ABSTRACT
Availability and safety of food ranks among the basic requirements for human beings. The importance of the food producing sector, inclusive of feed manufacturing, demands a high level of regulation and control. This paper will present and discuss the relationships in the triangle of legislation, the background of hazards with a biological nature, and opportunities for monitoring methods, most notable for prion-based diseases as primary issue. The European Union legislation for prevention of prion-based diseases since 2000 is presented and discussed. The definitions and circumscriptions of groups of species will be analysed in the view biological classification and evolutionary relationships. The state of the art of monitoring methods is presented and discussed. Methods based on visual markers (microscopy), DNA-based methods (PCR), protein-based methods (ELISA, mass spectroscopy, proteomics), near infrared oriented methods and combinations thereof are being evaluated. It is argued that the use in legislation of non-homogeneous groups of species in a biological sense will hamper the optimal design of monitoring methods. Proper definitions are considered to act as bridges between legal demands and suitable analytical methods for effective monitoring. Definitions including specified groups of species instead of single species are more effective for monitoring in a range of cases. Besides the desire of precise circumscription of animal groups targeted by legislation, processed products need well defined definitions as well. Most notable examples are blood versus blood products, and hydrolysis of several types of material. The WISE principle for harmonising the design of legislation and of analytical methods is discussed. This principle includes the elements Witful (reasonable legal principles), Indicative (clear limits between prohibition and authorisation), Societal demands (public health, environment, economy), and Enforceable (presence of suited monitoring methods) in order to promote a balanced effort for reaching the desired level of safety in the food production chain.
Graphical Abstract
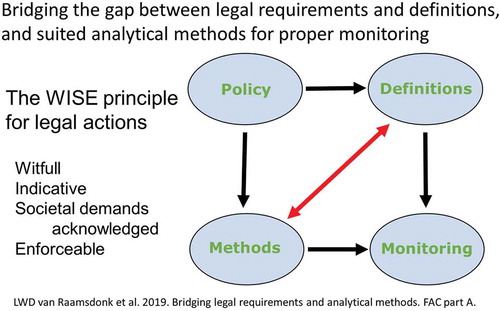
Introduction
The sector of food production, being the largest economic sector in the European Union (EU), ranks among the top three of most regulated sectors (van der Meulen Citation2014). Together with housing and clothing, it provides one of the basic resources of human life, including food availability, healthy nutrition and safety (Maslow Citation1943, Citation1945; Cianci and Gambrel Citation2003). In the EU, the major principles are laid down in the General Food Law (GFL), Regulation (EC) 178/2002 (European Commission Citation2002a). Any legal measure is typically intended to serve society. Social and health demands, environmental constraints and economic interests are taken into consideration, and a balanced approach of these factors is subjected to public debate and a political process for decision (see, e.g. Randall Citation2009). A distinction has to be made between food and feed in legislation which is intended to safeguard safety and quality. Differences among these two areas of food production are due to different health requirements, different sets of hazards, limitations due to hazardous transfer from feed to food, and to different legislation for novel food and feed ingredients (van der Spiegel et al. Citation2013). The need to raise and sustain the highest safety standards implies to develop and apply well approved methods for monitoring. A set of methods for enforcement of the legal requirements in feed is published in Regulation (EU) 152/2009 (European Commission Citation2009a), primarily focusing on a range of chemical compounds, but including methods for detection and identification of animal proteins (European Commission Citation2013a). This Regulation supports a part of the full range of legal restrictions and demands. Complementary methods are available from organisations such as CEN and ISO (CEN Citation2018; ISO Citation2018). Overviews of safety and quality issues have been published for food (Pico Citation2012) and feed (Fink-Gremmels Citation2012). In the view of this impressive range of topics, and considering the issue of the match between legal measures and the availability of facilitating analytical methods, this paper will focus on animal by-products in feed as most probable cause of Transmissible Spongiform Encephalopathy (TSE, prion diseases; Imran and Mahmood Citation2011) in livestock. In the framework of animal by-products in feed, two issues are primarily important. Safety is enforced by the prevention of TSEs effectuated by the Ruminant ban and additionally by rules of hygiene, and ethical concerns are translated to the Species-to-species ban (anti-cannibalism). Besides the animal health issues of TSEs, the related variant Creutzfeld-Jacob disease (vCJD) in humans has been and is an evenly important concern (Collee Citation2000; Imran and Mahmood Citation2011). The prevention of TSE, most notably but not restricted to Bovine Spongiform Encephalopathy (BSE) and scrapie in sheep, is targeting a biological agent in a biological organism. A fundamental factor is susceptibility of the organism to the agent, which has usually a genetic component. For example, sheep races with low susceptibility for scrapie have been bred successfully (Sartore et al. Citation2013; Stepanek and Horin Citation2017).
The biological and evolutionary background of the species and species groups involved is an undeniable framework for the assessment of risks and for the design of monitoring methods. It is therefore necessary to evaluate the relevant aspects of legislative demands, biological background and analytical opportunities. This paper presents an outline of European legislation related to the use of animal proteins in feed as part of the food production chain, the biological structure and classification of species and groups involved, and an overview of monitoring methods. The importance of legal definitions extracted from a biological framework will be discussed. Definitions as bridges will be used as basis to explore the relationships among the three areas of interest as presented here, and the future development of monitoring opportunities will be evaluated.
Legislative outline
The first case of BSE officially reported, in England, occurred in 1985 (Pain Citation1987). At that time, the term ‘prion’ was already coined for an agent that causes several degenerative diseases, which were recognised as being transmissible to animals (Prusiner Citation1984). In total a number as high as over 180,000 cases have been reported for the United Kingdom with a peak in 1992, although a retrospective analysis assumed an even higher number of 300,000 occurrences in France (Rosa Citation2006). In November 2000, the EU witnessed the occurrence of cattle suffering from BSE in Germany, Spain and Portugal. For Germany these incidences were not the first ones. During the last decade of the twentieth century other incidences had been encountered in Germany. However, at this time, authorities at the national and EU level responded very quickly, and EU-wide measures started to be discussed. Internet news stories provide an historical overview (Chronology of mad... Citation2000; Die Chronologie... Citation2000, Europe’s mad... Citation2000, Germany confirms... Citation2000). Within half a year a severe set of bans was agreed among the EU Council and the European Parliament (European Commission Citation2001). Also in late 2000 the principal decision was made to raise a European authority for food and feed safety, effectively laid down in Regulation (EC) 178/2002. In the current section of this paper the structure and further development of the EU legislation for the eradication of TSEs will be presented briefly (see Supplemental Material 1). In this section the term ‘eradication’ is used as reference to the terminology in legislation. An overview of the pre-2000 situation is published by Collee (Citation2000). All reference to EU Regulations and Directives are meant to refer to the most recent versions, including amendments. In some cases the EU provides consolidated versions of Regulations and Directives, which show integrated compilations of the original texts with all amendments up to the date of publication.
A pivotal role for the ban on processed animal proteins (PAPs) in feed is given to article 7 of Regulation (EC) 999/2001. Article 7 paragraph 1 prohibited the feeding of proteins originating from mammals to ruminants. This prohibition was amended in 2006 from a ban to feed PAPs from mammals to a ban to feed PAPs from animals in general (European Commission Citation2006). Paragraph 2 of the same article provides additional prohibitions, along with further provisions according to paragraph 3. Paragraph 2 makes reference to Annex IV of the same Regulation with specifications on feeding and the introduction of the ‘Extended feed ban’ (‘Extended’ refers to the phrasing in Article 7 part 2). Articles 8 and 9 give further prohibitions concerning Specified Risk Material and specifications on the use of ruminant material. An additional Regulation, (EC) 1774/2002 (European Commission Citation2002b), was put in force with additional measures on anti-cannibalism (Species-to-species ban), procedures for processing (rendering or incineration), and derogations for fur and pet animals and caught fish. Already at that time the intention was to gradually lift the ‘Extended feed ban’ (or ‘Total ban’; Marchis et al. Citation2017) and maintain only the principal prohibitions necessary for the eradication of TSEs, most notably BSE in cattle and scrapie in sheep: the permanent ‘Ruminant ban’, complemented with the ‘Species-to-species ban’ (see for a further reading e.g. van Raamsdonk et al. Citation2017b). On the other hand, extreme caution was needed to take correct decisions for every new derogation and exemption of the existing bans. In order to organise the relaxation in the most gradual way, Regulation (EC) 999/2001 has been amended 67 times since its first publication in 2001 (status as of July 2018) with the most recent relaxation of the release of seven species of insects allowed in feed for aquaculture (European Commission Citation2017a).
The principle of general bans with derogations and exemptions is unique in feed legislation. Where in most other legislation the principle ‘allowed, provided that limits are obeyed’ is applied, in PAP legislation the principle ‘prohibited, unless specifically exempted’ is used. The mentioned Annex IV on animal feeding, originally less than one page, consists of 19 pages in the consolidated version of Regulation (EC) 999/2001 as of 1 January 2018. Regulation (EC) 1774/2002 has been replaced with Regulation (EC) 1069/2009 (European Commission Citation2009e) with a connected implementing Regulation (EU) 142/2011 (European Commission Citation2011). The consolidated versions of this set of three Regulations now comprise a total of almost 500 pages. Besides measures for feeding of farmed animals, separation of different materials during storage and transport, cleaning, processing of animal proteins (rendering), hygiene (microbiological safety), further restrictions on the use of PAPs besides feeding, trade with and risk categories of third countries, and control regimes are regulated. The regulation on trade of PAPs with third countries implies that producers outside the EU have to comply to major parts of the EU legislation. The consequence of this situation is that the current evaluation of EU law and the analytical methods to support this is reaching further than to EU member states only.
The list of derogations or exemptions for specific materials currently includes milk, colostrum and products thereof, eggs and egg products, most hydrolysed proteins, feather meal, collagen, dicalcium phosphate and tricalcium phosphate (TCP) of animal origin, fish meal for non-ruminants and for unweaned ruminants, PAPs originating from non-ruminants (including seven species of insects) for feeding of aquaculture animals, blood and blood products for specific use, shells from shellfish, and starfish. This listing is not intended to be complete but to serve as an indication of the large range of materials involved. Some of the mentioned materials are simply excluded from the definition of PAP (European Commission Citation2011), others are only subjected to specific exemptions (see Supplemental Material 1). Provided the presence of a specified national risk assessment, bone spicules (small needle-like structures) of insignificant amounts might be present in compound feeds. This derogation was started after the finding of bone fragments in beet pulp in 2004, originating from soil remnants (Regulation (EC) 1292/2005, European Commission Citation2005a) This derogation was later extended to all vegetal ingredients (Regulation (EC) 163/2009, European Commission Citation2009b) amending Regulation (EC) 999/2001. Feed for pets (dogs and cats in shelters), fur animals, zoo animals, circus animals, maggots and worms for fishing bait (European Commission Citation2013b) are generally exempted from most aspects of the bans on PAPs. The Species-to-species ban remains valid for every farmed animal except fur animals, although a mink version of TSE (TME; Imran and Mahmood Citation2011) exists.
Wherever a derogation applies, these materials should be originating from Category III material according to the definition in Regulation (EC) 1069/2009, implemented by Commission Regulation (EU) 142/2011. This category is the by-product of the slaughter of healthy animals, of which the product is fit and intended for human consumption. PAPs from other animals, such as fallen stock and ill or medically-treated animals, certain organs capable of carrying prions (specified risk material, SRM) and foetuses are categorised as Category I or II, which are not allowed to enter the food production chain. In order to have the opportunity for monitoring, the Category I and II materials should be marked with glycerol-triheptanoate (GTH), consisting of fatty acids that do not occur in nature, but which are easily detectable (European Commission 2011).
This brief overview of legislation shows three major feed bans for eradication or prevention of TSEs, or for related issues: the Ruminant ban, the Extended feed ban, and the Species-to-species ban. The following sections will focus on the necessary biological background, and the current state of the analytical methods, the latter mostly restricted to the latest developments since the last overview (Veys et al. Citation2012b). The discussion will focus on the synthesis of the three domains.
Biological aspects of the definition of groups
For a practical and effective implementation of legal measures, a clear scope of a measure and of definitions of relevant terms are essential. In order to evaluate the match between legislative measures and the analytical methods necessary for monitoring, definitions in the view of relevant scopes will be discussed here. The term PAP, as a series of other terms, is defined in the appropriate legislation. The definition of terms related to analytical issues or to biological background is a bit more complicated. At first an important term is the use of the word ‘species’. There is a range of different definitions for the term ‘species’, depending on the background (Dickinson Citation1999). For animals a species concept based on reproductive isolation could suffice in most cases, but in the view of legal requirements other choices could be made. Notwithstanding the type of definition applied, a species is principally indicated by a binomial consisting of two Latin words. A trinomen, consisting of three Latin words, could be used to indicate a subgroup within a species. Groups of species are organised in a genus (plural: genera), which in turn are grouped together in tribae or subfamilies, families, orders and classes. Intermediate hierarchical levels exist. For example, the species cattle (binomen Bos taurus) belongs to the genus Bos, the subfamiliy Bovinae, the family Bovidae, the suborder Ruminantia, the order Artiodactyla and the class Mammalia. Most of the indications that are currently applied in the EU legislation such as bovine, caprine, solipeds, pigeon and poultry do not point to species in the biological sense but to groups at a higher (sometimes arbitrary) classification level. For instance, the subfamily Caprinae includes both sheep and goat, together with some other species. In the following paragraphs the issues in definitions will be explored. In order to get a wider view on the subject, other Regulations or Directives then the primary ones already presented will be mentioned as well.
Mammals
The Regulations, as presented in the previous section of this paper, frequently refer to ruminants as target animal for prohibitions. Directive 2003/85/EC (European Commission Citation2003b) refers to ‘any … animal of the suborders Ruminantia, Suina and Tylopoda of the order Artiodactyla’ (). Furthermore, Decision (EU) 2016/645 (European Commission Citation2016a) with a very specific scope (protective measures against lumpy skin disease in Bulgaria) makes a comparable reference. Other legal actions refer to more specific groups such as ‘bovine’, whether or not including buffalo or bison, porcine, ovine and caprine animals, or to species, in some particular situations indicated by their scientific names. provides an overview.
Table 1. A selection of mammal related references laid down in EU legislation.
The quote of suborders in an order (European Commission 2003) refers to the levels in the biological classification system. shows the classification of Artiodactyla (Ungulates; hoofed mammals) with only the relevant (sub)groups based on molecular data (Gatesy et al. Citation1997; Fernandez and Vrba Citation2005; Bai et al. Citation2014). This classification was known in general sense based on classical data, and is largely approved by new evidence. A major change resulting from molecular data is the grouping of the Cetacea among the other groups of the Artiodactyla, and the separate position of the yak, formerly part of the genus Bos. The bovine group comprises the genus Bos with B. taurus (cattle) as most important species, bison, buffalo and some exotic African genera, including nyala and kudu. Ovine (sheep) and caprine (goat) species both belong to the same taxonomic group. Other important groups belonging to the ruminants include antelopes and cervids (deer, elk, moose and relatives). The Suina and Tylopoda are sister groups to the group Ruminantia-Cetacea. Horses and relatives (‘solipeds’) are uneven-toed ungulates. Elephants belong to this group as well, but they have no relevance for the current discussion.
Figure 1. Phylogenetic tree of the order Artiodactyla (ruminants and related groups). Only species and groups relevant for legislation on the eradication of TSEs are shown. Text blocks indicate the types of TSE found in ruminants and related groups. For further explanation see text.
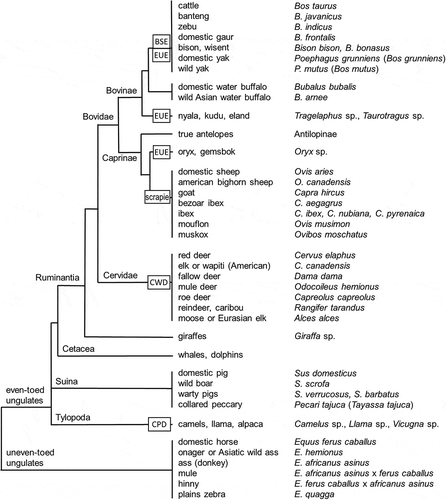
In order to present the range of species susceptible to TSEs, the occurrence of several types of TSEs is indicated as well in . The bovine version (BSE) and scrapie in sheep, and human forms of prion diseases such as vCJD and Kuru, are substantially documented. An overview is given by Collinge et al. (Citation2006). Further insight in background and mechanisms of Chronic Wasting Disease (CWD) in cervids is recently achieved. It was first encountered in Northern America in a still increasing area; (Imran and Mahmood Citation2011; Haley and Hoover Citation2015; Hannaoui et al. Citation2017). A total of five incidences of CWD was found in moose and reindeer in Norway during 2016 (VMK Citation2017). Latest counts (oral communication April 2018) include 20 cases in Norway (reindeer 18, moose 1, deer 1) and one case in moose in March 2018 in Finland. Other sources refer to three cases in moose in Norway, and two different strains of the causing prions (First case... Citation2018; Norwegian Veterinary Institute Citation2018). An extensive literature overview, current status and risk assessment has been carried out by EFSA, also pertaining to regions outside Europe (EFSA BIOHAZ Panel Citation2017). Transmission routes include faeces, urine, nasal secretions, saliva, milk, semen and antler velvet. Blood and meat are shown to be capable of containing prions, in contrast to BSE, which renders the application of the term SRM not practicable. Plants such as alfalfa, corn and tomatoes can take up the prions causing CWD (Pritzkow et al. Citation2015). Since the mentioned excreta occur freely in nature, licking and eating can be sources for infection. In addition, maternal transmission is encountered experimentally. With this variety of transmission routes, CWD can be considered highly contagious, unprecedented among TSEs (Hannaoui et al. Citation2017). Squirrel monkeys (Race et al. Citation2009) and most recently macaques (Czub et al. Citation2017) appeared to be susceptible to CWD prions. Several in vitro and in vivo human model systems showed responses resembling sporadic Creutzfeld-Jacob disease after infection with CWD prions (sCJD; Barria et al. Citation2011, Citation2014; Davenport et al. Citation2015). New emergent strains of CWD prions can contribute to the expansion of the host range (Herbst et al. Citation2017; Norwegian Veterinary Institute. Citation2018). Between 1986 and 1998 a version of TSE was found among zoo animals, indicated as Exotic Ungulate Encephalopathy (EUE). This affected animals belonging to greater kudu, nyala, common eland, several Oryx species, an Ankole-Watusi cow (an African cattle breed), and an American bison. Infection likely occurred via feed. The agent was finally identified as being identical to that of BSE (Collee Citation2000; Imran and Mahmood Citation2011). The most interesting insights of this temporary set of EUE infections are the apparent susceptibility of bison to the BSE type of prions, and the range of species susceptible to BSE outside the subfamily Bovinae (e.g. Oryx). There are no infections reported of buffalo by a TSE. There are considerable genetic differences for the relevant genes reported between cattle and buffalo, as is the situation among species of the genus Tragelaphus and cattle (Zhao et al. Citation2015). Nevertheless, since kudu and nyala are apparently susceptible for BSE, it cannot be ruled out that buffalo is susceptible as well. Both a precise description and circumscription of the group of Ruminants is most relevant for a proper basis to the Ruminant ban. Most recently a camel version of TSE (Camel Prion Disease, CPD) was discovered in dromedary camels in Algeria (Babelhadj et al. Citation2018). Camels and relatives are not belonging to the suborder Ruminantia ().
The applicability of the animal species or groups as mentioned in legislation () depends on the scope of the particular Regulation in the view of the biological classification as presented in . The reference to a suborder (Ruminantia) in the order Artiodactyla (European Commission 2016) is relevant for the protection against TSEs in general. However, the Regulations as presented in the previous section ‘Legislative outline’, as the TSE Roadmap 2 (European Commission Citation2010), do not contain any reference to the definition or circumscription of the suborder Ruminantia, but they frequently quote the term ‘ruminants’. Furthermore, the majority of the remaining legislation as listed in , restrict the groups as shown in to single species (sheep, goat, swine) or to a group of species of one genus (bovine), in a few cases accompanied by their Latin names. A detailed overview of groups is provided in the new version of Annex I to Regulation (EC) 396/2005 (European Commission Citation2005c; European Commission Citation2018). This Annex I is now divided in two parts, making a division between the main farmed animals in Part A, and a range of additional species or species groups in Part B (). The domestic yak in the version of 2018 of Annex I is indicated by the Latin name ‘Poëphagus mutus grunniens’ (with the incorrect use of the diaeresis on top of the ‘e’ in Poëphagus). The position of the yak in a separated genus Poephagus close to the genus Bison is based on DNA diversity (Wu et al. Citation2010; Bai et al. Citation2014), but hybrids between yaks and other species of the genus Bos do exist, indicating their still close relationship (Takeda et al. Citation2004). This complicated situation indicates to treat the Bovinae as one unseparable group (). The trinomen for bovine in Regulation (EU) 2018/62 (Bos primigenius taurus) is not valid nor correct. If the choice is to consider cattle (B. taurus) and auroch (B. primigenius) one species, the subspecific trinomen should be B. taurus taurus and B. taurus primigenius, respectively (Wilson and Reeder Citation2005).
The issue of definitions applies also to other groups which are minor sources to the food production chain. A group called solipeds was introduced in Regulation (EC) 853/2004 (European Commission Citation2004a). Other Regulations such as Regulation (EU) 212/2013 (European Commission Citation2013c) present a category consisting of ‘horses, asses, mules, hinnies; Equus spp.’. The four common names exclude other species of the genus Equus such as zebra and onager (see ). The common name ‘ass’ might be confusing, since two species are indicated by that common name. In cases where the adjective ‘Asiatic’ is dropped, the meaning remains unclear. Another example is the relationship between Lagomorpha and Rodentia, which are two different orders of mammal classification, although the rodents are considered part of the lagomorphs in Regulation (EC) 853/2004.
Birds
There is a number of definitions to describe the term ‘poultry’ in European legislation (), partly depending on the purpose and scope. General definitions (European Commission Citation2004b) and definitions focusing on safety (European Commission Citation2014) include five groups: chicken, geese, duck, turkey and guinea fowl. Geese and duck apply to one or few genera, hence comprise several species, although sometimes specific species are indicated by their Latin name (European Commission 2013). The Regulations in the area of safety (European Commission 2014) mention ostrich and pigeon as well, but the typographic structure (five animal species or groups between dashes; ) seems to include only these five in the strict description of poultry. Here, geese (plural) is supported by one species (Anser anser), the common name of Numida meleagris is helmeted guinea fowl, the common name of Coturnix coturnix (common quail) is not listed at all, and pigeon is restricted to members of the Columba genus, whereas other genera of pigeon and dove exist as well. With respect to trade (European Commission Citation2008; European Commission Citation2009c; European Commission Citation2013d) the definition is extended to include quails, pheasants and partridges (all belonging to the Galloanserae), and pigeons and ratites (ostrich, emu, nandu and related species), which are classified outside the Galloanserae. The legislation on the Common Customs Tariff (European Commission Citation2016b) and the preceding versions, see ) include the same species or groups as applied in legislation on safety. A much extended definition is applied in the area of animal welfare and hygiene that serves the protection against zoonoses which can principally attack any bird that is reared or kept in captivity for commercial purposes (European Commission Citation2005b). In the scope of food and feed safety and more specific the animal protein ban in feed (Regulation (EC) 999/2001), the more strict description of ‘poultry’ including the species of major economic importance is the most relevant one. This definition is also used in Regulation (EC) 882/2004, which serves as the general basis for feed and food control. In contrast to the species chicken and turkey, the groups geese and duck are mentioned in broad sense, that is several genera could be included in the indication ‘duck’ and ‘geese’ (see ). The definition of poultry, as proposed by the European Union Reference Laboratory for the detection of Animal Proteins in feeding stuffs (EURL-AP) is adopted from the legislation on the Common Customs Tariff (EURL-AP presentation at annual meeting in Vienna, 2011, Pers. comm.).
Table 2. Selection of poultry definitions laid down in EU legislation.
Figure 2. Phylogenetic tree of poultry and related groups. Only species and groups relevant for legislation on the eradication of TSEs are shown. Bold/underlined: narrow definition of poultry; dashed underlining: wide definition of poultry. For further explanation see text.
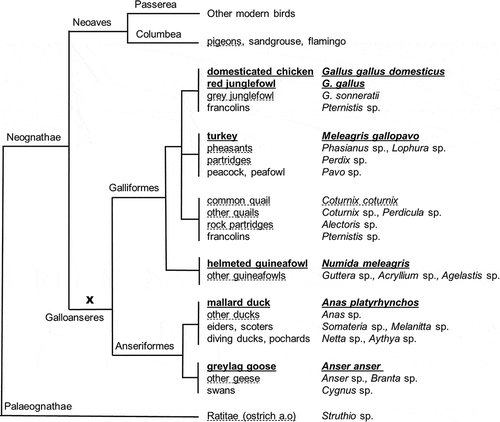
The term fowl can be used for birds in general but usually refers to birds belonging to Galliformes (gamefowl or landfowl) and Anseriformes (waterfowl). Together they form the fowl clade Galloanserae (; Eo et al. Citation2009; Islam et al. Citation2014; Jarvis et al. Citation2014). Ratites (Ratitae) are any of a diverse group of large, flightless birds of the superorder Palaeognathae, and comprise several families (Eo et al. Citation2009). In general, two groups, formally at the level of orders, can be distinguished with respect to poultry: Galliformes (among them chicken, turkey, guinea fowl, pheasant, quail, partridge) and Anseriformes (among them geese, swans and duck). Any subgroup included in the orders Galliformes and Anseriformes include species or genera that are neither included in the narrow nor in the wide definition of poultry, which is specifically indicated in . This situation can be clarified from the viewpoint of practical application (safety of avian meat for consumption, captive fowl vs. game fowl in trade, etc.), but it complicates the requirements for analytical methods.
Hydrolysed proteins
In the Feed Catalogue (European Commission Citation2013e), amended according to Regulation (EU) 2017/1017, European Commission Citation2017b), hydrolysis is described as ‘Reduction of molecular size by appropriate treatment with water and either heat/pressure, enzymes or acid/alkali’. The definitions used in the implementing Regulation (EU) 142/2011 and in the Feed Catalogue are identical. Regulation (EU) 142/2011 provides one further addition in Annex IV on the maximum molecular weight of proteins derived from ruminant material (). The definition of the process of hydrolysis in the Feed Catalogue () provides several versions of that process with different effects. In practice, feather meal is usually hydrolysed in order to improve the digestibility considerably. This result is achieved by heating at high pressure and temperature during prolonged time. The requirements for this hydrolysis exceeds considerably the minimal requirements of rendering of PAPs (European Commission 2011). Usually hydrolysed feather meal is still recognisable by means of visual examination (Veys et al. Citation2012a). Hydrolysation by means of acid or alkali treatment is usually applied to other products, such as mucosa protein. The appearance after processing and spray-drying is that of a fine granulate. Normally, the relationship with the original product and species origin cannot be observed.
Table 3. Indications of hydrolysed proteins of animal origin used EU legislation.
The definition and position of hydrolysed proteins in legislation leaves space for interpretation. Two major issues need attention: the degree of hydrolysation and the species of which the material originated are at stake. The portion of the material that is allowed to show characteristics of the original material is not specified. This implies that traces of the original material, for example muscle fibres, can be evaluated as the result of an incomplete hydrolysation, but other interpretations are still valid. The size of the resulting peptides is limited to a maximum of 10 kDalton for material originating from ruminants, which leaves all hydrolysed proteins of other sources undefined. Administrative control could reveal whether a particular batch of hydrolysed proteins is based on ruminant material or on another species. In the view that mislabelling can occur, analytical methods should be available for monitoring hydrolysed products. This aspect will be discussed in the following section.
Insects
Insect are the most prominent class of the phylum of arthropods, along with the crustacean (lobsters, crabs, krill) and arachnoids (spiders, mites), with an estimated number of nearly 1 million species (van Raamsdonk et al. Citation2017b, appendix 1). From 1 June 2017, seven specified species of insects are allowed as ingredient of feed for aquaculture (Regulation (EU) 893/2017 (European Commission Citation2017c) amending Regulations (EC) 999/2001 and (EU) 142/2011). These are black soldier fly (Hermetia illucens), common housefly (Musca domestica), yellow mealworm (Tenebrio molitor), lesser mealworm (Alphitobius diaperinus), house cricket (Acheta domesticus), banded cricket (Gryllodes sigillatus) and field cricket (Gryllus assimilis). Each of these genera, except Tenebrio with two species, all consist of a range of species (Insecta... Citation2018). With the publication of a list of allowed species the white list approach was chosen. The monitoring of all other, prohibited, species is then close to infinitely complicated (van Raamsdonk et al. Citation2017b).
Monitoring methods for PAPs
Enforcement can be achieved in a range of occasions by administrative control of documentation. Such procedures can be applied in trade, custom control, process control in rendering farms, etc. In order to establish the relation between the documents and the material which they cover, however, physical analysis is required. Difference has to be made between pure materials and mixtures. In the case of animal proteins, pure materials have to be identified for approval of the administrative indication. Mixtures, for example compound feeds, need a first step of detection of any prohibited substance, which can be carried out by microscopy in a range of occasions, followed by formal identification by means of DNA analysis, if anything was detected. The main parameters for analytical methods relevant for the current discussion are sensitivity (the quality of the detection) and specificity (quality of the opportunity for identification). Other parameters such as repeatability and reproducibility are always sufficiently approved before the publication of any method. Organisation such as ISO and CEN apply guidelines for the procedures for validation. In 2007 the EURL-AP started its activities primarily focusing on the development and improvement of analytical methods.
Processing procedures are an integral part of the nature of PAPs. There is a range of seven described procedures for rendering (European Commission 2011). The intention is to inactivate prions as much as possible, with the side effect of damaging other structures. This effect will hamper the detection and identification efficiency of monitoring methods. The denaturation of proteins is used in two studies as parameter for measuring the extent of the rendering by means of a pork ELISA (Von Holst et al. Citation2000, Citation2001). Both studies were targeting the two rendering methods mentioned in legislation prior to the legal authorisation of the set of seven methods in 2002. Six of these seven methods are defined by specific circumstances with parameters for particle size, temperature (and pressure), and duration. The seventh method is defined by a set of microbiological standards. Since then, experiments revealed that the principle rendering procedure of 20 min heating at 133°C (method 1) has limited effect on the activity of prions. As one of the alternatives, inactivation of prions is claimed to be quite effective when the material is treated by a purified solution of hypochlorous acid, with a putative activity reduction of 103–106 with limited side effects (Hughson et al. Citation2016; Giles et al. Citation2017). Proteins in general are severely damaged during rendering with respect to their tertiary structure. ELISA studies demonstrated that rendered bovine material can be detected at 0.5% by using two commercial kits (van Raamsdonk et al. Citation2015a), whereas rendered pork material cannot be detected unambiguously (four commercial kits; RIKILT, unpublished results of experiments carried out in 2017). DNA is damaged as well, but experiments showed that after rendering at 145°C still positive signals can be found (Woodgate et al. Citation2009). Strategies to circumvent poor quality of DNA include the applicability of multi-copy targets and target sequences preferably shorter than 100 base pairs (Fumière et al. Citation2009), or the targeting of two mitochondrial genes in a multiplex PCR (Ali et al. Citation2015; Motalib Hossain et al. Citation2017). Visible markers are relatively unaffected. Even a severely processed animal by-product such as TCP can be recognisable with visual markers (van Raamsdonk et al. Citation2013).
An overview of the pre-2007 methodological developments can be found in van Raamsdonk et al. (Citation2007). Reports on the state of the art of methodology have been subsequently published (Fumière et al. Citation2009; Jørgensen and Baeten Citation2012; Veys et al. Citation2012b). In this section the current state of the methodological tools for the detection and identification of animal materials will be discussed.
Visual structures and staining: microscopy
In 2001, at the start of the EU wide ‘Ruminant ban’ and ‘Extended feed ban’, the only analytical method at hand was the microscopic method (European Commission Citation1998). The main visual target is the presence of bone fragments. By extracting the fragments with high specific density from the sample, a concentration of the main target can be achieved with a factor 50 on average, depending on type of feed and level of PAP contamination, enhancing the sensitivity of the visual method. A set of interlaboratory studies provides documentation on this concentration (van Raamsdonk et al. Citation2012a, Citation2013, Citation2014, Citation2015c, Citation2016). The method was improved in 2003 (European Commission Citation2003a). With a quite satisfactory sensitivity of 0.02% (Engling et al. Citation2000) a total feed ban could be sufficiently enforced. Since then, a more detailed procedure for microscopic analysis was developed and published (European Commission 2013). Besides a major application as detection method, the principal difference to be made in the interest of identification is between terrestrial animals and fish. This discrimination acts at a classification level higher than those expressed in and : terrestrial animals comprise both mammals and birds (see figure 6.1 in van Raamsdonk et al. Citation2012b). A lot of documentation has been raised in that respect (Liu et al. Citation2011; van Raamsdonk, Prins, et al. Citation2017). The visual characteristics which could be the basis for discrimination at a lower classification level, that is between mammals and birds (poultry) are documented (van Raamsdonk et al. Citation2012b). Difficulties for visual identification pertain to the situation that certain groups of free floating sea invertebrates (e.g. krill) and starfish are included in the definition of fish (European Commission Citation2017d), amending Regulation (EC) 142/2011), and the relative resemblance of (fish) bone particles of mammals and of salmon (Veys et al. Citation2011; van Raamsdonk et al. Citation2012a).
A basic element of the microscopic method is separation of fractions of a sample based on specific density. The standard application is based on making a sediment of heavy particles from a ground sample in tetrachloroethylene (TCE, specific density D = 1.62 g/cm3), containing minerals and bone fragments, if present. The effect is a concentration of the target particles. The strategy to use (mixtures of) solvents with other densities was applied in several modifications of the procedure for sample preparation. The French method was based on two solvents: TCE and a mixture of TCE and tetrabromoethane (D = 2.4), resulting in two sediments (Michard and Ziebal Citation1999; Gizzi et al. Citation2004; Liu et al. Citation2011). This method was phased out for sensitivity reasons (see van Raamsdonk et al. Citation2007). An approach with an adjusted density by using a mixture of two solvents was used to separate muscle fibres from the remainder of the sample, intended for in situ identification. The mix of solvents was based on TCE and heptane (D = 1.33), established after testing a range of solvents (Bremer et al. Citation2012). A more recent modification of the procedure was initiated by the need to concentrate fragments of insects, by using a mixture of two solvents (TCE and petroleum ether, approximate D = 1.26; Veys et al., Citation2018). Based on these results it is to be expected that the insect strategy with D = 1.26 will results in a concentration of muscle fibres in the flotate as well, along with the chitinous parts of the insects.
Specific staining methods for visual detection are available, but these need thorough documentation. Insects share the existence of a chitin based exoskeleton with the other arthropods, most notably krill. A proof of concept was provided for specific microscopic staining procedures to detect chitin fragments, and to discriminate between the krill and insect version of chitin (Ottoboni et al. Citation2017). The use of Tetramethylbenzidine for the detection of blood products and the Alizarin staining for colouring of bone fragments, as part of Regulation (EC) 152/2009, appeared to be not particularly specific (van Raamsdonk et al. Citation2011, Citation2017a). Since these results are preliminary, further testing and appropriate validation is needed prior to reliable application.
DNA based methods: PCR
With the gradual diversification of the ban on animal proteins, a more specific method for identification was needed. DNA can be considered the basal level of information in plants and animals. Already at an early stage of the bans on PAPs, this level of information was chosen as basis for the identification of ruminants. The legislation demands to be able to distinguish, at least, ruminant from non-ruminant PAPs for protection against TSEs.
A ruminant PCR method (EURL-AP Citation2017), validated in an interlaboratory study by the network of National Reference Laboratories (NRLs; Fumière et al. Citation2016a; Olsvik et al. Citation2017) is available for identification of ruminant DNA in processed animal proteins. This method targets a multicopy nuclear repeat gene sequence and is specific for ruminants (European Commission 2009). Tests on specificity revealed positive signals for cattle (Bos taurus), sheep (Ovis aries), goat (Capra hircus), red deer (Cervus elaphus) and roe deer (Capreolus capreolus) and absence of signals for 42 other animals and plants including seven non-ruminant terrestrial mammals (EURL-AP Citation2017). The five species giving positive signals cover most of the subgroups of the ruminants (), but no information is given for the other species of the genus Bos, for elk, reindeer, bison and for buffalo. The multicopy background of the target DNA allows to have a high level of sensitivity to detect ruminants despite the effects of rendering. An alternative method was developed with a specificity covering nine ruminant species, including mouflon and some additional cervid species (Pegels et al. Citation2011). Other attempts focus primarily on bovine material, being a subset of ruminant diversity (Yancy et al. Citation2009; Myers et al. Citation2010). A specific conventional PCR method for detection of water buffalo was recently developed (Motalib Hossain et al. Citation2017). The DNA extraction method applied influences the sensitivity (Myers et al. Citation2010) and is fixed in the EU official method (EURL-AP Citation2017).
Besides the Ruminant ban, the Species-to-species ban needs methodological support too. Since June 1st, 2013 pig and poultry processed proteins are allowed in fish feed (European Commission Citation2013f). In the TSE Roadmap 2 paths are described for re-introduction of non-ruminant PAPs into animal feed (European Commission Citation2010). It is planned that sometime in the near future pig PAPs may be allowed in poultry feed and/or poultry PAPs may be allowed in pig feed. Prerequisites for reintroduction of pig and poultry processed animal proteins in feed for land animals (non-ruminant) are specific detection methods for pig and poultry PAPs. For pig, several sensitive and species specific methods are described in literature (López-Andreo et al. Citation2012; Pegels et al. Citation2012a). A mitochondrial DNA method for pig detection has been set-up and was interlaboratory-validated by the EURL-AP and NRL network. This method will become mandatory in the EU (Fumière, Marien, Maljean, et al. 2016). The EURL-AP has also developed a method for poultry material for which the specificity is restricted to chicken and turkey, being the most prominent species in poultry PAPs. This method has been interlaboratory validated by the EURL-AP and NRL network. In addition, methods have been published for each of the separate targets of chicken, turkey, a mallard duck hybrid and greylag goose (Pegels et al. Citation2012b). Other studies into the detection of avian material provide only specificity results for the discrimination between single representatives of the group Galloanserae and non-avian species (Yancy et al. Citation2009; Pegels et al. Citation2014). Ostrich showed a signal as well in the study of Pegels et al. (Citation2014). The lack of other avian species in these two studies might imply that avian material in general is targeted. This situation can cause a problem when monitoring fish meals, which usually contain DNA of sea birds such as gulls (EURL-AP, personal communication). A specific and sensitive method for the detection of DNA of the sea gull species Larus michahellis has been published (Kesmen et al. Citation2013). A different approach was followed for the development of a method that specifically detects the species listed in the definition for poultry as used in EU legislation for food and feed safety (: chicken, geese, duck, turkey, guinea fowl). This list presents several problems. At first, single species as well as genera are listed. Secondly, the five species(-groups) belong to two different orders, the Galliformes and the Anseriformes. Lastly, a whole range of birds, such as swans, francolins, pheasants and peacock, are more closely related to some of the listed birds then they are related to each other, but all these other species(-groups) are excluded (). Therefore, this alternative approach consists of one detection system for chicken and turkey (Galliformes; at the species level), and a second one for duck and geese (Anseriformes; at the genus level), based on an in silico analysis of DNA diversity in chicken, turkey, two ducks and three goose species, specifically excluding ostrich, common wood pigeon and two gull species (Scholtens et al. Citation2017). These two methods were constructed to be able to run complementary in one reaction as a single poultry detection method. Experiments with rendered material revealed positive signals for four duck species, two geese species, chicken and turkey, and late signals for helmeted guinea fowl and turkestan hill dove. A total of 32 other species, among them a number of birds, gave no signal. A secondary achievement is the possibility to detect chicken/turkey and duck/geese separately (Scholtens et al. Citation2017, Citation2019). Finally, in the interest of the Species-to-species ban, identification of other species such as dog and cat could be of interest (Ali et al. Citation2015).
Some results for DNA based detection or identification of insects have been published (see overviews in Ottoboni et al. (Citation2017) and van Raamsdonk et al. (Citation2017b)). A general test for the detection of the presence of insects based on the gene coding for 18S RNA showed a positive signal for wheat and tomato as well (Debode et al. Citation2017). Using the wingless gene sequence instead, the detection of yellow mealworm (Tenebrio molitor) at the species level appeared to be successful. Specificity testing included 33 other insects species, 15 other non-insect animal species and seven plant species. The lesser mealworm (Alphitobius diaperinus) yielded no signal. The only close relative to the yellow meal worm (dark mealworm, T. obscurus) was not included in the study (Debode et al. Citation2017). The situation might exist that the test detects Tenebrio at the genus level, or might even include related genera. A PCR based method for the detection of black soldier fly has been developed with a high level of specificity and sensitivity (Zagon et al. Citation2018). Methods for detection of insects species which are authorised in fish feeds in the EU, such as yellow mealworm and black soldier fly, are restricted in applicability to detect carry-over to production lines for other types of feed in which insects are still prohibited. Then it would be valuable to design such methods based on feed for terrestrial animals instead of aquafeed as in the study of Zagon et al. (Citation2018). Moreover, a scope covering (large) groups of insects at higher classification levels might be more powerful for monitoring the complete ban of insects in feed for terrestrial animals (Sint et al. Citation2014).
The omnipresence of DNA in living nature is an advantage, and it is a limiting factor at the same time. Legal ingredients contain the same DNA information as prohibited ingredients of the same species. The most important examples are milk and milk products, proteins derived from hides and skin of ruminants, gelatine and blood products of pigs, and feather meal and eggs of poultry. Also, hydrolysed material may contain detectable DNA (Scholtens et al., Citation2019) while this is not considered to be a PAP. A related problem is the usual presence of gulls and other sea birds in caught fishmeal. In the latter case a poultry detection method with a too wide specificity will give inappropriate results. Therefore, precise flow schemes with a defined order of application of microscopy and PCR are available for feed for aquaculture and for feed for terrestrial animals, developed by the EURL-AP (EURL-AP Citation2015). These schemes will prevent at least a part of the possible confusion. Another approach is to apply methods with a specificity not only for species, but for tissues as well.
Protein based methods: antibodies
Proteins are principally based on the same information as provided by DNA. There is, however, an important difference. DNA is present in every cell, while the expressed protein is only present in the tissue where the protein is targeted to. This characteristic provides the major advantage of protein based methods to identify species-tissue combinations. In the view of the rendering procedures of PAPs, heat stability of proteins is an important constraint. Troponin appears to be a protein that is heat resistant and highly expressed in muscle tissues, functioning, along with myosin and actin, in the muscle contraction complex of skeletal muscles. Cardiac muscle fibres show specified versions of troponin. Although these proteins are found in a wide range of animals including insects (Oota and Saitou Citation1999; Wendt and Leonard Citation1999) with a rather conserved function, differences between species and species groups do exist in the sequence of amino acids.
Antibodies have been raised against species-specific versions of troponin, which are applied in a range of commercially available ELISA kits. The specificity and sensitivity of these kits usually need additional evaluation. Two commercial ruminant kits have been demonstrated to be sufficiently specific with a sensitivity of ruminant PAP in six different pig and poultry PAPs at a level of 0.5% (Bremer et al. Citation2013; van Raamsdonk et al. Citation2015a). Four commercial kits for the detection of troponin in pig PAPs have been evaluated. It appeared that for all four kits both the sensitivity and specificity showed issues. One notable issue relates to the erroneous detection of pig blood products (RIKILT, unpublished results). It appeared to be difficult to detect bovine specific markers based on peptide sequences, detectable at 1%, in porcine and vegetal matrices (Marbaix et al. Citation2016). In the framework of a project with public-private cooperation RIKILT tried to develop a poultry specific antibody with no success until now. Based on troponin as target, in silico analysis of the sequence revealed only one amino acid difference for discriminating chicken and turkey from American crow (Corvus brachyrhynchos) and killdeer (Charadrius vociferus). These two species are not particularly related to poultry, as they do not belong to the Galloanserae (RIKILT, unpublished results).
Tissue- or processing specific methods
Besides the legal set of rendering methods, additional processes are described in the Feed Catalogue, such as hydrolysis (European Commission 2013 The effect is a modification of the material, and the final structure or composition cannot be predicted. The consequences of this situation can range from identification issues caused by the identical background of materials, such as blood (generally prohibited) versus blood products (generally authorised), to full conversion of the product, such as single amino acids after hydrolysis of proteins.
Principally, proteomics refers to methods which detect the protein composition of specific species-tissue combinations. Collagen ranges among the most abundant animal proteins, which can be subjected to proteomic procedures (Reece et al. Citation2012). Liquid Chromatography coupled with Mass Spectroscopy (LC-MS) has been applied with biomarkers to detect haemoglobin, casein and beta-lactoglobulin, as markers for blood products and milk products, respectively (Lecrenier, Lecrenier et al. Citation2016, Citation2018). Four different feeds for aquaculture have been used as matrices. The different proteins were successfully detected at a level of 0.1%. One feed showed a signal with the casein biomarker, which was interpreted as the correct detection of milk products in that feed (Lecrenier et al. Citation2018). The distinction between ruminant and porcine blood products was not evaluated, since one concatenated biomarker was designed for both haemoglobin origins. Eight materials originating from bovine (2), ovine (1), porcine (2) and poultry (3) have been subjected to a proteomic analysis based on ESI-MS/MS linked to an UltiMate 3000 nano-UPLC system (Rasinger et al. Citation2016). This study was carried out simultaneously in two laboratories with highly comparable results. The phylogenetic representation of the results showed a primary distinction between mammal and avian materials. Depending on the laboratory subset, either bovine muscle or bovine carcass material showed the highest conformity with ovine carcass instead of being mutually related (Rasinger et al. Citation2016). An approach to specifically distinguish between some authorised and prohibited materials using LC-ESI-MS/MS was followed by Marchis et al. (Citation2017). Biomarkers from a diversity of proteins were selected to successfully identify three types of PAPs (bovine, pork, fish) and to distinguish these from four different milk and whey samples (Marchis et al. Citation2017). Blood and blood products were not included. In a study of Steinhilber et al. (Citation2018) non-ruminant PAPs, a ruminant PAP, two milk powder samples and two spray dried plasma samples were successfully discriminated by a procedure including protein digestion followed by immunoprecipitation for selection of the target peptides, and application of a nanoflow Ultra High Pressure LC system coupled to quadrupole orbitrap MS. The legal requirement of detection of 0.1% (w/w) of a target in a vegetal compound feed was not achieved in all cases (Steinhilber et al. Citation2018). A major factor in these approaches is the time consumption and cost level, making them less applicable for routine analyses.
With respect to hydrolysed proteins two major analytical targets should be addressed as consequences of the legal requirements: molecular weight and source. It can be assumed that a high degree of hydrolysation, resulting in a high share of very small peptides or even single amino acids, will imply a limited availability of a species-specific target. A range of methods is available for characterisation of protein hydrolysates (Kristinsson and Rasco Citation2000). More specifically, Sodium dodecyl sulphate polyacrylamide gel electrophoresis (SDS-PAGE) and size exclusion high performance liquid chromatography (SEC-HPLC) can be used for establishing the distribution of the molecular weight of the remaining peptides after hydrolysis (Mekonnen et al. Citation2013; Quinto et al. Citation2018), but methods for identifying the source material are not available. Generally, available techniques or procedures for detection of molecular weight of proteins and peptides need optimisation and validation for the specific situation of monitoring hydrolysed proteins.
Profiling: spectral analysis
Identification methods based on profiles are targeting large arrays of data per sample without a necessary connection with specific components such as DNA or proteins. Advanced statistical analysis is necessary for extracting the discriminating factors. Visual light is just a small segment of the entire range of wavelengths of radiation, ready to be used for transfer of information. Near InfraRed Spectroscopy (NIRS) has been applied for detection and identification of PAPs from the beginning of the EU wide bans (Garrido-Varo et al. Citation2005; Murray et al. Citation2005). Being widely applied in composition analysis, it needs the presence of large databases with spectra for reference. The limit of detection for PAPs in feed by means of NIRS, as for any other ingredient contributing to the total composition, is generally higher than 1% (Veys et al. Citation2012b), considerably higher than the legal requirement. However, a general advantage of NIRS is the non-destructive nature and the broad applicability. One opportunity is the application of a portable spectrometer. A spectral database based on terrestrial animal PAPs, intended to detect biochemical characteristics such as crude protein, crude fat, ash, calcium and phosphor, was successfully transferred from a Foss NIR system to a portable NIR spectrometer (Garrido-Varo et al. Citation2016). In the assumed absence of methods for identifying pig and poultry PAPs, NIRS was tested to distinguish pork, poultry and fish PAPs. Correct identification was achieved in a validation set of 45 samples (15 each) of 92%, which is an improvement compared to a correctness of 83% using a traditional PLSDA model (Garrido-Novell et al. Citation2018).
Besides light, other sources of information can be used for applying profiling methods. Examples are the analysis of the volatile fraction of a sample, either pure PAPs or contaminated feeds (van Ruth et al. Citation2007) or the analysis of the fatty acid spectrum of PAPs (Pu et al. Citation2016). The opportunities resulting from these exploratory studies seems promising.
In situ combination of methods
Besides the combination of methods in a sequential order, where a second method is applied depending on the results of a firstly used method (EURL-AP Citation2015), two methods can be combined in a physical sense. The principle is to apply an identification method to material present on a microscopic slide (i.e. in situ), making a positive signal visible by means of a marker. The identification method can be based on a specific protein or a specific DNA sequence. In this way, troponin was detected in bovine muscle fibres using a visible light marker connected to a specific antibody (Bremer et al. Citation2012) and bovine DNA was detected in bone fragments by in situ PCR using a UV marker (Lecrenier et al. Citation2014). Another approach is microscopic dissection of individual muscle fibres from a slide, followed by PCR (Axmann et al. Citation2015).
The application of Near Infrared spectroscopy on single particles by means of a coupled microscope (NIRM) has the advantage that the high level expertise for visual identification can be replaced by automated identification based on a spectral database. The limitation of a high limit of detection as in NIRS can be avoided, as in visual light microscopy, since every particle is observed (Yang et al. Citation2011; Fernandez-Pierna et al. Citation2012). A comparable approach is the combination of Raman Spectroscopy with microscopy (Mandrile et al. Citation2017). One of the advantages of this combination is that authorised ingredients, milk products in particular, are expected to be correctly not detected, but this advantage was only mentioned and not evaluated further by Mandrile et al. (Citation2017). The correct discrimination between prohibited PAPs and legal ingredients originating from the same species is a shared advantage of visual light microscopy, protein-based methods, and all combination approaches in which microscopy (i.e. in situ identification) is included.
Marker: GTH, glycerol triheptanoate
Materials originating from animals not fit for human consumption (fallen stock, unhealthy or medicated animals, a.o.), from SRMs, and some other materials excluded for the food production chain could not, and still cannot, be distinguished from authorised materials based on intrinsic characteristics. In other words, the distinction between Category I and II material, and Category III material, need to be based on an additional marker. The addition to Category I and II material of the chemical marker GTH is mandatory. The analytical method for detection of GTH, based on gas chromatography coupled to detection by either mass spectroscopy or flame ionisation, is documented and validated (Von Holst et al. Citation2009; Woodgate et al. Citation2009; Boix et al. Citation2010).
Discussion
Definitions can be considered to act as connections between legislation and practical detection and identification procedures. Legal descriptions of groups in the sense of species composition are or should be the basis for the specificity of an analytical method. In a range of occasions only selected representatives of different biological groups, usually at the species level, are chosen to be legalised, prohibited or exempted in the legislation for PAPs. In ideal cases in modern biological classification, groups of living entities are defined and circumscribed on the basis of DNA information, and all the group members are assumed to have an evolutionary relationship with one common ancestor. This latter circumstance is of ultimate importance for the design of modern monitoring methods. The presence of a common ancestor allows to find characteristics at the DNA or protein level that are shared by all descendants, that is the species intended to be targeted in a analytical method, and ignoring a range of other species without a relationship with that ancestor which do not need enforcement. A group consisting of precisely all the descendants of one ancestor is indicated by the biological term ‘monophyletic’ (see de Queiroz and Donoghue (Citation1988) for a discussion on this terminology and other definitions for the concept of groups). The existence of groups that do not contain every descendent of an ancestor has little consequences in biological classification, but in the area of monitoring for legal enforcement, non-monophyletic groups are heterogeneous with, in some cases major, complications for the specificity of detection methods. The most striking example is that of poultry. Taking either a narrow or a broad definition for the circumscription of poultry (see underlinings in ), the species in the definitions include only a part of all the descendants of a common ancestor (indicated by X in ). This situation implies that a range of excluded species, having identical sequences for the vast majority of their DNA compared to the included species, can hardly be discriminated, or not at all. This situation is illustrated by the close resemblance of the troponin amino acid sequence of some poultry species and some other bird species even outside the Galloanserae (American crow, killdeer; RIKILT, unpublished results). The description of ‘domestic bovine (including Bubalus and Bison species)’ in Regulation (EC) 853/2004 might be meant to exclude nyala, kudu and relatives (), but then is still circumscribed as a homogeneous (‘monophyletic’) group, covering the main group of the Bovinae. In contrast, Regulation (EU) 2016/1821 mentions ‘bovine animals (cattle, buffalo)’, apparently excluding bison. This exclusion leaves the remainder as a ‘non-monophyletic’ group.
Throughout legislation binomen and trinomen are being used interchangeably. A trinomen consists of three parts in the Latin name: for example Bos primigenius taurus for cattle. This use is based on the opinion that after domestication a farmed animal should still be considered part of the wild genetic population and, hence, part of the original wild species, since interbreeding is likely to occur. This might be correct in a biological sense in a series of cases. However, in Annex I of Regulation (EU) 2018/62, difference is made between products of farmed animals (Part 1) and additional products (Part 2), which might be valuable from a legal point of view. Therefore, in a specific and practicable framework as in legislation, domestic animals might in principle be indicated at the species level using only binomen. Examples are Bos taurus instead of Bos primigenius taurus for cattle, Sus domesticus instead of Sus scrofa domesticus for pigs, Capra hircus instead of Capra aegragus hircus for goat, and Gallus domesticus instead Gallus gallus domesticus for chicken. One exemption to the use of Latin binomen can be the naming of the domestic horse. Its wild relatives, tarpan (Equus ferus ferus) and the Przewalski horse (E. ferus przewalski), are extinct or endangered, respectively, with the consequence that the domestic horse is the major group within the species E. ferus (Grubb Citation2005). It is commonly accepted to indicate the domestic horse with its trinomen. In general, the use of Latin binomen allows easy discrimination with the wild relatives and is principally not incorrect in the view of multiple existing definitions of ‘species’.
The use of Latin names at any level can help for clarification. The situation that mealworms are no real worms is sufficiently indicated in the list of authorised insects with their Latin names (European Commission 2017c). Starfish is indicated by its Latin species name in the definition of fish meal (European Commission 2017d), with the consequence that all other starfish species are apparently excluded. Shell fish is no fish either, but here the indication is given in a general sense, presumably pointing to bivalves (e.g. clams, mussels, oysters) or even more general to molluscs (including snails, squids a.o.). Under such circumstances of diverse, unclear or incomplete circumscriptions of targets it is extremely difficult to design a monitoring method with sufficient specificity. Since susceptibility to a version of prion disease, or more general to a certain zoonotic disease, might be an issue in all species of a certain group (see ), legal restrictions might be put in force at higher classification levels than species. It is then beneficial to include all species or representatives of that group in the definition. It is therefore advocated to define restrictions for (groups of) species at appropriate biological classification levels.
The discussion as presented demonstrates that definitions in the area of zoonotic diseases, acting as bridges between legal prohibitions or authorisations and analytical methods as tools for monitoring, require a proper and clear biological basis. This principle applies to other areas of biological products as well. A range of toxic plants is restricted as feed ingredient according to Directive 2002/32/EC (European Union Citation2002c). In most cases these plants are indicated as the species level. It was, however, recognised that seeds of Datura stramonium containing tropane alkaloids, cannot be distinguished from the seeds of other Datura species. Commission Directive 2009/124/EC note 8 (European Commission Citation2009d) amending Directive 2002/32/EC states: ‘As regards alkaloids in Datura sp., EFSA concluded in its opinion of 9 April 2008 that, since tropane alkaloids are present in all Datura sp., it is appropriate for the protection of animal health, in particular for pigs, to extend the maximum levels for D. stramonium L., as set out in Annex I to Directive 2002/32/EC, to all Datura sp.’ (EFSA Citation2008). Most families of plant toxins are present in groups of related species. An important example is the group of pyrrolizidine alkaloids, which is primarily present in the tribes Senecionae and Eupatoriae of the family Compositae, and in the species of the family Boraginaceae (Colegate and Stegelmeier Citation2012). The most notable species is ragwort (Senecio jacobaea). Together with groundsel (S. vulgaris) these species can infest fodder. It is therefore argued to define restrictions for toxic plants at appropriate biological classification levels higher than species (van Raamsdonk et al. Citation2015b). This applies not only to prohibited biological targets such as toxic plants and pests, but to groups of susceptible species as well. As far as traceable, the Datura example is so far the only known case in which a legal indication at the species level is raised to the genus level in order to assure the possibility for proper monitoring.
Recommendations
Definitions are primarily meant to function in the required scope. If, for example, the prevention of zoonotic diseases such as avian influenza, requires a definition of ‘poultry’ as broad as all birds in captivity, then the description should be applied as such. It could be recommended to use different terms to indicate these different definitions, in order to avoid confusion, in this example of the term ‘poultry’. If a term was already defined outside the legal framework, such as ‘ruminants’ in the biological domain, an adoption identical to the already existing definition would be beneficial. Based on the presented discussion, and considering all relevant factors, a first attempt can be given to provide balanced definitions of necessary categories in the monitoring of PAPs. Some definitions might include only very minor modification of existing ones, other descriptions are additional. It is the intention to refer to biological classification as precise as possible. A particular hierarchy depends on the biological data which are used as basis, and evaluations made by different taxonomists can result in different hierarchical levels, for example suborder versus order. The proposed descriptions are build up from the species level in most cases in order to be sure that the most important species are included no matter that classification will be used as reference. An overview of the proposals is presented in .
Table 4. Proposals for definitions for biological groupings in legislation for the eradication of TSEs.
Definitions as presented in intended to achieve the desired clear circumscriptions still seem to contain possibilities for open interpretation. The phrase ‘at least including’ points to the species or genera which needs to remain part of the defined group no matter which new biological evidence might suggest a change in biological classification. The addition ‘but not necessarily so’ opens the possibility that in a particular case inclusion or exclusion can be made specific. For example, ‘fish’ including the cartilaginous fish or excluding them will be based on the circumscription of a monophyletic group in both cases. Some terms are not included in . The list of insects in Regulation (EU) 893/2017 includes representatives of three insect orders: Diptera (flies and mosquitoes: black soldier fly, common housefly), Coleoptera (beetles: yellow mealworm, lesser mealworm), and Orthoptera (grasshoppers and relatives: house cricket, banded cricket and field cricket). Within the class of insects, the order Coleoptera is the largest group with an estimated 400,000 species (Hammond Citation1992). Grasshoppers, being kept for food consumption as well, are related to crickets, but currently not authorised. Since the current choice of insects is both limited and erratic in the sense of its distribution in the classification of insects, a definition or circumscription of a group is premature. The term ‘poultry’ is already used for different scopes and with different descriptions. An attempt to present a ‘final’ definition might lead to increased confusion instead of a solution. Therefore, other terms such as fowl are introduced. Other Regulations, particularly in legislation pertaining to trade, provide their own classification with coding structure, most notably in the Combined Nomenclature (European Commission 2016b). This classification points in most cases to terms as listed in . Finally, and most importantly, a basic set of definitions is readily present in current legislation concerning product typification, general terms such as several indications for ‘animal’, trade, process descriptions and manufacturing (Regulation (EC) 999/2001, article 3; Regulation (EC) 1069/2009, article 3; Regulation (EU) 142/2011, Annex I).
Legislation is intended, as previously stated, to reckon social and health demands, environmental constraints and economic interests. This can be extended to facilitate good opportunities for analytical solutions. On the other hand, monitoring methods are just instruments for effective and efficient enforcement of legal measures. Besides clear definitions, good communication among legal experts and scientists in the course of the development of legal measures and opportunities for monitoring is vital. For several aspects in the monitoring of the presence of prohibited PAPs convenient or acceptable monitoring methods are at hand (). However, in several areas of the entire range of prohibitions monitoring tools are not readily available. This includes the monitoring of hydrolysed proteins, and the discrimination between prohibited and authorised versions of specifically processed materials. A method which is based on a biomarker that will co-detect bovine and porcine haemoglobin (Lecrenier et al. Citation2018) cannot be expected to distinguish among these two products, as is required by legislation. The information available for the construction of the biomarker, however, can act as basis for a further development of the method.
Table 5. Overview of analytical methods available and supporting EU legislation for Constituents of animal origin. Grey shading: methods to be optimised and validated.
The WISE principle for development of legislation has been launched to pay attention to several requirements: Witfull (reasonable legal principles), Indicative (clear limits between prohibition and authorisation), Societal demands (public health, environment, economy), and Enforceable (presence of suited monitoring methods) (van Raamsdonk et al. Citation2017b). Comparable principles were included in the issues listed by Robert Maxwell, which play their role in the risk evaluation of BSE in the United Kingdom (see Randall Citation2009). One notable issue addresses the question how to manage and improve the relationship between policy, politics and science (see Randall Citation2009: p. 78). The optimisation of the opportunity to have suited monitoring methods is dependent on the description and circumscription of the limits between prohibition and authorisation. Societal demands are of equal importance. Flexibility needs to remain at the maximum level for enforcement focusing on either public or animal health, environment or trade. If, for example in a case of aviary influenza, it renders necessary to restrict free ranging of all captive birds, a suited definition should provide a legal basis for monitoring. Domains such as international trade, animal welfare or human health have their individual needs for designing definitions supporting desired enforcement. Definitions as presented in , intended to achieve the desired clear limits, are developed in the view of sustainability by achieving maximum robustness and independency of future changes in biological classification. The current evaluation and recommendations have the intention to stimulate the further improvement of cooperation between legal action, enforcement and science, both analytical and biological, with the major aim to support society and public health.
Supplemental Material
Download PDF (163 KB)Acknowledgements
This study was financed by the Dutch Ministry of Agriculture, Nature Management and Food Quality, contract number WOT-02-004-016.
Disclosure statement
No potential conflict of interest was reported by the authors.
Supplementary Material
Supplementary data for this article can be accessed on the publisher’s website.
Additional information
Funding
References
- Ali ME, Razzak MA, Hamid SBA, Rahman MM, Amin MA, Rashid NRA. 2015. Multiplex PCR assay for the detection of five meat species forbidden in Islamic foods. Food Chem. 177:214–224.
- Axmann S, Adler A, Brandstettner AJ, Spadinger G, Weiss R, Strnad I. 2015. Species identification of processed animal proteins (PAPs) in animal feed containing feed materials from animal origin. Food Addit Contam Part A. 32:1089–1098.
- Babelhadj B, Di Bari MA, Pirisinu L, Chiappini B, Suheil Gaouar SB, Riccardi G, Marcon S, Agrimi U, Nonno R, Vaccari G. 2018. Prion disease in dromedary camels, Algeria. Emerg Infect Dis. 24:1029–1036.
- Bai JL, Jiang JY, Yang J. 2014. Taxonomy and phylogenesis of Chinese yak based on the complete sequence of mitochondrial cytochrome b gene in Tianzhu white yak, Poephagus grunniens. Asian J Anim Vet Adv. 9:345–354.
- Barria MA, Ironside JW, Head MW. 2014. Exploring the zoonotic potential of animal prion diseases in vivo and in vitro approaches. Prion. 8:85–91.
- Barria MA, Telling GC, Gambetti P, Mastrianni JA, Soto C. 2011. Generation of a new form of human PrPSc in vitro by interspecies transmission from cervid prions. J Biol Chem. 286:7490–7495.
- Boix A, Bellorini S, von Holst C. 2010. Validation of an analytical method for the determination of glyceroltriheptanoate (GTH) in processed animal by-products: results of a collaborative study. Food Addit Contam Part A. 27:793–800.
- Bremer MGEG, Fumière O, Hekman W, Marien A, Kemmers-Vonken A, Fernández Pierna JA, Vliege J, Baeten V, van Raamsdonk LWD, Berben G. 2012. Combination methods for PAP detection and species determination of animal particles. In: Jørgensen JS, Baeten V, editors. Detection, identification and quantification of processed animal proteins in feedingstuffs. Namur: Presses universitaires de Namur; p. 139–149.
- Bremer MGEG, Margry RJCF, Vaessen JCH, Van Doremalen AMH, Van Der Palen JGP, Van Kaathoven RGC, Kemmers-Vonken AEM, Van Raamsdonk LWD. 2013. Evaluation of a commercial ELISA for detection of ruminant processed animal proteins in non-ruminant processed animal proteins. J AOAC. 96:552–559.
- Chronology of mad cow crisis. 2000. CBS OnLine; [accessed 2018 Jul 24]. https://www.cbsnews.com/news/chronology-of-mad-cow-crisis/.
- CEN. 2018. Technical committee 327 animal feedingstuffs: methods of sampling and analysis; [accessed 2018 Jul 24]. http://standards.cen.eu/dyn/www/f?p=204:7:0::::FSP_ORG_ID:6308&cs=151B54DCC1DA2676999693FCE3A6F61BC.
- Cianci R, Gambrel PA. 2003. Maslow’s hierarchy of needs: does it apply in a collectivist culture. J Appl Manage Entrepr. 8:143.
- Colegate SM, Stegelmeier BL. 2012. Dietary exposure of livestock and humans to hepatotoxic natural products. In: Fink-Gremmels J, editor. Animal feed contamination. Cambridge (UK): Woodhead Publishing Ltd.
- Collee JG. 2000. Transmissible spongiform encephalopathies. In: Lund BM, Baird-Parker TC, Gould GW, editors. The microbiological safety and quality of food. Gaithersburg (MD): Aspen Publishers Inc; p. 1589–1624.
- Collinge J, Whitfield J, McKintosh E, Beck J, Mead S, Thomas DJ, Alpers MP. 2006. Kuru in the 21st century - an acquired human prion disease with very long incubation periods. Lancet. 367:2068–2074.
- Czub S, Schulz-Schaeffer W, Stahl-Hennig C, Beekes M, Schaetzl H, Motzkus D 2017. First evidence of intracranial and peroral transmission of Chronic Wasting Disease (CWD) into Cynomolgus macaques: a work in progress. Conference abstract PRION 2017; Edinburgh.
- Davenport KA, Henderson DM, Bian JF, Telling GC, Mathiason CK, Hoover EA. 2015. Insights into chronic wasting disease and bovine spongiform encephalopathy species barriers by use of real-time conversion. J Virol. 89:9524–9531.
- de Queiroz K, Donoghue MJ. 1988. Phylogenetic systematics and the species problem. Cladistics Int J Willi Hennig Soc. 4:317–338.
- Debode F, Marien A, Gerard A, Francis F, Fumière O, Berben G. 2017. Development of real-time PCR tests for the detection of Tenebrio molitor in food and feed. Food Addit Contam Part A. 34:1421–1426.
- Die Chronologie der BSE-Krise. 2000. Rinderseuche; Spiegel Online; [accessed 2018 Jul 24]. http://www.spiegel.de/politik/ausland/rinderseuche-die-chronologie-der-bse-krise-a-105210.html.
- Dickinson TA 1999. Species concepts in agamic complexes. In: van Raamsdonk LWD, Den Nijs JCM, editors. Proceedings of the VIIth symposium of the International Organisation of Plant Biosystematists, Amsterdam.
- Dobzhansky T, Alaya FJ, Stebbins GL, Valentine JW. 1977. Evolution. San Francisco (CA): Freeman and Company.
- [EFSA] European Food Safety Authority. 2008. Scientific opinion of the panel on contaminants in the food chain on a request from the European Commission on tropane alkaloids (from Datura sp.) as undesirable substances in animal feed. EFSA J. 691:1–55.
- [EFSA BIOHAZ Panel] EFSA Panel on Biological Hazards. 2017. Ricci A, Allende A, Bolton D, Chemaly M, Davies R, Fernández Escámez PS, Gironés R, Herman L, Koutsoumanis K, et al. Scientific opinion on chronic wasting disease (CWD) in cervids. Efsa J. 15:62.
- Engling FP, Jørgenson JS, Paradies-Severin I, Hahn H. 2000. Evidence of animal meal in feeds. FeedMagazine/Kraftfutter. 1:14–17.
- Eo SH, Bininda-Emonds ORP, Carroll JP. 2009. A phylogenetic supertree of the fowls (Galloanserae, Aves). Zool Scr. 38:465–481.
- [EURL-AP] European Union Reference Laboratory for Animal Proteins in feedingstuffs. 2015. EURL-AP standard operating procedure: operational protocols for the combination of light microscopy and PCR, Version 3.0; [accessed 2015 May 13]. http://eurl.craw.eu/en/187/method-of-reference-and-sops.
- [EURL-AP] European Union Reference Laboratory for Animal Proteins in feedingstuffs. 2017. EURL-AP standard operating procedure: detection of ruminant DNA in feed using real-time PCR, Version 1.2; [accessed 2017 Aug 17]. http://eurl.craw.eu/en/187/method-of-reference-and-sops.
- Europe’s Mad Cows. 2000. The Economist; [accessed 2018 Jul 24]. http://www.economist.com/node/436033
- European Commission. 1998 Nov 27. Commission directive 98/88/EC of 13 November 1998 establishing guidelines for the microscopic identification and estimation of constituents of animal origin for the official control of feedingstuffs. OJ L. 318:45–50. Luxembourg: The Publications Office of the European Union.
- European Commission. 2003a Dec 24. Commission directive 2003/126/EC of 23 December 2003 on the analytical method for the determination of constituents of animal origin for the official control of feedingstuffs. OJ L. 339:78–84. Luxembourg: The Publications Office of the European Union.
- European Commission. 2005a Aug 6. Commission regulation (EC) No 1292/2005 of 5 August 2005 amending Annex IV to Regulation (EC) No 999/2001 of the European Parliament and of the Council as regards animal nutrition. OJ L. 205:3–11. Luxembourg: The Publications Office of the European Union.
- European Commission. 2005b Dec 20. Directive 2005/94/EC of 20 December 2005 on Community measures for the control of avian influenza and repealing Directive 92/40/EEC. OJ L. 10:16–65.
- European Commission. 2008 Aug 8. Regulation (EC) No 798/2008 of 8 August 2008 laying down a list of third countries, territories, zones or compartments from which poultry and poultry products may be imported into and transit through the community and the veterinary certification requirements. OJ L. 226:1–94. Luxembourg: The Publications Office of the European Union.
- European Commission. 2009a Feb 26. Commission Regulation (EC) No 152/2009 of 27 January 2009 laying down the methods of sampling and analysis for the official control of feed. OJ L. 54:1–130. Luxembourg: The Publications Office of the European Union.
- European Commission. 2009b Feb 27. Commission Regulation (EC) No 163/2009 of 26 February 2009 amending Annex IV to Regulation (EC) No 999/2001 of the European Parliament and of the Council laying down rules for the prevention, control and eradication of certain transmissible spongiform encephalopathies. OJ L. 55:17–18.
- European Commission. 2009c Nov 30. Directive (EC) No 2009/158 of 30 November 2009 on animal health conditions governing intra-Community trade in, and imports from third countries of, poultry and hatching eggs. OJ L. 343:74–113.
- European Commission. 2009d Sep 26. Commission Directive 2009/124/EC of 25 September 2009 amending Annex I to Directive 2002/32/EC of the European Parliament and of the Council as regards maximum levels for arsenic, theobromine, Datura sp., Ricinus communis L., Croton tiglium L. and Abrus precatorius L. OJ L. 254:100–103.
- European Commission. 2010. The TSE roadmap 2. A strategy paper on transmissible spongiform encephalopathies for 2010-2015. SEC(2010)899. https://ec.europa.eu/food/sites/food/files/safety/docs/biosafety_food-borne-disease_tse_road-map2.pdf.
- European Commission. 2011 Feb 26. Commission regulation (EU) No 142/2011 of 25 February 2011 implementing Regulation (EC) No 1069/2009 of the European Parliament and of the Council laying down health rules as regards animal by-products and derived products not intended for human consumption and implementing Council Directive 97/78/EC as regards certain samples and items exempt from veterinary checks at the border under that directive. OJ L. 54:1–254. Luxembourg: The Publications Office of the European Union.
- European Commission. 2013a Jan 23. Commission Regulation (EU) No 51/2013 of 16 January 2013 amending Regulation (EC) No 152/2009 as regards the methods of analysis for the determination of constituents of animal origin for the official control of feed. OJ L. 20:33–43. Luxembourg: The Publications Office of the European Union.
- European Commission. 2013b Apr 6. Commission Regulation (EU) No 294/2013 of 14 March 2013 amending and correcting Regulation (EU) No 142/2011 implementing Regulation (EC) No 1069/2009 of the European Parliament and of the Council laying down health rules as regards animal by-products and derived products not intended for human consumption and implementing Council Directive 97/78/EC as regards certain samples and items exempt from veterinary checks at the border under that Directive. OJ L. 98:1–57.
- European Commission. 2013c Mar 11. Commission Regulation (EU) No 212/2013 of 11 March 2013 replacing Annex I to Regulation (EC) No 396/2005 of the European Parliament and of the Council as regards additions and modifications with respect to the products covered by that Annex. OJ L. 68:30–52.
- European Commission. 2013d Jan 7. Commission implementing Regulation (EU) No. 139/2013 of 7 January 2013 laying down animal health conditions for imports of certain birds into the Union and the quarantine conditions thereof. OJ L. 47:1–17.
- European Commission. 2013e Jan 30. Commission Regulation (EU) No 68/2013 of 16 January 2013 on the Catalogue of feed materials. OJ L. 29:1–64.
- European Commission. 2013f Jan 16. Commission Regulation (EU) No. 56/2013 of 16 January 2013 amending Annexes I and IV to Regulation (EC) No. 999/2001 of the European Parliament and of the Council laying down rules for the prevention, control and eradication of certain transmissible spongiform encephalopathies. OJ L. 21:3–16.
- European Commission. 2014 Jan 29. Commission Regulation No. 79/2014 of 29 January 2014 amending Annexes II, III and V to Regulation (EC) No 396/2005 of the European Parliament and of the Council as regards maximum residue levels for bifenazate, chlorpropham, esfenvalerate, fludioxonil and thiobencarb in or on certain products. OJ L. 27:9–55. Luxembourg: The Publications Office of the European Union
- European Commission. 2016a Apr 23. Commission implementing decision (EU) 2016/645 of 22 April 2016 concerning certain protective measures against lumpy skin disease in Bulgaria (notified under document C(2016) 3261). OJ L. 108:61–69. Luxembourg: The Publications Office of the European Union.
- European Commission. 2016b Oct 6. Commission implementing regulation (EU) 2016/1821 of 6 October 2016 amending Annex I to Council Regulation (EEC) No 2658/87 on the tariff and statistical nomenclature and on the common customs tariff. OJ L. 294:1–956.
- European Commission. 2017a Jan 17. Commission Implementing Regulation (EU) 2017/56 of 14 December 2016 concerning the authorisation of lactic acid, 4-oxovaleric acid, succinic acid, fumaric acid, ethyl acetoacetate, ethyl lactate, butyl lactate, ethyl 4-oxovalerate, diethyl succinate, diethyl malonate, butyl-O-butyryllactate, hex-3-enyl lactate, hexyl lactate, butyro-1,4-lactone, decano-1,5-lactone, undecano-1,5-lactone, pentano-1,4-lactone, nonano-1,5-lactone, octano-1,5-lactone, heptano-1,4-lactone and hexano-1,4-lactone as feed additives for all animal species. OJ L. 13:129–152. Luxembourg: The Publications Office of the European Union.
- European Commission. 2017b Jun 21. Commission regulation (EU) 2017/1017 of 15 June 2017 amending regulation (EU) No 68/2013 on the Catalogue of feed materials. OJ L. 159:48–119.
- European Commission. 2017c May 25. Commission regulation (EU) 2017/893 of 24 May 2017 amending annexes I and IV to Regulation (EC) No 999/2001 of the European parliament and of the council and annexes X, XIV and XV to commission regulation (EU) No 142/2011 as regards the provisions on processed animal protein. OJ L. 138:92–116.
- European Commission. 2017d May 9. Commission regulation (EU) 2017/786 of 8 May 2017 amending Regulation (EU) No 142/2011 as regards the definitions of fishmeal and fish oil. OJ L 119:1–3.
- European Commission. 2018 Jan 23. Commission regulation (EU) 2018/62 of 17 January 2018 replacing annex i to regulation (EC) No 396/2005 of the European parliament and of the council. OJ L. 18:1–73. Luxembourg: The Publications Office of the European Union.
- European Commission. 2001 May 31. Regulation (EC) No 999/2001 of the European parliament and of the council of 22 May 2001 laying down rules for the prevention, control and eradication of certain transmissible spongiform encephalopathies. OJ L. 147:1–40. Luxembourg: The Publications Office of the European Union.
- European Commission. 2002a Feb 1. Regulation (EC) No 178/2002 of the European parliament and of the council of 28 January 2002 laying down the general principles and requirements of food law, establishing the European food safety authority and laying down procedures in matters of food safety. OJ L. 31:1–24. Luxembourg: The Publications Office of the European Union.
- European Commission. 2002b Oct 10. Regulation (EC) No 1774/2002 of the European Parliament and of the Council of 3 October 2002 laying down health rules concerning animal by-products not intended for human consumption. OJ L. 273:1–95.
- European Commission. 2002c May 30. Directive 2002/32/EC of the European Parliament and of the Council of 7 May 2002 on undesirable substances in animal feed - Council statement. OJ L. 140:10–22.
- European Commission. 2003b Nov 22. Council directive 2003/85/EC of 29 September 2003 on community measures for the control of foot-and-mouth disease repealing directive 85/511/EEC and decisions 89/531/EEC and 91/665/EEC and amending directive 92/46/EEC. OJ L. 306:1–87. Luxembourg: The Publications Office of the European Union.
- European Commission. 2004a Apr 30. Regulation (EC) No 853/2004 of the European parliament and of the council of 29 April 2004 laying down specific hygiene rules for on the hygiene of foodstuffs. OJ L. 139:55–205. Luxembourg: The Publications Office of the European Union.
- European Commission. 2004b Apr 30. Regulation (EC) No 882/2004 of the European parliament and of the council of 29 April 2004 on official controls performed to ensure the verification of compliance with feed and food law, animal health and animal welfare rules. OJ L. 165:1–141.
- European Commission. 2005c Mar 16. Regulation (EC) No 396/2005 of the European parliament and of the council of 23 February 2005 on maximum residue levels of pesticides in or on food and feed of plant and animal origin and amending council directive 91/414/EEC. OJ L. 70:1–16. Luxembourg: The Publications Office of the European Union.
- European Commission. 2006 Dec 30. Regulation (EC) No 1923/2006 of the European parliament and of the council of 18 December 2006 amending regulation (EC) No 999/2001 laying down rules for the prevention, control and eradication of certain transmissible spongiform encephalopathies. OJ L. 404:1–8. Luxembourg: The Publications Office of the European Union.
- European Commission. 2009e Nov 14. Regulation (EC) No 1069/2009 of the European parliament and of the council of 21 October 2009 laying down health rules as regards animal by-products and derived products not intended for human consumption and repealing Regulation (EC) No 1774/2002 (animal by-products regulation). OJ L. 300:1–33.
- Fernandez MH, Vrba ES. 2005. A complete estimate of the phylogenetic relationships in Ruminantia: a dated species-level supertree of the extant ruminants. Biol Rev. 80:269–302.
- Fernandez-Pierna JA, Baeten V, Boix A, von Holst C, Perez Marin D, Garrido Varo A. 2012. Near infrared microscopy (NIRM). In: Jørgensen JS, Baeten V, editors. Detection, identification and quantification of processed animal proteins in feedingstuffs. Namur: Presses universitaires de Namur; p. 81–91.
- First case in Finland: elk dies due to chronic wasting disease. 2018. Uutiset – News; [accessed 2018 Jul 24]. https://yle.fi/uutiset/osasto/news/first_case_in_finland_elk_dies_due_to_chronic_wasting_disease/10108115.
- Fink-Gremmels J. 2012. Animal feed contamination. Effects on livestock and food safety. Cambridge: Woodhead Publishing Ltd.
- Fumière O, Marien A, Berben G. 2016a. Validation study of a real-time PCR method developed by TNO Triskelion bv for the detection of ruminant DNA in feedingstuffs. Final version 1.0. http://eurl.craw.eu/img/page/interlaboratory/Ruminant%20Validation%20Study%20-%20final%20version%201.0.pdf
- Fumière O, Marien A, Maljean J, Berben G 2016b. Validation of a selected pig real-time PCR assay for the detection of processed animal proteins in feedingstuffs. Proceedings of the Feed 2016, 5th International Feed Conference: Present and Future Challenges; Geel, Belgium. Abstract number 013.
- Fumière O, Veys P, Boix A, von Holst C, Baeten V, Berben G. 2009. Methods of detection, species identification and quantification of processed animal proteins in feedingstuffs. Biotechnol Agron Soc Environ. 13:59–70.
- Garrido-Novell C, Garrido-Varo A, Perez-Marín D, Emilio Guerrero J. 2018. Using spectral and textural data extracted from hyperspectral near infrared spectroscopy imaging to discriminate between processed pork, poultry and fish proteins. Chemometrics Intell Lab Syst. 172:90–99.
- Garrido-Varo A, Pérez-Marín MD, Guerrero JE, Gómez-Cabrera A, de la Haba MJ, Bautista J, Soldado A, Vicente F, Martínez A, de la Roza-Delgado B, et al. 2005. Near infrared spectroscopy for enforcement of European legislation concerning the use of animal byproducts in animal feeds. Biotechnol Agron Soc Environ. 9:3–9.
- Garrido-Varo A, Vega S, Maroto-Molina F, De la Haba MJ, Perez-Marin D. 2016. On-site quality control of processed land animal proteins using a portable micro-electro-mechanical-systems near infrared spectrometer. J Near Infrared Spectr. 24:47–58.
- Gatesy J, Amato G, Vrba E, Schaller G, DeSalle R. 1997. A cladistic analysis of mitochondrial ribosomal DNA from the Bovidae. Mol Phyl Evol. 7:303–319.
- Germany Confirms its first BSE case. 2000. The Independent; [accessed 2018 Jul 24]. http://www.independent.co.uk/news/world/europe/germany-confirms-its-first-bse-case-5367111.html.
- Giles K, Woerman AL, Berry DB, Prusiner SB. 2017. Bioassays and inactivation of prions. Cold Spring Harb Perspect Biol. 9(8):a023499.
- Gizzi G, von Holst C, Baeten V, Berben G, van Raamsdonk LWD. 2004. Determination of processed animal proteins, including meat and bone meal, in animal feed. J AOAC. 87(6):1334–1341.
- Grubb P. 2005. Mammal species of the world: a taxonomic and geographic reference. In: Order Perissodactyla. 3rd ed. Baltimore (MD): Johns Hopkins University Press; p. 630–631.
- Haley NJ, Hoover EA. 2015. Chronic wasting disease of cervids: current knowledge and future perspectives. Annu Rev Anim Biosci. 3:305–325.
- Hammond PM. 1992. Species inventory. global biodiversity, status of the earth’s living resources: a report. 1st ed. London: Chapman & Hall.
- Hannaoui S, Schatzl HM, Gilch S. 2017. Chronic wasting disease: emerging prions and their potential risk. PLoS Pathog. 11:e1006619.
- Herbst A, Velasquez CD, Triscott E, Aiken JM, McKenzie D. 2017. Chronic wasting disease prion strain emergence and host range expansion. Emerg Infect Dis. 23:1598–1600.
- Hughson AG, Race B, Kraus A, Sangaré LR, Robins L, Groveman BR, Saijo E, Phillips K, Contreras L, Dhaliwal V, et al. 2016. Inactivation of prions and amyloid seeds with hypochlorous acid. PLoS Pathog. 12(9):e1005914.
- Insecta encyclopedia of life. 2018. [accessed 2018 Jul 24]. http://www.eol.org/pages/344/overview.
- Imran M, Mahmood S. 2011. An overview of animal prion diseases. Virol J. 11:8.
- Islam FB, Uno Y, Nunome M, Nishimura O, Tarui H, Agata K, Matsuda Y. 2014. Comparison of the chromosome structures between the chicken and three Anserid species, the domestic duck (Anas platyrhynchos), Muscovy Duck (Cairina moschata), and Chinese goose (Anser cygnoides), and the delineation of their karyotype evolution by comparative chromosome mapping. J Poultry Sci. 51:1–13.
- [ISO] International Organization for Standardization. 2018. Technical committee 34/SC 10 animal feedingstuffs: [accessed 2018 Jul 24]. https://www.iso.org/committee/47924/x/catalogue.
- Jarvis ED, Mirarab S, Aberer AJ, Li B, Houde P, Li C, Ho SYW, Faircloth BC, Nabholz B, Howard JT, et al. 2014. Whole-genome analyses resolve early branches in the tree of life of modern birds. Science. 346:1320–1331.
- Jørgensen JS, Baeten V. 2012. Detection, identification and quantification of processed animal proteins in feedingstuffs. Namur: Presses universitaires de Namur.
- Kesmen Z, Celebi Y, Güllüce A, Yetim H. 2013. Detection of seagull meat in meat mixtures using real-time PCR analysis. Food Control. 34:47–49.
- Kristinsson HG, Rasco BA. 2000. Fish protein hydrolysates: production, biochemical, and functional properties. Crit Rev Food Sci Nutr. 40:43–81.
- Lecrenier MC, Ledoux Q, Berben G, Fumière O, Saegerman C, Baeten V, Veys P. 2014. Determination of the ruminant origin of bone particles using fluorescence in situ hybridization (FISH). Sci Rep. 4:5730.
- Lecrenier MC, Marbaix H, Dieu M, Veys P, Saegerman C, Raes M, Baeten V. 2016. Identification of specific bovine blood biomarkers with a non-targeted approach using HPLC ESI tandem mass spectrometry. Food Chem. 213:417–424.
- Lecrenier MC, Planque M, Dieu M, Veys P, Saegerman C, Gillard N, Baeten V. 2018. A mass spectrometry method for sensitive, specific and simultaneous detection of bovine blood meal, blood products and milk products in compound feed. Food Chem. 245:981–988.
- Liu X, Han LJ, Veys P, Baeten V, Jiang XP, Dardenne P. 2011. An overview of the legislation and light microscopy for detection of processed animal proteins in feeds. Micr Res Tech. 74:735–743.
- López-Andreo M, Aldeguer M, Guillén I, Gabaldón JA, Puyet A. 2012. Detection and quantification of meat species by qPCR in heat-processed food containing highly fragmented DNA. Food Chem. 134:518–523.
- Mandrile L, Amato G, Marchis D, Martra G, Rossi AM. 2017. Species-specific detection of processed animal proteins in feed by Raman spectroscopy. Food Chem. 229:268–275.
- Marbaix H, Budinger D, Dieu M, Fumièere O, Gillard N, Delahaut P, Mauro S, Raes M. 2016. Identification of proteins and peptide biomarkers for detecting banned processed animal proteins (PAPs) in meat and bone meal by mass spectrometry. J Agric Food Chem. 64:2405–2414.
- Marchis D, Altomare A, Gili M, Ostorero F, Khadjavi A, Corona C, Ru G, Cappelletti B, Gianelli S, Amadeo F, et al. 2017. LC-MS/MS identification of species-specific muscle peptides in processed animal proteins. J Agricult Food Chem. 65:10638–10650.
- Maslow AH. 1943. A theory of human motivation. Psychol Rev. 50:370–396.
- Maslow AH. 1945. Motivation and personality. New York (NY): Harper.
- Mekonnen TH, Mussone PG, Stashko N, Choi PY, Bressler DC. 2013. Recovery and characterization of proteinacious material recovered from thermal and alkaline hydrolyzed specified risk materials. Process Biochem. 48:885–892.
- Michard J, Ziebal R. 1999. Note concernant le protocole de détection des farines de viande, d’os et de poisson dans les aliments pour animaux. Annal Fals et de Expertise Chimique et Toxicol. 947:209–223.
- Motalib Hossain MA, Ali ME, Asing Hamid SBA, Mustafa S, Mohd Desa MN, Zaidul ISM. 2017. Targeting double genes in multiplex PCR for discriminating bovine, buffalo and porcine materials in food chain. Food Control. 73:175–184.
- Murray I, Garrido Varo A, Pérez Marín D, Guerrero JE, Baeten V, Dardenne P, Termes S, Zegers J, Frankhuizen R. 2005. Macroscopic near-infrared reflectance spectroscopy. In: STRATFEED coordinating team, editors. Stratfeed Strategies and methods to detect and quantify mammalian tissues in feedingstuffs. 27 p. Brussels: Office for Official Publications of the European Communities.
- Myers MJ, Farrell DE, Deaver CM, Mason J, Swaim HL, Yancy HF. 2010. Detection of rendered meat and bone meals by PCR is dependent on animal species of origin and DNA extraction method. J Food Prot. 73:1090–1096.
- Norwegian Veterinary Institute. 2018. CWD in Finland is different from the Nordfjella CWD type. Norwegian website March 8th 2018; [accessed 2018 Jul 24]. https://wwweng.vetinst.no/news/cwd-in-finland-is-different-from-the-nordfjella-cwd-type.
- Olsvik PA, Fumière O, Margry R, Berben G, Larsen N, Alm M, Berntssen MHG. 2017. Multi-laboratory evaluation of a PCR method for detection of ruminant DNA in commercial processed animal proteins. Food Control. 73:140–146.
- Oota S, Saitou N. 1999. Phylogenetic relationship of muscle tissues deduced from superimposition of gene trees. Mol Biol Evol. 16:856–867.
- Ottoboni M, Tretola M, Cheli F, Marchis D, Veys P, Baeten V, Pinotti L. 2017. Light microscopy with differential staining techniques for the characterisation and discrimination of insects versus marine arthropods processed animal proteins. Food Additives Contaminants Part A. 34:1377–1383.
- Pain S 1987. Brain disease drives cows wild. NewScientist Daily News 5 November 1987 [accessed 2018 Jul 24]. https://www.newscientist.com/article/dn111-brain-disease-drives-cows-wild.
- Pegels N, González I, Fernández S, García T, Martín R. 2012a. Sensitive detection of porcine DNA in processed animal proteins using a TaqMan real-time PCR assay. Food Additives Contaminants Part A. 29:1402–1412.
- Pegels N, González I, Garcia T, Martin R. 2011. Detection of banned ruminant-derived material in industrial feedstuffs by TaqMan real-time PCR assay. J Food Protection. 74:1300–1308.
- Pegels N, González I, Garcia T, Martin R. 2014. Avian-specific real-time PCR assay for authenticity control in farm animal feeds and pet foods. Food Chem. 142:39–47.
- Pegels N, González I, Lopez-Calleja I, Fernandez S, Garcia T, Martin R. 2012b. Evaluation of a TaqMan real-time PCR assay for detection of chicken, turkey, duck, and goose material in highly processed industrial feed samples. Poult Sci. 91:1709–1719.
- Pico Y. 2012. Chemical analysis of food. Techniques and application Amsterdam (NH). the Netherlands: Elsevier/Academic Press.
- Pritzkow S, Morales R, Moda F, Khan U, Telling GC, Hoover E, Soto C. 2015. Grass plants bind, retain, uptake, and transport infectious prions. Cell Rep. 11:1–8.
- Prusiner S. 1984. Some speculations about prions, amyloid, and Alzheimer’s disease. New England J Med. 310:661–663.
- Pu Q, Han L, Liu X. 2016. A new approach for species discrimination of different processed animal proteins based on fat characteristics. Eur J Lipid Sci Tech. 118:576–583.
- Quinto BPT, Albuquerque JV, Bezerra RS, Peixoto S, Soares R. 2018. Replacement of fishmeal by two types of fish protein hydrolysate in feed for postlarval shrimp Litopenaeus vannamei. Aquacult Nutr. 24:768–776.
- Race B, Meade-White KD, Miller MW, Barbian KD, Rubenstein R, LaFauci G, Cervenakova L, Favara C, Gardner D, Long D, et al. 2009. Susceptibilities of nonhuman primates to chronic wasting disease. Emerg Infect Dis. 15:1366–1376.
- Randall E. 2009. Food, risk and politics. Scare, scandal and crisis – insights into the risk politics of food safety Manchester. United Kingdom: Manchester University Press.
- Rasinger JD, Marbaix H, Dieu M, Fumière O, Mauro S, Palmblad M, Raes M, Berntssen MHG. 2016. Species and tissues specific differentiation of processed animal proteins in aquafeeds using proteomics tools. J Proteomics. 147:125–131.
- Reece P, Chassaigne H, Collins MJ, Buckley M, Bremer MGEG, Grundy H. 2012. Proteomic analysis of meat and bone meal and animal feed. In: Jørgensen JS, Baeten V, editors. Detection, identification and quantification of processed animal proteins in feedingstuffs. Namur: Presses universitaires de Namur; p. 113–124.
- Rosa J. 2006. How was the French BSE epidemic underestimated? C R Biol. 329:106–116.
- Sartore S, Rasero R, Colussi S, Acutis PL, Peletto S, Soglia D, Maione S, Spalenza V, Sacchi P. 2013. Effect of selection for scrapie resistance on genetic diversity in a rare and locally adapted sheep breed: the case of Sambucana. Livestock Science. 157:75–80.
- Scholtens IMJ, Prins TW, Margry RJCF, Dahlmans H, van Raamsdonk LWD. 2019. Applicability of the poultry qPCR method to detect DNA of poultry processed animal protein materials. Food Control. 96:53–58.
- Scholtens IMJ, Prins TW, van Raamsdonk LWD. 2017. Specificity of a novel TaqMan PCR method for detection of poultry DNA. Food Control. 73:532–539.
- Sint D, Niederklapfer B, Kaufmann R, Traugott M. 2014. Group-specific multiplex PCR detection systems for the identification of flying insect prey. Plos One. 9(12):e115501.
- Steinhilber AE, Schmidt FF, Naboulsi W, Planatscher H, Niedzwiecka A, Zagon J, Braeuning A, Lampen A, Joos TO, Poetz O. 2018. Mass spectrometry-based immunoassay for the quantification of banned ruminant processed animal proteins in vegetal feeds. Analytical Chem. 90:4135–4143.
- Stepanek O, Horin P. 2017. Genetic diversity of the prion protein gene (PRNP) coding sequence in Czech sheep and evaluation of the national breeding programme for resistance to scrapie in the Czech Republic. J Appl Gen. 58:111–121.
- Takeda K, Satoh M, Neopane SP, Kuwar BS, Joshi HD, Shrestha NP, Fujise H, Tasai M, Tagami T, Hanada H. 2004. Mitochondrial DNA analysis of Nepalese domestic dwarf cattle Lulu. Anim Sci J. 75:103–110.
- van der Meulen B. 2014. EU food law handbook. Wageningen (the Netherlands): Wageningen Academic Publishers.
- van der Spiegel M, Noordam MY, van der Fels-Klerx HJ. 2013. Safety of novel protein sources (insects, microalgae, seaweed, duckweed, and rapeseed) and legislative aspects for their application in food and feed production. Compr Rev Food Sci Food Saf. 12:662–678.
- van Raamsdonk LWD, Margry RJCF, van Kaathoven RGC, Bremer MGEG. 2015a. Inter-laboratory validation study of two immunochemical methods for detection of processed ruminant proteins. Food Chem. 185:333–339.
- van Raamsdonk LWD, Ozinga WA, Hoogenboom LAP, Mulder PPJ, Mol JGJ, Groot MJ, van der Fels-Klerx HJ, de Nijs M. 2015b. Exposure assessment of cattle via roughages to plants producing compounds of concern. Food Chem. 189:27–37.
- van Raamsdonk LWD, Pinckaers VGZ, Scholtens IMJ, Prins TW, van der Voet H, Vliege JJM. 2014. IAG ring test animal proteins 2014. Report 2014.011. Wageningen: RIKILT, p. 47.
- van Raamsdonk LWD, Pinckaers VGZ, Vliege JJM. 2012a. Animal proteins in feed. IAG ring test 2012. Wageningen: WUR, report RIKILT 2012.009.
- van Raamsdonk LWD, Pinotti L, Veys P, Campagnoli A, Paltanin C, Belinchón Crespo C, Jørgensen JS. 2012b. Markers for microscopic detection. In: Jørgensen JS, Baeten V, editors. Detection, identification and quantification of processed animal proteins in feedingstuffs. Namur: Les éditions namuroises; p. 59–69.
- van Raamsdonk LWD, Pinckaers VGZ, Vliege JJM 2013. Animal proteins in feed. IAG ring test 2013. Report 2013.016. Wageningen: RIKILT, p. 35.
- van Raamsdonk LWD, Prins TW, van de Rhee N, Vliege JJM, Pinckaers VGZ. 2017a. Microscopic recognition and identification of fish meal in compound feeds. Food Additives Contaminants Part A. 34:1364–1376.
- van Raamsdonk LWD, Scholtens IMJ, Ossenkoppele J, van Egmond H, Groot M 2011. Investigation into blood plasma in milk formula. Wageningen: WUR, report RIKILT 2011.003.
- van Raamsdonk LWD, van de Rhee N, Scholtens IMJ, Prins TW, Vliege JJM, Pinckaers VGZ. 2015c. IAG ring test animal proteins 2015. Report 2015.016. Wageningen: RIKILT, p. 32.
- van Raamsdonk LWD, van de Rhee N, Scholtens IMJ, Prins TW, Vliege JJM, Pinckaers VGZ. 2016. IAG ring test animal proteins 2016. Report 2016.008. Wageningen: RIKILT, p. 31.
- van Raamsdonk LWD, van der Fels-Klerx HJ, de Jong J. 2017b. New feed ingredients: the insect opportunity. Food Additives Contaminants Part A. 34:1384–1397.
- van Raamsdonk LWD, von Holst C, Baeten V, Berben G, Boix A, de Jong J. 2007. New developments in the detection and identification of processed animal proteins in feeds. Animal Feed Sci Techn. 133:63–83.
- van Ruth SM, van Raamsdonk L, Araghipour N, Colineau J. 2007. Detection of processed animal proteins in feedstuffs: evaluation of PTR-MS. Proceedings of the 3rd PTR-MS conference; Innsbruck: Innsbruck University Press, ISBN 3-902571-03-9, p. 238–242.
- Veys P, Baeten V. 2018. Protocol for the isolation of processed animal proteins from insects in feed and their identification by microscopy. Food Control. 92:496–504.
- Veys P, Berben G, Baeten V 2011. EURL-AP interlaboratory study microscopy 2010. Final version. Gembloux (Belgium).
- Veys P, Berben G, Baeten V 2012a. EURL-AP proficiency test microscopy 2011. Final version. Gembloux (Belgium).
- Veys P, Berben G, Dardenne P, Baeten V. 2012b. Detection and identification of animal by-products in animal feed for the control of transmissible spongiform encephalopathies. In: Fink-Gremmels J, editor. Animal Feed contamination. Cambridge (United Kingdom): Woodhead Publishing Ltd.
- VMK. 2017. CWD in Norway – a state of emergency for the future of cervids (Phase II). Opinion of the panel on Biological Hazards, ISBN: 978-82-8259-266-6. Oslo, Norway.
- Von Holst C, Boix A, Bellorini S, Serano F, Androni S, Verkuylen B, Margry R. 2009. Use of glyceroltriheptanoate as marker for processed animal by-products: development and validation of an analytical method. Food Additives Contaminants Part A. 26:466–474.
- Von Holst C, Honikel KO, Unglaub W, Kramer G, Anklam E. 2000. Determination of an appropriate heat treatment of animal waste using the ELISA technique: results of a validation study. Meat Sc. 54:1–7.
- Von Holst C, Unglaub W, Anklam E. 2001. Post process product control of rendering plant sterilization conditions by ELISA. J AOAC. 84:1793–1798.
- Wendt T, Leonard K. 1999. Structure of the insect troponin complex. J Mol Biol. 285:1845–1856.
- Wilson DE, Reeder DM. 2005. Bos taurus. Mammal species of the world: A taxonomic and geographic reference. 3rd ed. Baltimore (MD): Johns Hopkins University Press.
- Woodgate SL, van Den Hoven S, Vaessen J, Margry R. 2009. Control tools to detect processed animal proteins in feed and in animal by-products: specificity and challenges. Biotech Agron Soc Environ. 13(S):9–13.
- Wu SB, Zhang XY, Yue BS, Zeng B, Ran JG. 2010. Phylogenetic position of the takin (Budorcas taxicolor) and the yak (Bos grunniens) within the family Bovidae. Zootaxa. 2392:62–68.
- Yancy HF, Washington JD, Callahan L, Mason JA, Deaver CM, Farrell DE, Ha T, Sespico E, Falmlen D, Myers MJ. 2009. Development, evaluation, and peer verification of a rapid real-time PCR method for the detection of animal material. J Food Protection. 72:2368–2374.
- Yang ZL, Han LJ, Pierna JAF, Dardenne P, Baeten V. 2011. The potential of near infrared microscopy to detect, identify and quantify processed animal by-products. J near Infrared Spectr. 19:211–231.
- Zagon J, di Rienzo V, Potkura J, Lampen A, Braeuning A. 2018. A real-time PCR method for the detection of black soldier fly (Hermetia illucens) in feedstuff. Food Control 91:440–448.
- Zhao H, Du YL, Chen SM, Qing LL, Wang XY, Huang JF, Wu DD, Zhang YP. 2015. The prion protein gene polymorphisms associated with bovine spongiform encephalopathy susceptibility differ significantly between cattle and buffalo. Infect Genet Evol. 36:531–538.