Abstract
Actin filaments (F-actin) are the major structural component of excitatory synapses. In excitatory synapses, F-actin is enriched in presynaptic terminals and in postsynaptic dendritic spines, and actin dynamics – the spatiotemporally controlled assembly and disassembly of F-actin – have been implicated in pre- and postsynaptic physiology, additionally to their function in synapse morphology. Hence, actin binding proteins that control actin dynamics have moved into the focus as regulators of synapse morphology and physiology. Actin depolymerizing proteins of the ADF/cofilin family are important regulators of actin dynamics, and several recent studies highlighted the relevance of cofilin 1 for dendritic spine morphology, trafficking of postsynaptic glutamate receptors, and synaptic plasticity. Conversely, almost nothing was known about the synaptic function of ADF, a second ADF/cofilin family member present at excitatory synapses, and it remained unknown whether ADF/cofilin is relevant for presynaptic physiology. To comprehensively characterize the synaptic function of ADF/cofilin we made use of mutant mice lacking either ADF or cofilin 1 or both proteins. Our analysis revealed presynaptic defects (altered distribution and enhanced exocytosis of synaptic vesicles) and behavioral abnormalities reminiscent of attention deficit-hyperactivity disorder in double mutants that were not present in single mutants. Hence, by exploiting gene-targeted mice, we demonstrated the relevance of ADF for excitatory synapses, and we unraveled novel functions for ADF/cofilin in presynaptic physiology and behavior.
Actin-binding proteins (ABPs) of the ADF/cofilin family are crucial regulators of actin dynamics (for review seeCitation1), which can speed up actin dynamics by accelerating the dissociation of actin monomers (G-actin) from the minus end of actin filaments (F-actin) and by severing F-actin.Citation2,3 Depending on the local G-actin concentration and on the activity of ABPs that promote actin polymerization, ADF/cofilin-mediated filament severing can either result in net assembly or disassembly of F-actin.Citation4 Moreover, at high concentrations, ADF/cofilin can promote F-actin assembly by nucleating new and by stabilizing preexisting filaments.Citation5 Actin binding of ADF/cofilin is inhibited upon phosphorylation of a conserved serine residue at position 3 (Ser3), and LIM kinases (LIMK) have been recognized as important regulators of ADF/cofilin activity.Citation6 Indeed, analysis of LIMK1 mutant mice initially implicated ADF/cofilin in synapse physiology and suggested a role in dendritic spine morphology and synaptic plasticity.Citation7 The mammalian ADF/cofilin family consist of 3 proteins, namely cofilin 1 (non-muscle cofilin, n-cofilin), cofilin 2 (muscle-cofilin, m-cofilin), and ADF (actin-depolymerizing factor, destrin). While cofilin 1 and ADF show a broad tissue distribution, are both broadly expressed in the brain and present at excitatory synapses,Citation8-11 cofilin 2 is the major ADF/cofilin form in muscle cells and mutations in the human cofilin 2 gene CFL2 have been associated with nemaline myopathies.Citation12-15
In agreement with the postsynaptic defects described for LIMK1 mutant mice,Citation7 several studies demonstrated a crucial role of cofilin 1 in postsynaptic mechanisms (for review seeCitation16). These studies revealed that cofilin 1 controls i) actin dynamics in dendritic spines, ii) dendritic spine density and morphology, iii) trafficking of α-amino-3-hydroxy-5-methyl-4-isoxazolepropionic acid (AMPA) receptors, and ultimately iv) long-term potentiation (LTP) and long-term depression (LTD),Citation10,17-22 2 forms of synaptic plasticity that are widely considered as major cellular mechanisms underlying learning and memory.Citation23 Together, these studies let cofilin 1 emerge as an important regulatory factor that controls structural und functional aspects of synaptic plasticity via synaptic actin dynamics (Fig. 1). Moreover, they suggest a role in learning and memory, which indeed has been demonstrated by behavioral analysis of cofilin 1 mutant mice that performed worse in paradigms of associative learning or by accelerated extinction of aversive memory upon increased activity of ADF/cofilin in the rat infralimbic cortex.Citation10,21
ADF shares very similar biochemical properties with cofilin 1 (for review, seeCitation24), and, like cofilin 1, it was found broadly expressed in the adult brain and present in presynaptiC-terminals and in dendritic spines.Citation9,11 However, while the relevance of cofilin 1 for synapse physiology and behavior is well established,Citation16 relatively little is known about the role of ADF, because in vitro studies almost exclusively focused on cofilin 1, and ADF mutant mice did not display synaptic or behavioral defects.Citation11 However, we found elevated cofilin 1 levels in synaptic structures from ADF mutants,Citation11 and we therefore hypothesized i) functional redundancy of ADF and cofilin 1 in the mouse brain and ii) that cofilin 1 can compensate for the loss of ADF in excitatory synapses. To test these hypotheses and to comprehensively characterize the synaptic function of ADF/cofilin, we generated double mutant mice lacking both ADF and cofilin 1. Indeed, these mutants displayed synaptic defects that were not present in ADF or cofilin 1 single mutants, thereby validating our aforementioned working hypotheses.
Compared to cofilin 1 single mutants, dendritic spine size was strongly increased in the hippocampus and striatum of double mutants,Citation25,26 thus demonstrating that ADF is relevant for dendritic spine morphology, too. Moreover, our data let us suggest that cofilin 1 can fully compensate for the loss of ADF at excitatory synapses, while ADF can only partially rescue cofilin 1s inactivation. Notably, a role of ADF in spine morphology and a redundant function of ADF and cofilin 1 in dendritic spine morphology has just recently been confirmed in rat hippocampal slices.Citation22 While our initial characterization of double mutant mice revealed a role of ADF in spine morphology, its function in dendritic spine actin dynamics, AMPA receptor trafficking or synaptic plasticity has not been examined yet, but will be tackled in future studies.
The analysis of double mutants not only revealed a redundant postsynaptic function for ADF and cofilin 1, but also demonstrated their relevance for presynaptic physiology.Citation25,26 In hippocampal synapses of double mutants, we found an altered distribution of synaptic vesicles, including an elevated number of synaptic vesicles attached to the active zone.Citation25 Consequently, synaptic vesicle exocytosis was increased in hippocampal synapses from these mice, and, similarly, we found increased glutamate release in the striatum. Such defects were not present in ADF or cofilin 1 single mutants.Citation10,11,25,26 Hence, cofilin 1 emerged as the predominant ADF/cofilin form in dendritic spines, while ADF and cofilin 1 are apparently equally important for presynaptic physiology. Together, our analysis of double mutant mice unraveled a novel function for ADF/cofilin in neurotransmitter release, which is in line with presynaptic defects upon inactivation of upstream regulators such as RhoB, ROCK2, PAK, LIMK1 or slingshot.Citation7,27-30 Based on its relevance for actin dynamics, it is very likely that ADF/cofilin controls neurotransmitter release via regulating the presynaptic actin cytoskeleton, although this has not been demonstrated experimentally yet. However, in line with this idea, a pivotal role of actin dynamics in vesicle exocytosis has been demonstrated by exploiting actin drugs (for review seeCitation31) or by manipulating the activity of actin dynamics regulators such as profilin2 or DRR1.Citation32-34
Interestingly, double mutant mice displayed behavioral alterations that were not present in single mutants, again providing compelling evidences for overlapping functions of ADF or cofilin 1 in the mouse brain.Citation26 These alterations included strongly increased locomotor activity, working memory deficits, impulsivity and a paradoxical calming effect of pharmacological treatment with psychostimulants such as methylphenidate, thus closely modeling typical clinical symptoms of attention deficit-hyperactivity disorder (ADHD) for which methylphenidate is widely prescribed in clinical practice.Citation35 Hence, our analysis of double mutant mice revealed that defects in neuronal actin dynamics can cause behavioral abnormalities reminiscent of human psychiatric diseases, thereby suggesting defective neuronal actin dynamics as a pathomechanism in mental disorders. Indeed, several genes that have been associated with human psychiatric diseases such as schizophrenia (DISC1, SHANK2), autism spectrum disorders (SHANK2, SHANK3, FMRP) or ADHD (GIT1) have just recently been linked to neuronal actin dynamics.Citation36-42 Future studies will show whether defective neuronal actin dynamics caused by mutations in and/or dysregulation of ABPs can be causative for mental disorders in humans, and whether modulating the activity of ABPs will allow novel treatment strategies.
Figure 1. Schematic diagram illustrating the functions of ADF and cofilin 1 at excitatory synapses as deduced from the analyses of single and double mutant mice.Citation10,11,25,26 In ADF mutant mice, the morphology of excitatory synapses is unchanged and these mutants do not display any defects in pre- (distribution, recruitment or exocytosis of synaptic vesicles) or postsynaptic mechanisms (LTP, LTD). Conversely, dendritic spine profiles and the postsynaptic density (PSD) are both enlarged in cofilin 1 mutants, and these morphological changes are associated with reduced LTP, absence of LTD, and impaired extra-synaptic mobility of AMPA receptors (indicated by a thinner double arrow compared to the control synapse). Like in ADF mutants, presynaptic mechanisms are unchanged in cofilin 1 mutants. Compared to single mutants, dendritic spine size is further increased in double mutants lacking both ADF and cofilin 1, and double mutants display presynaptic defects that are not present in single mutants including an altered distribution, a reduced recruitment (indicated by the thinner arrow compared to control and single mutant synapses) and elevated exocytosis of synaptic vesicles. Whether AMPA receptor mobility is affected in ADF and/or double mutants, or whether LTP and LTD are impaired in double mutants has not been tested experimentally yet.
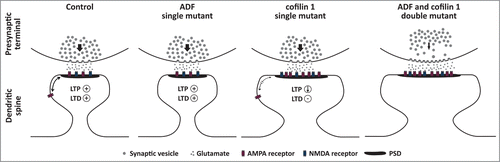
Disclosure of Potential Conflicts of Interest
No potential conflicts of interest were disclosed.
Funding
This work was supported by a Research grant (24/2014 MR) of the University Medical Center Giessen and Marburg (UKGM).
References
- Hild G, Kalmar L, Kardos R, Nyitrai M, Bugyi B. The other side of the coin: functional and structural versatility of ADF/cofilins. Eur J Cell Biol 2014; 93:238-51; PMID:24836399; http://dx.doi.org/10.1016/j.ejcb.2013.12.001
- Blanchoin L, Pollard TD. Mechanism of interaction of Acanthamoeba actophorin (ADF/Cofilin) with actin filaments. J Biol Chem 1999; 274:15538-46; PMID:10336448; http://dx.doi.org/10.1074/jbc.274.22.15538
- Maciver SK, Zot HG, Pollard TD. Characterization of actin filament severing by actophorin from Acanthamoeba castellanii. J Cell Biol 1991; 115:1611-20; PMID:1757465; http://dx.doi.org/10.1083/jcb.115.6.1611
- Van Troys M, Huyck L, Leyman S, Dhaese S, Vandekerkhove J, Ampe C. Ins and outs of ADF/cofilin activity and regulation. Eur J Cell Biol 2008; 87:649-67; PMID:18499298; http://dx.doi.org/10.1016/j.ejcb.2008.04.001
- Andrianantoandro E, Pollard TD. Mechanism of actin filament turnover by severing and nucleation at different concentrations of ADF/cofilin. Mol Cell 2006; 24:13-23; PMID:17018289; http://dx.doi.org/10.1016/j.molcel.2006.08.006
- Yang N, Higuchi O, Ohashi K, Nagata K, Wada A, Kangawa K, Nishida E, Mizuno K. Cofilin phosphorylation by LIM-kinase 1 and its role in Rac mediated actin reorganization. Nature 1998; 393:809-12; PMID:9655398; http://dx.doi.org/10.1038/31735
- Meng Y, Zhang Y, Tregoubov V, Janus C, Cruz L, Jackson M, Lu WY, MacDonald JF, Wang JY, Falls DL, et al. Abnormal spine morphology and enhanced LTP in LIMK-1 knockout mice. Neuron 2002; 35:121-33; PMID:12123613; http://dx.doi.org/10.1016/S0896-6273(02)00758-4
- Racz B, Weinberg RJ. Spatial organization of cofilin in dendritic spines. Neuroscience 2006; 138:447-56; PMID:16388910; http://dx.doi.org/10.1016/j.neuroscience.2005.11.025
- Bellenchi GC, Gurniak CB, Perlas E, Middei S, Ammassari-Teule M, Witke W. N-cofilin is associated with neuronal migration disorders and cell cycle control in the cerebral cortex. Genes Dev 2007; 21:2347-57; PMID:17875668; http://dx.doi.org/10.1101/gad.434307
- Rust MB, Gurniak CB, Renner M, Vara H, Morando L, Gorlich A, Sassoè-Pognetto M, Banchaabouchi MA, Giustetto M, Triller A, et al. Learning, AMPA receptor mobility and synaptic plasticity depend on n-cofilin-mediated actin dynamics. EMBO J 2010; 29:1889-902; PMID:20407421; http://dx.doi.org/10.1038/emboj.2010.72
- Görlich A, Wolf M, Zimmermann AM, Gurniak CB, Al Banchaabouchi M, Sassoe-Pognetto M, Witke W, Friauf E, Rust MB. N-Cofilin Can Compensate for the Loss of ADF in Excitatory Synapses. PloS One 2011; 6:e26789; PMID:22046357; http://dx.doi.org/10.1371/journal.pone.0026789
- Agrawal PB, Greenleaf RS, Tomczak KK, Lehtokari VL, Wallgren-Pettersson C, Wallefeld W, Laing NG, Darras BT, Maciver SK, Dormitzer PR, et al. Nemaline myopathy with minicores caused by mutation of the CFL2 gene encoding the skeletal muscle actin-binding protein, cofilin-2. Am J Hum Genet 2007; 80:162-7; PMID:17160903; http://dx.doi.org/10.1086/510402
- Ockeloen CW, Gilhuis HJ, Pfundt R, Kamsteeg EJ, Agrawal PB, Beggs AH, Dara Hama-Amin A, Diekstra A, Knoers NV, Lammens M, et al. Congenital myopathy caused by a novel missense mutation in the CFL2 gene. Neuromuscul Disord 2012; 22:632-9; PMID:22560515; http://dx.doi.org/10.1016/j.nmd.2012.03.008
- Gurniak CB, Chevessier F, Jokwitz M, Jönsson F, Perlas E, Richter H, Matern G, Boyl PP, Chaponnier C, Fürst D, et al. Severe protein aggregate myopathy in a knockout mouse model points to an essential role of cofilin2 in sarcomeric actin exchange and muscle maintenance. Eur J Cell Biol 2014; 93:252-266; PMID:24598388; http://dx.doi.org/10.1016/j.ejcb.2014.01.007
- Kremneva E, Makkonen MH, Skwarek-Maruszewska A, Gateva G, Michelot A, Dominguez R, Lappalainen P. Cofilin-2 Controls Actin Filament Length in Muscle Sarcomeres. Dev Cell 2014; 31:215-226; PMID:25373779; http://dx.doi.org/10.1016/j.devcel.2014.09.002
- Rust MB. ADF/cofilin: a crucial regulator of synapse physiology and behavior. Cell Mol Life Sci 2015; 72:3521-9; PMID:26037722; http://dx.doi.org/10.1007/s00018-015-1941-z
- Zhou Q, Homma KJ, Poo MM. Shrinkage of dendritic spines associated with long-term depression of hippocampal synapses. Neuron 2004; 44:749-57; PMID:15572107; http://dx.doi.org/10.1016/j.neuron.2004.11.011
- Morishita W, Marie H, Malenka RC. Distinct triggering and expression mechanisms underlie LTD of AMPA and NMDA synaptic responses. Nat Neurosci 2005; 8:1043-50; PMID:16025109; http://dx.doi.org/10.1038/nn1506
- Hotulainen P, Llano O, Smirnov S, Tanhuanpaa K, Faix J, Rivera C, Lappalainen P. Defining mechanisms of actin polymerization and depolymerization during dendritic spine morphogenesis. J Cell Biol 2009; 185:323-39; PMID:19380880; http://dx.doi.org/10.1083/jcb.200809046
- Gu J, Lee CW, Fan Y, Komlos D, Tang X, Sun C, Yu K, Hartzell HC, Chen G, Bamburg JR, et al. ADF/cofilin-mediated actin dynamics regulate AMPA receptor trafficking during synaptic plasticity. Nat Neurosci 2010; 13:1208-15; PMID:20835250; http://dx.doi.org/10.1038/nn.2634
- Wang Y, Dong Q, Xu XF, Feng X, Xin J, Wang DD, Yu H, Tian T, Chen ZY. Phosphorylation of cofilin regulates extinction of conditioned aversive memory via AMPAR trafficking. J Neurosci 2013; 33:6423-33; PMID:23575840; http://dx.doi.org/10.1523/JNEUROSCI.5107-12.2013
- Bosch M, Castro J, Saneyoshi T, Matsuno H, Sur M, Hayashi Y. Structural and molecular remodeling of dendritic spine substructures during long-term potentiation. Neuron 2014; 82:444-59; PMID:24742465; http://dx.doi.org/10.1016/j.neuron.2014.03.021
- Kessels HW, Malinow R. Synaptic AMPA receptor plasticity and behavior. Neuron 2009; 61:340-50; PMID:19217372; http://dx.doi.org/10.1016/j.neuron.2009.01.015
- Bernstein BW, Bamburg JR. ADF/cofilin: a functional node in cell biology. Trends Cell Biol 2010; 20:187-95; PMID:20133134; http://dx.doi.org/10.1016/j.tcb.2010.01.001
- Wolf M, Zimmermann AM, Gorlich A, Gurniak CB, Sassoe-Pognetto M, Friauf E, Witke W, Rust MB. ADF/Cofilin controls synaptic actin dynamics and regulates synaptic vesicle mobilization and exocytosis. Cereb Cortex 2015; 25:2863-75; PMID:24770705; http://dx.doi.org/10.1093/cercor/bhu081
- Zimmermann AM, Jene T, Wolf M, Gorlich A, Gurniak CB, Sassoe-Pognetto M, Witke W, Friauf E, Rust MB. Attention-Deficit/Hyperactivity Disorder-like Phenotype in a Mouse Model with Impaired Actin Dynamics. Biol Psychiatry 2015; 78:95-106; PMID:24768258; http://dx.doi.org/10.1016/j.biopsych.2014.03.011
- Zhou Z, Meng Y, Asrar S, Todorovski Z, Jia Z. A critical role of Rho-kinase ROCK2 in the regulation of spine and synaptic function. Neuropharmacology 2009; 56:81-9; PMID:18718479; http://dx.doi.org/10.1016/j.neuropharm.2008.07.031
- Yuen EY, Liu W, Kafri T, van Praag H, Yan Z. Regulation of AMPA receptor channels and synaptic plasticity by cofilin phosphatase Slingshot in cortical neurons. J Physiol 2010; 588:2361-71; PMID:20442266; http://dx.doi.org/10.1113/jphysiol.2009.186353
- McNair K, Spike R, Guilding C, Prendergast GC, Stone TW, Cobb SR, Morris BJ. A role for RhoB in synaptic plasticity and the regulation of neuronal morphology. J Neurosci 2010; 30:3508-17; PMID:20203211; http://dx.doi.org/10.1523/JNEUROSCI.5386-09.2010
- Huang W, Zhou Z, Asrar S, Henkelman M, Xie W, Jia Z. p21-Activated kinases 1 and 3 control brain size through coordinating neuronal complexity and synaptic properties. Mol Cell Biol 2011; 31:388-403; PMID:21115725; http://dx.doi.org/10.1128/MCB.00969-10
- Rust MB, Maritzen T. Relevance of presynaptic actin dynamics for synapse function and mouse behavior. Exp Cell Res 2015; 335:165-71; PMID:25579398; http://dx.doi.org/10.1016/j.yexcr.2014.12.020
- Pilo Boyl P, Di Nardo A, Mulle C, Sassoe-Pognetto M, Panzanelli P, Mele A, Kneussel M, Costantini V, Perlas E, Massimi M, et al. Profilin2 contributes to synaptic vesicle exocytosis, neuronal excitability, and novelty-seeking behavior. EMBO J 2007; 26:2991-3002; PMID:17541406; http://dx.doi.org/10.1038/sj.emboj.7601737
- Waites CL, Leal-Ortiz SA, Andlauer TF, Sigrist SJ, Garner CC. Piccolo regulates the dynamic assembly of presynaptic f-actin. J Neurosci 2011; 31:14250-63; PMID:21976510; http://dx.doi.org/10.1523/JNEUROSCI.1835-11.2011
- Schmidt MV, Schulke JP, Liebl C, Stiess M, Avrabos C, Bock J, Wochnik GM, Davies HA, Zimmermann N, Scharf SH, et al. Tumor suppressor down-regulated in renal cell carcinoma 1 (DRR1) is a stress-induced actin bundling factor that modulates synaptic efficacy and cognition. Proc Natl Acad Sci USA 2011; 108:17213-8; PMID:21969592; http://dx.doi.org/10.1073/pnas.1103318108
- Antshel KM, Hargrave TM, Simonescu M, Kaul P, Hendricks K, Faraone SV. Advances in understanding and treating ADHD. BMC Med 2011; 9:72; PMID:21658285; http://dx.doi.org/10.1186/1741-7015-9-72
- Reeve SP, Bassetto L, Genova GK, Kleyner Y, Leyssen M, Jackson FR, Hassan BA. The Drosophila fragile X mental retardation protein controls actin dynamics by directly regulating profilin in the brain. Curr Biol : CB 2005; 15:1156-63; PMID:NOT_FOUND; http://dx.doi.org/10.1016/j.cub.2005.05.050
- Won H, Mah W, Kim E, Kim JW, Hahm EK, Kim MH, Cho S, Kim J, Jang H, Cho SC, et al. GIT1 is associated with ADHD in humans and ADHD-like behaviors in mice. Nat Med 2011; 17:566-72; PMID:21499268; http://dx.doi.org/10.1038/nm.2330
- Durand CM, Perroy J, Loll F, Perrais D, Fagni L, Bourgeron T, Montcouquiol M, Sans N. SHANK3 mutations identified in autism lead to modification of dendritic spine morphology via an actin-dependent mechanism. Mol Psychiatry 2012; 17:71-84; PMID:21606927; http://dx.doi.org/10.1038/mp.2011.57
- Duffney LJ, Wei J, Cheng J, Liu W, Smith KR, Kittler JT, Yan Z. Shank3 deficiency induces NMDA receptor hypofunction via an actin-dependent mechanism. J Neurosci 2013; 33:15767-78; PMID:24089484; http://dx.doi.org/10.1523/JNEUROSCI.1175-13.2013
- Steinecke A, Gampe C, Nitzsche F, Bolz J. DISC1 knockdown impairs the tangential migration of cortical interneurons by affecting the actin cytoskeleton. Front Cell Neurosci 2014; 8:190; PMID:25071449; http://dx.doi.org/10.3389/fncel.2014.00190
- Peykov S, Berkel S, Schoen M, Weiss K, Degenhardt F, Strohmaier J, Weiss B, Proepper C, Schratt G, Nöthen MM, et al. Identification and functional characterization of rare SHANK2 variants in schizophrenia. Mol Psychiatry 2015; 20:1489-98; http://dx.doi.org/10.1038/mp.2014.172
- Duffney LJ, Zhong P, Wei J, Matas E, Cheng J, Qin L, Ma K, Dietz DM, Kajiwara Y, Buxbaum JD, et al. Autism-like Deficits in Shank3-Deficient Mice Are Rescued by Targeting Actin Regulators. Cell Rep 2015; 11(9):1400-13; PMID:26027926