ABSTRACT
Pyrrolizidine alkaloids (PAs) are secondary metabolites of plant families such as Asteraceae or Boraginaceae and are suspected to be genotoxic carcinogens. Recent investigations revealed their frequent occurrence in honey and particularly in tea. To obtain a comprehensive overview of the PA content in animal- and plant-derived food from the European market, and to provide a basis for future risk analysis, a total of 1105 samples were collected in 2014 and 2015. These comprised milk and milk products, eggs, meat and meat products, (herbal) teas, and (herbal) food supplements collected in supermarkets, retail shops, and via the internet. PAs were detected in a large proportion of plant-derived foods: 91% of the (herbal) teas and 60% of the food supplements contained at least one individual PA. All types of (herbal) teas investigated were found to contain PAs, with a mean concentration of 460 µg kg−1 dry tea (corresponding to 6.13 µg L−1 in [herbal] tea infusion). The highest mean concentrations were found in rooibos tea (599 µg kg−1 dry tea, 7.99 µg L−1 tea infusion) and the lowest in camomile tea (274 µg kg−1 dry tea, 3.65 µg L−1 tea infusion). Occurrence of PAs in food supplements was found to be highly variable, but in comparable ranges as for (herbal) tea. The highest concentrations were present in supplements containing plant material from known PA-producing plants. In contrast, only 2% of the animal-derived products, in particular 6% of milk samples and 1% of egg samples, contained PAs. Determined levels in milk were relatively low, ranged between 0.05 and 0.17 µg L−1 and only trace amounts of 0.10–0.12 µg kg−1 were found in eggs. No PAs were detected in the other animal-derived products.
Introduction
Pyrrolizidine alkaloids (PAs) are secondary plant metabolites that are mostly found in the botanical families of Asteraceae, Boraginaceae, and Fabaceae. Genera of each of these plant families such as Senecio, Eupatorium, Heliotropium, and Crotalaria have been reported as toxic to livestock and humans (Mohabbat et al. Citation1976; Tandon et al. Citation1976; Harper et al. Citation1985; Hill et al. Citation1997; Prakash et al. Citation1999; Shimshoni et al. Citation2015b; Kakar et al. Citation2010).
PAs consist of two fused five-membered rings with a nitrogen atom at the bridgehead (). This chemical structure moiety represents a classical tertiary amine and is called necine base. Depending on the mode of esterification of the two available hydroxyl groups of the necine base with so-called necic acids, a variety of ester derivatives are formed. Consequently, PAs occur as mono- or diesters, and the diesters can be open chained or macrocyclic PAs (). In plants, PAs will form N-oxide derivatives, but the ratio of the free base and the respective N-oxide form can vary considerably. No N-oxides are formed by the otonecines, as they consist of an azacyclooctene ring system that is methylated at the N-atom ()). According to their taxonomic occurrence, PAs are often grouped into subclasses of senecionine, lycopsamine, heliotrine and monocrotaline types. The senecionine-type PAs are generic for species of the Asteraceae tribe Senecioneae but are also found in Crotalaria species. The lycopsamine type occurs predominantly in the Boraginaceae, but also in the tribe Eupatorieae (Asteraceae). The heliotrine type is specifically produced by Heliotropium species (Boraginaceae) and the monocrotaline type by Crotalaria species (Fabaceae).
Figure 1. PAs consist of a necine base that can have an: (a) retronecine, (b) retronecine N-oxide, (c) heliotridine, (d) heliotrine N-oxide, or (e) otonecine structure. Each necine base is coupled with a necic acid to form four major groups of PAs: (f) a 12-ring macrocyclic diester (e.g. senecionine), (g) an open-chained monoester (e.g. lycopsamine), (h) an open-chained diester (e.g. lasiocarpine), or (i) an 11-ring membered macrocyclic diester (e.g. monocrotaline). Senkirkine, the most prominent representative of the otonecine type, is not shown in this figure, but is generally grouped with the 12-ring macrocyclic diester PAs.
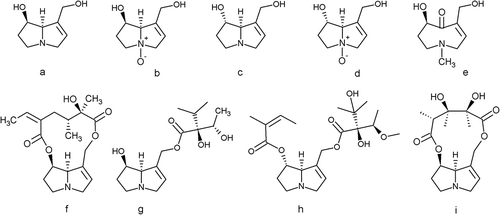
Acute poisoning with PAs in humans is associated with liver damage, whereas a subacute or chronic onset may lead to liver cirrhosis and pulmonary arterial hypertension (EFSA Citation2011). Experiments with rodents for investigation of chronic toxicity of PAs caused liver cell carcinoma and haemangioendothelial sarcoma and the International Agency for Research on Cancer (IARC) concluded that there was, depending on the PA congener, limited or sufficient evidence for the carcinogenicity of several PAs in experimental animals (IARC Citation2002; NTP Citation2008). Consequently, high concentrations of PAs in food and feed are undesired.
For animal-derived food, no large-scale investigations have been published; but PAs have been detected during carryover studies in milk (Dickinson et al. Citation1976; Hoogenboom et al. Citation2011) and eggs (Edgar and Smith Citation2000; Diaz et al. Citation2014; Mulder et al. Citation2016b). Previous carryover studies with ragwort in cows revealed a dose-related appearance of PA in milk and the overall carryover of PAs was estimated to be ~0.1%, but for specific compounds up to 4% (Dickinson et al. Citation1976; Hoogenboom et al. Citation2011). As bees also forage on plants containing these alkaloids, consequently, these compounds have been frequently detected in honey (Beales et al. Citation2004; Boppre et al. Citation2005; Kempf et al. Citation2010; Dübecke et al. Citation2011; EFSA Citation2011, Griffin et al. Citation2015, Bodi et al. Citation2014).
Recent studies revealed also that tea can be highly contaminated: total PA concentrations up to 5647 μg kg−1 have been detected in samples from the German retail market (Bodi et al. Citation2014), as well as in other countries (Griffin et al. Citation2015, Mathon et al. Citation2014, Shimsoni et al. Citation2015b). Comparable amounts were also detected in German-licensed herbal medicinal teas (Schulz et al. Citation2015). Based on the determined amounts, a first risk assessment was conducted by the Federal Institute for Risk Assessment, identifying a risk for those consumers, particularly children, who frequently drink large quantities of tea or herbal infusions (BfR Citation2013). The outcome of risk assessment and the fact that tea of all types can contain substantial amounts of PAs are relevant issues of food safety and emphasise the need for representative occurrence data of PAs in food. The EFSA funded a project among three partner institutes to conduct a study with the objective to analyse food products from different geographic regions in Europe (Mulder et al. Citation2015). In total, 1105 samples were investigated with emphasis on animal-derived products, such as milk, eggs, and meat products and on plant-derived products, such as (herbal) teas and food supplements. Analyses were performed using validated LC-MS/MS methods, which enabled detection at the lowest achievable levels, whereas the method scope focused on the PAs for which analytical standards were available (28 different commercial standards).
Materials and methods
Solvents, standards and reagents
Formic acid (analytical grade), sulphuric acid (98%, analytical grade), ammonia solution (32%, analytical grade), and methanol (LC-MS grade) were obtained from Merck (Darmstadt, Germany). Ammonium formate (LC-MS grade) was from Fluka (Seelze, Germany). Analytical PA standards were sourced from different suppliers: retrorsine (Re) from Sigma-Aldrich (Seelze, Germany); lasiocarpine (Lc), lasiocarpine N-oxide (LcNO), and heliotrine N-oxide (HeNO) from Cfm Oskar Tropitzsch (Marktredwitz, Germany); heliotrine (He) and trichodesmine (Td) from Latoxan (Valence, France); monocrotaline (Mc), senecionine (Sn), and seneciphylline (Sp) from Roth (Karlsruhe, Germany); and echimidine (Em), echimidine N-oxide (EmNO), erucifoline (Er), erucifoline N-oxide (ErNO), europine (Eu), europine N-oxide (EuNO), intermedine (Im), intermedine N-oxide (ImNO), jacobine (Jb), jacobine N-oxide (JbNO), lycopsamine (Ly), lycopsamine N-oxide (LyNO), monocrotaline N-oxide (McNO), retrorsine N-oxide (ReNO), senecionine N-oxide (SvNO), seneciphylline N-oxide (SpNO), senecivernine (Sv), senecivernine N-oxide (SvNO), and senkirkine (Sk) from Phytolab (Vestenbergsgreuth, Germany).
Animal-derived products were additionally analysed for indicine N-oxide (IdNO) and otosenine (Ot), obtained from Phytolab (Vestenbergsgreuth, Germany); florosenine (Fs) and jacoline (Jl), obtained from PRISNA (Leiden, the Netherlands); integerrimine (Ir) and integerrimine N-oxide (IrNO), kindly provided by Dr Trigo from the University of Campinas (Unicamp), São Paulo, Brazil; riddelliine (Rd) and riddelliine N-oxide (RdNO), kindly provided by NCTR (Jefferson, AR, USA); and trichodesmine N-oxide (TdNO), which was obtained by N-oxidation of trichodesmine. Epi-jacobine (epi-Jb), a synthetic non-natural isomer of jacobine (Mercachem, Nijmegen, the Netherlands), was used as internal standard in the analysis of animal-derived products.
Sample collection
Samples were collected in supermarkets, shops, and other retail outlets between January 2014 and April 2015 in six different countries (Germany, the Netherlands, Spain, France, Italy, and Greece). A limited number of (herbal) food supplements (15%) were purchased online. The sampling was conducted taking as guidance the methods of sampling for official control laboratories described in Commission Regulation (EC) No 401/2006. For each product, three items with the same expiration date and lot number were collected. After arrival at the laboratory, an aggregate sample of ~1 kg from the animal-derived products was prepared by combining equal amounts of the three collected items from which three subsamples of approximately 50 mL or 50 g were subsequently taken, uniquely coded, and stored at −20°C until analysis.
For the (herbal) teas and food supplements, a slightly different procedure was followed. One package of tea (bags) generally had a weight of 30–60 g. Three packages of same date and lot number were combined and homogenised to form a final aggregate of at least 100 g. For food supplements, the sampling procedure was based on the supposition that these food supplements are marketed in retail packages containing usually 30–120 capsules per retail package. Depending on the lot size, a single or two packages were taken. In each case, all capsules were combined and homogenised to form a final aggregate sample. From the aggregate sample three subsamples of 10–30 g were taken, uniquely coded, and stored at room temperature until analysis.
Sample preparation
Animal-derived food products
A detailed description of the sample preparation can be found elsewhere (Mulder et al. Citation2015, Citation2016b). Briefly, aliquots of 3 mL milk, 3 mL whole egg, 3 g cheese, 3 g yoghurt, 3 g meat, or 3 g liver were extracted with 30 mL of 0.2% aqueous formic acid solution and 15 mL hexane. Before extraction, 30 μL of epi-jacobine IS solution (1000 μg L−1 in methanol) was added to the samples. The hexane layer and proteinaceous middle layer were discarded after centrifugation (15 min at 2600 g). Concentrated ammonia (25%) was added to adjust the pH of the remaining solution to 9–10. Five mL (liver), 10 mL (egg, meat), or 15 mL (milk, yoghurt, cheese) of the aqueous extract was used for further clean-up by SPE over a StrataX 200 mg, 6 cc cartridge (Phenomenex, Torrance, CA, USA). The cartridges were conditioned with 6 mL methanol, followed by 6 mL ammonia solution (0.1%). The cartridges were loaded with the sample extract, washed with 6 mL ammonia solution (0.1%), and dried under vacuum (using a vacuum manifold) for 5–10 min. PAs were eluted from the cartridges with 6 mL of methanol. The eluates were dried under a nitrogen stream in a warmed water bath (50°C; TurboVap, Zymark, Uppsala, Sweden) and reconstituted in 500 µL of methanol/water (10/90, v/v). The reconstituted sample extracts were filtered using 0.45 µm PTFE 500 µL filtervials (UniPrep, Whatman, Maidstone, UK).
Samples were quantified against a 7-point calibration curve of PA standards in the corresponding blank material: 0–5 μg kg−1 in milk and yoghurt, 0–10 μg kg−1 in egg and meat. Cheese and liver samples were quantified by means of standard addition (10 μg kg−1) to the sample.
(Herbal) teas and supplements
A detailed description of the sample preparation has already been published (Bodi et al. Citation2014; Mulder et al. Citation2015). Briefly, for homogenisation all samples were mixed with dry ice (at a mass ratio of 2:1) and ground to a particle size of 500 µm using an ultra-centrifugal mill (ZM 200; Retsch, Haan, Germany).
The extraction procedure for ready-to-drink products was based on the protocol described in ISO 3103 (ISO Citation1980). A 2 g amount of the homogenised tea was transferred to a tea infusion bag (t-sac, Hannover, Germany). The tea bag was positioned in a glass beaker of 250 mL, and 150 mL of boiling water was poured onto the tea bag. The infusion was steeped for 5 min and the tea bag was removed. After cooling down, the infusion was filtered through a fluted filter paper. An aliquot of 50 mL was used for further clean-up by SPE as described below.
The extraction procedure for dry supplements was performed as follows: a 2 g amount of sample was extracted with 20 mL of 0.05 M sulphuric acid solution by sonication (15 min). The supernatant was decanted after centrifugation. Extraction was repeated and the combined supernatants were brought to pH 6–7 with 2.5 M ammonia solution and passed through a fluted filter paper; 10 mL of the filtered extract was used for SPE clean-up.
The SPE clean-up of tea infusions and herbal extracts was carried out with reversed-phase C18 SPE cartridges (Discovery DSC-C18 500 mg/6 mL; Supelco, Bellefonte, PA, USA), which were conditioned with 5 mL methanol and 5 mL water. Then, the cartridges were loaded with 50 mL of the (herbal) tea infusion, or with 10 mL of the filtered herbal extract, washed with 5 mL of water and dried under vacuum (using a vacuum manifold) for 5–10 min. PAs were eluted from the cartridges in two steps with 5 mL each of methanol or 2.5% (1.4 M) ammonia in methanol in the case of black and green tea samples. The combined eluates were dried under a nitrogen stream in a warmed water bath (50°C; TurboVap) and reconstituted in 1 mL of methanol/water (5/95, v/v). The reconstituted samples were filtered through a 0.2 μm membrane filter before LC-MS/MS analysis.
Samples were quantified using an 8-point calibration curve of PA standards in corresponding extracts prepared from blank material: 0.03–4.00 μg L−1 in tea infusions and 8–1200 μg kg−1 in herbal supplements.
Oily plant-derived food supplements
For oily food supplements, an amount of 5.0 g was extracted with 15 mL of 0.05 M sulphuric acid in methanol by overhead shaking (15 min). The supernatant was decanted after centrifugation. Extraction was repeated and an aliquot of 25 mL of combined supernatants was used for further clean-up by SPE. The SPE clean-up was carried out using cation exchange SPE cartridges (Bond Elut Plexa PCX, 500 mg/6 mL), which were conditioned with 5 mL of methanol and 5 mL of 0.05 M sulphuric acid in methanol. Then, the cartridge was loaded with 25 mL of the sample extract, washed with 8 mL of methanol, and dried under vacuum (using a vacuum manifold) for 5–10 min. PAs were eluted from the cartridge in two steps with 5 mL each of methanol containing 2.5% ammonia. The combined eluates were dried under a nitrogen stream in a heated water bath (50°C; TurboVap) and the drying residue was reconstituted in 1 mL of methanol/water (5/95, v/v). The reconstituted samples were filtered through a 0.2 μm membrane filter before LC-MS/MS analysis.
For quantification, extracts of blank samples were prepared according to the sample preparation procedure. The blank extracts were used to prepare 7-point PA calibration curves, corresponding to 1.2–60 μg kg−1 in oil-based food supplements.
LOD, LOQ, recovery, and precision data
Animal-derived food
The lowest calibration point with acceptable precision was taken as LOQ. Individual LOD values were estimated on the basis of the signal-to-noise (S/N) ratio of the lowest point of the calibration curve in blank matrix. For the LOD, a S/N of 6 was taken for both transitions. LODs varied, in the ranges of 0.03–0.05 μg L−1 for milk and yoghurt samples; 0.05–0.15 μg kg−1 for whole egg, cheese, and chicken and pork meat samples; and 0.1–0.25 μg kg−1 for beef meat and liver samples. LOQ values typically were 2–5× higher than the LODs. Except for riddelliine N-oxide, recovery rates of the individual PAs were acceptable to good in milk (74–107%), egg (56–103%), and meat (63–91%). Recovery for riddelliine N-oxide was 30–45% in these matrices, possibly due to a lower stability of this compound under the alkaline conditions used during SPE clean-up. Individual LODs, LOQs, and recovery rates are given as Supplementary Tables S1 and S2, respectively.
Plant-derived food
LOD and LOQ values were established according to the standard method DIN 32,645 (DIN, Citation2008). For this purpose, three replicates of five calibration levels that cover the lower calibration range were prepared and analysed. Individual LODs, LOQs, and recovery rates are given as Supplementary Tables S3 to S6. For tea and dry food supplements, the obtained LODs ranged between 0.3 and 2.3 μg kg−1. The subgroup of bee products yielded lower LODs (0.2–0.6 μg kg−1), while for oily supplements higher LODs (0.9–3.8 μg kg−1) were obtained.
Recovery rates were determined by repeated analysis of fortified blank material at concentrations of 20, 150, or 200 μg kg−1. Mean recovery rates ranged from 72% to 122% with a RSD of 1–20% in fennel, mixed herbal, and rooibos teas. Slightly lower recovery rates ranging from 45% to 98% with a RSD of 1–14% were determined in camomile and black teas.
Performance criteria obtained during in-house validation were confirmed during a collaborative validation study conducted for the analysis of PAs in herbal tea (BfR Citation2015).
LC-MS/MS analysis (animal-derived food)
PAs analysis in the animal-derived food products was performed on a LC-MS/MS system consisting of a Waters Acquity UPLC coupled to a Xevo TQ-S tandem mass spectrometer (Waters, Milford, MA, USA). PAs were analysed in positive ESI mode (ESI+). Two MRM transitions were measured per analyte ().
Table 1. Mass spectrometric conditions (positive ESI) used for the analysis of PAs in animal-derived food products.
Chromatographic separation was achieved on a 150 × 2.1 mm, 1.7 µm particle size, UPLC BEH C18 analytical column (Waters). Eluent A was prepared from 100% water containing 6.5 mM ammonium hydroxide and eluent B from 100% acetonitrile containing 1.2 mM ammonium hydroxide. A gradient elution was performed as follows: 0–1 min 100% A/0% B, 12.0 min 50% A/50% B, 12.2–15 min 100% A/0% B. A flow rate of 400 µL min−1 was applied and 5 µL were injected. The column temperature was maintained at 50°C.
LC-MS/MS analysis (plant-derived food)
Analysis of PAs in ESI+ mode was performed on a LC-MS/MS system consisting of an UHPLC (Ultimate 3000; Thermo Scientific, San Jose, CA, USA) coupled to a Triple Stage Quadrupole mass spectrometer (TSQ Vantage; Thermo Scientific). Two MRM transitions were measured per analyte ().
Table 2. Mass spectrometric conditions (positive ESI) used for the analysis of PAs in tea infusion and supplements.
Chromatographic separation was achieved on a 150 × 2.1 mm, 1.9 µm particle size, C18 Hypersil Gold column fitted with a guard column (Thermo Scientific, Germany). Eluent A was prepared from 100% water containing 0.1% formic acid and 5 mM ammonium formate and eluent B from 95% methanol and 5% water containing 0.1% formic acid and 5 mM ammonium formate. A gradient elution was performed as follows: 0–0.5 min 95% A/5% B, 7.0 min 50% A/50% B, 7.5 min 20% A/80% B, 7.6–9.0 min 0% A/100% B, 9.1–15 min 95% A/5% B. A flow rate of 300 µL min−1 was applied and 10 µL were injected.
Results and discussion
Occurrence data for pas in animal-derived food
In total 746 animal-derived products were investigated for the presence of 35 individual PAs. The splitting of samples into milk and milk products, eggs, and meat as well as the ratio of conventional and organic samples is shown in . PAs were detected above the LOD in a small number of milk and egg samples, but no positive findings were recorded for yoghurt, cheese, infant formula, meat, or liver samples.
Table 3. Overview of samples analysed and results obtained for PA in animal- and plant-derived food products.
In 10 out of 169 cow milk samples and in one out of 13 goat milk samples, the presence of one or two PAs could be confirmed. The determined concentrations in the milk samples were near the LOQ of the method and ranged between 0.05 and 0.16 µg L−1. A slight tendency was observed that PAs were more often found in samples from organic production (5/44; 11%) than from regular production (6/132; 4.3%), but the number of samples is too small to draw conclusions on this point. Concerning the individual detected PAs, six different compounds were found, representing the macrocyclic PAs (jacoline, retrorsine) and otonecine-type (senkirkine, otosenine) representative of Senecio as well as a monoester (lycopsamine/intermedine) and an open-chained diester PA (echimidine), suggesting that plant material of Boraginaceae spp. was the cause of contamination. But contrary to the putative causative plants, where a ratio between free base and N-oxide form is always observed, only PAs in the free-base form were found and no N-oxides were detected. This is in accordance with the PA transfer study conducted by Hoogenboom et al. (Citation2011), who attributed this to the role of the rumen in digesting the plant material, whereby the N-oxides are degraded or converted to free bases. Interestingly, in this transfer study it was reported that for three of the PAs detected in this survey (jacoline, senkirkine, and otosenine) the carryover rate was relatively high (Hoogenboom et al. Citation2011). The relatively high incidence of PAs present in milk (around 6%) may appear surprising, but a similar rate of 5–10% positive samples has been found in two follow-up surveys on milk, sampled in Germany and in the Netherlands (unpublished data). Milk is a commodity that is processed in large-scale facilities; while this ensures effective dilution if any contaminated milk from a dairy farm is included, it also results in an increased rate of (low-level) contaminated milk being offered in retail outlets.
Contamination of eggs with PAs was found in only two out of 205 analysed samples (1%) and determined levels were very low (0.10–0.12 µg kg−1). The individual PAs found were similar to those found in milk, and again only the free-base form was detected. Transfer of PAs to eggs has recently been reported (Diaz et al. Citation2014; Mulder et al. Citation2016b). Usaramine, an isomer of retrorsine, and the major PA contaminant in the feed administered by Diaz et al. (Citation2014), was found in dried eggs in levels up to 900 µg kg−1. In the study of Mulder et al. (Citation2016b), transfer rates of a large number of PAs were calculated based on the levels detected in the contaminated feeds and in whole eggs. The highest transfer rate (1.2%) was calculated for jacoline, followed by sceleratine (0.98%). Moderate transfer rates, in the order of 0.2–0.5%, were found for retrorsine, usaramine, otosenine, rinderine, and heliosupine. The finding in this survey, that contamination of eggs with PAs is relatively rare, may indicate that chicken feed is not often contaminated with PA-containing plant material; but it should also be recognised that a mechanism of large-scale ‘dilution’ as for milk is not likely for eggs. Contaminated eggs produced by a given farm will end up in a limited number of shops. While this reduces the chance of encountering positive eggs, it could mean that contaminated eggs contain higher levels of PAs.
None of the analysed bovine, porcine, or poultry meat and liver samples contained measurable amounts of PAs. Mulder et al. (Citation2016b), in their transfer study of laying hens, also analysed muscle and liver tissue of the exposed hens, revealing the presence of PA residues in these tissues. Depending on the plant material administered, PA concentrations in muscle tissue were 2–5× lower and in liver tissue 1.5–3× higher than in egg, while the PA profiles were similar in the matrices. The same situation may apply as discussed above for eggs: little ‘dilution’ of positives, but low likeliness of encountering one.
Occurrence data for pas in plant-derived food
(Herbal) tea
In total 168 (herbal) tea infusions were investigated for the presence of 28 individual PAs. Tea infusions were prepared according to an established protocol (ISO Citation1980). In order to compare results in tea infusions to PA amounts reported by other studies in dry tea in µg kg−1, all results have been multiplied by a factor of 75 to obtain the concentration in the dry product. To verify the validity of this approach, a selection of 38 tea samples collected in the survey, comprising the different types of tea and covering a wide concentration range (30–4400 µg kg−1), were analysed with both methods. For the individual PAs a reasonable correlation between the methods was obtained (). Overall, the efficiency of extraction of PAs by steeping 5 min in boiling water was ~85% compared with extraction by agitation with aqueous sulphuric acid for15 min at room temperature. The extraction efficiency for individual samples, however, varied from 40 to 250%. Nevertheless, extraction of PAs from dry tea by boiling water appears to be more efficient than that of a set of tropane alkaloids from tea using the same extraction conditions (Mulder et al. Citation2016a). In that study, a similar variation in results was observed, but the overall extraction efficiency using boiling water was only ~50%.
Figure 2. Mean concentration of PAs extracted from dry tea when preparing tea infusion with near-boiling water (light grey) and with aqueous sulphuric acid at room temperature (dark grey). A set of 38 samples differing in PA composition and concentration was analysed. Between brackets, the number of samples containing the PA.
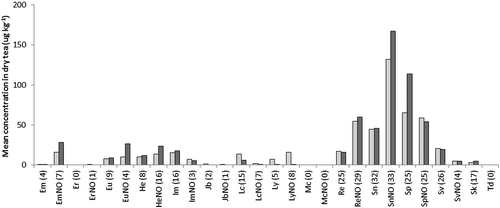
Results for the tea samples () revealed the presence of PAs in all types of tea and most samples (91%) contained one or more PAs, indicating that contamination of teas with PA-producing plants is very common. Detected amounts differed among the various types of tea and the highest contamination – with regard to maximum, mean and median concentration – was observed in rooibos tea, while green tea showed the lowest median concentration and camomile tea the lowest maximum and mean concentration (). In this study, the PA concentrations in the tea infusion, calculated as the sum of 28 PAs, revealed slightly higher results but in comparable ranges compared with previous studies where only 17 PAs were investigated (Bodi et al. Citation2014).
Overall, the highest concentrations were detected for N-oxides of macrocyclic diesters senecionine and retrorsine and their respective free bases, which belong to the senecionine type. Further, the monoester N-oxides of lycopsamine- and heliotrine-type PAs were also present in high concentrations, while the monocrotaline type was nearly absent. There was a strong co-occurrence of PA N-oxides and their respective free bases, while in most cases the N-oxide form was present in a higher concentration. In total, N-oxide PAs accounted for 64% of the PA content and PA free bases for 36%. Those ratios are in line with the general observation that PA N-oxides predominate over PA free bases in PA-producing plants (Molyneux et al. Citation1979; Johnson et al. Citation1985; Boppre et al. Citation2005; Kempf et al. Citation2008; Dübecke et al. Citation2011; These et al. Citation2013). Comparing the results of this study (sum of 28 PAs) with those obtained for 17 PAs (Bodi et al. Citation2014) for the newly included reference standards, it can be deduced that N-oxides of europine, echimidine, lycopsamine, and intermedine are the most relevant for assessment of the PA content in tea ().
Comparing the detected PA pattern of various types of tea, for rooibos tea a quite distinct pattern was detected ()): the mean concentrations of individual PAs were much higher than in any other type of tea, and the PA profile contained almost exclusively senecionine-type PAs. Almost all samples contained retrorsine, senecionine, senecivernine, and their N-oxides, as well as low levels of senkirkine. The contribution is dominated by senecionine N-oxide, as this single PA contributes almost 50% to the mean PA content. Together, the senecionine-type PAs were responsible for 98% of the PA content in rooibos tea. The type of PAs found in rooibos tea strongly point to contamination with Senecio spp. Rooibos tea is typically produced in South Africa, which is known for the presence of many toxic Senecio species (Stewart and Steenkamp Citation2001). Recently, Senecio angustifolia was identified as a likely source of contamination of rooibos tea (Van Wyk et al. Citation2017).
Figure 3. Mean concentrations of individual PAs in: (a) black tea (n = 33), (b) green tea (n = 26), (c) rooibos tea (n = 22), (d) camomile tea (n = 35), (e) peppermint tea (n = 35), and (f) mixed herbs (n = 22), The PAs are grouped into four categories: senecionine type (light grey), lycopsamine type (dark grey), heliotrine type (white), and monocrotaline type (black). Between brackets, the number of samples containing the PA.
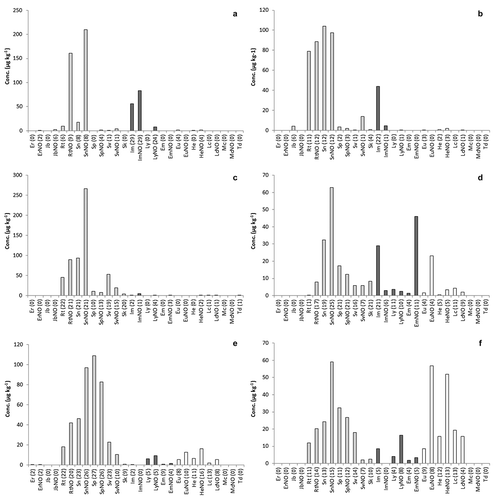
In green and black tea, the PAs that occurred most frequently belong to the senecionine and lycopsamine types, while the heliotrine type was almost absent ((a,b)). The PA profile was dominated by retrorsine, senecionine and intermedine and their respective N-oxides, together contributing >95% of the total PA content. The difference between black and green was that black tea contained much higher contents of the respective N-oxide form. Senecionine and retrorsine are produced in high concentrations in the Senecio species, while intermedine is a typical representative for species belonging to the Boraginaceae family including the genera of Anchusa, Borago, Symphytum, and Echium, but also in Eupatorium species (family of Asteraceae) (Hartmann and Witte Citation1995; El-Shazly and Wink Citation2014).
Peppermint tea was predominantly contaminated with senecionine-type PAs with minor contributions of heliotrine-type PAs ()). In contrast to rooibos tea, where seneciphylline and its N-oxide were minor compounds, these PAs – together with senecionine N-oxide – were the major compounds present in peppermint tea. The PA pattern in peppermint tea bears a strong resemblance to that of Senecio vulgaris, a common weed of arable areas in the moderate climate zone (de Nijs et al. Citation2014).
The PA pattern in camomile tea is complex, showing contributions of senecionine-, lycopsamine-, and heliotrine-type PAs ()) and suggesting production areas from different geographical regions.
In ) the distribution of PAs in mixed herbal teas is shown. In this sample group, PAs of senecionine and heliotrine types were found at the highest concentration, while PAs of the lycopsamine type occurred in less abundance. Senecionine- and heliotrine-type PAs accounted for >90% of the PA content in mixed herbal teas; senecionine, europine, and heliotrine N-oxides are the three main PAs found in this type of tea, together accounting for almost 40% of the PA content. This PA pattern indicates that species of Senecio and Heliotropium are the most relevant contaminating species during production of mixed herbal teas. However, the mean concentrations of individual PAs were relatively low compared with other types of teas.
The results were evaluated to assess whether there might be a correlation between the PA content and the form of production, as ~20% of the purchased teas were derived from organic production and products were available either as loose tea or as tea bags. Results of mean PA contents for organic and non-organic teas indicate that teas from organic production tended to contain lower PA concentrations (). Further, sorting the results according to the type of packing revealed a tendency towards lower PA contents in loose teas compared with teas in bags, especially for non-organic tea. When a comparison between traditional and organic production per tea type is made, it is evident that, for most tea types, organically produced tea contained lower amounts of PAs than traditionally produced tea ()). The only exception was camomile tea, where organic tea contained twice the PA amount compared with regular tea. Similarly, for most tea types mean PA levels were significantly lower in loose tea than in tea bags, except for peppermint and mixed herbal tea ()). The results indicate that, notwithstanding the diversity of contamination found, the way of production (organic versus traditional, loose versus tea bags) may be important with respect to the level of PA contamination in the final product.
Food supplements
A total of 191 food supplements were investigated that had been offered at the market in the form of tablets (containing dry ingredients), dragées (containing dry or oily ingredients), granulates (e.g. bee pollen), extract solutions, or as herbal teas. The latter were intended to be prepared as infusions and were, therefore, analysed according to the tea protocol. A special focus was given to supplements containing PA-producing plants, from which dried parts or extracts are used as (homeopathic) preparations in traditional medicine. In this study supplements containing Borago, Echium, Eupatorium, Lithospermum, Symphytum, Petasites, and Tussilago were collected and investigated. Supplements containing Senecio could not be found in retail shops or on the internet. An overview is given in .
Including the bee products, a total of 140 herbal food supplements were investigated that should not, according to their labels, contain any ingredients of known PA-producing plants. Should PAs be detected, these findings should be considered as contamination. Many of the herbal supplements contained material of a single plant species, but others could be mixtures of up to 10 or more different plant species. In 63% of these 140 samples PAs were detected with a mean concentration of 326 µg kg−1 and a median value of 8.9 µg kg−1. The large difference between mean and median concentrations indicates that only a few samples contained comparatively high PA concentrations, while most of the samples exhibited a low or negligible PA content. Indeed, only 13 supplements (9%) contained >1000 µg kg−1 PAs, while for the (herbal) tea this was the case for 21 samples (13%). Generally, in the positive samples all types of PAs could be detected, but the PA profile was often dominated by echimidine, lycopsamine, intermedine, and their N-oxide forms ().
Supplements of bee products containing bee pollen (n = 12) as well as products containing propolis and royal jelly (n=17) were investigated for their PA content. The PA content of pollen products is shown in ). In 11 of the 12 pollen products, PAs were detected with a mean concentration of 576 µg kg−1, while for the propolis and royal jelly products, a comparatively low mean amount of 7.6 µg kg−1 was found. Typically, lycopsamine-type PAs in combination with smaller amounts of senecionine-type PAs were present in the products, with echimidine and its N-oxide as major contributors. This PA profile is consistent with the average PA composition reported for honey samples in earlier studies (Dübecke et al. Citation2011; EFSA Citation2011). It is, however, interesting to note that the bee products contained a higher proportion of PAs in their N-oxide form than honey products, especially compared with the retail honeys where PA N-oxides are almost absent.
Figure 5. Mean concentrations of individual PAs in: (a) bee pollen supplements (n = 12), (b) St John’s wort supplements (n = 15), (c) supplements made from a single plant (n = 60), (d) supplements made from a mixture of plants (n = 18), (e) herbal teas made from PA-containing plants (n = 12), and (f) supplements based on PA-plant material (n = 18). The PAs are grouped into four categories: senecionine type (light grey), lycopsamine type (dark grey), heliotrine type (white), and monocrotaline type (black). Between brackets, the number of samples containing the PA.
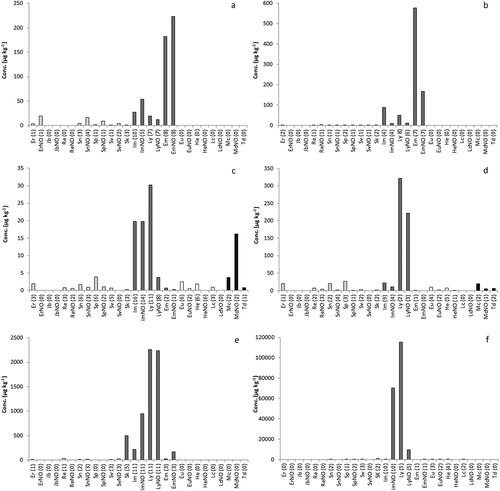
Within the herbal supplements an interesting subgroup is those containing St John’s wort (Hypericum perforatum) products, of which 15 samples were collected. In all but two samples, PAs were detected with a relatively high mean concentration of 924 µg kg−1 and median concentration of 722 µg kg−1. The detected PAs exclusively belonged to the lycopsamine-type PAs, with echimidine and its N-oxide present in the highest concentration ()), indicating a contamination with plant material likely from species of the Boraginaceae family. The results for St John’s wort supplements were in striking contrast to supplements containing valerian (Valeriana officinalis). Of the 18 samples included in this study, only five contained measurable amounts of PAs and the mean content for this group was only 32 µg kg−1 ().
The other supplements containing a single plant species (n = 60) or a mixture of species (n = 18) are shown in and ), respectively. The mixed herbal samples on average contained higher amounts of PAs than the single species supplements, 713 and 190 µg kg−1, respectively (). The same was found for the median and maximum values. The percentage of samples containing PAs was also higher for the mixed herbal samples (78%) than for the single species samples (60%). The lycopsamine-type PAs dominated in both groups of samples, with smaller contributions of senecionine- and monocrotaline-type PAs. Lycopsamine, together with its N-oxide, were the most important PAs, followed by intermedine and its N-oxide. The results indicate that composite herbal supplements are more likely to be contaminated with PA-containing plant material than products containing one major plant ingredient. Nevertheless, the general PA pattern is similar, an indication perhaps that the number of relevant Boraginaceae and/or Eupatorium species responsible for the contamination of this broad group of products is limited.
Supplements based on material from PA-producing plants yielded PA concentrations ranging from < LOD up to 2,410,275 µg kg−1 (see ). Interestingly, the levels in the 12 supplements to be prepared as herbal teas (6438 µg kg−1) were on average lower than the 18 supplements in the form of tablets or capsules (196,534 µg kg−1). On the other hand, the median concentration was higher for the herbal tea samples (1626 µg kg−1) than for the dry supplements (39 µg kg−1). All herbal tea samples from PA-producing plants contained PAs >100 µg kg−1, but for the dry supplements the picture was more diverse. Two herbal supplements containing Eupatorium spp. plant material contained PAs in a proportion of the dry mass of 0.24% and 0.11%, respectively. These values are well within the range of PA concentrations of producing plants reported in the literature (Molyneux et al. Citation1979; Stegelmeier Citation2011; These et al. Citation2013). However, 10 out of the 18 dry supplements contained PAs in concentrations between < LOD and 50 µg kg−1. Most of the investigated supplements with PA-producing plants belonged to the Boraginaceae family or were species from Eupatorium; consequently, samples mainly contained PAs of the lycopsamine type (see and )). This is reflected in the results: predominantly the monoesters lycopsamine, intermedine, and their respective N-oxides were detected. Plants belonging to the genus Petasites and Tussilago from the Asteraceae family produce senecionine-type PAs – in particular senkirkine, which is an otonecine-type PA with regard to the necine base. Substantial concentrations of senkirkine were detected in the five investigated Tussilago samples (mean PA content: 4674 µg kg−1), but in contrast the five Petasites samples contained only traces of PAs (mean: 7.2 µg kg−1).
No PAs were detected in oil-based products (). Most of the oil-based supplements were produced from seeds from PA-producing plants such as Borago (n = 19) and Echium (n = 2) species and furthermore one from St John’s wort, soya, and primrose. As all other supplements that contained PA-producing plants exhibited high PA concentrations, it can be concluded that due to their hydrophilic structure PAs are only co-extracted to a minor content in the lipophilic oil fraction of the plants or seeds or effectively removed during the subsequent oil refinement process (Cramer et al. Citation2014).
Conclusions
This study comprehensively investigated the occurrence of PAs in animal-derived food as well as (herbal) teas and (herbal) food supplements from six European countries. The results revealed that in animal products, PAs were only detectable in trace amounts and only in a few cases. Contamination of milk, eggs, and meat products with significant levels of PAs seems to be rare in the European Union. This is likely due to a combination of the situation that animal feed is rarely highly contaminated and the fact that metabolic processes in the animals lead to an efficient reduction of the ingested PAs.
In contrast, 60% of the (herbal) food supplements and 92% of (herbal) teas contained measurable amounts of PAs. The mean concentration was 460 µg kg−1 dry tea (corresponding to 6.13 µg L−1 in [herbal] tea infusion). Based on the available toxicological data, in 2011 a margin of exposure (MOE) of 1:10.000 was estimated by EFSA for a daily exposure of 7 ng PAs kg−1 body weight. Although the calculation was based on a worst-case scenario approach, it is evident from the collected data that the daily consumption of one cup of tea in 40% of cases could result in a MOE lower than 1:10.000. Considering the fact that almost all types of tea contained high PA amounts, this presents a relevant food safety issue. Within various types of tea, different PA profiles can be detected; but generally, PAs of the senecionine type dominated – in particular senecionine, seneciphylline, senecivernine, retrorsine, and the corresponding N-oxides, which together accounted for 76% of total PA content.
Investigation of food supplements focused on supplements that explicitly contained material of PA-producing plants, on supplements with no labelling concerning ingredients being PA producers, and on bee products. In 60% of all investigated samples, PAs were detected; but the concentrations were highly variable. As expected, the highest PA levels were found in herbal food supplements made from plant material of known PA producers in concentrations up to 2,410,275 µg kg−1 (0.24%). Supplements containing oil-based extracts of PA-producing plants, on the other hand, were free of PAs, indicating that the hydrophilic PAs will not be co-extracted in the lipophilic oil fraction, or are effectively removed during oil refinement. Investigated bee products showed highest PA concentrations for pollen products, while propolis and royal jelly products contained only traces of PAs.
Disclaimer
The sole responsibility of the information presented here lays with the authors and EFSA cannot be held responsible for the views displayed in this publication and/or in conjunction with the activities for which the grant is used or for any use that may be made of the information presented therein.
Acknowledgments
Albert Ribas Agusti and Lluis Lujan Quesada are acknowledged for sampling in Spain, France and Italy, Tsakalidis Analysis & Testing lab for sampling in Greece. Hester van der Top, Susannah de Witte, Wolfgang Herkt, Angelika Hiller, Ines Schirrman, Jessica Vetter, Albert Ribas Agusti and Lluis Lujan Quesada are acknowledged for sample preparation and analyses.
Disclosure statement
No potential conflict of interest was reported by the authors.
Additional information
Funding
References
- Beales KA, Betteridge K, Colegate SM, Edgar JA. 2004. Solid-phase extraction and LC-MS analysis of pyrrolizidine alkaloids in honeys. J Agric Food Chem. 52:6664–6672.
- BfR 2013. Scientific opinion [Internet]. [accessed 2014 June 26]. http://www.bfr.bund.de/cm/349/pyrrolizidinealkaloids-in-herbal-teas-and-teas.pdf
- BfR. 2015. International collaborative study for the Determination of pyrrolizidine alkaloids in honey and herbal tea by SPE-LC-MS/MS. [Internet]. [accessed 2015 May 08]. http://www.bfr.bund.de/cm/350/international-collaborative-study-for-the-determination-of-pyrrolizidine-alkaloids-in-honey-and-herbal-tea-by-spe-lc-ms-ms.pdf
- Bodi D, Ronczka S, Gottschalk C, Behr N, Skibba A, Wagner M, Lahrssen-Wiederholt M, Preiss-Weigert A, These A. 2014. Determination of pyrrolizidine alkaloids in tea, herbal drugs and honey. Food Addit Contam Part A. 31:1886–1895.
- Boppre M, Colegate SM, Edgar JA. 2005. Pyrrolizidine alkaloids of Echium vulgare honey found in pure pollen. J Agric Food Chem. 53:594–600.
- Cramer L, Fleck G, Horn G, Beuerle T. 2014. Process development of lappula squarrosa oil refinement: monitoring of pyrrolizidine alkaloids in boraginaceae seed oils. J Am Oil Chem Soc. 91:721–731.
- de Nijs WCM, Elbers IJW, Mulder PPJ. 2014. Inter-laboratory comparison study for pyrrolizidine alkaloids in animal feed using spiked and incurred material. Food Addit Contam Part A. 31:288–299.
- Diaz GJ, Almeida LX, Gardner DR. 2014. Effects of dietary Crotalaria pallida seeds on the health and performance of laying hens and evaluation of residues in eggs. Res Vet Sci. 97:297–303.
- Dickinson JO, Cooke MP, King RR, Mohamed PA. 1976. Milk transfer of pyrrolizidine alkaloids in cattle. J Amer Vet Med Association. 169:1192–1196.
- DIN. 2008. DIN 32645:2008-11: Chemical Analysis - Decision limit, detection limit and determination limit under repeatability conditions - Terms, methods, evaluation. Berlin: Deutsches Institut für Normung.
- Dübecke A, Beckh G, Lullmann C. 2011. Pyrrolizidine alkaloids in honey and bee pollen. Food Addit Contam Part A. 28:348–358.
- Edgar JA, Smith LW. 2000. Transfer of pyrrolizidine alkaloids into eggs: food safety implications. Acs Sym Ser. 745:118–128.
- EFSA. 2011. Panel on contaminants in the food chain (CONTAM); Scientific opinion on pyrrolizidine alkaloids in food and feed. EFSA J. 9(2406):134 pp.
- El-Shazly A, Wink M. 2014. Diversity of pyrrolizidine alkaloids in the Boraginaceae structures, distribution, and biological properties. Diversity. 6:188–282.
- Griffin CT, Gosetto F, Danaher M, Sabatini S, Furey A. 2014. Investigation of targeted pyrrolizidine alkaloids in traditional Chinese medicines and selected herbal teas sourced in Ireland using LC-ESI-MS/MS. Food Addit Contam Part A. 31:940–961.
- Griffin CT, O’Mahony J, Danaher M, Furey A. 2015. Liquid chromatography tandem mass spectrometry detection of targeted pyrrolizidine alkaloids in honeys purchased within Ireland. Food Anal Methods. 8:18–31.
- Harper PAW, Walker KH, Krahenbuhl RE, Christie BM. 1985. Pyrrolizidine alkaloid poisoning in calves due to contamination of straw by Heliotropium-Europeum. Aust Vet J. 62:382–383.
- Hartmann T, Witte L. 1995. Pyrrolizidine alkaloids: chemical, biological and chemoecological aspects. In: S.W. Pelletier editor. Alkaloids: chemical and biological perspectives, vol. 9. Oxford, UK: Pergamon Press; p. 155–233.
- Hill BD, Gaul KL, Noble JW. 1997. Poisoning of feedlot cattle by seeds of Heliotropium europaeum. Aust Vet J. 75:360–361.
- Hoogenboom LA, Mulder PP, Zeilmaker MJ, van den Top HJ, Remmelink GJ, Brandon EF, Klijnstra M, Meijer GA, Schothorst R, van Egmond HP. 2011. Carry-over of pyrrolizidine alkaloids from feed to milk in dairy cows. Food Addit Contam Part A. 28:359–372.
- IARC 2002. Some traditional herbal medicines, some mycotoxins, naphthalene and styrene. IARC Monographs on Evaluation of Carcinogenic Risks to Humans. 82. http://monographs.iarc.fr/ENG/Monographs/vol82/mono82.pdf
- ISO. 1980. ISO standard 3103: 1980. Tea – Preparation of liquor for use in sensory tests.
- Johnson AE, Molyneux RJ, Merrill GB. 1985. Chemistry of toxic range plants - variation in pyrrolizidine alkaloid content of Senecio, Amsinckia, and Crotalaria Species. J Agric Food Chem. 33:50–55.
- Kakar F, Akbarian Z, Leslie T, Mustafa ML, Watson J, van Egmond HP, Omar MF, Mofleh J. 2010. An outbreak of hepatic veno-occlusive disease in Western afghanistan associated with exposure to wheat flour contaminated with pyrrolizidine alkaloids. J Toxicol. 313280:1–7.
- Kempf M, Beuerle T, Buehringer M, Denner M, Trost D, von der Ohe K, Bhavanam VBR, Schreier P. 2008. Pyrrolizidine alkaloids in honey: risk analysis by gas chromatography-mass spectrometry. Mol Nutr Food Res. 52:1193–1200.
- Kempf M, Wittig M, Schönfeld K, Cramer L, Schreier P, Beuerle T. 2010. Pyrrolizidine alkaloids in food: downstream contamination in the food chain caused by honey and pollen. Food Addit Contam Part A. 28:7–13.
- Mathon C, Edder P, Bieri S, Christen P. 2014. Survey of pyrrolizidine alkaloids in teas and herbal teas on the Swiss market using HPLC-MS/MS. Anal Bioanal Chem. 406:7345–7354.
- Mohabbat O, Srivastava RN, Younos MS, Merzad AA, Sediq GG, Aram GN. 1976. Outbreak of hepatic veno-occlusive disease in Northwestern Afghanistan. Lancet. 2:269–271.
- Molyneux RJ, Johnson AE, Roitman JN, Benson ME. 1979. Chemistry of toxic range plants - determination of pyrrolizidine alkaloid content and composition in senecio species by nuclear magnetic-resonance spectroscopy. J Agric Food Chem. 27:494–499.
- Mulder PPJ, De Nijs M, Castellari M, Hortos M, MacDonald S, Crews C, Hajslova J, Stranska M. 2016a. Occurrence of tropane alkaloids in food. EFSA Supporting Publication. 2016:EN–1140, 200. http://onlinelibrary.wiley.com/doi/10.2903/sp.efsa.2016.EN-1140/epdf.
- Mulder PPJ, de Witte SL, Stoopen G, van der Meulen J, Wikselaar P, Gruys E, Groot M, Hoogenboom LAP. 2016b. Transfer of pyrrolizidine alkaloids from various herbs to eggs and meat in laying hens. Food Addit Contam Part A. 33:1826–1839.
- Mulder PPJ, López Sánchez P, Castellari M, Preiss-Weigert A, These A. 2015. External scientific report “Occurrence of pyrrolizidine alkaloids in food”, EFSA supporting publication 2015:EN-859. EFSA J. 12(8). http://onlinelibrary.wiley.com/doi/10.2903/sp.efsa.2015.EN-859/full
- NTP. Final Report on Carcinogens Background Document for Riddelliine. 2008. http://ntp.niehs.nih.gov/files/Riddelliine-FINAL_(11_Aug_2008)_508.pdf
- Prakash AS, Pereira TN, Reilly PEB, Seawright AA. 1999. Pyrrolizidine alkaloids in human diet. Mutat Res-Gen Tox En. 443:53–67.
- Schulz M, Meins J, Diemert S, Zagermann-Muncke P, Goebel R, Schrenk D, Schubert-Zsilavecz M, Abdel-Tawab M. 2015. Detection of pyrrolizidine alkaloids in German licensed herbal medicinal teas. Phytomedicine. 22:648–656.
- Shimshoni JA, Duebecke A, Mulder PPJ, Cuneah O, Barel S. 2015a. Pyrrolizidine and tropane alkaloids in teas and the herbal teas peppermint, rooibos and chamomile in the Israeli market. Food Addit Contam Part A. 32:2058–2067.
- Shimshoni JA, Mulder PPJ, Bouznach A, Edery N, Pasval I, Barel S, Abd-El Khaliq M, Perl S. 2015b. Heliotropium europaeum poisoning in cattle and analysis of its pyrrolizidine alkaloid profile. J Agric Food Chem. 63:1664–1672.
- Stegelmeier BL. 2011. Pyrrolizidine alkaloid-containing toxic plants (Senecio, Crotalaria, Cynoglossum, Amsinckia, Heliotropium, and Echium spp.). Vet Clin N Am-Food A. 27:419–423.
- Stewart MJ, Steenkamp V. 2001. Pyrrolizidine poisoning: a neglected area in human toxicology. Ther Drug Monit. 23:698–708.
- Tandon BN, Tandon RK, Tandon HD, Narndranathan M, Joshi YK. 1976. Epidemic of veno-occlusive disease of liver in Central India. Lancet. 2:271–272.
- These A, Bodi D, Ronczka S, Lahrssen-Wiederholt M, Preiss-Weigert A. 2013. Structural screening by multiple reaction monitoring as a new approach for tandem mass spectrometry - presented for the determination of pyrrolizidine alkaloids in plants. Anal Bioanal Chem. 22:1245–1256.
- Van Wyk BE, Stander MA, Long HS. 2017. Senecio angustifolius as the major source of pyrrolizidine alkaloid contamination of rooibos tea (Aspalathus linearis). S Afr J Bot. 110:124–131.