ABSTRACT
In the legacy of Thomas Henry Huxley, and his ‘epigenetic’ philosophy of biology, cells are proposed to represent a trinity of three memory-storing media: Senome, Epigenome, and Genome that together comprise a cell-wide informational architecture. Our current preferential focus on the Genome needs to be complemented by a similar focus on the Epigenome and a here proposed Senome, representing the sum of all the sensory experiences of the cognitive cell and its sensing apparatus. Only then will biology be in a position to embrace the whole complexity of the eukaryotic cell, understanding its true nature which allows the communicative assembly of cells in the form of sentient multicellular organisms.
Introduction
Since the discovery of DNA and its role in coding proteins some sixty years ago, the biological sciences have accomplished a breakthrough revolution, evolving from an imprecise, descriptive science of associations into its more mature status that can stand in equivalent juxtaposition to the previously dominating fields of physics and chemistry. However, in order to complete this revolution, something fundamental is still missing from modern biology, as evidenced by difficulties in tackling the “hard questions” such as biological nature of feelings, emotions, qualia (subjective sum of all sensory experiences) and consciousness. This is not any trivial problem. Illumination of these phenomena is crucial for our understanding of our human nature as well as the world around us, both its biotic and abiotic parts.
In the legacy of Thomas Henry Huxley [Citation1], cells are proposed to represent a trinity of three memory-storing media. Upstream of the genome and epigenome, the bioelectric senome acts in close association with the plasma membrane. Recent advances in neurosciences make it clear that feelings, emotions, qualia and consciousness are very real biological phenomena based on chemistry and fundamental biology [Citation2–Citation7]. Sleep, qualia, consciousness and cognition, all appear to represent essential living qualities, not only for humans, but for all living organisms as they struggle to survive in a hostile environment [Citation8–Citation17]. However, the true nature of these inherent phenomena is still elusive and highly controversial.
If feelings, emotions, cognition, qualia and consciousness are based on neurochemical toolkits and processes [Citation2,Citation3,Citation7,Citation9,Citation18–Citation21], their true nature should be decipherable. Nevertheless, we obviously fail in this crucial aim, suggesting that our current approach is missing some essential aspects of these phenomena and/or our approaches and methodology are flawed. Here we suggest that in order to succeed in resolving the biological basis of these fundamental aspects of living organisms, we need to add a new science to our biological agenda that focuses on sensory biology linked to cellular cognition and behavior. This new science of feelings, emotions, qualia and consciousness must be able to tackle these issues from an evolutionary perspective [Citation4–Citation7,Citation16,Citation18–Citation22]. Its further requirement is an explanation of the emergence of a variety of elusive biological phenomena in their hypothetical ancient proto-states at the level of the simplest unicellular organisms [Citation7,Citation14,Citation16,Citation22,Citation23], such as proto-feelings, proto-emotions, proto-qualia, proto-consciousness [Citation7,Citation24] which could then be further engaged as complexation in multicellular organisms.
Box 1. Components and bioelectric field-like nature of Senome with implications for multicellularity and social cognition.
The cell in the 21st century
Recently it is emerging that all cells [Citation25] demonstrate basal cognition [Citation8,Citation14,Citation16,Citation23,Citation26–Citation33]. Indeed, it has been argued that life should properly be defined through the property of self-referential cognition [Citation31,Citation34]. Furthermore, recent advances have also revealed that neuronal aspects and cognitive capacities are valid concepts for unicellular organisms too [Citation14,Citation16,Citation22,Citation35]. Even bacteria show sensory complexity and sensory-motor circuits between plasma membrane-based sensors and correspondent motor responses of their flagella modulated by previous experiences [Citation14,Citation36]. It has also been demonstrated that plants have complex sensory systems which feed into their adaptive behavior [Citation37–Citation39]. Tropisms of plant organs, such as gravitropism and phototropism, are based on cellular-based sensory events that are spatially separated from motoric zones [Citation37,Citation39,Citation40]. Rapid electrical and slower chemical cell-cell communications, accomplished across plant synapses, are inter-linking and integrate sensory-motoric circuits of plant organs. Although these plant synapses differ in their molecular toolkit and some structural aspects from the animal neuronal synapses, they are organized through very similar principles and also perform analogous tasks [Citation39–Citation46]. Furthermore, neuronal synapses have deep evolutionary origins and have evolved from proto-synapses [Citation9,Citation47] which might underlie primordial sentience based on proto-feelings, proto-emotions, proto-qualia, and proto-consciousness [Citation7,Citation24]. It can be argued that such a deep evolutionary origin of neurons and synapses implies that these derivative qualities are inherent aspects of cellular organisms which are as fundamental to unicellular existence as they are known to be for multicellular organisms [Citation8,Citation30,Citation43,Citation48].
All biological organisms during their evolution are shaped by their self-referential adaptation to an ever-changing environment [Citation31,Citation49]. As this environment is often rather hostile and extremely variable, all organisms in order to survive need to extract, process, store and use faithful and effective information about their environment [Citation49,Citation50]. It has been previously argued that this process of information extraction and reception, and its further communication and deployment, is based on a consistent reiterative self-referential information cycle that undergirds all cellular life [Citation31,Citation34,Citation49–Citation51]. In such circumstances, genetic information and epigenetic inputs must be viewed as elements of a toolkit as part of the cell-wide information cycle by which cellular organism sense and adapt to environmental stresses [Citation51]. As a derivative then, information which had been encoded in DNA sequences of any ancient organisms was first perceived as sensory information. This means that sensory information is upstream of DNA information. Since there is no indication that DNA directly participates in the acquisition of sensory data, its use as memory and as a reproductive tool is a dependent function of an entire cellular sensory architecture by which environmental cues can be assessed and deployed. As this is information-dependent action, the cell is best understood as a form of informational architecture which is heavily dependent on sensory informational cues received from the external environment [Citation49,Citation51,Citation52].
It is therefore proposed that insofar as DNA sequence coding for a particular product is termed the gene, each particular sensory experience subjectively perceived by any organism should be termed the sene. In order to survive, organisms must retrieve as much information as possible in form of the senes that relates to the true nature of their environment. It could be imagined that those organisms which were not able to retrieve senes that truly corresponded to their environment were weeded-out via filtering Darwinian selection. In this process, the complexity and fidelity of the senome continuously increased during biological evolution. This senomic information is essential for any organism, not only with respect to its adaptive behavior (akin software), but also as safe storage of their most relevant aspects within their genomes (akin hardware). Together, these constitute the informational architecture of the living cell as essential elements of cell-wide cognitive information management system. It is this dynamical coupling that unites the senome with genome to critically intersect with the variety of memory-encoding mechanisms that characterize the fully functioning cell. It is argued that in order to unravel this inherent duality of dynamic interactions between sensory and DNA/RNA-based information, the biological sciences must put more efforts on sensory aspects of biology as an integration in which sensory information and memory are both sides of the “same coin” that can instill a new biological sciences of the new 21st Century.
Senome of cellular life
Therefore, in accordance with the genetic sciences, in which the sum of all genes is termed the Genome and that science which aims at illuminating all processes related to the genome is called Genomics; the sum of all Senes should be termed the Senome and the science behind it, Senomics. Only such a focus and approach to these self-referential phenomena will allow us to fully understand their biological basis as well as their true non-genomic and primary nature. Since any viewpoint that the cell is a functional automaton no longer comports with scientific data, those sources of informational input and the complex mechanisms by which information is assessed, communicated, deployed and managed becomes the new ground state of any detailed understanding of cellular life or the holobionts that are its collective product [Citation31,Citation53]. As each biological organism is inevitably a subject, and its world-view is constructed internally from its particular self-referenced Senome evolved during its specific evolutionary trajectory and enfolded during its individual life, it is obvious that the Senome of each organism is unique.
Moreover, dynamic environment–organismal interplay shapes Senomes of individual organisms during their life, so that even two genetic twin organisms will move apart with respect to their Senomes, according to their unique personal experiences which necessarily accumulate during their individual life histories. Importantly, although this has been conventionally acknowledged as a function of acquired epigenetic experiences, the Senome concept includes the memory encoded within the non-genomic structured arrangement of molecules [Citation18–Citation21,Citation54] that also gets transferred through cell divisions or between generations [Citation54]. Further too, the Senome includes the variety of sensory fields, such as electromagnetic, electrical, vibratory, or mechano-transduction, that store senomic information and transmit towards epigenome and genome (Box 1).
Clearly, replicating and cycling stores of information perpetuate life. It is argued that is the summation of all of these informational factors: Genome, Epigenome, and Senome. All these three systems, interwoven together, form the fully capable and adaptable cell. All three are also required participants in the complex interrelationships and communications that enable life and its survival.
In the case of unicellular organisms, the major organ for storing of genetic information is prokaryotic nucleoid or eukaryotic nucleus. On the other hand, the major organ for storing senomic information in all unicellular organisms is the plasma membrane, which represents the sensory boundary of the cell. This sensory boundary not only retrieves information from the environment but also displays this information akin to a holographic screen [Citation55]. The plasma membrane is enriched with diverse sensors which are optimized during their evolution to retrieve faithfully information from the environment [Citation56]. Selective and ordered activation of these sensors at the plasma membrane generates a 4-dimensional (spatial coordinates and time) senomic model of the outside world and assures its continuous updating [Citation55]. In other words, the Senome is a smart perceptual “gate” which first receives and then channels all the acquired sensory information into the interior of the organism [Citation55]. Here, this information is used to drive downstream metabolic and motoric processes according to the proscriptions of the cell’s information management system [Citation31,Citation34]. Therefore, it can be proposed that the Senome is the collective attachment to the informational matrix of the environment that propels internal cycles of biological information upon which all cells depend. Moreover, the emphasis of long-term information, which predominately proves to be relevant for organismal survival, is stored in the form of DNA sequences within the genome.
In the last ten years, epigenomics has emerged as an important counterpart of genomics in regulating biological organisms [Citation57–Citation60]. Epigenomics encompasses chemical modifications of DNA such as methylation, histones, chromatin-remodeling protein complexes [Citation61], and other nuclear proteins, chaperones and some aspects of the dynamic cytoplasmic cytoskeleton [Citation62]. All of these are deeply interlinked with the endocytic matrix [Citation63] that integrates the Senome with the epigenome and genome [Citation63].
It is increasingly apparent that epigenomics is relevant for neuronal identity and plasticity, as well as mental states, disorders and behavior [Citation57–Citation59,Citation64,Citation65]. Certainly too, it is assumed that the genome of any organism has a similar over-arching function. Yet, neither the epigenome nor the intrinsic genome have provided satisfying answers to the complexity of human behavior (see the last section too). Therefore, it is proposed that in order to understand the cells that comprise any complex organism, such as ourselves, the addition of interactive senomics into our agenda is required. That Senome governs the primary aspect of living systems, encompassing both the epigenome and genome (). Within the complex informational architecture of the cell, any environmental change first stimulates plasma membrane sensors which respond within seconds [Citation56]. Only later, within minutes and hours, there might be downstream changes to the cytoskeleton, or other parts of the cell, such its epigenome. And, only then, will there be consequential expression from the genome.
Figure 1. Senomic view of a cell.
In this schematic and highly reduced view of a cell, the Senome is shown in blue, the Epigenome in yellow, and the Genome in red. The Senome encompasses the plasma membrane equipped with ion channels and transporters, vesicles and whole endocytic network. The epigenome encompasses all structures feeding from the Senome to the Genome, acting as a smart “translator” for dynamic feedbacks between the Senome and the Genome.
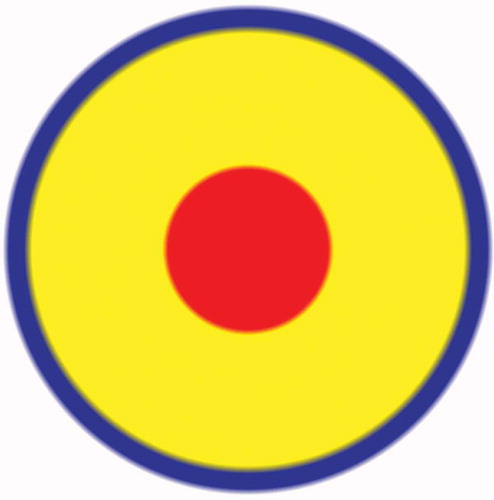
Implications of the cellular Senome
Our understanding of the cell and its intelligent adaptations will always remain incomplete if exclusively explored through epigenetics and genomics. When genes are properly assessed as among those tools that cells deploy in their extraction and use of information, then the need for the cell to have a sensing apparatus to respond to environmental stress becomes apparent. As an integral aspect of the cell, the senome comprises the summation of its active boundary conditions, including the plasma membrane amd the memory-encoded heritable molecular structural arrangements that particiate in the assessment, deployment and communication of information both within and without the cell.
All together, the Senome, Epigenome, and Genome form the entire informational architecture of the cell. It has been previously argued that the cellular form should be regarded as the biological expression of a self-referential information management system [Citation34]. Within that self-referential cognitive frame, the Senome becomes a required link within any whole cell concept. It is the vital conduit of informational content and its transfer to the embedded systematic memory of the cell that maintains its homeostasic equipoise. It is well accepted that the cell attempts to sustain its most efficient homeostatic moment through the use and communication of self-referential information. Therefore, that process of sustaining homeostasis can no longer be viewed within a thermostatic model. Instead, it must be seen as a function of the active problem-solving capacity of any cell [Citation34,Citation66–Citation71]. In such circumstances, a Senome becomes a requirement. It is a necesssary intercessory instrument interposed between the cell and its environment. Thus, the Senome is the means by which cell responses, including epigenetic and genomic ones, coordinate in continuous confrontation with external environmental stresses.
It can be argued that there is justification in viewing the plasma membrane-based Senome as a kind of cellular “brain” [Citation36]. When so considered, the behavioral complexity of archaea, bacteria and diverse unicellular eukaryotes can be explained [Citation14,Citation18–Citation21,Citation72,Citation73] In like manner, the intelligence of syncytial plasmodia Physarum polycephalum [Citation16,Citation74–Citation76], as well as the intelligence of plants and their robust communicative/cognitive faculties can be sufficiently understood [Citation8,Citation77–Citation82]. Thus, the Senome provides a background through which the basal cognitive capacities of the cell, can be explored along pathways toward the type of consciousness that is typically ascribed to animals, and eventually to ourselves. The Senome can be seen as the link between qualia, as a unicellular form of sensorium, and higher levels of subjective consciousness as fundamental to the informational connections that ultimately apply to inherently cellular complex holobionic organisms. As the cellular interface between the outward environment and the living interior of cell, it can be further proposed that the Senome is at the express interface of physics and biology through which physical stimuli are translated into biological experiences that ultimately leads to human sentience (Box 1). As an explicit example, the Senome first generates electric signals at the plasma membrane and then transforms these electric signals into the chemical “language” of signaling networks impinging on the epigenome [Citation56]. Only when contemporary biology will integrate the Genome, Epigenome and Senome altogether, will it be able to embrace the whole complexity of the eukaryotic cell to understand communicative assembly of cells in the form of multicellular organisms [Citation43,Citation46,Citation48,Citation49,Citation51,Citation68–Citation71].
Components of the Senome and their relevances for life
As proposed in this Opinion paper, the Senome is composed primarilly of a limiting plasma membrane (Box 1) which is the phospholipid double-layer equipped with myriad of proteins, lipids, sugars and other carbohydrates [Citation83,Citation84]. Characteristic features of the plasma membrane include its self-organization and inherent association with the actin cytoskeleton via its inner leaflet and diverse extracellular structures via its outer leaflet [Citation83–Citation89]. All life known on this planet is cellular. It is the assembly of lipid membranes that allowed the emergence of cellular life [Citation90–Citation92]. Membrane-based senes give all organisms their agency which is the central feature for their acting as genuinely living organisms. The totality of all senes is the Senome which underlies embodied cognition and sense of Self (see also the Glossary), allowing organisms to act in their own interest.
A very strong argument in a favour of the Senome for the still mysterious phenomenon of life is provided by viruses which are inert non-living structures as long as they are outside of their host cells [Citation93]. However when inside their host cells and included within the senomic context, viruses can actively manipulate cellular membranes and the cytoskeleton. This allows them to form unique viral membraneous compartments, ultimately reaching access to the nucleus for both their replication and egress [Citation94–Citation97]. Similarly DNA acts as genetic blueprint of cells only if placed within the senomic context of cells whereas outside of cell, DNA is merely biologically inert macromolecule [Citation68,Citation98].
Implications of the cellular Senome for multicellularity and social cognition
Finally, the Senome has potency to explain multicellularity based on cell-cell communication and cognition (Box 1) [Citation68–Citation71,Citation99]. Social and emotional closeness, sympathy, bonding/friendship of humans are emergent self-similar aspects of shared subject Senomes, as seen for example in similar neural responses to sensory stimulation [Citation49,Citation100–Citation103]. All this suggests that a shared species specific Senome, as collective information and communication, underlies social networks in humans [Citation100,Citation101]. Therefore, it is argued that the Senome has direct pertinence for understanding human perception and understanding of mental signals from outside (memes) or from within our own bodies (perceived as emotions and feelings) and further relevances for both individual and social cognition [Citation100–Citation107], that ultimately leads to the theory of mind [Citation5,Citation106,Citation108,Citation109] and human cultural evolution [Citation110–Citation113].
Conclusions
The Senome translates physical signals from the outside world into the physico-chemical language of cells. It is central feature for all cellular life as it allows DNA and RNA to support life processes. Without membranes generating the Senome, DNA and RNA are rather inert macromolecule not able to support the living processes. We propose that the Senome is the inherent partner of the Genome and the Epigenome. The Senome underlies sensory order [Citation114] which gives all cellular organisms a sense of time and agency [Citation115]. Therefore, the Senome can be seen as the nexus between the prescribed cognitive capacities of the cell, its informational architecture and its working characteristics. All this require orderly regulation [Citation115]. The Senome functions as a plasma membrane-based cell-wide sensory organ of assessment and action that permits continuous coordinated organismal-environmental complementarity. When placed in the context of cellular cognition, the Senome is that aspect of the cellular life that interrogates the environment and guides cellular responses and adaptation as a problem-solving phenomenon. The Senome should be added to our biological agenda as an additional means by which the entire complexity of the sentient and cognitive cell, and those crucial communicative aspects that lead to complex multicellular organisms, can be understood. Perhaps through this new exploration, based on inherent and primordial cell processes, a path towards the long-sought unification between physics and biology will be revealed.
(Glossary)
The Senome Concept in Short
Sene: Sensor-based sensory experience (event) which extract from environment information of adaptive value for the organism.
Senome: Sum of all sensory information of cell (or an organism) generated by the plasma membrane and its derived endosomal membranes. Sensors process and integrate sensory experiences into adaptive behavioural outputs. The Senome includes electric, magnetic and mechanic fields generated by (around as well as within) the organism – these then feed-back back into the epigenome and, finally, into the genome. At the multicellular level, Senome is represented by the sum of all synapses. Senome generates the Self – the subject – and drives its behaviour.
Self: Sum of all sensory information of an organism, integrated with information encoded both in the epigenome and genome, which allows generation of the organism subjective identity.
Sentience: Ability to perceive and feel environment subjectively.
Subject: Organism (agent) which has subjective sensory experiences generating its subjective consciousness, constructing its own world-view (active agency on, and relationship with, its environment).
Organism (Agent): Living system endowed with the Senome – Epigenome – Genome complex; capable of responses to stimuli, reproduction, growth and development, and maintenance of homeostasis. The organism is active in altering of physical environment to construct its own living niche as a problem-solving entity. It is capable of communication with, and manipulation of, other organisms sharing the same niche and environement.
Genome: Sum of all protein coding DNA/RNA-based hereditary information of an organism.
Epigenome: Sum of all non-coding DNA/RNA-based hereditary information of an organism. Besides the machinery regulating package of DNA into the chromatin and chromosomes, the epigenome includes also all structures acting as templates for their own copying. [Citation149]
Acknowledgments
František Baluška is thankful to Professor Ladislav Kováč (Comenius University Bratislava, Slovakia) for very stimulating discussions on topics covered in this Opinion paper.
Disclosure statement
No potential conflict of interest was reported by the authors.
References
- Richmond ML. Thomas Henry Huxley’s developmental view of the cell. Nat Rev Mol Cell Biol. 2001;3:61–65.
- Panksepp J. Affective neurosciences. The foundation of human and animal emotions. Oxford, UK: Oxford University Press; 1998.
- Van Dort CJ, Baghdoyan HA, Lydic R. Neurochemical modulators of sleep and anesthetic states. Int Anesthesiol Clin. 2008;46:75–104.
- Mashour GA, Alkire MT. Evolution of consciousness: phylogeny, ontogeny, and emergence from general anesthesia. Proc Natl Acad Sci USA. 2013;110(Suppl2):10357–10364.
- Searle J. Theory of mind and Darwin’s legacy. Proc Natl Acad Sci USA. 2013;110(Suppl2):10343–10348.
- Curwen C. Music-colour synaesthesia: concept, context and qualia. Conscious Cogn. 2018;61:94–106.
- Bronfman ZZ, Ginsburg S, Jablonka E. The transition to minimal consciousness through the evolution of associative learning. Front Psychol. 2016;7:1954.
- Baluška F, Mancuso S. Plant neurobiology: from sensory biology, via plant communication, to social plant behavior. Cogn Process. 2009;10(Suppl 1):S3–S7.
- Grant SGN. A general basis for cognition in the evolution of synapse signaling complexes. Cold Spring Harb Symp Quant Biol. 2010;74:249.
- Trewavas AJ, Baluška F. The ubiquity of consciousness. The ubiquity of consciousness, cognition and intelligence in life. EMBO Rep. 2011;12:1221–1225.
- Perouansky M. The quest for a unified model of anesthetic action: a century in Claude Bernard’s shadow. Anesthesiology. 2012;117:465–474.
- Grémiaux A, Yokawa K, Mancuso S, et al. Plant anesthesia supports similarities between animals and plants: Claude Bernard’s forgotten studies. Plant Signal Behav. 2014;9:e27886.
- Rinaldi A. Reawakening anaesthesia research. EMBO Rep. 2014;15:1113–1118.
- Lyon P. The cognitive cell: bacterial behavior reconsidered. Front Microbiol. 2015;6:264.
- Baluška F, Yokawa K, Mancuso S, et al. Understanding of anesthesia - why consciousness is essential for life and not based on genes. Commun Integr Biol. 2016;9:e1238118.
- Vallverdú J, Castro O, Mayne R, et al. Slime mould: the fundamental mechanisms of biological cognition. Biosystems. 2018;165:57–70.
- Yokawa K, Kagenishi T, Pavlovic A, et al. Anaesthetics stop diverse plant organ movements, affect endocytic vesicle recycling and ROS homeostasis, and block action potentials in Venus flytraps. Ann Bot. 2018. In press.
- Kováč L. Fundamental principles of cognitive biology. Evol Cogn. 2000;6:51.
- Kováč L. Life, chemistry and cognition. EMBO Rep. 2006;7:562.
- Kováč L. Information and knowledge in biology - time for reappraisal. Plant Signal Behav. 2007;2:65.
- Kováč L. Bioenergetics - a key to brain and mind. Commun Integr Biol. 2008;1:114.
- Greenspan R. An introduction to nervous systems. New York (NY): Cold Spring Harbor Laboratory Press; 2007.
- Keijzer FA. Evolutionary convergence and biologically embodied cognition. Inter Foc. 2017;7:20160123.
- Hobson JA. REM sleep and dreaming: towards a theory of protoconsciousness. Nat Rev Neurosci. 2009;10:803–813.
- Koseska A, Bastiaens PI. Cell signaling as a cognitive process. EMBO J. 2017;36:568–582.
- Shapiro J. Bacteria are small but not stupid: cognition, natural genetic engineering and socio-bacteriology. Stud Hist Phil Biol Biomed Sci Part C. 2997;38:807–819.
- Shapiro JA. Mobile DNA and evolution in the 21st century. Mobile DNA. 2010;1:4.
- Shapiro JA. Evolution. A view from the 21st century. Saddle River, NJ: FT Press Science; 2011.
- Shapiro JA. Living organisms author their read-write genomes in evolution. Biology (Basel). 2017;6:42.
- Baluška F, Mancuso S. Deep evolutionary origins of neurobiology: turning the essence of ‘neural’ upside-down. Commun Integr Biol. 2009;2:62–65.
- Miller WB. Cognition, information fields and hologenomic entanglement: evolution in light and shadow. Biology (Basel). 2016;5:21.
- Ford BJ. On intelligence in cells: the case for whole cell biology. Interdiscip Sci Rev. 2009;34:350–365.
- Ford BJ. Cellular intelligence: microphenomenology and the realities of being. Prog Biophys Mol Biol. 2017;131:273.
- Miller WB. Biological information systems: evolution as cognition-based information management. Prog Biophys Mol Biol. 2018;134:1.
- Baluška F, Levin M. On having no head: cognition throughout biological systems. Front Psychol. 2016;7:902.
- Stock J, Levit M. Signal transduction: hair brains in bacterial chemotaxis. Curr Biol. 2000;10:R11–4.
- Weiler EW. Sensory principles of higher plants. Angew Chem Int Ed. 2003;42:392–411.
- Brenner ED, Stahlberg R, Mancuso S, et al. Plant neurobiology: an integrated view of plant signaling. Trends Plant Sci. 2006;11:413–419.
- Baluška F, Mancuso S, Volkmann D, et al. The ‘root-brain’ hypothesis of Charles and Francis Darwin: revival after more than 125 years. Plant Signal Behav. 2009;4:1121–1127.
- Baluška F, Mancuso S. Root apex transition zone as oscillatory zone. Front Plant Sci. 2013;4:354.
- Baluška F, Volkmann D, Menzel D. Plant synapses: actin-based domains for cell-to-cell communication. Trends Plant Sci. 2005;10:106–111.
- Schlicht M, Strnad M, Scanlon MJ, et al. Auxin immunolocalization implicates vesicular neurotransmitter-like mode of polar auxin transport in root apices. Plant Signal Behav. 2006;1:122–133.
- Baluška F. Cell-cell channels, viruses, and evolution: via infection, parasitism, and symbiosis toward higher levels of biological complexity. Ann N Y Acad Sci. 2009;1178:106–119.
- Baluška F, Mancuso S. Microorganism and filamentous fungi drive evolution of plant synapses. Front Cell Infect Microbiol. 2013;3:44.
- Mettbach U, Strnad M, Mancuso S, et al. Immunogold-EM analysis reveal Brefeldin A-sensitive clusters of auxin in Arabidopsis root apex cells. Commun Integr Biol. 2017;10:e1327105.
- Baluška F, Mancuso S. Synaptic view of eukaryotic cell. Int J Gen Syst. 2014;43:740–756.
- Kosik KN. Exploring the early origins of the synapse by comparative genomics. Biol Lett. 2009;5:108–111.
- Baluška F. Rethinking origins of multicellularity: convergent evolution of epithelia in plants. Bioessays. 2012;34:1085.
- Agnati LF, Baluška F, Barlow PW, et al. Mosaic, self-similarity logic, and biological attraction principles: three explanatory instruments in biology. Commun Integr Biol. 2009;2:552–563.
- Torday JS, Miller WB. A systems approach to physiologic evolution: from micelles to consciousness. J Cell Physiol. 2017;1:162–167.
- Miller WB, Torday JS. A systematic approach to cancer: evolution beyond selection. Clin Transl Med. 2017;6:2.
- Marijuán PC, Navarro J, del Moral R. How the living is in the world: an inquiry into the informational choreographies of life. Prog Biophys Mol Biol. 2015;31:469–480.
- Gilbert SF, Sapp J, Tauber AI. A symbiotic view of life: we have never been individuals. Q Rev Biol. 2012;87:325–341.
- Jose AM. Replicating and cycling stores of information perpetuate life. BioEssays. 2018;40:e1700161.
- Lintilhac PM. Toward a theory of cellularity – speculations on the nature of the living cell. BioScience. 1999;49:59–68.
- Loewenstein W. Physics in mind: a quantum view of the brain. New York: Basic Books; 2013.
- Kato T. Epigenomics in psychiatry. Neuropsychobiology. 2009;60:2–4.
- Mohammad HP, Baylin SB. Linking cell signaling and the epigenetic machinery. Nat Biotechnol. 2010;28:1033–1038.
- Gallegos DA, Chan U, Chen LF, et al. Chromatin regulation of neuronal maturation and plasticity. Trends Neurosci. 2018;41:311–324.
- Marasca F, Bodega B, Orlando V. How polycomb-mediated cell memory deals with a changing environment: variations in PcG complexes and proteins assortment convey plasticity to epigenetic regulation as a response to environment. BioEssays. 2018;40:e1700137.
- Ho L, Crabtree GR. Chromatin remodelling during development. Nature. 2010;463:474–484.
- Fletcher DA, Mullins RD. Cell mechanics and the cytoskeleton. Nature. 2010;463:485–492.
- Scita G, Di Fiore PP. The endocytic matrix. Nature. 2010;463:464–473.
- Borrelli E, Nestler EJ, Allis CD, et al. Decoding the epigenetic language of neuronal plasticity. Neuron. 2008;60:961–974.
- Patalano S, Hore TA, Reik W, et al. Shifting behaviour: epigenetic reprogramming in eusocial insects. Curr Opin Cell Biol. 2012;24:367–373.
- Popper K. All life is problem solving. London, UK: Routledge, Taylor and Francis Group; 2001.
- Niemann H-J. Karl Popper and the two new secrets of life. Tübingen: Mohr Siebeck; 2014.
- Baluška F, Witzany G. Life is more than a computer running DNA software. World J Biol Chem. 2014;5:275–278.
- Witzany G. The biocommunication method: on the road to an integrative biology. Commun Integr Biol. 2016;9:e1164374.
- De Loof A. From Darwin’s on the origin of species by means of natural selection to the evolution of life with communication activity as its very essence and driving force (= mega-evolution). Life Excit Biol. 2015;3:153–189.
- De Loof A. The Evolution of” Life”: a metadarwinian integrative approach. Commun Integr Biol. 2017;3:e1301335.
- Hellingwerf KJ. Bacterial observations: a rudimentary form of intelligence? Trends Microbiol. 2005;13:152–158.
- Van Duijn M, Keijzer F, Franken D. Principles of minimal cognition: casting cognition as sensorimotor coordination. Adapt Behav. 2006;14:157–170.
- Ueda T. An intelligent slime mold: a self-organizing system of cell shape and information. Lect Notes Complex Syst. 2005;3:221–253.
- Ball P. Cellular memory hints at the origins of intelligence. Nature. 2008;451:385.
- Dussutour A, Latty T, Beekman M, et al. Amoeboid organism solves complex nutritional challenges. Proc Natl Acad Sci USA. 2010;107:4607–4611.
- Calvo Garzón F, Keijzer F. Cognition in plants. In: Baluška F, ed. Plant-environment interactions from behavioural perspective. Berlin - Heidelberg - New York: Springer Verlag; 2009. p. 247–266.
- Trewavas A. Aspects of plant intelligence. Ann Bot. 2003;92:1–20.
- Trewavas A. Plant intelligence. Naturwissenschaften. 2005;92:401–413.
- Trewavas A. Intelligence, cognition, and language of green plants. Front Psychol. 2016;7:588.
- Calvo P, Sahi VP, Trewavas A. Are plants sentient? Plant Cell Environ. 2017;40:2858–2869.
- Mian IS, Rose C. Communication theory and multicellular biology. Integr Biol. 2011;3:350–367.
- Nicolson GL. The Fluid-Mosaic model of membrane structure: still relevant to understanding the structure, function and dynamics of biological membranes after more than 40 years. Biochim Biophys Acta. 2014;1838:1451–1466.
- Goñi FM. The basic structure and dynamics of cell membranes: an update of the Singer-Nicolson model. Biochim Biophys Acta. 2014;1838:1467–1476.
- Kaiser H-J, Lingwood D, Levental I, et al. Order of lipid phases in model and plasma membranes. Proc Natl Acad Sci USA. 2009;106:16645–16650.
- Sych T, Mély Y, Römer W. Lipid self-assembly and lectin-induced reorganization of the plasma membrane. Philos Trans R Soc Lond B Biol Sci. 2018;373:1747.
- Honigmann A, Pralle A. Compartmentalization of the cell membrane. J Mol Biol. 2016;428:4739–4748.
- Krapf D. Compartmentalization of the plasma membrane. Curr Opin Cell Biol. 2018;53:15–21.
- Sadegh S, Higgins JL, Mannion PC, et al. The plasma membrane is compartmentalized by a self-similar cortical actin meshwork. Phys Rev X. 2017;7:011031.
- Lane N, Martin WF. The origin of membrane bioenergetics. Cell. 2012;151:1406–1416.
- Deamer D. The role of lipid membranes in life’s origin. Life. 2017;7:5.
- Lane N. Proton gradients and the origin of life. BioEssays. 2017;39:6.
- Moreira D, López-García P. Ten reasons to exclude viruses from the tree of life. Nat Rev Microbiol. 2009;7:306–311.
- Claverie J-M. Viruses take center stage in cellular evolution. Genome Biol. 2006;7:110.
- Hegde NR, Maddur MS, Kaveri SV, et al. Reasons to include viruses in the tree of life. Nat Rev Microbiol. 2009;7:615.
- Forterre P. Defining life: the virus viewpoint. Orig Life Evol Biosph. 2010;40:151–160.
- Ruiz-Saenz J, Rodas JD. Viruses, virophages, and their living nature. Acta Virol. 2010;54:85–90.
- Annila A, Baverstock K. Genes without prominence: a reappraisal of the foundations of biology. J R Soc Interface. 2014;11:20131017.
- Miller WB Jr, Torday JS. Four domains: the fundamental unicell and post-Darwinian cognition-based evolution. Progr Biophys Mol Biol. 2018. In press.
- Parkinson C, Kleinbaum AM, Wheatley T. Similar neural responses predict friendship. Nat Commun. 2018;9:332.
- Dunbar RIM. The anatomy of friendship. Trends Cogn Sci. 2018;22:32–51.
- Panksepp J, Panksepp JB. Toward a cross-species understanding of empathy. Trends Neurosci. 2013;36:489–496.
- Peil KT. Emotion: the self-regulatory sense. Glob Adv Health Med. 2014;3:80–108.
- Blackmore S. Memes and the evolution of religion: we need memetics, too. Behav Brain Sci. 2016;39:e5.
- Schaden G, Patin C. Semiotic systems with duality of patterning and the issue of cultural replicators. Hist Philos Life Sci. 2017;40:4.
- Fortier J, Besnard J, Allain P. Theory of mind, empathy and emotion perception in cortical and subcortical neurodegenerative diseases. Rev Neurol (Paris). 2018;174:237–246.
- Wittmann MK, Lockwood PL, Rushworth MFS. Neural mechanisms of social cognition in primates. Annu Rev Neurosci. 2018;41. In press.
- Westby CE. Social neuroscience and theory of mind. Folia Phoniatr Logop. 2014;66:7–17.
- Wang Y, Olson IR. The original social network: white matter and social cognition. Trends Cogn Sci. 2018;22:504–516.
- Kováč L. Science, an essential part of culture. EMBO Rep. 2006;7:128–132.
- Kováč L. Finitics - a plea for biological realism. EMBO Rep. 2008;9:703–708.
- Laland K, Odling-Smee J, Myles S. How culture shaped the human genome: bringing genetics and the human sciences together. Nat Rev Genet. 2010;11:137–148.
- Pagel M. Human language as a culturally transmitted replicator. Nat Rev Genet. 2009;10:405–415.
- Hayek FA. The sensory order. An inquiry into the foundations of the theoretical psychology. Chicago (IL): University of Chicago Press; 1952.
- Vuorre M. On time, causation and the sense of agency. J Consc Stud. 2017;3-4:203–215.
- Olivotto M, Arcangeli A, Carlà M, et al. Electric fields at the plasma membrane level: a neglected element in the mechanisms of cell signalling. BioEssays. 1996;18:495–504.
- Veech RL, Kashiwaya Y, Gates DN, et al. The energetics of ion distribution: the origin of the resting electric potential of cells. IUBMB Life. 2002;54:241–252.
- Scott BH. Electric fields in plants. Ann Rev Plant Biol. 1967;18:409–418.
- Weisenseel MH, Nuccitelli R, Jaffe LF. Large electrical currents traverse growing pollen tubes. J Cell Biol. 1975;66:556–567.
- Weisenseel MH, Dorn A, Jaffe LF. Natural H+ currents traverse growing roots and root hairs of barley (Hordeum vulgare L.). Plant Physiol. 1979;64:512–518.
- Iwabuchi A, Yano M, Shimizu H. Development of extracellular electric pattern around Lepidium roots: its possible role in root growth and gravitropism. Protoplasma. 1989;148:94–100.
- Miller AL, Gow NA. Correlation between root-generated ionic currents, pH, fusicoccin, indoleacetic acid, and growth of the primary root of Zea mays. Plant Physiol. 1989;89:1198–1206.
- Collings DA, White RG, Overall RL. Ionic current changes associated with the gravity-induced bending response in roots of Zea mays L. Plant Physiol. 1992;100:1417–1426.
- Baluška F, Wan Y-L. Physical control over endocytosis. In: Šamaj J, ed. Endocytosis in plants. Berlin, Germany: Springer-Verlag; 2012. p. 123–149.
- Baluška F, Mancuso S. Ion channels in plants: from bioelectricity, via signaling, to behavioral actions. Plant Signal Behav. 2013;8:e23009.
- Zhou S-A, Uesaka M. Bioelectrodynamics in living organisms. Int J Engin Sc. 2006;44:67–92.
- Levin M. Endogenous bioelectrical networks store non-genetic patterning information during development and regeneration. J Physiol. 2014;592:2295–2305.
- Levin M, Pezzulo G, Finkelstein JM. Endogenous bioelectric signaling networks: exploiting voltage gradients for cntrol of growth and form. Annu Rev Biomed Eng. 2017;19:353–387.
- Tseng A, Levin M. Cracking the bioelectric code: probing endogenous ionic controls of pattern formation. Commun Integr Biol. 2013;6:e22595.
- Levin M, Martyniuk CJ. The bioelectric code: an ancient computational medium for dynamic control of growth and form. Biosystems. 2018;164:76–93.
- Fröhlich F, McCormick DA. Endogenous electric fields may guide neocortical network activity. Neuron. 2010;67:129–143.
- Anastassiou CA, Perin R, Markram H, et al. Ephaptic coupling of cortical neurons. Nat Neurosci. 2011;14:217–223.
- Anastassiou CA, Koch C. Ephaptic coupling to endogenous electric field activity: why bother? Curr Opin Neurobiol. 2015;31:95–103.
- Goldwyn JH1, Rinzel J. Neuronal coupling by endogenous electric fields: cable theory and applications to coincidence detector neurons in the auditory brain stem. J Neurophysiol. 2016;115:2033–2051.
- Martinez-Banaclocha M. Ephaptic coupling of cortical neurons: possible contribution of astroglial magnetic fields? Neuroscience. 2018;370:37–45.
- Nunez PL, Srinivasan R. Electric fields of the brain: the neurophysics of EEG. Second ed. Oxford, UK: Oxford University Press; 2006.
- Buzsáki G, Anastassiou CA, Koch C. The origin of extracellular fields and currents -EEG, ECoG, LFP and spikes. Nat Rev Neurosci. 2012;13:407–420.
- Fels D. The double-aspect of life. Biol. 2018;7:28.
- Mouritsen H. Magnetoreception in birds and its use for long-distance migration. In: Sturkie’s avian physiology. Sixth ed. 2015. Associated Press; p. 113–133. DOI::10.1007/978-3-642-10,769-6_20.
- Wiltschko R, Thalau P, Gehring D, et al. Magnetoreception in birds: the effect of radio-frequency fields. J R Soc Interface. 2015;12:103.
- Wiltschko R, Ahmad M, Nießner C, et al. Light-dependent magnetoreception in birds: the crucial step occurs in the dark. J R Soc Interface. 2015;13:2015.
- Pinzon-Rodriguez A, Muheim R. Zebra finches have a light-dependent magnetic compass similar to migratory birds. J Exp Biol. 2017;220:1202–1209.
- Günther A, Einwich A, Sjulstok E, et al. Double-cone localization and seasonal expression pattern suggest a role in magnetoreception for European robin cryptochrome 4. Curr Biol. 2018;28:211–223.
- Catania KC. Electric eels concentrate their electric field to induce involuntary fatigue instruggling prey. Curr Biol. 2015;25:2889–2898.
- Clarke D, Whitney H, Sutton G, et al. Detection and learning of floral electric fields by bumblebees. Science. 2013;340:66–69.
- Clarke D, Morley E, Robert D. The bee, the flower, and the electric field: electric ecology and aerial electroreception. J Comp Physiol A Neuroethol Sens Neural Behav Physiol. 2017;203:737–748.
- Rohan M, Parow A, Stoll AL, et al. Low-field magnetic stimulation in bipolar depression using an MRI-based stimulator. Am J Psych. 2004;161:93–98.
- Rohan ML, Yamamoto RT, Ravichandran CT, et al. Rapid mood-elevating effects of low field magnetic stimulation in depression. Biol Psych. 2014;76:186–193.
- Fields C, Levin M. Multiscale memory and bioelectric error correction in the cytoplasm-cytoskeleton-membrane system. Wiley Interdiscip Rev Syst Biol Med. 2018;10:e1410.