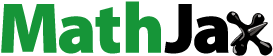
ABSTRACT
DSTA4637S, a novel THIOMAB™ antibody-antibiotic conjugate (TAC) against Staphylococcus aureus (S. aureus), is currently being investigated as a potential therapy for complicated S. aureus bloodstream infections. DSTA4637S is composed of a monoclonal THIOMABTM IgG1 recognizing S. aureus linked to a rifamycin-class antibiotic (dmDNA31) via a protease-cleavable linker. The pharmacokinetics (PK) of DSTA4637A (a liquid formulation of DSTA4637S) and its unconjugated antibody MSTA3852A were characterized in rats and monkeys. Systemic concentrations of three analytes, total antibody (TAb), antibody-conjugated dmDNA31 (ac-dmDNA31), and unconjugated dmDNA31, were measured to describe complex TAC PK in nonclinical studies. In rats and monkeys, following intravenous administration of a single dose of DSTA4637A, systemic concentration-time profiles of both TAb and ac-dmDNA31 were bi-exponential, characterized by a short distribution phase and a long elimination phase as expected for a monoclonal antibody-based therapeutic. Systemic exposures of both TAb and ac-dmDNA31 were dose proportional over the dose range tested, and ac-dmDNA31 cleared 2–3 times faster than TAb. Unconjugated dmDNA31 plasma concentrations were low (<4 ng/mL) in every study regardless of dose. In this report, an integrated semi-mechanistic PK model for two analytes (TAb and ac-dmDNA31) was successfully developed and was able to well describe the complicated DSTA4637A PK in mice, rats and monkeys. DSTA4637S human PK was predicted reasonably well using this model with allometric scaling of PK parameters from monkey data. This work provides insights into PK behaviors of DSTA4637A in preclinical species and informs clinical translatability of these observed results and further clinical development.
Abbreviations: ADC: Antibody-drug conjugate; AUCinf: time curve extrapolated to infinity; ac-dmDNA31: antibody-conjugated dmDNA31; Cmax: maximum concentration observed; DAR: drug-to-antibody ratio; CL: clearance; CLD: distribution clearance; CL1: systemic clearance of all DAR species; kDC: deconjugation rate constant; PK: Pharmacokinetics; IV: Intravenous; IgG: Immunoglobulin G; mAb: monoclonal antibody; S. aureus: Staphylococcus aureus; TAC: THIOMABTM antibody-antibiotic conjugate; TDC: THIOMABTM antibody-drug conjugate; TAb: total antibody; t1/2, λz: terminal half-life; vc linker: valine-citrulline linker; Vss: volume of distribution at steady state; Vc: volume of distribution for the central compartment; Vp: the volume of distribution for the peripheral compartment.
Introduction
Staphylococcus aureus (S. aureus) is a leading cause of human bacterial infections worldwide, with serious complications such as sepsis, infective endocarditis, and osteomyelitis occurring following dissemination of the bacteria into the bloodstream.Citation1 Despite appropriate antibiotic treatment, invasive S. aureus infections are associated with mortality rates up to 40% attributed to metastatic, recurrent, and/or relapsing infections.Citation2,Citation3 One proposed mechanism by which S. aureus resists treatment with antibiotics is via its ability to survive within host phagocytic cells.Citation4 Therapeutic approaches targeting intracellular persistence may provide novel treatment options for invasive S. aureus infections.Citation5,Citation6
DSTA4637S is a THIOMAB™Citation7 antibody-antibiotic conjugate (TAC) that contains an engineered human immunoglobulin G1 (IgG1) anti-S. aureus monoclonal antibody (mAb), MSTA3852A, and a novel antibiotic, dmDNA31 (4-dimethylamino piperidino-hydroxybenzoxazino rifamycin), linked through a protease-cleavable valine-citrulline (vc) linker. The antibiotic, dmDNA31, is a rifamycin class antibiotic with potent bactericidal activity against S. aureus. The antibody intermediate (i.e., unconjugated antibody), MSTA3852A, specifically binds to the β-O-linked N-acetylglucosamine sugar modification of wall teichoic acid (WTA), a major cell wall component of S. aureus. A cysteine is substituted for a valine (V211C) in each light chain of the antibody to allow site-directed conjugation of the linker-drug, vc-dmDNA31, with nearly uniform stoichiometry (i.e., an average drug-to-antibody ratio [DAR] of two vc-dmDNA31 molecules per antibody). The in vitro experiments by Lehar et al.Citation5 demonstrated that when the TAC-opsonized S. aureus bacteria enter the intracellular environment such as a phagolysosome, host proteases such as cathepsins cleave the linker and readily release the antibiotic in its active form. When bacteria are released from pre-existing intracellular reservoirs into the extracellular space, the prolonged presence of TAC in the systemic circulation could ensure immediate “tagging” of these bacteria before they can escape to neighboring or distant cells, thus mitigating further spread of the infection. These properties provide the rationale for developing DSTA4637S, in combination with the current standard of care anti-staphylococcal antibiotics, as a novel therapeutic approach to treat severe methicillin-sensitive and methicillin-resistant S. aureus infections. DSTA4637S is currently in clinical development for the treatment of S. aureus bloodstream infections (bacteremia) (ClinicalTrials.gov Identifier: NCT02596399 [Phase 1], NCT03162250 [Phase 1b]).
DSTA4637S refers to the molecule in the lyophilized drug product presentation intended for use in clinical studies, while DSTA4637A refers to the liquid presentation used for nonclinical studies. DSTA4637A has demonstrated enhanced killing of S. aureus in in vitro and in vivo nonclinical studies compared with vancomycin.Citation5,Citation6 Pharmacokinetics (PK) of DSTA4637A in mice has been reported previously.Citation8 In this report, we extended these studies to characterize the PK of DSTA4637A and its unconjugated antibody MSTA3852A in rats and cynomolgus monkeys to further understand the PK behaviors of DSTA4637A in preclinical species and to inform translation to humans and further clinical development. As described by Zhou et al.,Citation8 the characterization of PK for DSTA4637A included the quantification of three key analytes:Citation9 DSTA4637A total antibody (TAb, measurement of all drug-to-antibody ratios [DARs] of DSTA4637A including fully conjugated [DAR = 2], partially deconjugated [DAR = 1], and fully deconjugated [DAR = 0] anti-S. aureus antibodies), DSTA4637A conjugate determined as antibody-conjugated dmDNA31 (ac-dmDNA31, measurement of dmDNA31 conjugated to the antibody), and unconjugated dmDNA31 (measurement of dmDNA31 that is not conjugated to the antibody through the vc linker).
To improve our understanding of the complex PK behaviors of DSTA4637A in different species (mouse, rat and monkey) with a translational application, a semi-mechanistic PK model was developed based on a previously published modelCitation10 using these multiple analytes. This model was then used for DSTA4637S human PK prediction with allometric scaling of PK parameters from monkey data only.
Results
Pharmacokinetics of DSTA4637A and MSTA3852A in rats
The PK profiles of DSTA4637A TAb and DSTA4637A conjugate (measured as antibody-conjugated dmDNA31, ac-dmDNA31) following a single intravenous (IV) administration of DSTA4637A in Sprague Dawley (SD) rats at dose levels of 1, 25, or 50 mg/kg are shown in and PK parameters are summarized in and . The serum concentration-time profiles of DSTA4637A TAb were characterized by a short distribution phase and a longer elimination phase, as expected for a mAb-based therapeutic (). The systemic exposure of DSTA4637A TAb [maximum concentration observed (Cmax), and area under the concentration–time curve extrapolated to infinity (AUC0-inf)] was dose proportional over the dose range of 1 to 50 mg/kg, which is expected given the absence of target antigen in non-infected rats. The mean clearance (CL) of TAb ranged from 9.26 to 11.4 mL/day/kg, mean terminal half-life (t1/2, λz) ranged from 6.77 to 9.27 days, and mean volume of distribution at steady state (Vss) ranged from 86.1 to 122 mL/kg over the dose range tested ().
Table 1. Mean ± SD non-compartmental PK parameters of DSTA4637A TAb and MSTA3852A following a single IV administration of DSTA4637A or MSTA3852A in rats.
Table 2. Mean ± SD non-compartmental PK parameters of DSTA4637A conjugate (measured as antibody-conjugated dmDNA31 [ac-dmDNA31]) following a single IV administration of DSTA4637A in rats.
Figure 1. Group mean (± SD) concentration-time profiles of (a) DSTA4637A TAb and MSTA3852A and (b) DSTA4637A conjugate (measured as antibody-conjugated dmDNA31 [ac-dmDNA31]) following a single IV administration of DSTA4637A at 1, 25, or 50 mg/kg, or MSTA3852A at 1, 25, or 50 mg/kg in rats. n = 6 animals per group. Representation of Figure 1 in molar units is in supplementary materials (Supplementary Figure 1).
![Figure 1. Group mean (± SD) concentration-time profiles of (a) DSTA4637A TAb and MSTA3852A and (b) DSTA4637A conjugate (measured as antibody-conjugated dmDNA31 [ac-dmDNA31]) following a single IV administration of DSTA4637A at 1, 25, or 50 mg/kg, or MSTA3852A at 1, 25, or 50 mg/kg in rats. n = 6 animals per group. Representation of Figure 1 in molar units is in supplementary materials (Supplementary Figure 1).](/cms/asset/b4001682-6f7f-4101-90ff-8491c75e2dc0/kmab_a_1627152_f0001_c.jpg)
The concentrations of DSTA4637A conjugate (measured as antibody-conjugated dmDNA31, ac-dmDNA31) reached their peak immediately after a single IV administration of DSTA4637A and decreased over time in a manner similar to the DSTA4637A TAb concentrations (i.e., a bi-exponential disposition pattern ()). The mean CL of ac-dmDNA31 following 1 to 50 mg/kg DSTA4637A administration in rats ranged from 23.1 to 24.7 mL/day/kg, which was about two times faster than that of DSTA4637A TAb (). The mean t1/2, λz of ac-dmDNA31 following 1 to 50 mg/kg DSTA4637A administration ranged from 2.60 to 3.25 days, which was about two to three times shorter than that of DSTA4637A TAb. The mean Vss of ac-dmDNA31 ranged from 73.2 to 86.2 mL/kg, which was comparable to that of DSTA4637A TAb.
Unconjugated dmDNA31 plasma concentrations after administration of DSTA4637A were low across all groups (). The highest group mean Cmax was 1.15 ng/mL following the 50 mg/kg dose and peaked at 10 minutes post-dose (individual Cmax ranging from 0.868 ng/mL (0.937 nM) to 1.51 ng/mL (1.63 nM)).
Figure 2. Group mean (± SD) concentration-time profiles of unconjugated dmDNA31 following a single IV administration of DSTA4637A at 1, 25, or 50 mg/kg in rats. n = 6 animals per group. Unconjugated dmDNA31 concentration for all animals (n = 6) in the 1 mg/kg group were below LLOQ for all time points. Unconjugated dmDNA31 concentration in 1 of 6 animals was below LLOQ on Day 2 in the 25 mg/kg group. Unconjugated dmDNA31 concentration were below LLOQ from Day 2 to Day 29 and Day 7 to Day 29, respectively, for all animals (n = 6) in the 25 mg/kg group and the 50 mg/kg group. Representation of Figure 2 in molar units is in supplementary materials (Supplementary Figure 2).
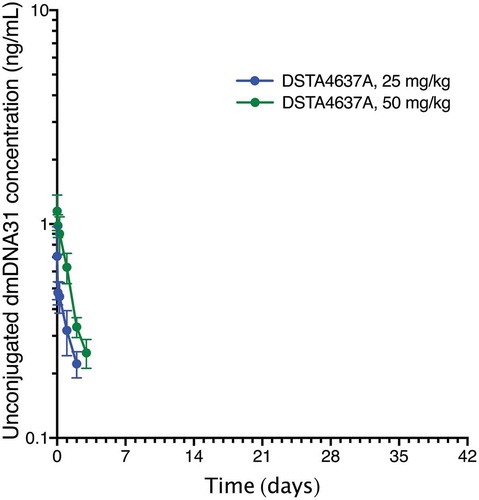
The PK profiles of MSTA3852A following a single IV administration of MSTA3852A in SD rats at dose levels of 1, 25, or 50 mg/kg are shown in , and PK parameters are summarized in . The PK of MSTA3852A was as expected for a human IgG1 antibody and consistent with other typical IgG1 antibodies.Citation11 MSTA3852A mean CL following IV administration of 1 to 50 mg/kg of MSTA3852A ranged from 8.94 to 11.0 mL/day/kg; mean t1/2, λz ranged from 3.85 to 7.65 days. These values were within twofold of those of DSTA4637A TAb, suggesting a minimal effect of dmDNA31 conjugation on antibody CL in rats.
Pharmacokinetics of DSTA4637A and MSTA3852A in cynomolgus monkeys
The PK profiles of DSTA4637A TAb and DSTA4637A conjugate (measured as antibody-conjugated dmDNA31, ac-dmDNA31) following a single IV administration of DSTA4637A in cynomolgus monkeys at dose levels of 1, 15, or 150 mg/kg are shown in , and PK parameters are summarized in and . The concentration-time profiles of DSTA4637A TAb () and ac-dmDNA31 () were bi-exponential and characterized by a short distribution phase and a longer elimination phase as expected for a mAb--based therapeutic as well. Exposure (Cmax, and AUC0-inf) of both analytes was dose proportional over the dose range of 1 to 150 mg/kg, which is expected given the absence of the target antigen in non-infected cynomolgus monkeys. DSTA4637A TAb mean CL following IV administration of 1, 15, or 150 mg/kg DSTA4637A was 5.29, 5.29, and 6.48 mL/day, respectively; mean t1/2, λz was 10.5, 11.2, and 11.2 days, respectively, and mean Vss was 73.9, 78.8, and 88.3 mL/kg, respectively ().
Table 3. Mean ± SD non-compartmental PK parameters of DSTA4637A TAb and MSTA3852A following a single IV administration of DSTA4637A or MSTA3852A in cynomolgus monkeys.
Table 4. Mean ± SD noncompartmental PK parameters of DSTA4637A conjugate (measured as antibody-conjugated dmDNA31 [ac-dmDNA31]) following a single IV administration of DSTA4637A in cynomolgus monkeys.
Figure 3. Group mean (± SD) concentration-time profiles of (a) DSTA4637A TAb and MSTA3852A and (b) DSTA4637A conjugate (measured as antibody-conjugated dmDNA31 [ac-dmDNA31]) following a single IV administration of DSTA4637A at 1, 15, or 150 mg/kg, or MSTA3852A at 50 mg/kg in cynomolgus monkeys. n = 3 for all time-points, except for DSTA4637A TAb in the 1 mg/kg group, for which n = 2 at Day 35 and n = 1 at Day 42. Representation of Figure 3 in molar units is in supplementary materials (Supplementary Figure 3).
![Figure 3. Group mean (± SD) concentration-time profiles of (a) DSTA4637A TAb and MSTA3852A and (b) DSTA4637A conjugate (measured as antibody-conjugated dmDNA31 [ac-dmDNA31]) following a single IV administration of DSTA4637A at 1, 15, or 150 mg/kg, or MSTA3852A at 50 mg/kg in cynomolgus monkeys. n = 3 for all time-points, except for DSTA4637A TAb in the 1 mg/kg group, for which n = 2 at Day 35 and n = 1 at Day 42. Representation of Figure 3 in molar units is in supplementary materials (Supplementary Figure 3).](/cms/asset/0b016b37-bcba-48ff-971c-154dddd06130/kmab_a_1627152_f0003_c.jpg)
The concentrations of ac-dmDNA31 reached their peak immediately after a single IV administration of DSTA4637A and decreased over time in a manner similar to the TAb concentrations (). The mean CL of ac-dmDNA31 following 1, 15 or 150 mg/kg DSTA4637A administration was 15.1, 16.2 and 21.5 mL/day/kg, respectively, which was about three times faster than that of the DSTA4637A TAb ( and ). The mean t1/2, λz of ac-dmDNA31 following 1, 15 or 150 mg/kg DSTA4637A administration was 4.04, 4.33 and 4.11 days, respectively, which was about two to three times shorter than that of DSTA4637A TAb. The mean Vss of ac-dmDNA31 ranged from 72.6 to 88.1 mL/kg, which was comparable to that of DSTA4637A TAb.
Unconjugated dmDNA31, following DSTA4637A administration, had higher concentrations at higher DSTA4637A dose levels (). The mean Cmax of unconjugated dmDNA31 for animals that received 150 mg/kg of DSTA4637A was 3.07 ng/mL and peaked at 6 hours post-dose. Overall, unconjugated dmDNA31 concentrations were very low across all dose levels, with concentrations ranging from 0.217 ng/mL (0.234 nM) to 3.75 ng/mL (4.04 nM, less than 1% of ac-dmDNA31).
Figure 4. Group mean (± SD) concentration-time profiles of unconjugated dmDNA31 following a single IV administration of DSTA4637A at 1, 15, or 150 mg/kg in cynomolgus monkeys. n = 3 for all time-points. Unconjugated dmDNA31 concentration for all animals (n = 3) in the 1 mg/kg group were below LLOQ for all time points. Unconjugated dmDNA31 concentration were below LLOQ from Day 2 to Day 42 and Day 14 to Day 42, respectively, for all animals (n = 3) in the 15 mg/kg group and the 150 mg/kg group. Representation of Figure 4 in molar units is in supplementary materials (Supplementary Figure 4).
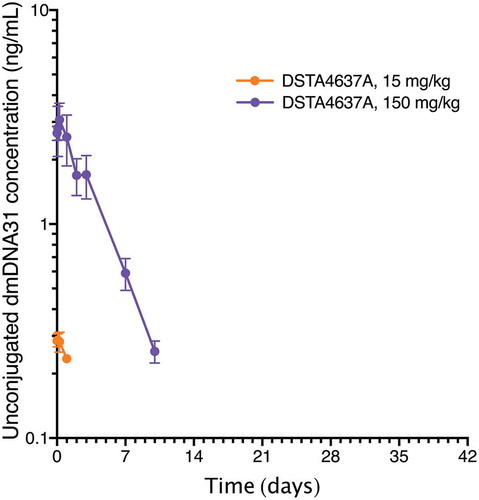
The PK of MSTA3852A in cynomolgus monkeys following a single IV administration of 50 mg/kg MSTA3852A () was as expected for a human IgG1 antibody and was consistent with other typical IgG1 antibodies developed by Genentech.Citation11,Citation12 The CL and t1/2, λz of MSTA3852A were 4.50 mL/day/kg and 13.9 days, respectively. These values were within twofold of those of DSTA4637A TAb, suggesting a minimal effect of the dmDNA31 conjugation on the antibody CL in cynomolgus monkeys.
Immunogenicity of DSTA4637A in cynomolgus monkeys
The incidence of anti-drug antibody (ADA) in cynomolgus monkeys dosed with MSTA3852A was 0% (0 of 3). Only one of the nine monkeys developed ADAs after receiving DSTA4637A (incidence of ADA, 11%). A second monkey dosed with DSTA4637A had a positive baseline signal that was unaffected after treatment with DSTA4637A. These two animals had low post-baseline ADA titers and PK profiles similar to the ADA negative animals (data not shown). Therefore, ADAs had no apparent impact on the DSTA4637A PK in cynomolgus monkeys.
Semi-mechanistic pharmacokinetic modeling of DSTA4637A PK in mouse, rat and monkey
An integrated semi-mechanistic PK model structure () was developed to describe the complex PK profiles of DSTA4637A based on known mechanisms of DSTA4637A disposition obtained from experimental multi-analyte PK profiles in pre-clinical species, including mouse, rat and cynomolgus monkey. The model, which is based on and modified from a previously published model by Sukumaran et al.,Citation10 focuses on antibody-containing species (fully deconjugated antibody (DAR = 0, i.e., DAR0), drug-conjugated antibody (DAR = 1 or 2, i.e., DAR1 or DAR2), and antibody-conjugated drug (i.e., ac-dmDNA31)), and each DAR species (DAR0, DAR1 and DAR2) is explicitly represented. Of note, only antibody-conjugated drug (i.e., ac-dmDNA31) and TAb (i.e., all drug-antibody ratios (DARs = 0, 1 and 2) of DSTA4637A) are represented in the model with corresponding experimental measurements used for model fitting; unconjugated drug (i.e., unconjugated dmDNA31) is not modeled. Sequential deconjugation of the drug (dmDNA31) from the antibody, resulting in the conversion of higher DAR species to lower DAR species, is modeled as an irreversible first-order process, assuming the deconjugation rate constant (kDCi) for each DAR species is proportional to its DAR value. In addition to this deconjugation-driven clearance of DAR1 and DAR2 species, the model also includes antibody clearance due to catabolism of the antibody-containing species (DAR0, DAR1 and DAR2). A key feature of this model that distinguishes it from Sukumaran’s work is the representation of the same antibody systemic clearance (CL1) for all DAR species (i.e., CL1, DAR0 = CL1, DAR1 = CL1, DAR2), because the catabolic clearance of low-DAR (DAR0, DAR1 and DAR2) species are expected to be very similarCitation13 (see more detail in the discussion).
Figure 5. Schematic of an integrated mechanism-based pharmacokinetic model for DSTA4637A across preclinical species.
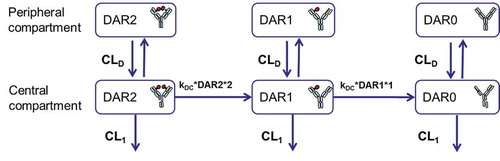
The same structural model described the observed TAb and ac-dmDNA31 concentration-time profiles in mouse, rat and monkey well across all tested dose levels (). Model parameter estimates are summarized in . There was an overall agreement between the predicted and observed data; no systematic bias was identified by the diagnostic plots (Supplementary Figure 5) of the final model identified no systematic bias. The CL1 of all DAR species (i.e., DAR0, DAR1 and DAR2) was estimated as 4.83 mL/day/kg, 8.60 mL/day/kg and 5.82 mL/day/kg in mice, rats and monkey, respectively. The distribution clearance (CLD) is assumed to be common across all DAR species (i.e., DAR0, DAR1 and DAR2) and was estimated as 109 mL/day/kg, 61.4 mL/day/kg and 28.2 mL/day/kg in mice, rats and monkeys, respectively. The volume of distribution parameters including the volume of distribution for the central compartment (Vc) and the volume of distribution for the peripheral compartment (Vp) are also assumed to be common for DAR0, DAR1 and DAR2 species. Vc and Vp were estimated to be 47.7 mL/kg and 59.6 mL/kg, respectively, in mice; 43.3 mL/kg and 66.2 mL/kg, respectively, in rats; and 44.6 mL/kg and 41.3 mL/kg, respectively, in monkeys. The deconjugation rate constants were estimated as 0.345 1/day, 0.451 1/day and 0.302 1/day for mice, rats and monkeys, respectively.
Table 5. Parameter estimates by fitting DSTA4637A PK data (DSTA4637A TAb and DSTA4637A conjugate (measured as antibody-conjugated dmDNA31 [ac-dmDNA31])) in mice, rats and cynomolgus monkeys to the model shown in and predicted human PK parameters.
Figure 6. DSTA4637A TAb and DSTA4637A conjugate (measured as antibody-conjugated dmDNA31 [ac-dmDNA31]) concentration measurements (closed circles) and model fits (solid lines) in (a) mouse, (b) rat and (c) cynomolgus monkey.
![Figure 6. DSTA4637A TAb and DSTA4637A conjugate (measured as antibody-conjugated dmDNA31 [ac-dmDNA31]) concentration measurements (closed circles) and model fits (solid lines) in (a) mouse, (b) rat and (c) cynomolgus monkey.](/cms/asset/e9a3b53c-f6b6-4937-b016-376c42a0216f/kmab_a_1627152_f0006_c.jpg)
A local sensitivity analysis was also performed to assess the sensitivity of model outputs (i.e., AUCs as a surrogate for the concentration – time profiles of TAb and ac-dmDNA31) in the current final model to changes in individual parameters. The analysis was only conducted for the final model in monkey due to the structural similarity across preclinical species. Small changes in AUC values would suggest that exposure is relatively insensitive to the adjusted parameter. CL1 was found to be the most important parameter influencing the TAb plasma concentrations (). A 10% decrease of CL1 led to a 9% increase in the AUC value of TAb. TAb concentration is expected to be relatively insensitive to deconjugation rate constant (kDC) (). However, kDC was found to be the key parameter affecting ac-dmDNA31 exposure, together with Vc ().
Figure 7. The plots represent the percent changes of AUC values of DSTA4637A TAb (a) and DSTA4637A conjugate (measured as antibody-conjugated dmDNA31 [ac-dmDNA31]) (b) following ± 10% changes of a given parameter value (i.e., antibody systemic clearance (CL1), deconjugation rate constant (kDC) distribution clearance (CLD), the volume of distribution for the central compartment (Vc) and the volume of distribution for the peripheral compartment (Vp)). The analysis was performed with simulations at a single IV dose of 5 mg/kg DSTA4637A in monkeys. Filled and empty bars represent – 10% and +10% changes of a given parameter, respectively.
![Figure 7. The plots represent the percent changes of AUC values of DSTA4637A TAb (a) and DSTA4637A conjugate (measured as antibody-conjugated dmDNA31 [ac-dmDNA31]) (b) following ± 10% changes of a given parameter value (i.e., antibody systemic clearance (CL1), deconjugation rate constant (kDC) distribution clearance (CLD), the volume of distribution for the central compartment (Vc) and the volume of distribution for the peripheral compartment (Vp)). The analysis was performed with simulations at a single IV dose of 5 mg/kg DSTA4637A in monkeys. Filled and empty bars represent – 10% and +10% changes of a given parameter, respectively.](/cms/asset/1bc6b728-3d1d-4365-95b5-b99b995503c8/kmab_a_1627152_f0007_b.gif)
Model-based clinical translation for DSTA4637A pharmacokinetics
lists the predicted human PK parameters for DSTA4637S based on monkey data only. CL1 and CLD were predicted as 2.75 and 13.3 mL/day/kg, respectively, while exponent of 0.75 was used for scaling based on body weight. The predicted Vc and Vp are the same as those estimated for monkeys since exponent of 1 was used for scaling based on body weight. The deconjugation rate constant in humans was assumed to be the same as that in monkeys.
DSTA4637S PK was evaluated in healthy volunteers in a Phase 1 clinical study (ClinicalTrials.gov Identifier: NCT02596399). shows observed DSTA4637S TAb and ac-dmDNA31 concentrations from the Phase 1 studyCitation14 overlaid with predicted concentrations for DSTA4637S TAb and ac-dmDNA31 from the integrated PK model in conjunction with predicted human PK parameters. The predictions from the integrated PK model using human PK parameters obtained by allometric scaling of monkey PK parameters agreed well with observed DSTA4637S TAb and ac-dmDNA31 concentrations in healthy volunteers ( and Supplementary Table 1), albeit with an under-prediction of concentrations during the initial distribution phase for both DSTA4637S TAb and throughout the time-course of ac-dmDNA31.
Figure 8. Observed (open circle) and model-predicted [median (dashed line), 95% confidence interval (blue-shaded area)] concentration-time profiles normalized by dose for (a) DSTA4637S TAb and (b) DSTA4637S conjugate (measured as antibody-conjugated dmDNA31 [ac-dmDNA31]) in a Phase I clinical trial (NCT02596399) following a single IV administration of DSTA4637S. Insert: A zoom of the first 10 days of observed (open circle) and model-predicted [median (dashed line), 95% confidence interval (blue-shaded area)] concentration-time profiles normalized by dose. Figures in the linear scale are in supplementary materials (Supplementary Figure 6).
![Figure 8. Observed (open circle) and model-predicted [median (dashed line), 95% confidence interval (blue-shaded area)] concentration-time profiles normalized by dose for (a) DSTA4637S TAb and (b) DSTA4637S conjugate (measured as antibody-conjugated dmDNA31 [ac-dmDNA31]) in a Phase I clinical trial (NCT02596399) following a single IV administration of DSTA4637S. Insert: A zoom of the first 10 days of observed (open circle) and model-predicted [median (dashed line), 95% confidence interval (blue-shaded area)] concentration-time profiles normalized by dose. Figures in the linear scale are in supplementary materials (Supplementary Figure 6).](/cms/asset/f68bd013-4009-484d-bc9c-0e77a3556c5b/kmab_a_1627152_f0008_c.jpg)
Discussion
In this report, we conducted studies to characterize the PK of DSTA4637A, an anti-S. aureus THIOMABTM antibody-antibiotic conjugate, in rats and monkeys, and evaluated the potential impact of the conjugation process on the PK of DSTA4637A by comparing it to the unconjugated anti-S. aureus antibody MSTA3852A. This expands our previous report on DSTA4637A PK characterization in mice.Citation8 Using our experimental measurements from mice, rats and cynomolgus monkeys, a semi-mechanistic PK model was developed and parameter estimates were obtained by simultaneously fitting the model to DSTA4637A TAb and ac-dmDNA31 PK data at all dose levels for each animal species. We further used this model to predict DSTA4637S human PK in conjunction with allometric scaling of monkey PK parameters. This semi-mechanistic PK model improves our understanding of the complex PK behavior of DSTA4637A in different preclinical animal species with a translational application for human PK prediction.
In rats and cynomolgus monkeys, the DSTA4637A TAb and MSTA3852A antibody (unconjugated anti-S. aureus antibody) demonstrated PK behaviors expected of mAbs, characterized by short distribution, relatively slow clearance, and long half-life.Citation15,Citation16 The PK of DSTA4637A TAb in rats and cynomolgus monkeys was also comparable to the PK of MSTA3852A, indicating there was a minimal impact of the conjugation of dmDNA31 on the PK of the MSTA3852A, similar to what was previously observed in mice.Citation8 Therefore, consistent with the current understanding of antibody-drug conjugate (ADC) PK, the disposition of DSTA4637A TAb and DSTA4637A conjugate (measured as ac-dmDNA31) is more like that of an antibody than a small molecule chemical entity.Citation17 The Vss of ac-dmDNA31 was similar to that of the DSTA4637A TAb, indicating that the distribution of ac-dmDNA31 was primarily governed by the distribution of the antibody. The DSTA4637A conjugate (measured as ac-dmDNA31) plasma concentrations decreased over time in a manner similar to DSTA4637A TAb concentrations in rats and cynomolgus monkeys. This similarity demonstrates that the DSTA4637A does not rapidly deconjugate in vivo, but rather the dmDNA31 remains linked to the antibody, emulating the pharmacokinetics of the antibody component of the DSTA4637A. These observations are consistent with those observed in the mouse PK studies reported by Zhou et al.Citation8
For ADCs, the PK of an antibody may be altered by the conjugation of the drug. Several factors that could contribute to this change, including drug load and hydrophobicity, have been investigated and discussed in more detail by Kamath et al.Citation17 ADCs with lower drug loads (DAR of 2 or 4) had slower clearance values and longer half-lives compared to an ADC with a higher drug load (DAR of 8).Citation18 THIOMAB™ antibody technology, which uses site-specific conjugation methods with engineered cysteines to produce a more homogenous ADC, can improve ADC properties including PK, such as a slower CL.Citation7 The conjugation method applied to DSTA4637A, a TAC, allows dmDNA31 to be conjugated with defined stoichiometry without disruption of the inter-chain disulfide bonds of the antibody; hence, there was minimal impact of the conjugate process on PK of the TAb and improved the PK, in contrast to ADCs using other conjugation chemistry approaches such as lysine or inter-chain disulfides. Our data indicate that DSTA4637A TAb has a slower clearance and a longer half-life in rats and monkeys compared with other TAb for other ADCs. This may be partially due to the low DARs (average of 1.9, range 0–2)Citation6 of DSTA4637A relative to traditional ADCs containing higher DARs species (e.g., trastuzumab-DM1, DAR average of 3.5, range 0–8).Citation9 In addition, dmDNA31 is more hydrophilic than tubulin inhibitors (e.g., DM1, MMAE), that may also contribute to the well-behaved PK, such as the long half-life and the slow CL, of DSTA4637A.
ac-dmDNA31 cleared 2–3 times faster than TAb in preclinical species, likely due to the deconjugation process, which is consistent with reported ADC PK properties.Citation19 Deconjugation of DSTA4637A is mainly driven by maleimide exchange and possible metabolism of the payload, dmDNA31 (Genentech unpublished data). However, ac-dmDNA31 still has a longer half-life in rats and monkeys (3–4 days) due to a slower CL of total Ab.
Different PK modeling approaches have been used to describe ADC/THIOMABTM antibody-drug conjugate (TDC) disposition and to facilitate the development of ADC/TDCs. Of note, TAC is one subset of TDC, with the antibiotic as the conjugated drug. The simple ones are basic two-compartmentalCitation20 or three-compartmentalCitation21 models, which describe the observed PK profiles for a specific ADC analyte (typically antibody conjugate (measured as antibody-conjugated drug or as a conjugated antibody) concentrations in serum/plasma). Other PK modeling approaches include semi-mechanistic PK models using a series of transit compartments to describe the deconjugation process from higher to lower DAR species,Citation10,Citation22,Citation23 multi-scale mechanistic PK models in the cellular level, plasma and tissueCitation24 and physiologically based pharmacokinetic (PBPK) models.Citation25 The semi-mechanistic PK model developed by Sukumaran et al.Citation10 incorporated the effects of DAR on drug deconjugation and antibody clearance processes. The structure of our mathematical model, which is adapted from Sukumaran’s model, was developed based on the known mechanisms of deconjugation and observed impact of the DAR on the PK of ADCs in general. Specifically, our model accounts for DAR-dependent drug deconjugation and proteolytic degradation of DSTA4637A, and was assessed by the simultaneous fitting of different PK analytes in the preclinical animal species (mouse, rat and monkey). Our model assumes that the DAR-dependent drug deconjugation is sequential and linear, as prior models have.Citation10,Citation26 In contrast, Lu et al.Citation23 utilized a Weibull distribution function to describe DAR-dependent sequential drug deconjugation for anti-CD79b-MMAE ADC with DAR ranging from 0 to 8 in monkeys. The sequential deconjugation assumption is based on the observations by Sukumaran et al.,Citation13 in which purified TDCs with DAR 1, 2 and 4 were administered to the mice and concentrations of different DAR species over time were measured. DSTA4637A, a THIOMABTM antibody conjugate with DAR of 2, has the same chemical structure as those purified TDCs with DAR of 2 described in Sukumaran’s paper.Citation13 Immediately post-intravenous administration of 5 mg/kg of DAR2 thio-trastuzumab-vc-MMAE, the DAR2 fraction was almost 100%, which then declined over time. The decrease of the DAR2 fraction corresponded to the simultaneous increase in the DAR1 fraction, which started at 0% post-dose, increased over time, and began to decline after reaching a maximum percent of fraction around day 14. The total antibody, DAR2, and DAR1 concentration profiles were best described by a high-to-low-DAR sequential transition model. Xu et al.Citation27 reported similar observations for anti-MUC16 TDC (DAR as 2) in cynomolgus monkeys, which further supports the sequential deconjugation assumption. It is assumed that deconjugation of DSTA4637A occurs only in the central compartment in our model. Alternative models that incorporated deconjugation clearances of the conjugate in the peripheral compartments (i.e., tissue sites) did not improve the model fitting, possibly due to the measurement of only serum/plasma concentrations of each analyte in our studies. This has been observed by Lu et al.Citation26 as well.
One key difference in our model compared to Sukumaran’s model is that we assume that the catabolic antibody clearance is independent of the DAR, which is supported by our observation that PK of DSTA4637A TAb was comparable with that of MSTA3842A. In Sukumaran’s model, the model estimated less than ~5% increase in clearance of DAR0 compared to an unconjugated antibody for anti-STEAP1-vc-MMAE ADC.Citation10 Furthermore, the DAR-dependent clearance for ADC species with DAR 1 and 2 were increased by ~6% and ~8%, respectively, compared to unconjugated antibody, whereas significant increases in estimated clearance occurred at higher DAR (4–8). The above data suggested that DAR-dependent antibody clearance could be negligible for ADC species with low DARs (i.e., DAR ≤2).Citation10 Sun et al.Citation28 have reported that there was similar PK and tissue distribution of an ADC in a cleavable linker format with DAR≤ 5.8. In addition, without PK characterization of individual DAR species, we may not have sufficient data to estimate DAR-dependent antibody clearance.
Model-informed drug development (MIDD) improves our understanding of molecule behaviors such as complicated PK characteristics. In our study, the same structural model was able to describe the observed DSTA4637A TAb and DSTA4637A conjugate (measured as ac-dmDNA31) concentration-time profiles in mice, rats and monkeys very well. The final estimated parameters from the integrated DSTA4637A PK model quantified the contribution of proteolytic degradation and deconjugation in clearing the DSTA4637A conjugate (measured as antibody-conjugated dmDNA31, ac-dmDNA31), and suggested a similar drug deconjugation process across all three preclinical species. This adds an important insight in understanding the complex PK behavior of DSTA4637A, and lays the foundation for the use of the preclinical estimate of deconjugation rate constant (kDC) for human PK predictions. The in vitro plasma stability study demonstrated that overall plasma stability profiles of DSTA4637A (DSTA4637A TAb and ac-dmDNA31 concentration profiles over 96 hours in vitro incubation) were comparable across mouse, rat, monkey, and human (data not shown). These data further support a similar drug deconjugation process across preclinical species and humans.
Another key aspect of MIDD is the translational prediction of human PK from preclinical PK. Non-human primate, usually the cynomolgus monkey, is the most relevant species for antibody-based therapeutics preclinical PK translational because 1) Most therapeutic mAbs bind to nonhuman primate antigens more often than to rodent antigens due to the greater sequence homology observed between nonhuman primates and humans; and 2) The binding epitope, binding affinity to antigen, binding affinity to the neonatal Fc receptor (FcRn), tissue cross-reactivity profiles and disposition and elimination pathways of mAbs are similar in nonhuman primates and humans. Several retrospective analyses showed that the CL and V for a mAb with linear PK can be predicted reasonably well by allometric scaling with a fixed scaling exponent using cynomolgus monkey PK data only.Citation11,Citation29–Citation32 However, their recommendation on the allometric exponent for CL is different: either 0.75, 0.85, or 0.9. The value of 1 is always preferred for volumes of distribution. TAC PK is driven by its mAb component, so it is reasonable to only use monkey data for human PK prediction as well. To date, there have been very few examples published on ADC interspecies scaling. Li et al.Citation33 and Haddish‐Berhane et al.Citation34 proposed using 1 as an allometric exponent on CL for ADC based on monkey data only. Bouillon-Pichault et al.Citation35 reported that 1 as an allometric exponent on CL worked well for one ADC SAR566658, while 0.75 worked better for another ADC SAR3419. Sukumaran et al.Citation10 used an exponent of 0.85 for CL and CLD and an exponent of 1 for other parameters (distribution parameters, DAR-dependent antibody clearance, and drug deconjugation rate constants) for anti-STEAP1-vc-MMAE human PK projection.
Here, the integrated PK model and parameter estimates from the monkey PK data were used for predicting human PK profiles for DSTA4637S and the model-based PK projections were compared with measured DSTA4637S TAb and ac-dmDNA31 concentrations from Phase 1 dose escalation study in healthy volunteers. Body weight-based allometric scaling based on monkey data only was used for predicting human PK with an exponent of 0.75 for CL1 and CLD and an exponent of 1 for Vc and Vp. There is no scaling of the de-conjugation rate as discussed above. The model projections matched well with the observed clinical data from the Phase 1 dose escalation study, which suggests that the model may be useful for translational clinical predictions. Scaling of both CL1 and CLD using either the standard exponent of 0.85 associated with conventional antibodiesCitation11 or of 1 associated with ADCsCitation33,Citation34 was also evaluated, but yielded poor fits (data not shown).
Concentrations during the initial distribution phase of DSTA4637S TAb and throughout the time-course for ac-dmDNA31 in healthy volunteers were under-predicted by the translated model, although they were still in an acceptable predicted range (within twofold error, and Supplementary Table 1). This could possibly reflect additional deconjugation processes for DSTA4637S in healthy volunteers. Of note, disease status could affect the PK of DSTA4637S in patients with S. aureus bacteremia, such as deconjugation process, clearance and volume of distribution, which are the most important parameters affecting dmDNA31 exposure as shown in the sensitivity analysis. The current ongoing clinical study in patients (NCT03162250) will provide more insights on the translation of DSTA4637S PK from preclinical to clinical studies.
Our current integrated PK model only describes PK for ac-dmDNA31 and DSTA4637A TAb (including DAR species with 0, 1, and 2), but could be extended to describe unconjugated dmDNA31 PK as well. Such an extension could lead to a further understanding of the elimination pathways for DSTA4637A conjugate and unconjugated dmDNA31 release in vivo. In the future, when a better understanding of dmDNA31 PK, especially metabolism, becomes available, the unconjugated dmDNA31 dynamic could be incorporated into the current model.
In conclusion, we characterized the complex PK of DSTA4637A in rats and cynomolgus monkeys and assessed the impact of drug conjugation on its PK. In rats and monkeys, following intravenous administration of a single dose of DSTA4637A, systemic concentration-time profiles of both DSTA4637A TAb and DSTA4637A conjugate (measured as antibody-conjugated dmDNA31, ac-dmDNA31) were bi-exponential and characterized by a short distribution phase and a long elimination phase as expected for a mAb-based therapeutic. Systemic exposures of both DSTA4637A TAb and ac-dmDNA31 were dose proportional over the dose range tested. Unconjugated dmDNA31 plasma concentrations were low (<4 ng/mL) in every study regardless of doses. The observed data indicated a minimal impact of the conjugation process on the PK of the unconjugated anti-S. aureus antibody in rats and monkeys. All these observations are consistent with the PK behavior we previously observed in mice.Citation8 An integrated PK model for two analytes (DSTA4637A TAb and ac-dmDNA31) was successfully developed and was able to describe DSTA4637A PK in mice, rats and monkeys. Human PK profiles for DSTA4637S were predicted reasonably well using this model and parameter estimates from the cynomolgus monkey PK data only with a scaling exponent of 0.75 on clearance and 1 on volume of distribution. Based on our findings, a more systemic examination of ADCs/TDCs interspecies scaling is warranted.
Materials and methods
Test material
DSTA4637A and MSTA3852A were generated at Genentech, Inc. (South San Francisco, CA) and were provided in liquid form. Before their use in the study, both materials were stored in a refrigerator set to maintain a temperature range of 2°C to 8°C.
DSTA4637A and MSTA3852A pharmacokinetic study in Sprague–Dawley rats
The PK study in SD rats was approved by the Institutional Animal Care and Use Committee at Genentech, Inc. A total of 27 female SD rats were assigned to six groups (n = 6 animals/group for DSTA4637A, n = 3 animals/group for MSTA3852A). Animals in Groups 1, 2, and 3 received a single IV injection of 50, 25 or 1 mg/kg of DSTA4637A, respectively. Animals in Group 4, 5, and 6 received a single IV injection of 50, 25, or 1 mg/kg of MSTA3852A (unconjugated antibody). Blood samples were collected from each rat at the following time points: 10 minutes, 1 and 6 hours, and 1, 2, 3, 7, 10, 14, 21, and 29 days post-dose. At selected times throughout the study, serum samples were collected and assayed for DSTA4637A TAb or MSTA3852A using a hybrid affinity-capture LC-MS/MS method. Plasma samples were collected and assayed for DSTA4637A conjugate (measured as antibody-conjugated dmDNA31 [ac-dmDNA31]) using another hybrid affinity-capture liquid chromatography-mass spectrometry (LC-MS)/MS method and unconjugated dmDNA31 concentrations by a LC-MS/MS method.
DSTA4637A and MSTA3852A pharmacokinetic study in cynomolgus monkeys
The PK study in cynomolgus monkeys was approved by the Institutional Animal Care and Use Committee and conducted at Covance Laboratories (Madison, WI). A total of 15 male cynomolgus monkeys were assigned to five groups (n = 3 animals/group). Animals in Group 1 received a single IV injection of vehicle. Animals in Groups 2, 3, and 4 received a single IV injection of 1, 15, or 150 mg/kg of DSTA4637A, respectively. Animals in Group 5 received a single IV injection of 50 mg/kg of MSTA3852A (unconjugated antibody). Blood samples were collected at following time points for PK measurements: pre-dose, 5 minutes and 2 and 6 hours post-dose, and 1, 2, 3, 7, 10, 14, 21, 28, 35, and 42 days post-dose. ADA samples were collected from animals in Groups 2 through 5 via the femoral vein for pre-dose and 14, 28, and 42 days post-dose.
At selected times throughout the study, serum samples were collected and assayed for DSTA4637A TAb or MSTA3852A using an affinity-capture LC-MS/MS method. Plasma samples were collected and assayed for DSTA4637A conjugate (measured as antibody-conjugated dmDNA31 [ac-dmDNA31]) using another affinity-capture LC-MS/MS method, and unconjugated dmDNA31 by an LC-MS/MS method.
Serum DSTA4637A TAb and MSTA3852A assay
DSTA4637A TAb (all drug to antibody ratios [DARs] of DSTA4637A including fully conjugated, partially deconjugated, and fully deconjugated antibodies, the molecular weight of DSTA4637A TAb is 149 kDa) concentrations and MSTA3852A concentrations were measured in monkey or rat serum samples by a generic hybrid ligand binding LC-MS/MS assay. Protein A-coated magnetic beads were used to capture DSTA4637A TAb or MSTA3852A from serum followed by enzymatic digestion. A representative signature peptide, unique to human antibodies and not present in monkey or rat antibodies, was used as a surrogate of the antibody concentration originating from DSTA4637A or MSTA3852A and analyzed using LC/MS/MS (i.e., multiple reaction monitoring). The lower limit of quantitation (LLOQ) of the assay was 1 μg/mL.
Plasma DSTA4637A conjugate assay
DSTA4637A conjugate (measured as antibody-conjugated dmDNA31 [ac-dmDNA31], the total concentration of dmDNA31 conjugated to the antibody, the molecular weight of dmDNA31 is 926 Da) was determined in monkey lithium-heparin or rat lithium-heparin plasma samples following the method described below. This procedure used Protein A resin to affinity-capture DSTA4637A from plasma, followed by the enzyme-mediated release of dmDNA31 from DSTA4637A. The released dmDNA31 was analyzed using LC-MS/MS with electrospray ionization. The LLOQ of the assay was 0.464 ng/mL.
Plasma unconjugated dmDNA31 assay
Unconjugated dmDNA31 was measured in monkey lithium-heparin or rat lithium-heparin plasma samples using LC-MS/MS with electrospray ionization after extraction by protein precipitation. This method measures dmDNA31 in circulation that is not conjugated to the antibody through the MC-vc-PAB linker. The LLOQ of the assay was 0.185 ng/mL.
Anti-drug antibody assay
The presence of ADAs was determined in cynomolgus monkey serum by a bridging immunoassay. Serum samples are co-incubated with biotin-DSTA4637A and digoxigenin-DSTA4637A conjugated reagents to capture ADAs in the sample, followed by immobilization of the complexes in streptavidin-coated plates and detection with a murine anti-digoxin mAb labeled with horseradish peroxidase (Jackson ImmunoResearch, Inc. (West Grove, PA, USA), cat.no. 200-032-156).
Pharmacokinetic data analysis
Non-compartmental pharmacokinetic analysis
Nominal sample collection times and dosing were used in the data analysis. Concentrations that were below LLOQ were interpreted to be missing for graphical and summary presentation and were excluded from the datasets used to estimate individual PK parameters. Individual DSTA4637A TAb, MSTA3852A and ac-dmDNA31 concentration-time data in rats and monkeys were analyzed using a non-compartmental model by Phoenix® WinNonlin® (Model 201, WinNonlin, version 5.2.1; Pharsight Corporation; Mountain View, CA). Individual concentration-time profiles in rats and cynomolgus monkeys were used to estimate the following PK parameters for DSTA4637A TAb, MSTA3852A and ac-dmDNA31: maximum concentration observed (Cmax), area under the concentration-time curve extrapolated to infinity (AUC0-inf), clearance (CL), volume of distribution at steady state (VSS), and terminal half-life (t1/2, λz). Since individual concentration-time profiles were determined for each animal, PK parameter estimates were provided as the average along with the standard deviation (SD) here.
Semi-mechanistic pharmacokinetic modeling
depicts the schematic of the integrated platform PK model developed for DSTA4637A. The model consists of 3 distinct species: DARi representing DSTA4637A at DAR values of i = 0, 1 and 2. Consistent with PK profiles for mAbs and other ADCs/TDCs, each species in the model is represented using two compartments, a central and a peripheral compartment. All DSTA4637A species, regardless of DAR, are assumed to share the same distribution parameter values, i.e., volume of distribution of central compartment (VC), volume of distribution of peripheral compartment (VP) and distribution clearance (CLD). A sequential de-conjugation of drug from the DSTA4637A is represented, resulting in the conversion of higher DAR species to lower DAR species in the central compartment. The de-conjugation rate (rDCi) of each DAR species is represented as an irreversible first-order process with a rate constant proportional to its DAR (kDC,i = kDC × i), such that, for DAR of i = 0–2:
Systemic clearance, CL1, for each DAR species is modeled as a DAR-independent process. Thus, the differential equations describing the central and peripheral compartments of the different species (in amount as molar/kg) at DAR of i = 0–2, DARi,C and DARi,P respectively, with initial conditions, are represented as:
At time = 0, all DSTS4637A are DAR2 species. Of note, if i = 2, then the first term of the right hand of Equation (2) will be 0 since there is no DAR3 species.
Total antibody molar concentrations for DSTA4637A and ac-dmDNA31are calculated as:
The model described above was used to fit DSTA4673A TAb and ac-dmDNA31 concentration data in mouse, rats and monkeys separately. Model development, data fitting, parameter estimation and simulations were performed in SimBiology® (The Mathworks, Inc., Natick, MA). Residual variability was modeled using the additive (a) plus proportional (b) variance model. The goodness-of-fit was assessed by model convergence, visual inspection of the fitted curves, graphical evaluation and standard error of the estimated parameters.
A local sensitivity analysis of the final model output to model parameters was performed by assessing the percent change of AUC values of DSTA4637A TAb and ac-dmDNA31 with ± 10% alteration in a given model parameter values (Equation 11), using the software Berkeley Madonna (version 8.3.11).
AUCSIM refers to the AUC value calculated with the final estimated set of parameters, and AUC±10% is the AUC obtained following a 10% increase or decrease in a given parameter value. The analysis was performed with simulations at the IV dose of 1mg/kg DSTA4637A in monkeys.
Model-based clinical translation for DSTA4637A PK
The integrated semi-mechanistic PK model and parameter estimates from the monkey PK data were used for predicting human PK profiles for DSTA4637S TAb and ac-dmDNA31 concentrations in humans. Body weight-based allometric scaling based on monkey data only was used for predicting human PK parameters. A scaling exponent of 0.75 was used to estimate human CL1, and human CLD. A scaling exponent of 1 was used to estimate Vc and Vp in humans. A typical body weight of 3.5 kg and 70 kg for monkeys and humans, respectively, was used. There is no scaling of DAR-dependent deconjugation rate constant (kDC). The projected human PK parameters were then used to simulate DSTA4637S TAb and ac-dmDNA31 concentration time-profile in humans by NONMEM (version 7.3.0, ICON Development Solutions, Ellicott City, MD). For the simulations (n = 1000), the inter-individual variability on CL1 and V1 was assumed to be 30% based on what is generally observed in humans for mAbs.Citation16
Disclosure of Potential Conflicts of Interest
No potential conflicts of interest were disclosed.
Supplemental Material
Download MS Word (1.7 MB)Acknowledgments
The authors would like to thank Anshin BioSolutions for editing this manuscript. The anti-S.aureus antibody was discovered in collaboration with Symphogen. The linker was licensed from Seattle Genetics.
Supplemental Material
Supplemental data for this article can be accessed on the publisher’s website.
Additional information
Funding
References
- DeLeo FR, Chambers HF. Reemergence of antibiotic-resistant Staphylococcus aureus in the genomics era. J Clin Invest. 2009;119:2464–674. doi:10.1172/JCI38226.
- van Hal SJ, Jensen SO, Vaska VL, Espedido BA, Paterson DL, Gosbell IB. Predictors of mortality in Staphylococcus aureus Bacteremia. Clin Microbiol Rev. 2012;25:362–86. doi:10.1128/CMR.05022-11.
- Tong SY, Davis JS, Eichenberger E, Holland TL, Fowler VG Jr. Staphylococcus aureus infections: epidemiology, pathophysiology, clinical manifestations, and management. Clin Microbiol Rev. 2015;28:603–61. doi:10.1128/CMR.00134-14.
- Thwaites GE, Gant V. Are bloodstream leukocytes Trojan Horses for the metastasis of Staphylococcus aureus?. Nat Rev Microbiol. 2011;9:215–22. doi:10.1038/nrmicro2508.
- Lehar SM, Pillow T, Xu M, Staben L, Kajihara KK, Vandlen R, DePalatis L, Raab H, Hazenbos WL, Morisaki JH, et al. Novel antibody-antibiotic conjugate eliminates intracellular S. aureus. Nature. 2015;527:323–28. doi:10.1038/nature16057.
- Mariathasan S, Tan MW. Antibody-antibiotic conjugates: A novel therapeutic platform against bacterial infections. Trends Mol Med. 2017;23:135–49. doi:10.1016/j.molmed.2016.12.008.
- Shen BQ, Xu K, Liu L, Raab H, Bhakta S, Kenrick M, Parsons-Reponte KL, Tien J, Yu S-F, Mai E, et al. Conjugation site modulates the in vivo stability and therapeutic activity of antibody-drug conjugates. Nat Biotechnol. 2012;30:184–89. doi:10.1038/nbt.2108.
- Zhou C, Lehar S, Gutierrez J, Rosenberger CM, Ljumanovic N, Dinoso J, Koppada N, Hong K, Baruch A, Carrasco-Triguero M, et al. Pharmacokinetics and pharmacodynamics of DSTA4637A: A novel THIOMAB antibody antibiotic conjugate against Staphylococcus aureus in mice. mAbs. 2016;8:1612–19. doi:10.1080/19420862.2016.1229722.
- Kaur S, Xu K, Saad OM, Dere RC, Carrasco-Triguero M. Bioanalytical assay strategies for the development of antibody-drug conjugate biotherapeutics. Bioanalysis. 2013;5:201–26. doi:10.4155/bio.12.299.
- Sukumaran S, Zhang C, Leipold DD, Saad OM, Xu K, Gadkar K, Samineni D, Wang B, Milojic-Blair M, Carrasco-Triguero M, et al. Development and translational application of an integrated, mechanistic model of antibody-drug conjugate pharmacokinetics. Aaps J. 2017;19:130–40. doi:10.1208/s12248-016-9993-z.
- Deng R, Iyer S, Theil FP, Mortensen DL, Fielder PJ, Prabhu S. Projecting human pharmacokinetics of therapeutic antibodies from nonclinical data: what have we learned? mAbs. 2011;3:61–66. doi:10.4161/mabs.3.1.13799.
- Hotzel I, Theil FP, Bernstein LJ, Prabhu S, Deng R, Quintana L, Lutman J, Sibia R, Chan P, Bumbaca D, et al. A strategy for risk mitigation of antibodies with fast clearance. mAbs. 2012;4:753–60. doi:10.4161/mabs.22189.
- Sukumaran S, Gadkar K, Zhang C, Bhakta S, Liu L, Xu K, Raab H, Yu S-F, Mai E, Fourie-O’Donohue A, et al. Mechanism-based pharmacokinetic/pharmacodynamic model for THIOMAB drug conjugates. Pharm Res. 2015;32:1884–93. doi:10.1007/s11095-014-1582-1.
- Peck M, Rothenberg ME, Deng R, Lewin-Koh N, She G, Kamath AV, Carrasco-Triguero M, Saad O,Castro A,Teufel L, et al. A Phase 1, Randomized, Single-Ascending-Dose Study To Investigate the Safety, Tolerability, and Pharmacokinetics of DSTA4637S, an Anti-Staphylococcus aureus Thiomab Antibody-Antibiotic Conjugate, in Healthy Volunteers. Antimicrob Agents Chemother. 2019 May 24;63(6). pii: e02588-18. doi:10.1128/AAC.02588-18
- Deng R, Jin F, Prabhu S, Iyer S. Monoclonal antibodies: what are the pharmacokinetic and pharmacodynamic considerations for drug development? Expert Opin Drug Metab Toxicol. 2012;8:141–60. doi:10.1517/17425255.2012.643868.
- Ryman JT, Meibohm B. Pharmacokinetics of monoclonal antibodies. Cpt. 2017;6:576–88.
- Kamath AV, Iyer S. Challenges and advances in the assessment of the disposition of antibody-drug conjugates. Biopharm Drug Dispos. 2016;37:66–74. doi:10.1002/bdd.1957.
- Hamblett KJ, Senter PD, Chace DF, Sun MM, Lenox J, Cerveny CG, Kissler KM, Bernhardt SX, Kopcha AK, Zabinski RF, et al. Effects of drug loading on the antitumor activity of a monoclonal antibody drug conjugate. Clin Cancer Res. 2004;10:7063–70. doi:10.1158/1078-0432.CCR-04-0789.
- Kamath AV, Iyer S. Preclinical pharmacokinetic considerations for the development of antibody drug conjugates. Pharm Res. 2015;32:3470–79. doi:10.1007/s11095-014-1584-z.
- Lu D, Girish S, Gao Y, Wang B, Yi JH, Guardino E, Samant M, Cobleigh M, Rimawi M, Conte P, et al. Population pharmacokinetics of trastuzumab emtansine (T-DM1), a HER2-targeted antibody-drug conjugate, in patients with HER2-positive metastatic breast cancer: clinical implications of the effect of covariates. Cancer Chemother Pharmacol. 2014;74:399–410. doi:10.1007/s00280-014-2500-2.
- Suri A, Mould DR, Liu Y, Jang G, Venkatakrishnan K. Population PK and exposure-response relationships for the antibody-drug conjugate brentuximab vedotin in CTCL patients in the phase III ALCANZA study. Clin Pharmacol Ther. 2018;104:989–99. doi:10.1002/cpt.1037.
- Bender B, Leipold DD, Xu K, Shen BQ, Tibbitts J, Friberg LE. A mechanistic pharmacokinetic model elucidating the disposition of trastuzumab emtansine (T-DM1), an antibody-drug conjugate (ADC) for treatment of metastatic breast cancer. Aaps J. 2014;16:994–1008. doi:10.1208/s12248-014-9618-3.
- Lu D, Jin JY, Girish S, Agarwal P, Li D, Prabhu S, Dere RC, Saad OM, Nazzal D, Koppada N, et al. Semi-mechanistic multiple-analyte pharmacokinetic model for an antibody-drug-conjugate in cynomolgus monkeys. Pharm Res. 2015;32:1907–19. doi:10.1007/s11095-014-1585-y.
- Shah DK, Haddish-Berhane N, Betts A. Bench to bedside translation of antibody drug conjugates using a multiscale mechanistic PK/PD model: a case study with brentuximab-vedotin. J Pharmacokinet Pharmacodyn. 2012;39:643–59. doi:10.1007/s10928-012-9276-y.
- Khot A, Tibbitts J, Rock D, Shah DK. Development of a translational physiologically based pharmacokinetic model for antibody-drug conjugates: A case study with T-DM1. Aaps J. 2017;19:1715–34. doi:10.1208/s12248-017-0131-3.
- Lu D, Gibiansky L, Agarwal P, Dere RC, Li C, Chu YW, et al. Integrated two-analyte population pharmacokinetic model for antibody-drug conjugates in patients: implications for reducing pharmacokinetic sampling. CPT Pharmacometrics Syst Pharmacol. 2016;5:665–73.
- Xu K, Liu L, Saad OM, Baudys J, Williams L, Leipold D, Shen B, Raab H, Junutula JR, Kim A, et al. Characterization of intact antibody-drug conjugates from plasma/serum in vivo by affinity capture capillary liquid chromatography-mass spectrometry. Anal Biochem. 2011;412:56–66. doi:10.1016/j.ab.2011.01.004.
- Sun X, Ponte JF, Yoder NC, Laleau R, Coccia J, Lanieri L, Qiu Q, Wu R, Hong E, Bogalhas M, et al. Effects of drug-antibody ratio on pharmacokinetics, biodistribution, efficacy, and tolerability of antibody-maytansinoid conjugates. Bioconjug Chem. 2017;28:1371–81. doi:10.1021/acs.bioconjchem.7b00062.
- Dong JQ, Salinger DH, Endres CJ, Gibbs JP, Hsu CP, Stouch BJ, Hurh E, Gibbs MA. Quantitative prediction of human pharmacokinetics for monoclonal antibodies: retrospective analysis of monkey as a single species for first-in-human prediction. Clin Pharmacokinet. 2011;50:131–42. doi:10.2165/11537430-000000000-00000.
- Ling J, Zhou H, Jiao Q, Davis HM. Interspecies scaling of therapeutic monoclonal antibodies: initial look. J Clin Pharmacol. 2009;49:1382–402. doi:10.1177/0091270009337134.
- Wang J, Iyer S, Fielder PJ, Davis JD, Deng R. Projecting human pharmacokinetics of monoclonal antibodies from nonclinical data: comparative evaluation of prediction approaches in early drug development. Biopharm Drug Dispos. 2016;37:51–65. doi:10.1002/bdd.1952.
- Wang W, Prueksaritanont T. Prediction of human clearance of therapeutic proteins: simple allometric scaling method revisited. Biopharm Drug Dispos. 2010;31:253–63.
- Li C, Zhang C, Deng R, Leipold D, Li D, Latifi B, Gao Y, Zhang C, Li Z,Miles D, et al. Prediction of human pharmacokinetics of antibody-drug conjugates from nonclinical data. Clin Transl Sci. 2019 May 22. doi:10.1111/cts.12649.
- Haddish-Berhane N, Shah DK, Ma D, Leal M, Gerber HP, Sapra P, Barton HA, Betts AM. On translation of antibody drug conjugates efficacy from mouse experimental tumors to the clinic: a PK/PD approach. J Pharmacokinet Pharmacodyn. 2013;40:557–71. doi:10.1007/s10928-013-9329-x.
- Bouillon-Pichault M, Brillac C, Amara C, Nicolazzi C, Fagniez N, Fau JB, Koiwai K, Ziti-Ljajic S, Veyrat-Follet C. Translational model-based strategy to guide the choice of clinical doses for antibody-drug conjugates. J Clin Pharmacol. 2017;57:865–75. doi:10.1002/jcph.869.