liquid handling workstation. An MCA384 head was converted into an MCA96 head using an Extended Volume Adaptor where 4 channels were merged into one with increased volume capacity of 500 µl (4× 125 µL) per channel. 500 µL tips were used for packing the tip columns because they enabled faster transfer of larger volumes. Tips with ≤200 µL capacity were typically used for accurate transfer of smaller volumes. The 8-Channel LiHa was connected to 5 mL syringes and used wide-bore fixed tips for speedier dispensing of large amount of wash buffer or resins. A six-port pump module and a wash station were also installed for fast washing of the tips. A Hettich 4 bucket centrifuge was installed underneath the platform with a cut-out on the deck to make the centrifuge accessible to the RoMa arm.
The deck layout () on the Tecan EVO200 was configured such that 36 locations for SBS format plates were directly accessible to the MCA96 head. Stacking hotels were installed on the back and left side of the deck to hold an additional 32 plates. The hotels offered better economy and reliability for adding extra capacity than stackers or carousels. Scripts were written to follow the process scheme shown in . For this workflow, a capture plate, a flow through plate and two wash plates were transferred to the deck from hotels before each purification and transferred back to hotel after purification. No other plate movement was programmed in the scripts so that automated execution could be more reliable. This minimized the possibility of some rare failures that were observed while testing protocols, which happened during movement of culture plates or elution plates and could cause total loss of samples. When all the sites and hotels were utilized, up to 8 deep-well culture plates could be purified consecutively by tip column mode, without manual intervention. It would typically take approximately 12 hours to complete 8 consecutive tip column purifications, which was often set up for overnight execution.
Figure 1. (a) Tecan EVO 200 deck layout, and (b) automation process scheme for 1 mL tip column mode purification of 8 consecutive plates in a continuous run. In this layout, there are 8 boxes of regular 200 µL tips on the right side of the deck and 1 box of 500 µL Tip Columns (Tip Clmn, rectangle with dark border). Abbreviations for labware labels: P1 – P8 (plate # 1 – 8), Capt. (Capture), F.T. (Flow through), CIP (Cleaning in place buffer), Cult. (Culture Media), Clmn. (Column), Equili. (Equilibration).
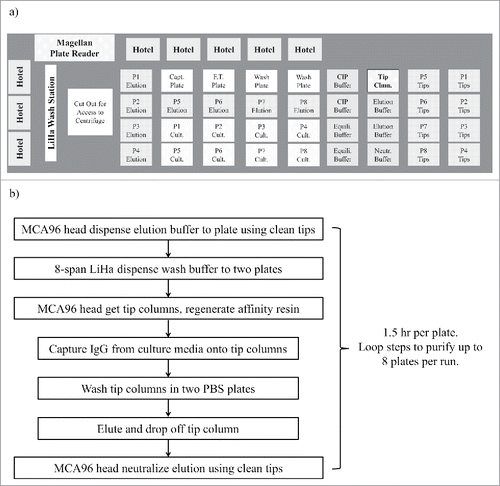
The throughput of tip column mode purification for larger sample volume (> 2 mL) would be significantly less as it would take more time to capture. Therefore, we developed a batch mode purification process, that offered enhanced flexibility for adding different types or amounts of resin to the supernatant to be purified and provided better throughput for larger volume. For batch mode purification, we used a custom-built Dynamic Devices Lynx LM1200 for resin addition, culture harvest transfer and vacuum washing filter plates. Its SVP-96 tip head was capable of holding 1250 µL wide-bore tips and could dispense most commercially manufactured resins without tip blockage. Although the 8-channel fixed tip setup on Tecan EVO200 could also be used for resin transfer, its throughput was much slower than the SVP-96 tip head on the LM1200 instrument. The LM1200 deck was configured with 42 sites for SBS format plates, enough for setting up 4 filter plates to be used for batch mode purification in a single run (). This set-up gave us the flexibility of combining up to 4 plates of culture (1 mL per well) into one filter plate, and allowed up to 4 mL of culture for each clone to be purified in this process. We used the centrifugation capabilities of the Tecan EVO 200 to carry out the final steps of batch mode filtration-eluting the filter plates, with up to 4 filter plates being be processed in one run. By using both the LM1200 and EVO 200, all the transfer and elution steps take approximately 1.5 hours for batch mode purification of 4 filter plates (). When all capture plates were incubated with purification resin at the same time (overnight), a total of 16 plates could be batch purified in a typical 8-hour working day.
Figure 2. (a) Dynamic Device Lynx 1200 deck layout, and (b) automation process scheme for 1–4 mL batch mode purification of 4 parallel plates using 4 filter plates. In this layout, there are 5 boxes of regular 1250 µL tips on the right side of the deck, 1 box for transferring PBS, 4 boxes for transferring culture media (P1 - P4). Abbreviations for labware labels: P1 – P4 (plate # 1 – 4), Reserv. (Reservoir), Vac. (Vacuum), Cult. (Culture Media), Capt. (Capture).
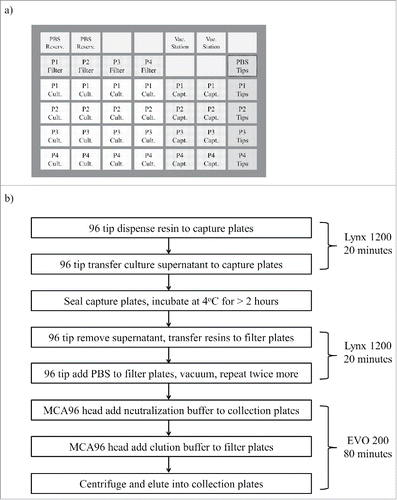
Culture treatment for HTP purification
Almost all of our purification workflows started with hybridoma or transient cell cultures. Therefore, removal of the mammalian cells before capture was necessary to prevent blockage/clogging. We initially tried filtering to remove cells using deep-well filter plates with pore sizes of 1 – 10 microns, but these filter plates cost significantly more than other materials used in the purification workflow. In addition, these filter wells were often clogged by cell debris. Instead, we found that centrifugation (1000 g for 5 min) provided a faster and inexpensive alternative for cell separation. The centrifugation was normally done in a large centrifuge (Sorvall HT6, Thermo Fisher Scientific) with capacity of 12 deep-well blocks per run (manual loading/unloading) or in the Hettich centrifuge with 4 blocks per run (automatically managed by the Tecan EVO 200). After centrifugation, the supernatant was transferred to capture plates by liquid handling system with a fixed aspiration position set approximately 2 mm above the cell pellets. For the tip column mode purification, supernatant transfer into empty deep-well blocks was done by 96 tip head with tip columns mounted. This step was immediately followed by slowly pipetting the supernatant up and down in order to capture antibody onto the resin packed in the tip columns. For batch mode purification, supernatant transfer was done by 96 tip head with regular tips, into capture plates to which purification resin was already added.
Optimization of HTP affinity capture for human IgG1
For our HTP purification workflows, we targeted a desired recovery greater than 60% when medium to high amounts of IgG were generated in cell culture. In our transient production workflow, human IgG were generated from HTP culture in 96 deep-well blocks with a final volume about 1 mL per well. Some of our high expression constructs could produce approximately 360 µg human IgG per mL of culture.Citation4 We chose to use a high amount, 500 µg human IgG1 spiked in 1 mL phosphate-buffered saline (PBS) or blank culture media, for most of the purification process optimization experiments. The optimized workflows were also tested with purification of lower amounts of IgG to ensure satisfactory recovery was achieved.
Affinity purification of antibodies using an engineered Protein A resin, MabSelect SuRe resin (GE Healthcare Life Sciences), is extensively validated and widely implemented in commercial manufacturing applications.Citation9 The binding capacity in HTP batch mode or tip column mode were determined in saturating conditions by loading 2 mg antibody onto 20 µL of resin. Experimentally, we determined that the resin had a “static” binding capacity of 62±3 µg human IgG1 per µL of resin in batch mode purification with overnight incubation (). We decided to use 20 µL of resin for each mL of culture harvest as it would capture more than 1000 µg of human IgG1 at capacity, which was a small amount of resin, but sufficient to capture IgG produced during normal transient mammalian expression.
Figure 3. Optimizing automated HTP purification in tip columns packed with MabSelect SuRe. (a) 2 mg human IgG1 in 1 mL PBS was loaded over resin in each experiment. Concentrations before and after capture were used to calculate the amount that was bound on the resin (n = 4). Human IgG1 binding capacity was measured for batch mode capture for 16 hours at 4˚C with 20 µL resin (62±3 µg) and tip column capture by pipetting total of 2, 4, 6, 8, and 9 cycles (37±1, 46±1, 52±1, 54±1, and 55±2 µg). (b) resin-bound IgG was eluted twice from tip column with 160 µL of 50 mM phosphoric acid at pH 3.0, 3.2, 3.4, and 3.6 (n = 4 for each pH tested). Buffers at pH3.4 or above were inefficient in eluting IgG from the tip column. (c) eluted IgG concentration, and (d) IgG recovery after treating tip columns with different volumes (100 – 200 µL) of elution buffer (50 mM phosphoric acid pH3.0 buffer). Each 20 µL MabSelect SuRe tip column was loaded with 500 µg IgG in (b – d).
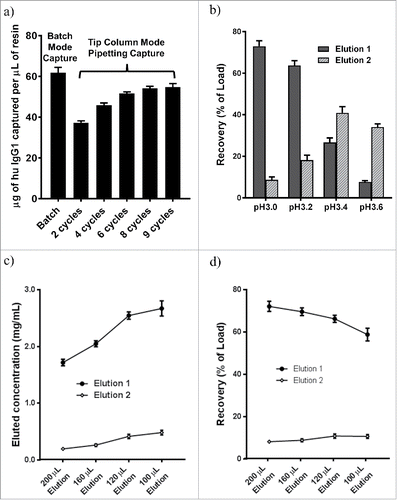
For tip column purification, we used 20 µL of packed resin and tested various combinations of pipetting (aspiration-dispensing) cycles and speeds, which gave different “dynamic” binding capacity. We found that multiple cycles of faster pipetting speed (500 µL per min, i.e., <0.04 min tip column residence time per cycle) gave sufficient antibody capture within a short total capture time. In tip column mode, more pipetting cycles increased the binding capacity of tip columns, but the change was smaller after 6 cycles of pipetting. When the binding capacity was measured with 6, 8 or 9 pipetting cycles at a speed of 500 µL per minute, 52±1, 54±1or 55±2 µg human IgG1, respectively, were captured per µL of resin (). The tip column capture capacity was slightly lower than the batch mode binding capacity because the resin in tip columns had only 30 to 45 min contact time (6 to 9 pipetting cycles) to the loading material vs a 16 hr contact time in batch mode. We chose to use a total of 8 cycles, which included 1 cycle for transfer from culture harvest plate and 1 cycle for transfer to a flow-through plate, with all pipetting steps carried out at 500 µL per min, resulting in efficient capture in a total capture duration of approximately 40 min for 1 mL of culture media.
Optimization of HTP elution conditions for human IgG1
A typical purification protocol for human IgG1 from MabSelect SuRe tip column was reported previously,Citation4 and other buffers with pH 3.0 or lower have been used for elution of antibodies from Protein A based resins.Citation5 In general, a less acidic buffer is preferred for antibody elution due to the propensity for antibody instability and the need for neutralization in acidic buffers. We evaluated elution of human IgGs (excluding IgG3) with 50 mM phosphoric acid at pH 3.0, pH 3.2, pH 3.4, and pH 3.6 and found that pH 3.0 always gave satisfactory elution (73±3%), while buffer with pH 3.4 and higher gave lower yields and inconsistent elution (). Other buffers such as 10 mM citrate, 100 mM glycine, 50 mM acetic acid, at pH 3.0 or lower could effectively elute IgG bound on MabSelect SuRe resin. For our routine microscale purification of human IgGs, we decided to use 50 mM phosphoric acid pH 3.0 for elution and neutralized with 1/15 volume of 200 mM sodium phosphate, 300 mM sodium chloride, pH 11.0, resulting in a final formulation with pH ∼6.0. This elution-neutralization scheme avoided some buffers such as glycine or Tris which were often incompatible with some downstream assays and screening applications.
Higher final IgG concentration in the eluate was desirable for downstream applications, which could be obtained via a smaller elution volume. We compared IgG product concentration and recovery vs elution volume with range of 200 to 100 µL, equivalent of 10x to 5x of bed volume. Recovered IgG concentration increased from 1.7 mg/ml to 2.7 mg/ml, an ∼ 56% increase, when elution volume was decreased from 200 µL to 100 µL for purifying 500 µg human IgG (). However, the total recovery decreased from 72% to 59% when elution volume was decreased from 200 µL to 100 µL (). Some of the loss in recovery by a smaller elution volume was partially recovered in the second elution, which contained <20% of total recovery and would not be collected in order to keep the eluted antibody at a high concentration in a small volume. We chose to elute tip columns containing 20 µL MabSelect SuRe resin with 160 µL elution buffer to achieve a reasonable balance between optimal recovery and product concentration. Proportionally larger elution volume was used for more resin.
For batch mode purification of human IgG, we used 20 µL MabSelect SuRe resin per mL of culture harvest. During batch mode purification, some resin could be left in deep-well blocks after transferring from deep-well blocks to filter plates, causing lower recovery efficiency. To minimize this resin loss, we added 0.5 mL PBS to each well of the deep-well blocks after first resin transfer, washed the wells and transferred again to the filter plates. With optimized automated pipetting and the extra resin transfer, we observed that batch mode purification with 20 µL or more MabSelect SuRe resin showed similar recovery efficiency as the tip column mode when same elution conditions were used. However, residual amounts of resin would not be transferred due to sticking to tips or deep-well blocks, which could be a significant resin loss for smaller amounts of resin (< 20 µL) and could cause lower purification recovery. Extra washes and pipetting steps optimized for more complete resin resuspension/transfer would maximize batch mode purification recovery, particularly when smaller amount of resin was used. For antibody purification that needed larger yields and higher product concentration, we typically used replicated expressions of 1 mL culture and, using the batch mode purification to save time, purified the combined culture harvests into a smaller final volume (such as 120 µL elution per 20 µL resin).
Quality of antibody purified by HTP process
We compared the product quality of different modes of purification of the same human IgG expression culture using analytical size exclusion chromatography (SEC), sodium dodecyl sulfate polyacrylamide gel electrophoresis (SDS-PAGE) and liquid chromatography-mass spectrometry (LC-MS), three common methods to show IgG aggregation, degradation, post-translational modification or contaminants. Conventional column, HTP tip column and batch mode showed the same monomer content (94.2±0.8%, 93.8±0.9%, 94.2±0.7%) and the same aggregation peaks by analytical SEC (, and ). The same product quality was observed for these three purification modes by SDS-PAGE analysis. LC-MS identified the same light chain and heavy chain mass peaks with expected glycosylation pattern, and no degradation or contaminants were detected ( and ). These analyses confirmed that our two modes of HTP microscale purification generated antibodies with similar quality as conventional column mode purification carried out using an AKTA FPLC system.
Figure 4. Comparing the quality of a human IgG1 purified by (i) conventional column, (ii) automated tip column and (iii) batch mode. (a) Analytical SEC profiles of purified IgG1. (b) Reduced SDS-PAGE, M: Mark 12 standard. (c) Non-reduced SDS-PAGE. (d) LC-MS intact mass analysis of light chain. (e) LC-MS intact mass analysis of heavy chain. No significant difference in purity or measured masses was observed in purified IgG from automated processes compared to a conventional column process. Glycosylation variants abbreviations: Man5 (Man5GlcNAc2), G0F (GlcNAc2Man3GlcNAc2), G1F (GalGlcNAc2Man3(Fuc)GlcNAc2), where: Man (mannose), GlcNAc (N-acetylglucosamine), Gal (Galactose), Fuc (Fucose).
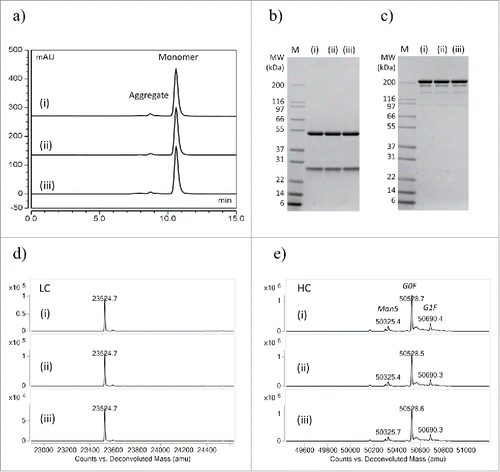
Table 1. SEC analysis results of purified products from different purification modes.
Optimization of rat IgG2a HTP purification
Rat IgG2a does not bind to MabSelect SuRe or other protein A-based resins, therefore protein G-based resin was normally used for purification of rat IgG2a. Tip columns packed with same amount of resin (20 µL) allowed us to compare different resins accurately at microscale level. We compared purification of rat IgG2a by using recombinant protein G resin or GammaBind Plus resin (resin coupled with affinity improved protein G, GE Healthcare) under various capture conditions (). The capture efficiency of rat IgG2a using protein G is significantly affected by pH or salt condition changes and had a low binding capacity under physiological (pH 7.2) buffer conditions ( and ). Efficient IgG recovery often requires addition of salt and pH adjustment to capture and wash buffer. On the other hand, GammaBind Plus resin showed overall better binding capacity to rat IgG2a, and was also more tolerant to pH or salt condition changes (). Since purification using GammaBind Plus obviated the need to pretreat culture supernatants, it provided an opportunity for a simpler purification workflow than with Protein G and other resins. GammaBind Plus resin was chosen for purification of all our rat hybridoma cultures where isotypes were often unknown and the IgG2a isotype was often encountered. The binding of all rat IgG to GammaBind Plus appeared to be stronger than to protein G. We also found that washing the GammaBind Plus resin with a moderately acidic buffer of low ionic strength prior to elution improved recoveries significantly. For example, washing 20 µL GammaBind Plus resin tip column with 2 mM sodium phosphate, 30 mM sodium chloride, pH 5.0 and subsequent elution with 190 µL of 10 mM citrate, pH 2.9 yielded better elution (51±3% recovery) than washing with 1x PBS (33±1% recovery) (First elution, ). For rat hybridoma cell lines cultured in 96 deep-well blocks, a yield of ∼50–100 µg IgG was expected per mL of culture. We found that 20 µL GammaBind Plus resin was sufficient to capture this amount as the “static” binding capacity of GammaBind Plus resin for rat IgG2a when evaluated in batch mode was measured at approximately 15 µg/ µL resin and higher capacity for other IgG isotypes (unpublished data).
Table 2. Comparison of rat IgG2a capture on resin under different loading conditions.
Figure 5. Evaluating sensitivity of GammaBind Plus (panel a) and Protein G (panel b) to sodium chloride (NaCl) concentration and pH for binding rat IgG2a. Data from were plotted using JMP11 software (SAS, Cary, NC). (c) Recovery efficiency with different wash condition: 150 µg rat IgG2a was loaded onto 20 µL GammaBind Plus resin tip columns, then washed with 1x PBS twice, followed by washing with low salt pH 5 (2 mM sodium phosphate, 30 mM sodium chloride) buffer or 1x PBS, finally eluted thrice with 190 µL of 10 mM citrate pH2.9 buffer, resulting in 51 ± 3% or 33 ± 1% recoveries respectively in elution 1.
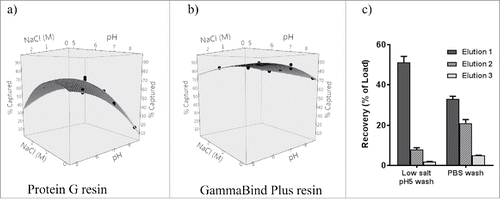
Reusability of tip columns after multiple regenerations and batch mode resin recovery
MabSelect SuRe resin can be regenerated up to hundred times in conventional columns after cleaning in place (CIP) with up to 0.5 M NaOH or 6 M guanidine according to manufacturer's instructions. We used two SBS format reservoirs containing 250 mL of 0.2 M NaOH for the tip column's CIP on liquid handling systems. Since tip columns were the most expensive consumables in the purification workflow, it was desirable for the tip columns to have consistent performance over multiple uses. We investigated the impact of multiple tip column uses on antibody recovery, when each cycle of tip column use was followed by a CIP cycle. For purifying high amounts (500 µg) of IgG, we only observed a small (∼4%) drop in recovery, from 73±1% following first use to 69±2% following 22nd use (). For purifying lower amounts of IgG (100 µg), the decrease in recovery was smaller at ∼3% (). For purifying the lowest amount of IgG, set at 20 µg, no loss in recovery was observed (). This indicated that tip columns could tolerate at least 22 CIP cycles before significant loss in the recovery. In another experiment, replicate expressions of a control human IgG1 antibody were carried out 96 deep-well blocks and purified by the same set of tip columns after 3 and 7 cycles of regeneration. The first set of replicate plates yielded 320±13 µg IgG, while the second set of replicate plates yielded 316±16 µg. These replicate purifications also showed very consistent recovery for all wells in each plate (). In practice, we typically re-use MabSelect SuRe tip columns nearly 20 times, resulting in significant tip column purification cost savings.
Figure 6. Regeneration of MabSelect SuRe tip columns (20 µL resin). Before each use, the tip columns were regenerated using 0.2 M NaOH, followed by equilibration with 0.2 M Tris pH 7.0 and PBS. After each regeneration, known amount of human IgG1 spiked into 1 mL blank media was captured onto tip columns and purified into 160 µL final volume (single elution) in automated HTP process: (a) 500 µg IgG spiked; (b) 100 µg spiked; (c) 20 µg IgG spiked (n = 12 each panel). Less than 5% drop of recovery was observed after 22 regenerations in all tested spiking amount, giving very high re-usability of the tip columns packed with MabSelect SuRe resin. (d) a human IgG1 was expressed in replicated 96 deep-well blocks, the 1st replicate block were purified by the tip columns at 3rd use, while the 2nd replicate deep block was purified by the same set of tip columns at 7th use, with regeneration before each use. The tip columns showed consistent yield among individual columns and after different regeneration cycles (3x vs 7x).
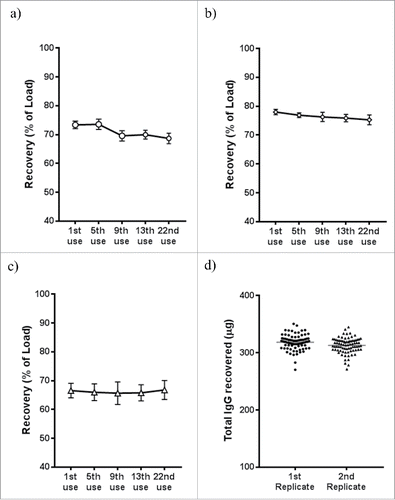
Following manufacturer's protocol, GammaBind Plus was stripped with 6 M guanidine and re-equilibrated with PBS in our HTP workflow. We observed high consistency in GammaBind Plus resin tip columns and no significant binding capacity loss after multiple CIP cycles. However, we experienced reduced flow-rate in traditional columns packed with GammaBind Plus after CIP, most likely because the GammaBind Plus resin was not as rigid as MabSelect SuRe, and resin compaction resulted in clogging. Although the tip columns packed with 20 – 40 µL GammaBind Plus resin were less likely to get clogged, we normally used the GammaBind Plus resin tip columns approximately 10 times before disposal, as compared to 20 times for MabSelect SuRe tip columns.
For resins (MabSelect SuRe and GammaBind Plus) used in batch mode purification, we re-used the resin only three to four times (after regeneration) because the resin beads tended to clump after more repeated uses, and block tips while pipetting on liquid handling systems. In addition, resin cost in batch mode purification was insignificant compared to packed tip columns. We typically stored filter plates containing resins at 4˚C after batch mode purification and re-suspended in 0.5 mL PBS. The same resin from multiple plates can be combined into one 96-well filter plate on a vacuum washing station on the Lynx 1200 deck for stripping and regeneration. Resin used in batch mode from up to 20 plates could be automatically recovered and regenerated in approximately 2 hours by the liquid handling system.
Discussion
We developed two modes of micro-scale purification, tip column mode and batch mode, on two liquid handling systems for automated affinity purification of antibodies. Both of the liquid handling platforms, Tecan EVO 200 and Dynamic Devices Lynx 1200, are standard liquid handling systems with simple customization for purification purposes. These platforms provide walk-away purification workflows with a combined purification capacity of more than 20 plates of 96 samples per day. Most of our initial development and optimization were carried out with human IgG1 using MabSelect SuRe resin, which is coupled with alkaline resistant protein A domain and is a standard resin for purification of human IgG1 in commercial manufacturing.Citation10 Our routine evaluation by analytical SEC, SDS-PAGE and MS of IgG purified by MabSelect SuRe resin showed similar quality from HTP purification process and conventional column purification platforms. Other resins coupled with new engineered protein A ligand for higher capacity of human IgG, such as TOYOPEARL AF-rProtein A HC-650F (Tosoh Bioscience LLC, King of Prussia, PA), can be easily adopted in these automated purification workflows. Higher resin binding capacity can provide a further advantage by reducing the amount of resin needed for efficient capture. In addition, the elution volume can be reduced, resulting in higher product concentration for screening assays. The automated small-scale purification platform described here makes it very easy for comparative evaluations of newly developed resins and their adoption into a HTP purification process.
In addition to human IgG, many other antibody isotypes or species are encountered in early antibody discovery when hybridoma technology is used. These include IgG isotypes from mouse, rat, rabbit, hamster and other species. Most of these antibodies can be robustly purified by suitable commercial resins. Arora and colleagues reviewed how to choose protein A, protein G, protein L or other affinity based resin for purification of different antibody isotypes.Citation11 Recombinant protein A has been reported to have weak binding for mouse IgG1,Citation12 which is a commonly found hybridoma isotype. However, we found MabSelect SuRe, an engineered form of Protein A with a binding capacity of ∼ 25 µg mouse IgG1 per µL resin under physiological conditions, to be a suitable resin in our HTP purification workflows (data not shown). In fact, we have used MabSelect SuRe resin successfully for purification of all hybridoma antibodies generated from mouse, rabbit and hamster. Of the hybridoma antibodies, rat IgG2a had been challenging for HTP purification due to poor capacity or difficulty of elution with most commercial resins. illustrates two robust resins that we commonly use for HTP purification of IgGs from microscale transient expression or hybridoma culture and their typical purification parameters. Using the automated HTP purification platforms, we were able to conduct in-parallel evaluation of multiple binding, washing and elution conditions using GammaBind Plus resin for purification of rat IgG2a. This process allowed rapid identification of optimized conditions to be used in automated HTP processes for all rat hybridoma purification.
Table 3. Parameters for microscale HTP purification of IgGs from transient expression or hybridoma culture.
Our automated HTP workflows have been extensively used in supporting HTP screening for many antibody discovery and engineering campaigns, where hundreds to thousands of clones are routinely purified with yields normally more than 50 µg each, sufficient for 3 – 4 screening assays. Costs of consumables, which include resin, tip columns, tips, filter plates, plates, and buffers, are approximately $1.00 per sample for the tip column mode and approximately $1.50 per sample for the batch mode. The tip mode consumable cost is lower because tip columns are reused up to 20 times and the process doesn't use expensive filter plates. However, the batch mode provides more parallel purification and saves more time when purifying thousands of clones. These workflows are based on affinity purification, which is not limited to purification of antibodies. Purification of many other proteins or targets of interest can be explored in these kinds of workflows for HTP production if suitable affinity resins can be generated. Furthermore, other modes of purification, such as ion exchange or hydrophobic interaction, can be explored if step gradient elution can be developed for automated microscale purification. Introduction of automated workflows allows for the minimization of operation errors, such as contamination and sample mix-ups, and better implementation of sample tracking and data handling by easier integration with lab information management systems.Citation13 In the new drug discovery era, targets have become more difficult and the cost of bringing new molecules to clinics has increased dramatically.Citation14 Modern screening technologies have made it possible to screen in ultra-HTP mode using miniaturized assay formats to reduce cost. By using the automated microscale production workflows as described in this work, high-quality material can be rapidly generated at lower costs to accelerate drug discovery and bring effective therapies faster to patients in need.
Materials and methods
Antibody transient expression and quantitation
Mammalian expression vectors containing heavy chain (HC) and light chain (LC) of a human IgG1 (pertuzumab) were purified using plasmid Giga kit (Cat#12191, Qiagen). HTP transient expression of IgG was carried out as described previously.Citation4 Briefly, Expi293F™ cells were cultivated at 37˚C, 8% CO2, 80% humidity to log phase, then 850 µL cells at 2.0eCitation6 cells/mL was seeded in Axygen 96× 2mL deep-well blocks (Cat# P-DW-20-C-S, Corning) shaken at 1000 rpm and 3 mm orbital diameter. A total of 1 µg plasmid DNA (HC:LC in a 1:1 ratio) was diluted in 100 µL DMEM-based medium with 0.23 mM 25 kDa linear PEI and incubated for 10 minutes before adding to approximately 1 mL Expi293F™ cultures. The transfected cells were fed with 50 µL nutrients on the second day and cultivated for a total of 7 days prior to harvest via centrifugation to pellet the cells. IgG titer in culture supernatants were estimated by ELISA.
Conventional column purification of human IgG
A HiTrap™ column packed with 1 mL MabSelect SuRe resin (Cat# 29-0491-04, GE Healthcare Life Sciences) was used for conventional affinity column purification. Culture supernatant was filtered through a 0.45 µm membrane and loaded onto the column at 0.5 mL/min. Unbound proteins and media were washed off the column with PBS pH 7.5 until the UV trace (measured by A280) came down to baseline, followed by elution with 50 mM phosphoric acid, pH 3.0. Fractions containing IgG were pooled and neutralized with 1/15 volume of 200 mM sodium phosphate, 3 M sodium chloride, pH 11.0. Purified IgG concentration was determined by UV absorbance using an extinction coefficient of 1.37 (mg/mL)−1cm−1 at 280 nm.
Liquid handling systems, labware and automation scripting
The automated purification workflows were developed on two separate liquid handling platforms. One was a customized Tecan Freedom EVO 200 (Morrisville, NC) liquid handling system equipped with MCA96 head for 500 µL tips, a robotic manipulator (RoMa), an 8-channel liquid handling arm (LiHa), a Magellan plate reader, a Hettich centrifuge, a high-speed wash pump, and a six-way port for system buffer selection. The other liquid handler was a Dynamic Devices Lynx LM1200 (Wilmington, DE) equipped with a 96-tip head for 1250 µL tips, autofill reservoir and vacuum stations. UV transparent microplates (Corning Inc., Corning, NY) and other standard SBS format labware were used. Tecan EVOware standard software was used to write scripts to execute fully automated tip column purification, centrifugation and IgG quantitation by measuring UV absorbance on the Tecan instrument. Dynamic Devices Method Manager 2.0 was used to script the steps involved in automated tip column as well as semi-automated batch mode purification.
HTP tip column purification of human IgG from transient expression culture
For purification of up to 500 µg human IgG, 500 µL Tecan tips custom packed (Glygen Corp., Columbia, MD) with 20 µL MabSelect SuRe resin (Cat#17543803, GE Healthcare Life Sciences) were used on Tecan instrument and 1250 µL Lynx tips (PhyNexus, San Jose, CA) with 20 µL MabSelect SuRe resin were used on Dynamic Device instrument. Before tip column purification, culture harvests were centrifuged at 2000 rpm for 5 min to pellet cells if cells were present. On each of these liquid handling systems, the culture supernatants (up to tip capacity, ∼400 µL for Tecan tip columns and ∼1000 µL for Lynx tip columns) were transferred to 96× 2mL deep-well blocks, pipetted up and down for 6 cycles, then transferred to a flow through plate, which gave a total of 8 cycles for capture, including the two transfers. All these transfer and capture steps were executed with the tip columns directly by pipetting at 500 µL/min, and these steps were repeated until all culture supernatant was processed. Unbound proteins and media components were washed off the tip columns by pipetting for 6 cycles in two consecutive 96× 2mL deep-well blocks with 1.5 mL PBS in each well. Finally, the captured antibodies were eluted (twice for higher recovery) from the tip columns with up to 200 µL phosphoric acid, pH 3.0 in microplates. During each elution, elution buffer was pipetted through tip column at 500 µL/min for 3 cycles, which gave a total elution contact time of ∼ 2 min for pipetting a volume of 150 – 200 µL. Eluted IgG was neutralized with 1/15 volume of 200 mM sodium phosphate, 3 M sodium chloride, pH 11.0. Tip columns were stripped by pipetting 6 cycles in two blocks of 0.2 M NaOH (or 6 M guanidine with 50 mM Tris, pH 8) then regenerated in two blocks of PBS. Tip columns were regenerated and used nearly 20 times before they were discarded.
HTP tip column purification of rat IgG
For purification of up to 200 µg rat IgG, tip columns were custom packed or purchased as above using 20 µL GammaBind Plus Sepharose (Cat#17088602, GE Healthcare Life Sciences). For purification of more rat IgG, 20 µL incrementally more resin was packed in each tip column, for example, total of 40 µL resin tip column was used for purification of up to 400 µg rat IgG. Rat IgG capture onto tip column, washing and elution was performed as above described above except for the optimized elution step, where 2 mM sodium phosphate with 30 mM sodium chloride, pH 5.0 was used for washing before elution, 190 µL 10 mM citric acid, pH 2.9 was used for elution, and 1/15 volume of 0.4 M Tris pH 8.7 was used for neutralization. GammaBind Plus tip columns were stripped by pipetting 6 cycles in two blocks of 6 M guanidine with 50 mM Tris, pH 8, then regenerated in two blocks of PBS. Tip columns were regenerated and used typically 10 times before they were discarded.
HTP batch mode purification of human IgG
Culture harvests were centrifuged and supernatants were transferred to 96× 2 mL deep-well blocks using regular tips at 500 µL/min. For purification of up to 500 µg human IgG, 20 µL MabSelect SuRe resin was typically added per mL of culture supernatant to capture IgG. The blocks were sealed with silicone mats and secured with clamps either to a rotator or shaker. After rotating or agitating overnight, blocks were centrifuged at 2000 rpm for 5 min to pellet resin from culture supernatants. Using liquid handling system, excess supernatants were aspirated off to leave ∼0.5 mL in each well. The resin in the 0.5 mL remaining supernatant was resuspended using regular tips at 10 mL/min (for other transfers that do not have cell pellets, pipetting speed is normally >5 mL/min), then transferred to 96×2 mL deep-well filter plates (Cat#931919, Thomson Instrument Co.). To recover more capture resin, each well of the deep-well block was washed again with 0.5 mL PBS and the washes were transferred to the matched filter plates maintaining the same plate layout. Filter plates were centrifuged at 2000 rpm for 3 min to remove culture supernatant, subsequent washes, and elutions. 1.5 mL PBS was added to resin in each filter well to wash the resin. The filter plates were centrifuged or vacuumed to remove PBS. After completing three washes, up to 200 µL phosphoric acid, pH 3.0 was added to each well and incubated for 2 min before each centrifugation for IgG elution. The eluted IgG was collected in microplates by centrifugation, and neutralized by adding 1/15 volume of 200 mM sodium phosphate, 3 M sodium chloride, pH 11.0. Elutions were normally carried out twice for higher recovery.
HTP batch mode purification of rat IgG
For purification of up to 200 µg rat IgG, 20 µL GammaBind Plus resin was typically added to each mL of rat culture harvest in 96×2 mL deep-well blocks. More resin was added for purification of more than 200 µg rat IgG. Rat IgG capture onto resin, washing, and elution were performed as described above except for optimal elution, where 2 mM sodium phosphate with 30 mM sodium chloride, pH 5.0 was used for third washing, 190 µL of 10 mM citric acid, pH 2.9 was used for elution, and 1/15 volume of 0.4 M Tris, pH 8.7 was used for neutralization.
Analytical Size Exclusion Chromatography and SDS-PAGE evaluation of purified IgG
Analytical SEC was carried out on an HPLC (Ultimate 3000, Thermo Fisher Scientific) with 214 nm UV monitoring. 20 µL purified sample was injected onto a TSKgel super SW3000 column (Cat#18675, Tosoh Bioscience) at 0.35 mL/min using 200 mM potassium phosphate, 250 mM potassium chloride, pH 7.0 as the running buffer. For SDS-PAGE analysis, 4–12% NuPAGE Bis-Tris gel (Cat#NP0321BOX, Thermo Fisher Scientific) was used according to the manufacturer's instructions.
Mass spectrometry analysis of purified IgG
MS analysis was performed on an Agilent LC/MS 6224 time-of-flight mass spectrometer (Agilent, Santa Clara, CA). Approximately 2 µg of IgG was reduced with 50 mM dithiothreitol at 37°C for 20 minutes and injected onto a PLRP-S column (Agilent). IgG LC and HC were separated by 10–90% acetonitrile gradient in 10 min at 80°C. Raw m/z spectra were collected by MassHunter acquisition software and deconvoluted using MassHunter Analysis software (Agilent, Santa Clara, CA).
Abbreviations
mAb | = | monoclonal antibody |
IgG | = | immunoglobulin gamma |
HC | = | heavy chain |
LC | = | light chain |
HTP | = | high throughput |
CIP | = | cleaning in place |
SEC | = | size exclusion chromatography |
LC-MS | = | liquid chromatography mass spectrometry |
LiHa | = | Liquid handling arm |
RoMa | = | robotic moving arm |
Disclosure of potential conflicts of iInterest
No potential conflicts of interest were disclosed.
Acknowledgments
We wish to thank our colleagues within the Antibody Production Group who have provided valuable input during design and development of the automated high-throughput purification processes described in this manuscript. In particular, we would like to thank Farzam Farahi for his input during the evaluation and selection of a Dynamic Devices liquid handling system for automated purification. We would also like to thank Kurt Schroeder for his guidance and discussions during development of the microscale purification process.
References
- Reichert JM. Antibodies to watch in 2017. MAbs. 2017;9:167–81. doi:10.1080/19420862.2016.1269580. PMID:27960628.
- Ecker DM, Jones SD, Levine HL. The therapeutic monoclonal antibody market. MAbs. 2015;7:9–14. doi:10.4161/19420862.2015.989042. PMID:25529996.
- Bos AB, Duque JN, Bhakta S, Farahi F, Chirdon LA, Junutula JR, Harms PD, Wong AW. Development of a semi-automated high throughput transient transfection system. J Biotechnol. 2014;180:10–6. doi:10.1016/j.jbiotec.2014.03.027. PMID:24704608.
- Bos AB, Luan P, Duque JN, Reilly D, Harms PD, Wong AW. Optimization and automation of an end-to-end high throughput microscale transient protein production process. Biotechnol Bioeng. 2015;112:1832–42. doi:10.1002/bit.25601. PMID:25851051.
- Grodzki AC, Berenstein E. Antibody purification: Affinity Chromatography – Protein A and Protein G Sepharose. In Oliver C, Jamur MC, eds. Immunocytochemical Methods and Protocols. Totowa, NJ: Humana Press, 2010:33–41.
- Chhatre S, Titchener-Hooker NJ. Review: Microscale methods for high-throughput chromatography development in the pharmaceutical industry. J Chem Technol Biotechnol. 2009;84:927–40. doi:10.1002/jctb.2125.
- Schmidt PM, Abdo M, Butcher RE, Yap M-Y, Scotney PD, Ramunno ML, Martin-Roussety G, Owczarek C, Hardy MP, Chen CG, et al. A robust robotic high-throughput antibody purification platform. J Chromatogr A. 2016;1455:9–19. doi:10.1016/j.chroma.2016.05.076. PMID:27283099.
- Spooner J, Wilkinson T, Kemp BP. Current advances in the development of high-throughput purification strategies for the generation of therapeutic antibodies. Pharm Bioprocess. 2015 3:411–24. doi:10.4155/pbp.15.23.
- Wang L, Dembecki J, Jaffe N, O'Mara B, Cai H, Sparks C, Zhang J, Laino SG, Russell RJ, Wang M. A safe, effective, and facility compatible cleaning in place procedure for affinity resin in large-scale monoclonal antibody purification. J Chromatogr A. 2013;1308:86–95. doi:10.1016/j.chroma.2013.07.096. PMID:23953712.
- Yang L, Harding JD, Ivanov AV, Ramasubramanyan N, Dong DD. Effect of cleaning agents and additives on Protein A ligand degradation and chromatography performance. J Chromatogr A. 2015;1385:63–8. doi:10.1016/j.chroma.2015.01.068. PMID:25680549
- Arora S, Saxena V, Ayyar BV. Affinity chromatography: A versatile technique for antibody purification. Methods. 2017;116:84–94. doi:10.1016/j.ymeth.2016.12.010. PMID:28012937.
- Ey PL, Prowse SJ, Jenkin CR. Isolation of pure IgG1, IgG2a and IgG2b immunoglobulins from mouse serum using protein A-Sepharose. Immunochemistry. 1978;15:429–36. doi:10.1016/0161-5890(78)90070-6. PMID:30693.
- Chapman T. Lab automation and robotics: Automation on the move. Nature. 2003;421:661–6. PMID:12571603.
- DiMasi JA, Grabowski HG, Hansen RW. Innovation in the pharmaceutical industry: New estimates of R&D costs. J Health Econ. 2016;47:20–33. doi:10.1016/j.jhealeco.2016.01.012. PMID:26928437.