ABSTRACT
Measures that aim to reduce greenhouse gas emissions also have impacts on achieving other Sustainable Development Goals (SDGs). Given the enormous challenge of achieving the goals of the Paris Agreement and the SDGs, insight into these impacts provides information on how to improve the feasibility of climate change mitigation measures by maximizing the co-benefits and managing the risks of possible trade-offs across SDGs. In this paper, we explore the impact of 20 promising climate mitigation measures on achieving the other SDGs for 11 world regions. Using the IMAGE modelling framework, the paper explores the GHG emission reduction potential of these measures aggregated by the sector under three scenarios. Based on peer-reviewed articles, the impact of the measures on other SDGs is assessed for the top three sectors with the highest GHG reduction potential in each region. We conclude that the number of synergies between the selected climate change mitigation measures and other SDGs dwarf the number of trade-offs in all regions. The magnitude of these synergies and trade-offs, however, varies by regional and socio-economic context. In high- and middle-income regions, the mitigation measures show few trade-offs that are generally associated with technology choices that could aggravate inequality and impact biodiversity. In low-income regions, some measures, especially land-use related ones, could interfere with efforts to reduce poverty, end hunger and improve well-being, if not complemented by additional policies that aim to protect the poor from increasing food and energy prices.
1. Introduction
The Paris Agreement and the Sustainable Development Goals (SDGs) aim to strengthen the global collaboration to halt climate change and to promote sustainable development. Together, they call for action to mitigate and adapt to climate change, end poverty, promote prosperity and people’s well-being while protecting the environment (UN Citation2015). The success of achieving the SDGs is closely intertwined with actions to halt climate change and deal with its impacts. As such, SDG 13 focuses on combatting climate change and its consequences (UN Citation2015), and Article 7 of the Paris Agreement calls for “enhancing adaptive capacity, strengthening resilience and reducing vulnerability to climate change, with a view to contributing to sustainable development” (UN Citation2015).
Climate change mitigation requires harmonizing efforts at multiple levels: sectors, countries, and regions. Insight into these impacts provides information on how to improve the feasibility of climate change mitigation measures. This insight is important to maximize the potential co-benefits and minimize the potential adverse impacts across multiple targets. It could also ensure policy coherence at various levels of government and provide a systemic view of the relationships between the measures and the SDGs (Editorial Citation2020).
There are several studies that shed light on the interactions between climate change mitigation and the SDGs. For instance, Fujimori et al. (Citation2019) explore the relationship between food security and climate mitigation under four scenarios with different levels of mitigation stringency in the context of the Paris Agreement. Soergel et al. (Citation2021) show that ambitious climate policies are not enough to fully exploit the synergies between climate mitigation and SDGs and investigate the need for an additional sustainable development package for a more comprehensive sustainable development pathway. Von Stechow et al. (Citation2016) explores the synergies and trade-offs of alternative 2°C pathways across indicators relevant for energy-related SDGs and sustainable energy objectives, while Campagnolo and Davide (Citation2019) investigate potential synergies and trade-offs between emission reduction policies and sustainable development objectives focusing on SDG1 and SDG10. The study by Rafaj et al. (Citation2021) shows that, with the help of a multi-model comparison, the health benefits can be enhanced by coordinating climate change mitigation and air pollution policies. Other studies (Pradhan et al. Citation2017; McCollum et al. Citation2018; Nilsson et al. Citation2018; Singh et al. Citation2018) illustrate the interconnection between different SDGs. However, literature that is specifically devoted to exploring the impact of climate change mitigation measures on other SDGs within a regional context is limited. This can be concluded from the summary of studies available on this topic by the IPCC Special Report on Global Warming of 1.5°C (IPCC Citation2018), which shows that many analysed mitigation measures are rather aggregated with limited representation of regional differences, even though both the potential and the impacts of climate change mitigation measures differ by region. The difference in co-benefits and trade-offs between regions could come from differences in the level of socio-economic development, vulnerability to the effects of climate change, availability of and reliance on certain natural resources, or the availability or lack of certain industries. For instance, the choice of offshore wind in Europe has different synergies and trade-offs than wind parks in the highlands of Kenya. Similarly, the causes of deforestation vary across regions and halting deforestation has an impact on commercial loggers in South America and on poor households that collect wood for energy in large parts of the SSA.
In this paper, we take mitigation measures that have been implemented in one or more countries and are effective in mitigating climate change as a starting point. For 11 world regions, we analyse the potential for reducing emissions of these measures. For the mitigation measures with the highest potential to reduce emissions, we perform a literature search on the potential impact on other SDGs for each world region. A more detailed description of the process and method is presented in the next section. Given the enormous challenge of achieving the Paris Agreement climate objective and other SDGs, insight into the co-benefits and trade-offs of important mitigation measures on a regional level is important to fully exploit the synergies and manage risks between climate action and other SDGs. This paper is partly based on the report by Dagnachew et al. (Citation2021).
The rest of the paper is organized as follows: Section 2 presents (i) the list of the selected promising mitigation measures, ii) the methodology for identifying the sectors with the highest mitigation potential for each world region, and iii) the methodology for identifying co-benefits and trade-offs of these measures with other SDGs. Section 3 provides the results of the co-benefits and trade-offs between the most promising mitigation measures and the SDGs with regional context, and Section 4 discusses the results and concludes the paper.
2. Methodology
Promising climate change mitigation measures are the starting point of the study. We define promising mitigation measures not only in terms of the associated costs of implementation but also other important characteristics, such as equity, social and political feasibility, and market imperfections. Earlier studies (Kriegler et al. Citation2018; Roelfsema et al. Citation2018; van Soest et al. Citation2021) have assessed promising climate change mitigation measures that were successfully implemented in one or more countries and show a considerable potential in reducing greenhouse gas (GHG) emissions. The implementation of these measures in the short to medium term (i.e. one to three decades) has proven to be feasible. We build on van Soest et al. (Citation2021) who identified these good practice policies with large potential for climate change mitigation globally based on the studies by Roelfsema et al. (Citation2018), Kriegler et al. (Citation2018), and Fekete et al. (Citation2015). The selected mitigation measures have been identified in consultation with national experts (van Soest et al. Citation2021). This section presents the list of promising measures, the methodology for determining the potential emission reductions of these measures, and the methodology for assessing the impact of these measures on other SDGs.
2.1. Promising climate change mitigation measures
provides a list of promising climate change mitigation measures, in the literature referred to as “good practice policies”. van Soest et al. (Citation2021) identified these measures that have been implemented in one or more countries and demonstrated noticeable impact on GHG emissions. The measures are designed in consultation with national experts, go beyond the traditional cost-optimal pathways, and can be implemented by 2030. If scaled up, these good practice policies might be a more feasible and convincing strategy for climate change mitigation than a purely cost-optimal approach (van Soest et al. Citation2021). In general, the measures we take in this report are technology centred. Even though behavioural change (van Sluisveld et al. Citation2016), societal transformative processes (Geels et al. Citation2015), and power and influence of stakeholders (Wyborn et al. Citation2019) can significantly contribute to GHG emission reduction and sustainable development, it is beyond the scope of this research. The measures listed in are not sufficient to meet the goals of the Paris Agreement but are a good starting point in the right direction.
Table 1. Promising climate change mitigation measures based on successful implementation (Dagnachew et al. Citation2021; van Soest et al. Citation2021).
Table 2. Scenario descriptions (Dagnachew et al. Citation2021).
2.2. Determining emission reduction potentials of measures
Scenarios have been key to assessing climate change and climate change policy for several decades. They are used to explore how the future may develop under various alternative conditions and have played an important role in guiding decision-making. Long-term model-based scenarios can be used as tools to explore actions needed to achieve climate goals. The IMAGE-integrated assessment model (Stehfest et al. Citation2014) is used to assess the mitigation potential of the promising measures in eleven world regions (North America, Central and South America, Europe, the Middle East and North Africa (MENA), South Asia, Southeast Asia, China, the Russian Federation, sub-Saharan Africa (SSA), Japan & Korea, and the rest of the world). The IMAGE framework contains TIMER energy-system simulation model (van Vuuren et al. Citation2006), IMAGE-Land Management model (Doelman et al. Citation2018) and the GISMO health model (P. Lucas et al. Citation2019). These models together represent interactions between society, the biosphere and the climate system to assess various sustainability issues. The model simulates socio-economic and environmental parameters on a geographical grid of 30 by 30 min or 5 by 5 min, depending on the specific variable. The IMAGE 3.0 modelling framework has been used in similar studies in the past, and the results are published in peer-reviewed articles (Daioglou et al. Citation2012; van Ruijven et al. Citation2016; van Sluisveld et al. Citation2016; de Boer and van Vuuren Citation2017; Doelman et al. Citation2018; P. Lucas et al. Citation2019). A detailed description of the model can be found in the Supplementary Information (SI_01).
The assessment is done under three scenarios:
The current policies (Curpol) scenario includes implementation of climate, energy and land-use policies that are ratified as of 1 July 2019. The socio-economic projections are based on the middle-of-the road projections of the shared socio-economic pathways (SSPs) (O’Neill et al. Citation2015). More details on the policies under this scenario can be found in the SI_02.
The good practice policies (GPP) scenario includes the good practice policies of the measures listed in in addition to the policies under the current policies scenario. All the climate change mitigation measures are universally implemented; however, the details of the implementation vary regionally based on input from country experts (see SI_02 for more details on scenario protocol). We have implemented this scenario for low- and middle-income regions as it already leads to significant emission reductions in these regions compared with the curpol scenario.
The Bridge scenario (Bridge) is an ambitious scenario that, in addition to all the measures under the GPP scenario, imposes a CO2 price starting from 2030 to further reduce emissions in line with the 2°C target. This scenario is implemented in high-income regions only, for two reasons. First, the emission reductions in the GPP scenario are modest for high-income regions. Second, as recognized under the UNFCCC principle of common but differentiated responsibilities and respective capabilities (CBDR-RC), countries have different duties and abilities to address the impacts of climate change; hence, we target more ambitious emission reductions for high-income countries.
These scenarios are implemented in the IMAGE modelling framework to quantify the emission reduction potentials of the promising measures aggregated by sectors in each region. The mitigation potential of the measures is calculated by determining the difference in sectoral emissions between the Curpol scenario and either the Bridge scenario for North America, Europe and Japan & Korea or the GPP scenario for all other regions. To allow a deeper discussion of the co-benefits and trade-offs, for each region, we only discuss the mitigation measures for the three sectors with the highest mitigation potential and the most direct co-benefits and trade-offs. Different regions could exhibit different co-benefits and/or adverse impacts either because the relative importance of the measures varies according to the regional characteristics or because a given mitigation measure has region-specific co-benefits or trade-offs. summarizes the scenarios.
2.3. Assessment of impact on SDGs
The SDGs have 17 goals and no less than 169 targets. Investigating the impact of 20 mitigation measures on all these targets is far beyond the scope of this study. Therefore, our focus is on the synergies and trade-offs between the measures and SDGs that are most frequently presented in the literature for selected world regions. To identify these synergies and trade-offs, we used as a starting point the Special IPCC report on 1.5°C IPCC (IPCC Citation2018), and Frischmann et al. (Citation2020) complemented with peer-reviewed literature. For peer-reviewed literature, we used a Scopus search with keywords ((measure or topic) AND (synergies OR trade-offs) AND (sustainable AND development)) AND (climate AND (change OR mitigation)) AND PUBYEAR > 2014 AND (LIMIT-TO (EXACTKEYWORD, “sustainable development“)) AND (LIMIT-TO (LANGUAGE, “English”)). Most SDGs can be represented by a single overall target. Exceptions are SDG8 (decent work for all and inclusive and sustainable economic growth), SDG9 (Research & Development and Fast access to economic hub), SDG11 (Decent housing for all and Improve air quality in cities), and SDG15 (sustainably manage forests/combat desertification and halt biodiversity loss), for which we defined multiple targets, as different synergies and trade-offs were identified for these targets. The 10 most cited articles in each sector are reviewed. SI_04 contains the list of reviewed publications.
We have limited the assessment on direct co-benefits and trade-offs only. Increased access to modern and sustainable energy in developing countries due to increased power generation from renewable energy demonstrates a direct co-benefit. Increasing access to electricity could lead to lower use of biomass, which indirectly could reduce deforestation and forest degradation (indirect co-benefit).
3. Co-benefits and trade-offs
This section presents the results of the study. We start by presenting the emission reduction potential of the promising mitigation measures listed in aggregated by sector for each world region. Subsequently, the potential positive and negative impacts of these climate change mitigation measures on other SDGs are discussed, emphasizing the regional context.
3.1. Emission reduction potential of promising mitigation measures
provides an overview of the sectoral GHG emissions in 2050 of the mitigation pathways compared to 2015 and the curpol scenario, and summarizes the GHG emission reductions by sector in 2050. Below, the emission reduction potential in each sector is discussed for the regions where this potential belongs to the top-three of the sectoral mitigation potential.
Figure 1. GHG emissions of mitigation pathways compared to 2015 and current policy scenario in 2050. This figure shows the result of the model assessment of the emission reduction potentials of the various measures in eleven world regions. It shows sectoral emissions in 2015 and in 2050 for two scenarios, the current policies scenario and either the bridge scenario (Europe, North America, and Japan & Korea) or the good practice policies scenario (all other regions). The difference in emissions between the current policies and the mitigation scenario provides an indication of the sectoral mitigation potential in that region.
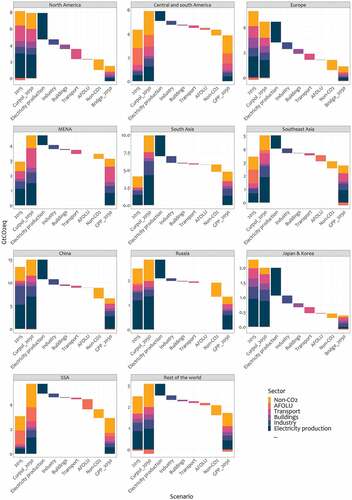
Figure 2. Contribution of sectors to GHG emission reduction under GPP and Bridge scenarios in 2050. The figure demonstrates that the sectors with the top three highest climate change mitigation potentials represent 75–95% of the emission reduction potential of the region.
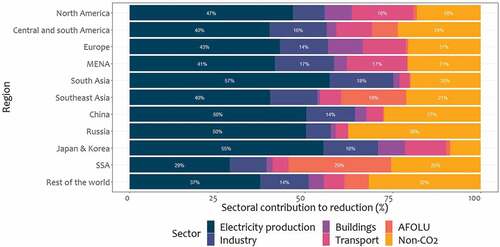
Power and heat generation is the single largest emitter accounting for about 36% of global fossil-fuel-related CO2 emissions in 2019 (Olivier and Peters Citation2020). As shown in , halting the installation of coal-fired plants without carbon capture and storage and increasing the share of renewables in electricity generation reduce emissions strongly in all regions. The global demand for electricity has been rapidly increasing since 1990 and this trend is projected to continue, driven by economic development, electrification of end-use services and climate change (Deetman et al. Citation2021). COVID-19 restrictions have resulted in a decrease in power generation, especially from carbon-intensive sources. However, there are signs of a rebound for coal-fired power plants outpacing low-carbon sources to fuel the recovery, especially in Asia (IEA Citation2021).
The industry sector, including process emissions, accounted for about 25% of global fossil-fuel-related CO2 emissions in 2019 (Olivier and Peters Citation2020). The promising measures in industry lead to considerable emission reduction, especially in Central and South America, Europe, South Asia, China, the Russian Federation and Japan & Korea (). The growth in energy consumption in South and Southeast Asia has been driven by increasing production in energy-intensive industries, while natural resource-related industries contribute significantly to the economic development of Central and South America (IRENA Citation2016). The manufacturing industry is the backbone of the Chinese industrialization with high energy consumption and GHG emissions (Liu et al. Citation2019), and it is the second largest CO2 emitting sector in the Russian Federation and Japan as well (Olivier and Peters Citation2020). For the industry sector, we looked at two promising climate change mitigation measures in the near- and medium-term: improving energy efficiency and installing carbon capture and storage (CCS). There is an opportunity to economically reduce global industrial emissions by 30% with existing technologies and that potential can grow to 60% with anticipated future technological innovations (ECE Citation2020). However, some industrial sectors, such as cement, steel and chemicals, may find it challenging to reduce emissions due to process emissions and the need for high-temperature heating. CCS has the potential to play a significant role in reducing emissions in these industries.
The Building sector contributed about 9% to global fossil-fuel-related CO2 emissions in 2019, without accounting for indirect emissions from power generation (Olivier and Peters Citation2020). The emission reduction potential of the promising measures is limited in absolute terms, either because emissions from buildings are relatively low to start with or because they already show a significant decline under the current policy scenario (). Still, in developed countries, renovations of existing buildings can strongly reduce energy demand. The largest share of the final energy consumption in buildings in most regions comes from space and water heating, which is predominantly fossil-fuel based. Therefore, replacing oil-based boilers in buildings by technologies such as heat pumps, electric boilers, solar heating systems and district heating is an important mitigation measure.
Almost 20% of global fossil-fuel-related CO2 emissions comes from the transport sector, with road transport taking the lion’s share (about 80%) of these emissions. In several regions, particularly North America and MENA, there is a lot of potential for mitigation in the transportation sector (). Already existing technologies have the potential to improve the average fuel efficiency of vehicles by 50% by 2050 relative to 2010 (IPCC Citation2015). The North American road transport sector is characterized by continuous growth in the number of passenger cars and the large stock of older vehicles. Effective measures to reduce emissions in the short- and medium-term include improvements in fuel efficiency and low-carbon fuel technologies as well as accelerated retirement of older, less fuel-efficient vehicles. Road transport dominates the sector in MENA, owing to the prevalence of fossil-fuel subsidies (Abbass et al. Citation2018). Increasing the share of non-fossil fuels in new vehicle sales is another climate change mitigation strategy. Bio-fuel powered vehicles emit fewer air pollutants than gasoline-powered vehicles, whereas electric vehicles are far more efficient than internal combustion vehicles and emit no tail-pipe emissions (Messagie Citation2014).
Aviation is a relatively small contributor to global CO2 emissions, responsible for around 2.5%. It is, however, among the fastest-growing emitters, and the demand is expected to bounce back after its dramatic fall due to COVID-19 to its pre-pandemic level within a couple of years (Sharmina et al. Citation2020). Retirement of less fuel-efficient aircraft and improving operational efficiency could result in a significant increase in overall fleet efficiency (IEA Citation2020). Promising measures for reducing GHG emissions in aviation include increasing efficiency and lowering the carbon intensity of fuel used in the sector.
Annual global emissions from land-use and land-cover changes between 2006 and 2015 are estimated to account for about 10% of total global CO2 emissions (Houghton and Nassikas Citation2017). Land-use-related measures are useful tools for climate change mitigation as they can remove CO2 from the atmosphere. Net forest conversion is one of the main sources of CO2 emissions in Southeast Asia, where forests are decreasing faster than any other region. Therefore, afforestation, reforestation, and preventing deforestation are all very effective ways to reduce CO2 levels in the atmosphere – either by reducing emissions (halting deforestation) or by increasing carbon uptake (afforestation and reforestation) (Calvin et al. Citation2016). Central and South America, Southeast Asia and SSA demonstrate significant potential for climate change mitigation by reducing land-use emissions that accounted for 33%, 29% and 37% of total regional GHG emissions, respectively, in 2015 ().
Finally, the promising measures to reduce non-CO2 emissions lead to large reductions in all regions. Non-CO2 GHG emissions included in this study are methane (CH4) and nitrous oxide (N2O). Methane is the largest contributor to climate change after CO2. The major sources of CH4 emissions vary by region: manure storage and enteric fermentation in North America and Central and South America, enteric fermentation in Europe and enteric fermentation and manure management in India due to the large livestock (Patra et al. Citation2013). Livestock breeds in SSA are numerous, and diverse and livestock manure is used as traditional fertilizer; however, there is a lack of adequate manure management, resulting in nutrient losses and CH4 emissions. Non-CO2 emissions are expected to rise as livestock populations grow, particularly in developing regions. Changing the present manure management practices could drastically cut livestock methane emissions. Some measures help reduce CH4 emission from enteric fermentation, reduction of ruminant livestock (for instance as a result of lifestyle change) or reduction in emission per unit of production (as the result of change in production practices or improving feed quality and digestibility, for instance; Calvin et al. Citation2016).
Venting and flaring of oil and natural gas is also identified as an important source of CH4 emissions (Citation2020). With expanded use of enhanced oil recovery and unconventional production amid advances in production technology, gas production in North America is expected to increase. Several countries in Central and South America are net exporters of oil and gas where venting and flaring produces significant amounts of CH4 as well as black carbon and N2O (Giwa et al. Citation2019). Similarly, the oil and gas industry in MENA is associated with high levels of CH4 emissions. Already existing technologies have the potential to reduce CH4 emissions from venting and flaring in various regions.
Another important source of CH4 emissions, particularly in Europe and South Asia, is waste from landfills (Gomez-Sanabria et al. Citation2022). There are several ways to economically reduce these emissions including increased recycling, energy recovery of biodegradable solid waste, and technologies for better conversion of waste to biomethane (Gambhir et al. Citation2017).
Nitrogen is essential to the productivity of crop, and animal production systems and the ever-growing demand for nitrogen-based fertilizers have led to a sharp increase in nitrogen pollution levels and related GHG emissions. There is evidence that nitrogen use in agriculture can be reduced without sacrificing crop yields through educating and training farmers on good management practices (Thompson et al. Citation2019). N2O is also generated as a by-product during nitric and adipic acid productions that are commonly utilized as feedstock in the manufacturing of fertilizer and synthetic fibres. Already available emission abatement technologies can contribute to reducing these emissions at low-cost without directly impacting production or other industrial emissions (Flederbach and Winch Citation2019).
3.2. Impact of mitigation measures on SDGs
In this section, the co-benefits and trade-offs of promising climate change mitigation measures on SDGs are discussed for each sector with emphasis on the three sectors with the largest mitigation potential in each region. The three sectors together represent 75–95% of the region’s potential for emission reduction in 2050 (see ). provides a summary of the co-benefits and trade-offs on the global level, and provides more detail on the regional level. More details for each region are given in the summary tables in SI_03.
These results are qualitatively evaluated and summarized in at the global level. The mitigation measures are listed in the rows and relevant SDG targets in the columns. Cells with an orange shading () indicate more potential trade-offs than potential co-benefits. A yellow shading (
) means the potential trade-offs are about equal to the potential co-benefits, depending on the chosen technology or the regional context. The lighter green shading (
) indicates more potential co-benefits than potential trade-offs and the darker green shading (
) indicates that the potential co-benefits far outweigh the potential trade-offs. Cells without colour indicate that there are no clear direct co-benefits or trade-offs. The summary table with more details and regional tables are presented in SI_03.
Figure 3. Co-benefits and trade-offs between climate change mitigation measures and SDGs by region. The figure presents the co-benefits and trade-offs aggregated by sector based on tables in SI_03. The scoring is done as follows; 2 points when the potential benefits outweigh the trade-offs, 1 point when there are more potential co-benefits than potential trade-offs, +1 and −1 points when the potential co-benefits and the potential trade-offs are about equal and −1 points when there are more potential trade-offs than potential co-benefits. The figure shows that in each region, there are considerably more co-benefits than trade-offs.
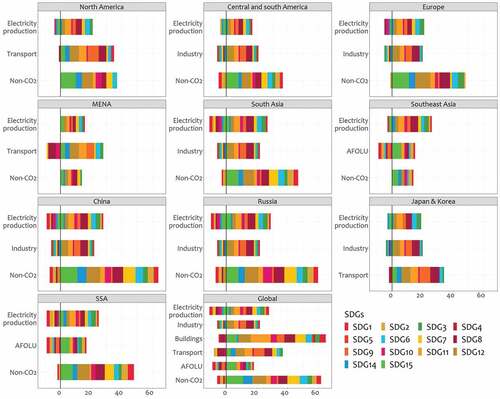
Table 3. Summary table of synergies and trade-offs between climate change mitigation measures and SDGs.
Power generation
Halting the installation of coal-fired plants without carbon capture and storage is one of the selected promising mitigation measures. Once built, coal-fired power plants can usually run for several decades, and the pollution from these plants is linked with severe environmental and human health consequences (Lv et al. Citation2016). Halting the construction of coal-fired plants without carbon capture and storage has therefore clear co-benefits with reducing air pollution (SDG11) and human health impacts (SDG3) (Lv et al. Citation2016), especially in dense urban centres of rapidly developing countries in Asia and SSA (McCollum et al. Citation2018). Since the coal mining process and coal-fired power plant operation are water-intensive, the resulting decline in coal use has a positive impact on water availability (SDG6) (DeNooyer et al. Citation2016). Combined with decreasing renewable energy prices, there are important economic benefits of shifting away from coal for electricity generation, particularly for industrialized regions such as North America, Europe, Russia, Japan & Korea. These benefits include more employment opportunities and avoiding the risk of stranded assets (Edwards et al. Citation2022), strengthening the efforts to achieve SDG8.
On the other hand, coal has been the major source of affordable and accessible energy in various developing countries, and it has been critical in supporting base-load electricity development (McHugh Citation2017). Restricting its use could thus impact the effort to provide universal access to electricity in Southeast Asia and SSA (SDG7). Coal reserves are found in almost every country in the world, and coal mining contributes significantly to several economies by providing employment and resources for export. The decline in coal-related activities could result in employment losses in the mining industry, especially in parts of North America, Europe, South Asia and the SSA, with impact on poverty alleviation in some of these regions, if not complemented by other policies (SDG1,8,10) (McHugh Citation2017).
Another promising climate change mitigation measure in the power sector is increasing the share of renewable power generation (solar, wind and hydro). Expanding renewable energy sources in the electricity generation has several co-benefits to other SDGs. It directly increases the share of renewable energy and improves energy efficiency in all regions (SDG7). Renewable energy technologies enable the provision of off-grid access to low-density settlements at much lower cost than on-grid systems, hence improving access to clean fuels in parts of Mexico, SSA and South Asia (SDG7) (Hoffacker et al. Citation2017). Accelerating the deployment of renewables also contributes to local economic growth (SDG8) by enhancing the demand for local services and goods if the technology is produced locally (Sharma and Balachandra Citation2015). In general, renewable energy technologies create more jobs, including jobs that are both decent and safe (SDG8) (Frischmann et al. Citation2020). By improving air quality (SDG11), switching from fossil-based energy sources to solar, wind, or hydro improves human health (SDG3) (Schwerhoff and Sy Citation2017). Replacing thermal power plants with solar- and wind-based renewable energy systems leads to considerably less water consumption and could enhance access to clean water and to reduce water scarcity in all regions (SDG6) (Hoffacker et al. Citation2017). Expanding renewable energy leads to less depletion of non-renewable resources, directly contributing to achieving SDG12, and ocean-based renewable energy systems enable marine resource protection (SDG14) (McCollum et al. Citation2018).
There are a few trade-offs associated with large-scale deployment of renewable energy systems, but these trade-offs are technology and location specific. Rapid deployment of relatively less matured technologies (for instance the expansion of offshore wind in Europe) or a preference for more advanced renewable technologies could result in an increase in energy prices in some regions. This affects affordability for the poor (SDG 1,7,10). In industrialized regions, particularly in North America and Europe, higher energy prices are also related to more stringent emission controls and higher feedstock costs. However, in some parts of North America, the cost of some renewable sources, such as onshore wind, is becoming very competitive with fossil fuels. The large land requirements of utility-scale renewable energy systems, such as solar farms and concentrated solar power, could affect issues surrounding availability of and access to land for local communities and land-sector sustainability (SDG15) (Hoffacker et al. Citation2017), and intensifies competition with other land services such as protecting biodiversity (SDG15) and agriculture (SDG2) (Fader et al. Citation2018). Large hydro dams have been linked to community displacements and adverse effects on natural ecosystems and their services (SDG15) (Fuso Nerini et al. Citation2018). There are also concerns regarding toxic elements released during the decommissioning and disposal of PV cells (SDG6,15), the noise from wind turbines that could harm human health (SDG3), ocean-based renewable energy systems competing with other marine activities (SDG14), and the wind turbine impact on bats and birds (SDG15) (Gasparatos et al. Citation2017).
Industry
The energy intensity of the industrial sector has declined steadily for decades, with some regional differences. The potential for further improvement is still large. The benefits of enhanced energy efficiency in the industry sector go beyond facilitating SDG7 target to increase energy access. It provides a way to balance economic competitiveness with climate change mitigation. Industrial energy efficiency on a large-scale can benefit the economy by lowering operating costs and boosting competitiveness and economic development in Central and South America, Europe, South Asia, China, the Russian Federation and Japan & Korea (SDG1,8,9,10) (Li and Tao Citation2017). It also increases productivity by cutting maintenance costs and improving production yields per unit of input (Johansson and Thollander Citation2018) as well as enabling the creation of new jobs in the energy service delivery sector (SDG8) (Malinauskaite et al. Citation2019). Other advantages include improved health (SDG3), new business opportunities (SDG1,8,10), improved energy security (SDG7), better environmental compliance, better working conditions (SDG8,11), enabling innovative and sustainable energy infrastructure (SDG9), improved air quality (SDG11) (Zhang et al. Citation2015a) particularly in the densely populated urban areas of rapidly developing regions as Central and South America, South Asia, and China (Zhang et al. Citation2015a), and minimizes waste in the whole value stream (SDG6,12) (Johansson and Thollander Citation2018).
The use of CCS is also recognized as an important promising climate change mitigation measure that can especially be implemented in carbon-intensive industries in Asia and Europe (Quader et al. Citation2016). CCS offers a cost-effective opportunity for emission reduction in new or existing refineries and iron, cement, ammonia, and chemical pulp industries (Quader et al. Citation2016). The supply chain of the CCS industry has the potential to become a significant source of employment, as well as help retain current jobs in related industries (SDG8). CCS could develop quality, reliable, sustainable and resilient infrastructure (SDG9), would overall lead to less air pollutants (SDG11) and promotes sustainable production (SDG12) (Wilberforce et al. Citation2019).
There are a few concerns about the implementation of CCS that could offset some of the benefits. These concerns include CO2 leakage from transportation and storage infrastructure that impacts human health and well-being and marine and coastal ecosystems (SDG3,14) (Rahman et al. Citation2017), a likely raise in production costs due to additional energy requirements (Quader et al. Citation2016) that could potentially affect electricity access and increase poverty in developing regions (SDG1,7), and expected increase in water use for cooling and processing (negatively impacting SDG6) (Smith et al. Citation2016). However, industrial CCS could improve energy efficiency if the processes are optimized (Bui et al. Citation2018), and some studies argue that there are several low-cost applications of CCS (Abanades et al. Citation2015).
Buildings
There are multiple benefits of improving the energy efficiency of appliances and buildings. It has the potential to reduce energy demand growth, especially in developing regions such as Asia and SSA, freeing up capital and capacity for expanding energy access (SDG7), while also lowering household energy expenditure and increasing disposable income (SDG1,10) (Aditya et al. Citation2017). By lowering local pollution and fossil-fuel use, efficient buildings promote health and quality of life (SDG3,11) (Aditya et al. Citation2017), improve energy security (SDG7), enhance competitiveness (SDG8), stimulate innovation (SDG9), and enable sustainable resource use (SDG12) (Nižetić et al. Citation2019). Efficiency improvements of cookstoves saves time and labour spent on fuel-wood collection and cooking meals and reduces health impacts that leads to a range of benefits for women in South Asia, Southeast Asia, and SSA (SDG5,3) (Mehetre et al. Citation2017). Energy efficiency measures create decent work opportunities (SDG8) (Allouhi et al. Citation2015); however, the net employment effect remains uncertain due to macroeconomic feedback.
Oil is an expensive fuel in most regions; hence, switching away from oil boilers could provide significant energy efficiency improvements (SDG7) and noticeable economic benefits that facilitates achieving SDG1 (Yang et al. Citation2018). In general, the transition away from oil use leads to lower emission of local air pollutants (SDG11) and prevents soil and groundwater pollution (SDG6,12,15) (Casasso et al. Citation2019) from oil leakage due to structural failure, corrosion, and loose fitting in the system. It also generates additional jobs in the building and heating industries (SDG8) and supports innovation to low-carbon heating technologies (SDG9) (Karner et al. Citation2017).
The main trade-off of the climate change mitigation measures in the building sector is related to the relatively high upfront investment costs (Allouhi et al. Citation2015). Even while these measures may result in lower energy costs, depending on how they are implemented, they may increase the risk of poverty (SDG1,10) and impede progress towards universal energy access (SDG7).
Transport
The co-benefits of improving fuel efficiency of passenger cars range from contributing to better access to energy services (SDG7) to improving local air quality (SDG11) by reducing particulate emissions, resulting in positive impacts on human health (SDG3) (Alam et al. Citation2018). The measure could also reduce water consumption and waste for transport fuel production (SDG6), reduce oil import and consumption (SDG12) and aid the transition to low-carbon transport. Measures to improve fuel efficiency could provide incentives for vehicle technology innovation (SDG9) (Simmons et al. Citation2015). Increasing efficiency could result in more jobs in the energy efficiency sector (SDG8), reduces fuel expenditure, increases disposable income (SDG10) and reduces energy use that improves resource efficiency (SDG12) (Lah Citation2015).
The large amount of investment required for passenger car efficiency improvements could serve as a barrier for vehicle manufacturers and consumers alike (SDG8) (Lah Citation2015). Likewise, the economy of oil-exporting regions, with high shares of fossil-fuel-related sectors in the economy, particularly in SSA and MENA, could be significantly impacted by the resulting decline in oil demand (SDG8,10) (Brand et al. Citation2020).
Wider adoption of non-fossil fuel vehicles, such as electric vehicles or vehicles on biofuels, decreases air pollution (SDG11) and related health effects (SDG3) (Du et al. Citation2017), increases the share of renewables in the global energy mix (SDG7), stimulates vehicle technology innovation and infrastructure development (SDG9), and promotes a more resilient and sustainable future for urban dwellers (SDG11) (Brand et al. Citation2020). If integrated with other policies that aim to offset increased vehicle use, fuel efficiency could result in lower use of fossil fuels, which would improve water and soil quality by reducing oil mining activities and oil spills (SDG6) and reduces in the overall environmental impact of the transport sector (SDG12,14,15) (Lah Citation2015). Electric cars also produce less noise pollution than conventional vehicles (Kester et al. Citation2018).
There are some trade-offs associated with the wider adoption of alternative fuels. If biofuels replace food crops, there could be a potential conflict with food security (SDG2). Rapid growth of electric vehicles could result in substantial variations in power demand if charging patterns are not properly managed (Kester et al. Citation2018), for instance in Europe and MENA, and disrupt electricity distribution networks and companies (impacting SDG7) (Brand et al. Citation2020). A transition to a predominantly electric car fleet could also force significant retraining of the existing workforce and restructuring of the automotive industry and disrupt the oil and gas sector (SDG8) (Brand et al. Citation2020).
Some of the co-benefits of increasing aviation energy efficiency include more efficient use of resources contributing to achieving SDG7 and SDG12, enhancing innovation into sustainability of the industry (SDG9) (Singh et al. Citation2019) and reducing air pollution (SDG11). Energy efficiency upgrades are generally labour-intensive and hence offer an opportunity for job creation (SDG8). The measure results in fuel efficiency gains that could deliver substantial cost savings for the aviation industry (Singh and Sharma Citation2016). An important trade-off is the disruption of the oil and gas industry (Brand et al. Citation2020), which could impact the economies of oil exporting countries, predominantly in MENA (SDG8,10).
Land use
Deforestation, mainly driven by commercial logging (illegal or poorly managed legal logging in South America, the Russian Federation, Southeast Asia, and SSA), large-scale and small-scale agriculture (for oil palm cultivation in Indonesia and Malaysia), cattle ranching (such as in Brazil), and logging for fuelwood (in large parts of SSA), present several challenges to sustainable development, and is the main driver of loss of species and biodiversity. Measures promoting afforestation and reforestation contribute directly to achieving the goal of halting biodiversity loss (SDG15) (Baumgartner Citation2019).
Forests and trees are also fundamental for food security and improved livelihoods (Bayrak and Marafa Citation2016). A substantial part of the household income for local households in developing countries comes from forest-related enterprises, contributing to achieving SDG1 as well as SDG10 (i.e. reducing relative poverty). Forests are important sources of nutritionally important foods, including wild fruits, vegetables and bush meat for nourishment (SDG2). Urban forests reduce harmful air pollutants (Lu et al. Citation2018) enabling the achievement of SDG3 and SDG11. Due to its labour-intensive nature as well as relatively low capital investment needs, targeted investments in forestry could create a large number of new job opportunities (SDG8,10) (Smith et al. Citation2019). However, large-scale afforestation could negatively affect SDG1 and SDG2 through increased food prices due to competition for land with food production (Doelman et al. Citation2020), especially in SSA and South Asia. It could also negatively affect local water supply by increased evapotranspiration (SDG6) (Lu et al. Citation2018).
There is synergy between halting deforestation and SDG15 that, amongst other goals, aims to combat desertification and halt and reverse land degradation. Additional co-benefits of the measure include reducing soil erosion and regulating weather that contributes to sustainable food production (SDG2) (Baumgartner Citation2019), improving local air quality (SDG11), and stimulating responsible consumption of natural resources (SDG12) (Smith et al. Citation2019). Deforestation generally increases erosion, which is associated with a higher sediment concentration in runoff and siltation (de Jong et al. Citation2018). Therefore, halting deforestation could improve access to clean water (SDG6). Halting deforestation calls for collaborations between forest management, local communities and the private sector that can foster local economic development (SDG1,10) (Nobre et al. Citation2016).
Underlying drivers of deforestation reflect wider social and economic issues, and hence, if they are not properly addressed, measures to halt deforestation could lead to reduced employment opportunities for households dependent on forest-based enterprises and smaller farmer-owned forests (impacting SDG1,8) (Baumgartner Citation2019). Amid the rapidly growing demand for food, deforestation in SSA is largely driven by smallholder agriculture. Hence, measures to halt deforestation could clash with the goal to eradicate hunger (SDG2) (Baumgartner Citation2019). Another common driver for deforestation is the growing expansion of road networks that connect production zones to markets. Promoting afforestation and halting deforestation could, therefore, impact market access and connectivity (SDG9) (Sy et al. Citation2015).
Non-CO2
Reducing non-CO2 emissions has considerable climate change mitigation potential in all regions. India, Brazil and China are the top three countries with the largest cattle inventory, and they are also in the top three of agricultural methane emissions. Most methane emissions in livestock farming come from cattle and selective breeding is one of the measures to reduce these emissions from enteric fermentation. The measure is cost-effective and therefore contributes to achieving SDG1 and SDG10 by increasing farm incomes. Other co-benefits include, enhancing the livestock sector’s contribution to national economic growth, facilitating global economic convergence (SDG8), contributing to food security as a result of increased supply of animal-sourced foods through better feeding and breeding (SDG2) (Mottet et al. Citation2018). The measure also reduces water demand from livestock systems (SDG6), improves productivity (Tait-Burkard et al. Citation2018) and feed-use efficiency (SDG12) (Mottet et al. Citation2018), and reduces and reverses land degradation for livestock expansion (SDG15). Although livestock present great opportunities for emission reduction, reducing livestock could adversely affect food prices and livelihoods of smallholder farmers, particularly in South Asia and the SSA (SDG1,2).
Livestock manure treatment with anaerobic digesters is a proven measure to reduce CH4 emissions. Converting livestock manure into biogas can facilitate access to modern and sustainable energy for millions of rural households, particularly in Central and South America, South Asia, Southeast Asia, China and SSA (SDG7) (Gupta et al. Citation2016). Bio-slurry, the by-product of biogas production, can be used as organic fertilizer (Gupta et al. Citation2016), promoting sustainable agriculture (SDG2,15). The measure also reduces nuisance smells and has a positive public health impact (SDG3), as well as limiting nutrient pollution in soil and water (SDG6). Proper management of manure contributes to reduction in waste and land and water pollution (SDG12) (Khoshnevisan et al. Citation2021) and creates additional economic activities (SDG8). However, livestock manure is the major fertilizer in many low-income countries, for instance in SSA. Large-scale implementation of anaerobic digesters could reduce their availability, which might affect the targets to end hunger and to decrease relative poverty (SDG1,2) (Gupta et al. Citation2016).
Large quantities of CH4 are released during coal mining and oil and natural gas production, processing, transmission and distribution. After a decline triggered by COVID-19, coal demand is increasing fast to meet the growing electricity demand in South Asia, resulting in an increase in CH4 emissions from coal mining. The technology to recover and use methane from coal mines is readily available. The recovered CH4, depending on the quality, can be used for power generation, district heating, boiler fuels, or purified and fed to natural gas distribution systems. Recovery and use of coal mine methane could reduce negative health impacts (SDG3), could enhance coal mine safety, could create additional economic activities (SDG8,10), could improve air and water quality (SDG11,14) (Haines et al. Citation2017), could promote responsible production and consumption (SDG12), could reduce the negative effects on the flora and fauna around the flaring site (SDG15) and could be a potential supply of a local clean energy source (SDG7) (Haines et al. Citation2017).
Reduction of venting and flaring has several co-benefits in addition to reducing non-CO2 emissions from gas and oil production. The captured gas facilitates universal energy services for all (SDG7) in SSA, contributes to improving well-being (SDG3) by reducing air and noise pollution (SDG11) and thermal radiation, and creates additional economic activities (SDG8) (Emam Citation2015). Halting venting and flaring also stimulates responsible consumption and production of natural resources (SDG12) and reduces the impact on land and ecosystem around the flaring site (SDG15) (Emam Citation2015). Vented gas is not visible to the naked eye, hence, if not properly monitored, restrictions in flaring could lead to an increase in venting. The cost of collecting and moving the gas could be a barrier to implementing this measure since the cost can initially be higher than the value of the gas itself (Soltanieh et al. Citation2016).
Excess nitrogen results in serious environmental, economic, and health impacts. As such, there are several potential benefits of reducing nitrogen use by improving efficiency in nitrogen application. It could reduce the cost of production, thereby improving food affordability (SDG2) and increasing incomes for farmers (SDG1,10) (Zhang et al. Citation2015b), as well as stimulating resource efficiency (SDG12). It also has the potential to reduce soil and groundwater contamination, eutrophication of freshwater and estuarine ecosystems (SDG6,14,15) (Zhang et al. Citation2015b). Reduced production costs, on the other hand, could increase the demand for food (also due to more waste) and feed, resulting in increased livestock production, partly offsetting the environmental benefits of nitrogen use efficiency (SDG15) (Herrero et al. Citation2021).
Reduced CH4 emissions from waste lead to less air pollution (SDG3,11), improve safety by reducing explosion and fire hazards (SDG11) and improve water quality by reducing pollution (SDG6,14) (Pujara et al. Citation2019). The gas can also be recovered and used for power generation or as a direct source of energy, providing an additional renewable energy source (SDG7). Collecting, converting and utilizing landfill gas generates income and creates jobs in the local community (SDG8) (Al-Hamamre et al. Citation2017). However, the measure needs high investment to build and instal landfill CH4 recovery systems (Al-Hamamre et al. Citation2017), and, if effective, CH4 gas recovery could overshadow waste reduction measures (SDG12).
Reducing N2O emissions from adipic/acid production in the industry offers a large potential for climate change mitigation amid projected growth in demand for fertilizer and synthetic fibres. Nitrogen oxide causes air pollution that is harmful to both human respiratory system and the environment when it dissolves in water, it forms acid rain, which damages vegetation and terrestrial and aquatic ecosystems. Reducing it thus has co-benefits with the sustainable development goals for clean water and sanitation (SDG6), life under water (SDG14) and life on land (SDG15) (Zhang et al. Citation2015b).
4. Discussion and conclusions
The aim of this paper is to identify the impact of climate change mitigation measures on other SDGs. To do this, we have focussed on the 20 best practice policies to reduce emissions in electricity generation, transport, buildings, industry, land use, and non-CO2 emissions identified by van Soest et al. (Citation2021). We evaluated the regional climate change mitigation potentials of these measures by comparing the emissions of the current policy scenario to the good practice policies (developing regions) or Bridge (developed regions) scenario. The most important impacts of these measures on other SDGs for eleven world regions have been identified based on an extensive literature review, using the Special IPCC report on 1.5°C (IPCC Citation2018) and Frischmann et al. (Citation2020) as a starting point. In addition to focusing on best practice policies that have already been proven successful in one or more countries, this paper adds the regional potentials of these measures and the regional difference in synergies and trade-offs (more details are presented in SI_03 and summarized in ).
The results show that measures taken to mitigate climate change also impact other SDGs. The identified co-benefits and trade-offs can be used to facilitate coherence of mitigation measures at various levels of decision-making while at the same time offering a systemic view of the impact of these measures in relation to the SDGs. However, the focus of the study is on the selected mitigation measures and the most direct impacts on SDGs; other related measures and practices that could influence sustainable development and the identification of indirect potential benefits and trade-offs were not considered. Moreover, the selected measures have a strong technological focus and the role of behavioural change, societal transformative processes or the role of power is not addressed. Some studies show that these factors can influence sustainable development and climate change mitigation. The measures we have analysed are tried and tested in some regions. Nonetheless, the effectiveness of these best practices depends on understanding the local context and, in some cases, implementing complementary policies that could support and protect vulnerable segments of society.
We conclude that, in line with the findings of other similar studies, the number of co-benefits of mitigation measures on other SDGs is larger than the number of trade-offs, not only at the global level as already concluded by earlier studies (IPCC Citation2018; Frischmann et al. Citation2020) but also at regional levels. The existing global studies show that the implementation of climate change mitigation options leads to multiple synergies across a range of SDGs, while at the same time, there could be trade-offs depending on how and when these measures are implemented. However, the regional differentiation of the impacts is limited in these studies. Out of the 20 measures analysed in this study, in all regions, the highest number of potential co-benefits are identified for increasing the share of renewable energy in electricity generation. The identified climate change mitigation measures in the building sector demonstrated several potential co-benefits for other SDGs. Some potential trade-offs are identified for measures in the industry and transport sectors, but again, the number of potential synergies is higher. In land use and non-CO2 sectors, the number of potential co-benefits and trade-offs depends on the type of measure and regional characteristics.
As mentioned earlier, our focus was on the most direct synergies and trade-offs observed. Studies show that the characteristics of these interactions could be influenced by several factors including the governance approach, the institutional context, the geographical place and scale, the time horizon, and the availability of natural resources (Nilsson et al. Citation2018; Iacobuţă et al. Citation2021). The regional diversity in this study partly addresses some of these factors. Our focus is on the impact in 2030, the target year for achieving SDGs, which limits our ability to capture the positive effects of short-term actions on long-term socio-economic development. Below, we discuss the important potential trade-offs identified in this study that can still be mitigated with targeted policy interventions.
The selected climate change mitigation measures show a much higher number of potential co-benefits than trade-offs in all regions. The magnitude of these co-benefits and trade-offs varies according to regional characteristics and socio-economic context. The potential trade-offs in North America, Europe, and Central and South America are largely related to technology choices that could exacerbate social inequality and impact biodiversity. Some of the mitigation measures could impede efforts to alleviate poverty, eradicate hunger and improve well-being in SSA, South Asia, and Southeast Asia. These impacts can be minimized by implementing complementary policies to protect the poor and vulnerable segment of society from increasing food and energy prices. The mitigation measures in MENA, if properly implemented, could benefit the effort to diversify oil-dependent economies and speed up reform. However, the resulting decline in oil consumption could stall regional economic reforms, deepening inequality.
Measures in electricity production show high mitigation potentials coupled with many potential co-benefits for other SDGs in all regions. In addition to contributing to SDG 7, increasing the share of renewable energy in power generation shows potential co-benefits with poverty alleviation, fighting hunger, improving access to healthcare, education, and clean water, and protecting life on land and in water. However, the choice of technology is relevant for two reasons; i) matured technologies could result in increasing electricity prices, and ii) some renewable technologies are associated with negative social and environmental impacts. Large hydropower dams, for instance, could lead to community displacements, as well as loss of natural forests and biodiversity. Reducing coal-fired power generation negatively impacts employment in coal-mining industries in North America, Europe, Asia and SSA and could, at least in the short run, lead to increasing electricity prices. These trade-offs can be addressed by stronger regulations, along with market-based instruments and pro-poor policies.
Land-use related climate change mitigation measures show relatively many potential trade-offs with other SDGs in low-income regions. The promising mitigation measures to reduce CO2 emissions from land use and non-CO2 emissions from agriculture, livestock, and waste are important for global emission reductions. Reducing deforestation and increasing reforestation have clear co-benefits with biodiversity and environmental SDGs. However, if not managed carefully, these measures could undermine sustainable development by threatening food and water security, livelihood, and infrastructure developments in SSA and Southeast Asia. To mitigate these impacts, the forestation measures could be complemented by pro-poor policies to strengthen land and resource rights, capabilities and local decision-making, small-scale farmer credit programmes and transfer payments to poor rural inhabitants for ecosystem services.
Availability of data
Data available on request from the authors
Acknowledgments
The research presented in this paper was funded by the Dutch Ministry of Foreign Affairs, the Netherlands, through its Directorate-General of Trade and International Cooperation. The authors would like to acknowledge the contribution of the work done by Heleen van Soest (van Soest et al. Citation2021) and Detlef van Vuuren to this article.
Disclosure statement
No potential conflict of interest was reported by the author(s).
References
- Abanades JC, Arias, B, Lyngfelt, A, Mattisson, T, Wiley, D. E., Li, H, Ho, M.T, Mangano, E, Brandani, S. 2015. Emerging CO2 capture systems. Int J Greenhouse Gas Control. 40:126–166.
- Abbass RA, Kumar P, El-Gendy A. 2018. An overview of monitoring and reduction strategies for health and climate change related emissions in the Middle East and North Africa region. Atmos Environ. 175:33–43. doi:10.1016/j.atmosenv.2017.11.061.
- Aditya L, Mahlia TMI, Rismanchi B, Ng HM, Hasan MH, Metselaar HSC, Muraza O, Aditiya HB. 2017. A review on insulation materials for energy conservation in buildings. Renew Sustain Energy Rev. 73:1352–1365. doi:10.1016/j.rser.2017.02.034.
- Alam MS, Hyde B, Duffy P, McNabola A. 2018. Analysing the co-benefits of transport fleet and fuel policies in reducing PM2.5 and CO2 emissions. J Clean Prod. 172:623–634. doi:10.1016/j.jclepro.2017.10.169.
- Al-Hamamre Z, Saidan M, Hararah M, Rawajfeh K, Alkhasawneh HE, Al-Shannag M. 2017. Wastes and biomass materials as sustainable-renewable energy resources for Jordan. Renew Sustain Energy Rev. 67:295–314. doi:10.1016/j.rser.2016.09.035.
- Allouhi A, El Fouih Y, Kousksou T, Jamil A, Zeraouli Y, Mourad Y. 2015. Energy consumption and efficiency in buildings: current status and future trends. J Clean Prod. 109:118–130. doi:10.1016/j.jclepro.2015.05.139.
- Baumgartner RJ. 2019. Sustainable development goals and the forest sector—a complex relationship. Forests. 10(2):152. doi:10.3390/f10020152.
- Bayrak M, Marafa L, Ten years of REDD+: a critical review of the impact of REDD+ on forest-dependent communities. Sustainability, 2016. 8(7).
- Brand C, Anable, J, Ketsopoulou, I, Watson, J. 2020. Road to zero or road to nowhere? Disrupting transport and energy in a zero carbon world. Energy Policy:139.
- Bui M, Adjiman, C.S, Bardow, A, Anthony, E.J, Boston, A, Brown, S, Fennell, P.S, Fuss, S, Galindo, A, Hackett, L.A, Hallett, J.P, Herzog, H.J, Jackson, G, Kemper, J, Krevor, S, Maitland, G.C, Matuszewski, M, Metcalfe, I.S, Petit, C, Puxty, G, Reimer, J, et al. 2018. Carbon capture and storage (CCS): the way forward. Energy Environ Sci. 11(5):1062–1176.
- Calel R, Mahdavi P. 2020. Opinion: the unintended consequences of antiflaring policies-and measures for mitigation. Proc Natl Acad Sci U S A. 117(23):12503–12507. doi:10.1073/pnas.2006774117.
- Calvin KV, Beach R, Gurgel A, Labriet M, Loboguerrero Rodriguez AM. 2016. Agriculture, forestry, and other land-use emissions in Latin America. Energy Econ. 56:615–624. doi:10.1016/j.eneco.2015.03.020.
- Campagnolo L, Davide M. 2019. Can the Paris deal boost SDGs achievement? An assessment of climate mitigation co-benefits or side-effects on poverty and inequality. World Dev. 122:96–109. doi:10.1016/j.worlddev.2019.05.015.
- Casasso A, Capodaglio P, Simonetto F, Sethi R. 2019. Environmental and economic benefits from the phase-out of residential oil heating: a study from the Aosta Valley Region (Italy). Sustainability. 11(13):3633. doi:10.3390/su11133633.
- Dagnachew A.G, Hof, A, van Soest, H, van Vuuren, D, et al. 2021. Climate change measures and sustainable development goals: mapping synergies and trade-offs to guide multi-level decision-making. The Hague: PBL Netherlands Environmental Assessment Agency; p. 55.
- Daioglou V, van Ruijven BJ, van Vuuren DP. 2012. Model projections for household energy use in developing countries. Energy. 37(1):601–615. doi:10.1016/j.energy.2011.10.044.
- de Boer HS, van Vuuren D. 2017. Representation of variable renewable energy sources in TIMER, an aggregated energy system simulation model. Energy Econ. 64:600–611.
- Deetman S, de Boer, H.S, Van Engelenburg, M, van der Voet, E, van Vuuren, D.P , et al. 2021. Projected material requirements for the global electricity infrastructure – generation, transmission and storage. Resources. Conserv Recyling:164. https://doi.org/10.1016/j.resconrec.2020.105200
- de Jong W, Pokorny B, Katila P, Galloway G, Pacheco P. 2018. Community forestry and the sustainable development goals: a two way street. Forests. 9(6):331. doi:10.3390/f9060331.
- DeNooyer TA, Peschel JM, Zhang Z, Stillwell AS. 2016. Integrating water resources and power generation: the energy–water nexus in Illinois. Appl Energy. 162:363–371. doi:10.1016/j.apenergy.2015.10.071.
- Doelman JC, Stehfest E, Tabeau A, van Meijl H, Lassaletta L, Gernaat DEHJ, Hermans K, Harmsen M, Daioglou V, Biemans H. 2018. Exploring SSP land-use dynamics using the IMAGE model: regional and gridded scenarios of land-use change and land-based climate change mitigation. Glob Environ Change. 48:119–135. doi:10.1016/j.gloenvcha.2017.11.014.
- Doelman JC, Stehfest E, Vuuren DP, Tabeau A, Hof AF, Braakhekke MC, Gernaat DEHJ, Berg M, Zeist W-J, Daioglou V, et al. 2020. Afforestation for climate change mitigation: potentials, risks and trade-offs. Glob Chang Biol. 26(3):1576–1591. doi:10.1111/gcb.14887
- Du J, Ouyang M, Chen J. 2017. Prospects for Chinese electric vehicle technologies in 2016–2020: ambition and rationality. Energy. 120:584–596. doi:10.1016/j.energy.2016.11.114.
- ECE. 2020. Draft Industrial Energy Efficiency Action Plan, and assessment of the role of the United Nations Economic Commission for Europe in delivering on it. Geneva: Economic Commission for Europe.
- Editorial. 2020. Time to revise the Sustainable Development Goals. Nature. 583:331–332.
- Edwards MR, Cui R, Bindl M, Hultman N, Mathur K, McJeon H, Iyer G, Song J, Zhao A. 2022. Quantifying the regional stranded asset risks from new coal plants under 1.5 °C. Environ Res Lett. 17(2):024029. doi:10.1088/1748-9326/ac4ec2.
- Emam A. 2015. Gas flaring in industry: an overview. Petroleum Coal. 57(7):23.
- Fader M, Cranmer, C, Lawford, R, Engel-Cox, J, et al. 2018. Toward an understanding of synergies and trade-offs between water, energy, and food SDG targets. Front Environ Sci:6. https://doi.org/10.3389/fenvs.2018.00112
- Fekete H, Roelfsema, M, Höhne, N, den Elzen, M, Forsell, N, Becerra, S, et al. 2015. Impacts of good practice policies on regional and global greenhouse gas emissions. Cologne, Germany: PBL/NewClimate Institute/IIASA.
- Flederbach B, Winch J, Adipic acid production issue paper. 2019, ClimeCo Corporation: Boyertown (Pennsylvania).
- Frischmann CJ, Mehra, M, Allard, R, Bayuk, K, Gouveia, JP, Gorman, MR, et al. 2020. Drawdown’s “system of solutions” helps to achieve the SDGs. Partnerships for the Goals:1–25 .https://doi.org/10.1007/978-3-319-71067-9_100-1
- Fujimori S, Hasegawa T, Krey V, Riahi K, Bertram C, Bodirsky BL, Bosetti V, Callen J, Després J, Doelman J, et al. 2019. A multi-model assessment of food security implications of climate change mitigation. Nat Sustain. 2(5):386–396. doi:10.1038/s41893-019-0286-2
- Fuso Nerini F, Tomei J, To LS, Bisaga I, Parikh P, Black M, Borrion A, Spataru C, Castán Broto V, Anandarajah G. 2018. Mapping synergies and trade-offs between energy and the Sustainable Development Goals. Nat Energy. 3(1):10–15. doi:10.1038/s41560-017-0036-5.
- Gambhir A, Napp, T, Hawkes, A, Höglund-Isaksson, L, Winiwarter, W, Purohit, P, Wagner, F, Bernie, D, Lowe, J, et al. 2017. The contribution of non-CO2 greenhouse gas mitigation to achieving long-term temperature goals. Vol. 10 5, . doi:10.3390/en10050602.
- Gasparatos A, Doll, CNH, Esteban, M, Ahmed, A, Olang, TA, et al. 2017. Renewable energy and biodiversity: implications for transitioning to a Green Economy. Renew Sustain Energy Rev. 70:161–184. https://doi.org/10.1016/j.rser.2016.08.030.
- Geels FW, McMeekin A, Mylan J, Southerton D. 2015. A critical appraisal of Sustainable Consumption and Production research: the reformist, revolutionary and reconfiguration positions. Glob Environ Change. 34:1–12. doi:10.1016/j.gloenvcha.2015.04.013.
- Giwa SO, Nwaokocha CN, Kuye SI, Adama KO. 2019. Gas flaring attendant impacts of criteria and particulate pollutants: a case of Niger Delta region of Nigeria. JKSUES. 31(3):209–217. doi:10.1016/j.jksues.2017.04.003.
- Gomez-Sanabria A, Kiesewetter G, Klimont Z, Schoepp W, Haberl H. 2022. Potential for future reductions of global GHG and air pollutants from circular waste management systems. Nat Commun. 13(1):106. doi:10.1038/s41467-021-27624-7.
- Gupta KK, Aneja KR, Rana D. 2016. Current status of cow dung as a bioresource for sustainable development. Bioresour Bioprocess. 3(1).
- Haines A, Amann M, Borgford-Parnell N, Leonard S, Kuylenstierna J, Shindell D. 2017. Short-lived climate pollutant mitigation and the Sustainable Development Goals. Nat Clim Chang. 7(12):863–869. doi:10.1038/s41558-017-0012-x.
- Herrero M, Thornton PK, Mason-D’Croz D, Palmer J, Bodirsky BL, Pradhan P, Barrett CB, Benton TG, Hall A, Pikaar I. 2021. Articulating the effect of food systems innovation on the Sustainable Development Goals. Lancet Planet Health. 5(1):e50–e62. doi:10.1016/S2542-5196(20)30277-1.
- Hoffacker MK, Allen MF, Hernandez RR. 2017. Land-sparing opportunities for solar energy development in agricultural landscapes: a case study of the Great Central Valley, CA, United States. Environ Sci Technol. 51(24):14472–14482. doi:10.1021/acs.est.7b05110.
- Houghton RA, Nassikas AA. 2017. Global and regional fluxes of carbon from land use and land cover change 1850–2015. Global Biogeochem Cycles. 31(3):456–472. doi:10.1002/2016GB005546.
- Iacobuţă GI, Höhne, N, van Soest, HL, Leemans, R, et al., Transitioning to low-carbon economies under the 2030 agenda: minimizing trade-offs and enhancing co-benefits of climate-change action for the SDGs. Sustainability, 2021. 13(19). https://doi.org/10.3390/su131910774
- IEA. 2020. Energy technology perspectives 2020. France: International Energy Agency.
- IEA. 2021. Global Energy Review 2021: assessing the effects of economic recoveries on global energy demand and CO2 emissions in 2021. France: International Energy Agency.
- IPCC, Climate Change 2014: synthesis report, in Contribution of Working Groups I, II and III to the Fifth Assessment Report of the Intergovernmental Panel on Climate Change. 2015, IPCC: Geneva (Switzerland). p. 151.
- IPCC, Global Warming of 1.5°C. An IPCC Special Report on the impacts of global warming of 1.5°C above pre-industrial levels and related global greenhouse gas emission pathways, in the context of strengthening the global response to the threat of climate change, sustainable development, and efforts to eradicate poverty , et al, Editors. 2018 Global Warming of 1.5°C. Cambridge, UK and New York, NY, USA: Cambridge University Press. https://doi.org/10.1017/9781009157940
- IRENA. 2016. Renewable energy market analysis: Latin America. Abu Dhabi:IRENA.
- Johansson MT, Thollander P. 2018. A review of barriers to and driving forces for improved energy efficiency in Swedish industry–recommendations for successful in-house energy management. Renew Sustain Energy Rev. 82:618–628. doi:10.1016/j.rser.2017.09.052.
- Karner K, Dißauer C, Enigl M, Strasser C, Schmid E. 2017. Environmental trade-offs between residential oil-fired and wood pellet heating systems: forecast scenarios for Austria until 2030. Renew Sustain Energy Rev. 80:868–879. doi:10.1016/j.rser.2017.05.242.
- Kester J, Noel L, Zarazua de Rubens G, Sovacool BK. 2018. Policy mechanisms to accelerate electric vehicle adoption: a qualitative review from the Nordic region. Renew Sustain Energy Rev. 94:719–731. doi:10.1016/j.rser.2018.05.067.
- Khoshnevisan B, Duan, N, Tsapekos, P, Awasthi, MK, Liu, Z, Mohammadi, A, Angelidaki, I, Tsang, DCW, Zhang, Z, Pan, J, Ma, L, Aghbashlo, M, Tabatabaei, M, Liu, H, et al. 2021. A critical review on livestock manure biorefinery technologies: sustainability, challenges, and future perspectives. Renew Sustain Energy Rev:135.
- Kriegler E, Bertram C, Kuramochi T, Jakob M, Pehl M, Stevanović M, Höhne N, Luderer G, Minx JC, Fekete H. 2018. Short term policies to keep the door open for Paris climate goals. Environ Res Lett. 13(7):074022. doi:10.1088/1748-9326/aac4f1.
- Lah O. 2015. The barriers to low-carbon land-transport and policies to overcome them. Eur Trans Res Rev. 7(1). doi:10.1007/s12544-014-0151-3.
- Li M-J, Tao W-Q. 2017. Review of methodologies and policies for evaluation of energy efficiency in high energy-consuming industry. Appl Energy. 187:203–215. doi:10.1016/j.apenergy.2016.11.039.
- Liu J, Yang, Q, Zhang, Y, Sun, W, Xu, Y, et al. 2019. Analysis of CO2 emissions in China’s manufacturing industry based on extended logarithmic mean division index decomposition. Sustainability. 11(1).
- Lucas, P. L., Hilderink, H. B. M., Janssen, P. H. M., Kc, S., van Vuuren, D. P., & Niessen, L. (2019). Future impacts of environmental factors on achieving the SDG target on child mortality—A synergistic assessment. Global Environmental Change, 57. https://doi.org/10.1016/j.gloenvcha.2019.05.009
- Lu C, Zhao T, Shi X, Cao S. 2018. Ecological restoration by afforestation may increase groundwater depth and create potentially large ecological and water opportunity costs in arid and semiarid China. J Clean Prod. 176:1213–1222. doi:10.1016/j.jclepro.2016.03.046.
- Lv C, Xu J, Xie H, Zeng Z, Wu Y. 2016. Equilibrium strategy based coal blending method for combined carbon and PM10 emissions reductions. Appl Energy. 183:1035–1052. doi:10.1016/j.apenergy.2016.09.028.
- Malinauskaite J, Jouhara H, Ahmad L, Milani M, Montorsi L, Venturelli M. 2019. Energy efficiency in industry: EU and national policies in Italy and the UK. Energy. 172:255–269. doi:10.1016/j.energy.2019.01.130.
- McCollum DL, Echeverri LG, Busch S, Pachauri S, Parkinson S, Rogelj J, Krey V, Minx JC, Nilsson M, Stevance A-S, et al. 2018. Connecting the sustainable development goals by their energy inter-linkages. Environ Res Lett. 13(3):033006. doi:10.1088/1748-9326/aaafe3
- McHugh L. 2017. World energy needs: a role for coal in the energy mix. In: Hester RE, Harrison RM, editors. Coal in the 21st century: energy needs, chemicals and environmental controls. Cambridge: Royal Society of Chemistry. p. 1–29. doi:10.1039/9781788010115.
- Mehetre SA, Panwar NL, Sharma D, Kumar H. 2017. Improved biomass cookstoves for sustainable development: a review. Renew Sustain Energy Rev. 73:672–687. doi:10.1016/j.rser.2017.01.150.
- Messagie M. 2014. Life cycle analysis of the climate impact of electric vehicles (Brussels: Vrije Universiteit Brussel). Vrije Universiteit Brussel - Research Group MOBI.
- Mottet A, Teillard F, Boettcher P, De’ Besi G, Besbes B. 2018. Review: domestic herbivores and food security: current contribution, trends and challenges for a sustainable development. Animal. 12(s2):s188–s198. doi:10.1017/S1751731118002215.
- Nilsson M, Chisholm E, Griggs D, Howden-Chapman P, McCollum D, Messerli P, Neumann B, Stevance A-S, Visbeck M, Stafford-Smith M, et al. 2018. Mapping interactions between the sustainable development goals: lessons learned and ways forward. Sustain Sci. 13(6):1489–1503. doi:10.1007/s11625-018-0604-z
- Nižetić S, Djilali, N, Papadopoulos, A, Rodrigues, JJPC, et al. 2019. Smart technologies for promotion of energy efficiency, utilization of sustainable resources and waste management. J Clean Prod. 231:565–591. https://doi.org/10.1016/j.jclepro.2019.04.397
- Nobre CA, Sampaio G, Borma LS, Castilla-Rubio JC, Silva JS, Cardoso M. 2016. Land-use and climate change risks in the Amazon and the need of a novel sustainable development paradigm. Proc Natl Acad Sci U S A. 113(39):10759–10768. doi:10.1073/pnas.1605516113.
- Olivier JGJ, Peters JAHW, Trends in global CO2 and total greenhouse gas emissions: 2020 report. 2020, The Hague: PBL Netherlands Environmental Assessment Agency.
- O’Neill BC, Kriegler, E, Ebi, KL, Kemp-Benedict, E, Riahi, K, Rothman, DS, van Ruijven, BJ, van Vuuren, DP, Birkmann, J, Kok, K, Levy, M, Solecki, W, et al. 2015. The roads ahead: narratives for shared socioeconomic pathways describing world futures in the 21st century. Glob Environ Change.
- Patra PK, Canadell JG, Houghton RA, Piao SL, Oh N-H, Ciais P, Manjunath KR, Chhabra A, Wang T, Bhattacharya T. 2013. The carbon budget of South Asia. Biogeosciences. 10(1):513–527. doi:10.5194/bg-10-513-2013.
- Pradhan P, Costa L, Rybski D, Lucht W, Kropp JP. 2017. A systematic study of sustainable development goal (SDG) interactions. Earth’s Future. 5(11):1169–1179. doi:10.1002/2017EF000632.
- Pujara Y, Pathak P, Sharma A, Govani J. 2019. Review on Indian Municipal Solid Waste Management practices for reduction of environmental impacts to achieve sustainable development goals. J Environ Manage. 248:109238. doi:10.1016/j.jenvman.2019.07.009.
- Quader MA, Ahmed S, Raja Ghazilla RA, Ahmed S, Dahari M. 2016. Evaluation of criteria for CO2 capture and storage in the iron and steel industry using the 2-tuple DEMATEL technique. J Clean Prod. 120:207–220. doi:10.1016/j.jclepro.2015.10.056.
- Rafaj P, Kiesewetter, G, Krey, V, Schöpp, W, Bertram, C, Drouet, L, Fricko, O, Shinichiro, F, Harmsen, M, Hilaire, J, Huppmann, D, Klimont, Z, Kolp, P, Aleluia Reis, L, van Vuuren, DP, et al. 2021. Air quality and health implications of 1.5–2°C climate pathways under considerations of ageing population: a multi-model scenario analysis. Environ Res Lett. 16(4):e045005.
- Rahman FA, Aziz MMA, Saidur R, Bakar WAWA, Hainin MR, Putrajaya R, Hassan NA. 2017. Pollution to solution: capture and sequestration of carbon dioxide (CO2) and its utilization as a renewable energy source for a sustainable future. Renew Sustain Energy Rev. 71:112–126. doi:10.1016/j.rser.2017.01.011.
- Roelfsema M, Fekete H, Höhne N, den Elzen M, Forsell N, Kuramochi T, de Coninck H, van Vuuren DP. 2018. Reducing global GHG emissions by replicating successful sector examples: the ‘good practice policies’ scenario. Climate Policy. 18(9):1103–1113. doi:10.1080/14693062.2018.1481356.
- Schwerhoff G, Sy M. 2017. Financing renewable energy in Africa – key challenge of the sustainable development goals. Renew Sustain Energy Rev. 75:393–401. doi:10.1016/j.rser.2016.11.004.
- Sharma T, Balachandra P. 2015. Benchmarking sustainability of Indian electricity system: an indicator approach. Appl Energy. 142:206–220. doi:10.1016/j.apenergy.2014.12.037.
- Sharmina M, Edelenbosch, OY, Wilson, C, Freeman, R, Gernaat, DEHJ, Gilbert, P, Larkin, A, Littleton, EW, Traut, M, van Vuuren, DP, Vaughan, NE, Wood, FR, Le Quéré, C, et al. 2020. Decarbonising the critical sectors of aviation, shipping, road freight and industry to limit warming to 1.5–2°C. Climate Policy:1–20.
- Simmons RA, Shaver GM, Tyner WE, Garimella SV. 2015. A benefit-cost assessment of new vehicle technologies and fuel economy in the U.S. market. Appl Energy. 157:940–952. doi:10.1016/j.apenergy.2015.01.068.
- Singh GG, Cisneros-Montemayor AM, Swartz W, Cheung W, Guy JA, Kenny T-A, McOwen CJ, Asch R, Geffert JL, Wabnitz CCC. 2018. A rapid assessment of co-benefits and trade-offs among Sustainable Development Goals. Marine Policy. 93:223–231. doi:10.1016/j.marpol.2017.05.030.
- Singh V, Sharma SK. 2016. Analyzing the moderating effects of respondent type and experience on the fuel efficiency improvement in air transport using structural equation modeling. Eur Trans Res Rev. 8(2). doi:10.1007/s12544-016-0199-3.
- Singh J, Sharma SK, Srivastava R. 2019. AHP-Entropy based priority assessment of factors to reduce aviation fuel consumption. Int J Syst Assur Eng Manag. 10(2):212–227.
- Smith P, Adams J, Beerling DJ, Beringer T, Calvin KV, Fuss S, Griscom B, Hagemann N, Kammann C, Kraxner F. 2019. Land-management options for greenhouse gas removal and their impacts on ecosystem services and the sustainable development goals. Annu Rev Environ Resour. 44(1):255–286. doi:10.1146/annurev-environ-101718-033129.
- Smith P, Davis SJ, Creutzig F, Fuss S, Minx J, Gabrielle B, Kato E, Jackson RB, Cowie A, Kriegler E. 2016. Biophysical and economic limits to negative CO2 emissions. Nat Clim Chang. 6(1):42–50. doi:10.1038/nclimate2870.
- Soergel B, Kriegler E, Weindl I, Rauner S, Dirnaichner A, Ruhe C, Hofmann M, Bauer N, Bertram C, Bodirsky BL, et al. 2021. A sustainable development pathway for climate action within the UN 2030 Agenda. Nat Clim Chang. 11(8):656–664. doi:10.1038/s41558-021-01098-3
- Soltanieh M, Zohrabian A, Gholipour MJ, Kalnay E. 2016. A review of global gas flaring and venting and impact on the environment: case study of Iran. Int J Greenhouse Gas Control. 49:488–509. doi:10.1016/j.ijggc.2016.02.010.
- Stehfest E, van Vuuren, DP, Kram, T, Bouwman, L, et al. 2014. Integrated Assessment of Global Environmental Change with IMAGE 3.0: model description and policy applications. The Hague: PBL Netherlands Environmental Assessment Agency.
- Sy VD, Herold M, Achard F, Beuchle R, Clevers JGPW, Lindquist E, Verchot L. 2015. Land use patterns and related carbon losses following deforestation in South America. Environ Res Lett. 10(12):124004. doi:10.1088/1748-9326/10/12/124004.
- Tait-Burkard C, Doeschl-Wilson A, McGrew MJ, Archibald AL, Sang HM, Houston RD, Whitelaw CB, Watson M. 2018. Livestock 2.0 – genome editing for fitter, healthier, and more productive farmed animals. Genome Biol. 19(1):204. doi:10.1186/s13059-018-1583-1.
- Thompson RL, Lassaletta L, Patra PK, Wilson C, Wells KC, Gressent A, Koffi EN, Chipperfield MP, Winiwarter W, Davidson EA. 2019. Acceleration of global N2O emissions seen from two decades of atmospheric inversion. Nat Clim Chang. 9(12):993–998. doi:10.1038/s41558-019-0613-7.
- UN General Assembly 21 October . 2015 Transforming our world: the 2030 agenda for sustainable development.https://www.refworld.org/docid/57b6e3e44.html
- van Ruijven BJ, van Vuuren DP, Boskaljon W, Neelis ML, Saygin D, Patel MK. 2016. Long-termmodel-based projections of energy use and CO2 emissions from the global steel and cement industries. Resources. Resour Conserv Recyling. 112:15–36. doi:10.1016/j.resconrec.2016.04.016.
- van Sluisveld MAE, Martínez SH, Daioglou V, van Vuuren DP. 2016. Exploring the implications of lifestyle change in 2 °C mitigation scenarios using the IMAGE integrated assessment model. Technol Forecast Soc Change. 102:309–319. doi:10.1016/j.techfore.2015.08.013.
- van Soest HL, Aleluia Reis L, Baptista LB, Bertram C, Després J, Drouet L, den Elzen M, Fragkos P, Fricko O, Fujimori S, et al. 2021. Global roll-out of comprehensive policy measures may aid in bridging emissions gap. Nat Commun. 12(1):6419. doi:10.1038/s41467-021-26595-z
- van Vuuren DP, van Ruijven, B, Hoogwijk, MM, Isaac, M, De Vries, HJM, et al. 2006. TIMER 2: model description and application. In: Bouwman AF, Kram T, Goldewijk KK, editors. Integrated modelling of global environmental change: an overview of IMAGE 2.4. Bilthoven: Netherlands Environmental Assessment Agency (MNP); p. 39–60.
- Von Stechow C, Minx, JC, Riahi, K, Jewell, J, McCollum, DL, Callaghan, MW, Bertram, C, Luderer, G, Baiocchi, G, et al. 2016. 2°C and SDGs: united they stand, divided they fall? Environ Res Lett:11(3.
- Wilberforce T, Baroutaji A, Soudan B, Al-Alami AH, Olabi AG. 2019. Outlook of carbon capture technology and challenges. Sci Total Environ. 657:56–72. doi:10.1016/j.scitotenv.2018.11.424.
- Wyborn C, Datta A, Montana J, Ryan M, Leith P, Chaffin B, Miller C, van Kerkhoff L. 2019. Co-producing sustainability: reordering the governance of science, policy, and practice. Annu Rev Environ Resour. 44(1):319–346. doi:10.1146/annurev-environ-101718-033103.
- Yang Y, Ren J, Solgaard HS, Xu D, Nguyen TT. 2018. Using multi‐criteria analysis to prioritize renewable energy home heating technologies. Sustain Energy Technol Assess. 29:36–43. doi:10.1016/j.seta.2018.06.005.
- Zhang X, Davidson EA, Mauzerall DL, Searchinger TD, Dumas P, Shen Y. 2015b. Managing nitrogen for sustainable development. Nature. 528(7580):51–59. doi:10.1038/nature15743.
- Zhang S, Worrell E, Crijns-Graus W. 2015a. Mapping and modeling multiple benefits of energy efficiency and emission mitigation in China’s cement industry at the provincial level. Appl Energy. 155:35–58. doi:10.1016/j.apenergy.2015.05.104.