ABSTRACT
Some of the chemicals in materials used for packaging food may leak into the food, resulting in human exposure. These include so-called Non-intentionally Added Substances (NIAS), many of them being unidentified and toxicologically uncharacterized. This raises the question of how to address their safety. An approach consisting of identification and toxicologically testing all of them appears neither feasible nor necessary. Instead, it has been proposed to use the threshold of toxicological concern (TTC) Cramer class III to prioritise unknown NIAS on which further safety investigations should focus. Use of the Cramer class III TTC for this purpose would be appropriate if amongst others sufficient evidence were available that the unknown chemicals were not acetylcholinesterase inhibitors or direct DNA-reactive mutagens. While knowledge of the material and analytical chemistry may efficiently address the first concern, the second could not be addressed in this way. An alternative would be use of a bioassay capable of detecting DNA-reactive mutagens at very low levels. No fully satisfactory bioassay was identified. The Ames test appeared the most suitable since it specifically detects DNA-reactive mutagens and the limit of biological detection of highly potent genotoxic carcinogens is low. It is proposed that for a specific migrate, the evidence for absence of mutagenicity based on the Ames test, together with analytical chemistry and information on packaging manufacture could allow application of the Cramer class III TTC to prioritise unknown NIAS. Recommendations, as well as research proposals, have been developed on sample preparation and bioassay improvement with the ultimate aim of improving limits of biological detection of mutagens. Although research is still necessary, the proposed approach should bring significant benefits over the current practices used for safety evaluation of food contact materials.
Introduction
Chemicals from which food contact materials (FCMs) are made can potentially migrate into food products and result in human exposure. These include those having a specified technological role in the manufacturing of the FCMs, such as monomers, plasticizers and antioxidants. These substances, often referred to as Intentionally Added Substances (IAS), are foreseeable and chemically identified. But it is also becoming increasingly apparent that FCMs can contain numerous other substances which are not directly added, the so-called Non-Intentionally Added Substances (NIAS). They originate from diverse sources including impurities in the starting raw materials (monomers, resins), as well as from chemical reactions and degradation occurring during the manufacturing processes. Their number and levels in FCMs may be more numerous and greater than what is generally reported for IAS. For specific FCMs, some NIAS may be anticipated based on knowledge of the chemistry and production processes and therefore can be structurally identified. But others are only partially characterised and many are chemically uncharacterized/unknown.
Classically, safety assessment of FCMs has focused mainly on IAS. These substances are known and readily available for testing. They can be submitted to standard regulatory toxicology studies from which safe levels of exposure can be established and this, combined with exposure estimates, can be used for risk assessment. The extent of toxicological testing depends upon anticipated exposure. Risk assessments can then be directly used to determine Specific Migration Limits (SML), on which risk management is based. However, it is increasingly evident that the currently used approach for safety assessment of FCMs is not entirely satisfactory and that greater focus on NIAS is warranted (Grob et al. Citation2006; Muncke Citation2009; Schug et al. Citation2013; Koster et al. Citation2015a; European Parliament Citation2016). According to European regulation, it should be ensured that migration of any chemical into food from FCMs does not result in quantities sufficient to endanger human health. The practical application of this principle is not straightforward since many NIAS are analytically (identification, quantification) and toxicologically uncharacterized. It is increasingly acknowledged that an approach based on the identification and quantification of all substances together with their toxicological investigation is neither practical (too resource consuming) nor desirable (many are likely to be of negligible concern). In this context, there is an urgent need to develop a robust and agreed upon methodology to identify those NIAS that are of potential concern, on which targeted additional investigations should be focused. This is particularly important for the ones potentially mutagenic for which no safe levels may be expected. In the present paper, the potential contribution of in vitro bioassays for that specific purpose has been addressed.
Safety assessment of FCMs: a new paradigm
It is widely accepted that safety assessment of a specific FCM involves migration studies using appropriate food simulants (European Commission Citation2011) consisting, for example, of ethanol and water in conjunction with relevant contact time and temperature conditions (European Commission Citation2011). The resulting migrates are then concentrated and submitted to a range of analytical procedures. Targeted and untargeted chemical analyses using gas chromatography (GC)- and liquid chromatography (LC)-mass spectrometry (MS), including high-resolution MS, provide key information on migrate composition (IAS and NIAS) including number, estimated levels and identity of chemicals present. In general, migrates appear on chromatograms as forests of peaks (), with some corresponding to fully or partially structurally characterised chemicals while many others remain unidentified.
Figure 1. Illustrative examples of forests of peaks. Total ion chromatograms of extracts from FCMs are presented, along with a line (dashed) representing a 10 μg/6 dm2 standard (right-hand panel). Such profiles are typical from many different FCM extracts or migrates.
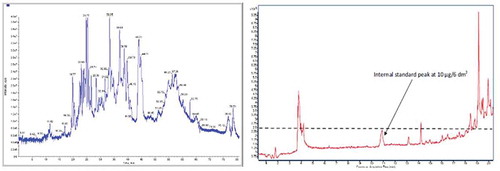
Analytical chemistry has allowed semi-quantitative information to be obtained rapidly on chemicals of either known or unknown structure using universal detection methods (e.g. flame ionisation detector, FID; charged aerosol detector, CAD) and internal standards. Taking into account the migration conditions and knowledge about intended application of the FCM in packaging food, analytical data can be converted into exposure estimates. The standard approach to address the potential significance to human health of such exposures is comparison with health-based information. For known, structurally characterised substances, this could be done using health-based guidance values (such as acceptable daily intakes (ADIs) or tolerable daily intakes (TDIs)) or, if these are not available or appropriate, by using specific regulatory toxicology data through the application of the margin of exposure approach. In cases where no, or very limited, toxicological information is available, the Threshold of Toxicological Concern (TTC) and/or computational toxicology may be applicable (Kroes et al. Citation2004; Schilter et al. Citation2014; EFSA CEP Panel Citation2016). The most challenging issue in the safety assessment of FCM migrates is dealing with unidentified, unknown NIAS. This is a particular concern for the ones that may exhibit mutagenic properties since no safe level may be expected.
Establishing level of safety concern of unidentified/unknown NIAS
An approach to establish the level of safety concern of unknown substances in complex food matrices has already been developed (Koster et al. Citation2011; Rennen et al. Citation2011) and its use in the frame of the safety assessment of FCMs (Koster et al. Citation2014, Citation2015a) and plant extracts (Koster et al. Citation2015b) has recently been described. Briefly, it is proposed to apply the TTC approach to prioritise unknowns (Barlow Citation2005). Application of the Cramer class III TTC would be appropriate if sufficient evidence is available that the chemicals under investigation do not belong to one of the following chemical categories (Kroes et al. Citation2004; EFSA CEP Panel Citation2016):
Compounds not adequately covered in the database used to derive the TTCs (e.g. inorganic chemicals, metals and organometallics, proteins, steroids, nanomaterials, radioactive substances and organosilicon compounds or chemicals that are known or predicted to accumulate)
High potency carcinogens (aflatoxin-like, azoxy-, N-nitroso-compounds, benzidines)
Chemicals with a structural alert for direct DNA-reactive genotoxicity
Acetylcholinesterase inhibitors (organophosphorus compounds, carbamates)
Obviously, for unknown substances, it is difficult to comply with the above criteria since most of them require prior knowledge of the chemical structure. However, several initiatives have proposed strategies to enable exclusion of such chemical classes even when in only partially characterised complex mixtures such as food matrices and FCM migrates containing unknown NIAS (Koster et al. Citation2011, Citation2014, Citation2015a). They include, amongst others, three key steps:
Exclusion dependent on sample sources (knowledge of the FCM chemistry and manufacturing).
Exclusion by sample preparation, chromatographic technique, and detection method.
Exclusion by targeted analysis.
The appropriate application of the three steps is considered to cover most of the chemical categories that need to be excluded before the Cramer class III TTC can be used. However, in addition to chemical considerations, the possibility of using in vitro bioassays has been proposed. Indeed, many chemicals to which the Cramer class III TTC should not be applied bear structural features triggering specific biological mechanisms and/or activities. These include steroids, dioxins, organophosphorus compounds and direct DNA reactive mutagenic carcinogens, which are associated, respectively, with activation of steroid nuclear receptors, activation of arylhydrocarbon receptor, acetylcholinesterase inhibition and gene mutation. Bioassays have been developed to determine all of these activities/mechanisms of action, such as for example the Ames test for DNA-reactive mutagens. The only real barrier to their use in this way is the limit of biological detection. Hence, the value of these types of tools to detect the presence, in uncharacterized mixtures of NIAS, of chemicals possessing alerts/activities incompatible with the application of the Cramer class III TTC was deemed worth considering.
Bioassays and the application of the Cramer class III TTC
Bioassays (also called biotests, biological tests) are defined in the Oxford dictionary as “measurement of the concentration or potency of a substance by its effect on living cells”. In toxicology, in vivo bioassays using laboratory animals have traditionally been the models of choice to identify points of departure for chemical risk assessment. In vitro bioassays specifically designed to determine genotoxic potential are also included in the standard range of tests required for chemical risk assessment. In addition, there is increasing effort to develop and apply in vitro methods for hazard identification and characterisation. For example, in applying the concept of the adverse outcome pathway (AOP), in vitro methods are expected to play important roles in the identification and characterisation of molecular initiating and key events driving the expression of toxicity. In this context, significant efforts are being made to design in vitro tests with the aim of covering endpoints and target organs/tissues most relevant to humans (Bal-Price et al. Citation2015). In addition, computational models fed with biochemical information on chemical fate and in vitro toxicological data are currently under development with the aim of extrapolating to oral in vivo intakes from concentrations in cell culture medium (Wambaugh et al. Citation2018). It is likely that, in the long term, this research will provide in vitro alternatives able to significantly replace experimental animals for hazard characterisation.
But in some situations, bioassays are not intended to provide data directly applicable to hazard characterisation. They were not designed with the aim of reflecting the in vivo situation but were developed to optimise their capability of detecting chemicals with specific bioactivities/properties (hazard identification or biodetection) (Schilter et al. Citation2018; Wambaugh et al. Citation2018). They can be considered analytical detectors based on biological, rather than physico-chemical, properties. Biodetection is valuable in a number of situations, for example when the activity of a chemical is more relevant than its actual quantity (e.g. in biopharmaceutical quality assurance). Bioassays have been shown to be valuable in screening complex mixtures of unknown composition (e.g. for waste and effluent management). They provide insight into the potential presence of toxicologically relevant chemicals in the mixture under investigation, enabling prioritisation of analytical resources to relevant substances in a cost-effective way. The present investigation focuses on the potential use of bioassays as biodetectors to support the application of the Cramer class III TTC for the evaluation of uncharacterized NIAS in food contact material extraction samples.
Numerous bioassays allowing the detection of a range of bioactivities are currently available. Most are based on different types of cells in culture and use various biological detection principles such as transcriptional activation and cell proliferation. With respect to supporting the use of the Cramer class III TTC, several of these bioassays may be of value in identifying chemicals to be excluded. For examples, bioassays based on activation of androgen and oestrogen receptors could be used to identify steroids (Brand et al. Citation2013), while bioassays measuring aryl hydrocarbon receptor activation already have a good record in the detection of dioxins in various matrices (Bovee et al. Citation1998; van der Burg et al. Citation2010a, Citation2010b; Eichbaum et al. Citation2014). Biochemical assays measuring acetylcholine esterase activity are also available and could be of use in identifying inhibitors such as organophosphorus compounds and carbamates (Tang et al. Citation2007). Use of such bioassays could theoretically be relevant in determining the suitability of the Cramer class III TTC in the assessment of NIAS in food-related mixtures that cannot be chemically completely characterised. This can be illustrated by the organophosphates and carbamates, which, if not excluded, would trigger the use of a TTC significantly more conservative than the one allocated to the Cramer class III. However, specifically regarding packaging safety, most of these bioassays would not be considered necessary since many of the restricted chemicals they detect would either be irrelevant for FCMs (e.g. steroids), or efficiently excluded through analytical chemistry e.g. GC-NDP (nitrogen-phosphorus detector) and/or LC-MS for organophosphates and/or carbamates (Koster et al. Citation2014). Genotoxic compounds may constitute the strongest case for the use of bioassay for their detection. Since they comprise a structurally heterogeneous group of chemicals with variable physico-chemical properties, an approach based on analytical chemistry alone, as described above, is unlikely to be practically applicable. Consequently, the present work was focused on the identification of direct DNA-reactive genotoxic chemicals in FCM migrates through the use of bioassays, and more specifically the Ames test since currently it constitutes the most suitable assay for that purpose.
Genotoxicity and direct DNA reactivity
Definitions
Genotoxicity is a broad term referring to the capability of chemicals to damage genetic material. Several molecular mechanisms resulting in genotoxicity have been described, for example direct covalent binding to DNA, DNA breakage, DNA cross-linking, DNA intercalation, oxidative stress, inhibition of topoisomerases and interference with DNA repair (EFSA Scientific Committee Citation2011; Dearfield et al. Citation2017). Mutagenicity has been defined as “the induction of permanent transmissible changes in the amount or structure of the genetic material of cells of organisms” (ECHA Citation2016). There are three basic types of mutagenicity, namely gene mutation, clastogenicity (alteration of chromosome structure) and aneuploidy (alteration of chromosome number). The genetic change is called mutation and the causal agent is referred to as a mutagen. Mutation is a sub-set of genotoxicity and therefore all mutagens are genotoxic, but not all genotoxic chemicals are mutagens. Mutations occurring in germ cells may give rise to heritable genetic defects while in somatic cells they may trigger neoplastic transformation leading to cancer. Hence, characterising the potential for mutagenicity is a high priority in the safety assessment of chemicals.
Understanding the mechanism of genotoxicity is of prime importance for the risk assessment of a chemical. Some of the known mechanisms of genotoxicity (e.g. oxidative stress, inhibition of topoisomerases) are considered to have a threshold for effect. For genotoxic carcinogens well documented to act through these types of mechanisms, a safe level of exposure can be established using standard uncertainty factors applied to a NOAEL/BMDL (No Observed Adverse Effect Level/Benchmark Dose Lower Confidence Limit) in experimental animal studies (COM Citation2010). If not tested experimentally for carcinogenicity, such substances could be assessed using Cramer-class-specific TTCs (Boobis et al. Citation2017). For chemicals or their metabolites known to induce mutations by directly modifying or reacting with DNA (direct DNA-reactive mutagens), a threshold for mutation induction (and resulting carcinogenicity) is not normally expected and therefore safe levels of exposure cannot be established with confidence. In terms of the TTC approach, direct DNA-reactive mutagens are not covered by any of the Cramer class TTCs and a much lower TTC value would have to be used (Kroes et al. Citation2004; Boobis et al. Citation2017). In consequence, to assume that unknown chemicals in a mixture belong to Cramer class III requires excluding the presence of direct DNA-reactive mutagens through actual testing of the mixture, as structural assessment is not possible. As highlighted in the next sections, the Ames test currently appears to be the most suitable assay for that purpose.
Genotoxicity testing
No single test can detect all of the key types of mutagenicity (gene mutation, clastogenicity, aneuploidy), and so a number of in vitro and in vivo bioassays have been developed to identify these different outcomes. For the specific purpose of the present work, in vitro test systems are considered the most appropriate. Validated internationally recognised procedures are available (e.g. OECD guidelines) for many of them. Additional tests are available with various degrees of validation (EFSA Scientific Committee Citation2011). Examples of tests capable of detecting direct DNA reactive mutagens are provided in .
Table 1. Standardised in vitro genotoxicity assays detecting direct DNA-reactive mutagens.
To thoroughly characterise all aspects of the genotoxic potential of chemicals, several bioassays covering the basic types of DNA-damage/mechanisms of action should be performed (Muller et al. Citation2003; Thybaud et al. Citation2007a, Citation2007b) using conditions where both parent compound and possible metabolites can be assessed. For regulatory purposes, it is usual to conduct a range of tests in a stepwise approach. This starts with in vitro bioassays followed, if required, by in vivo tests. Different combinations of assays have been investigated with regard to their reliability in predicting in vivo genotoxicity. A detailed analysis of published in vitro data for 557 rodent carcinogens and 405 in vivo genotoxins was undertaken by Kirkland et al. (Citation2011) (Kirkland et al. Citation2011). It was concluded that a set of two in vitro tests consisting of Ames test (OECD 471Footnote1, for gene mutation) and mammalian micronucleus assay (MNvit, for clastogenicity and aneuploidy) (OECD 487Footnote2) would be sufficient to detect reliably rodent genotoxic carcinogens and in vivo genotoxicants. This combination of bioassays as an initial core battery has been endorsed by several authors and organisations (Silano et al. Citation2008; EFSA Scientific Committee Citation2011; Kirkland et al. Citation2011; Luijten et al. Citation2016).
In its recommendations on genotoxicity testing, the European Food Safety Authority (EFSA) advises application of the following strategy:
A basic set of core in vitro tests (Ames and MNvit).
Clear negative results in both assays are considered indicative of absence of genotoxicity potential with sufficient certainty. No further testing would be required.
In the case of inconclusive, contradictory or equivocal results, further in vitro studies can be performed to clarify the genotoxic potential in vitro. Other tests may provide useful information at this stage.
In the case of positive results from in vitro tests, a review of all the available relevant data on the test substance should be undertaken to ensure the conduct of an appropriate in vivo study (or studies) to assess whether the genotoxic potential observed in vitro is expressed in vivo.
As mentioned earlier, the Cramer class III TTC is applicable to chemicals, which amongst other criteria, are not direct DNA-reactive mutagens. This infers that clastogens and aneugens, chemicals which are genotoxic via a mechanism affecting chromosomes, do not need to be identified. Clastogens, generally, require higher concentrations to generate positive responses in vitro than DNA reactive mutagens (Kirkland et al. Citation2016). As shown in , a number of validated tests to assess the induction of gene mutation are available. However, only the Ames test continues to be part of the “core” set of in vitro tests (the mammalian cell gene mutation tests are often used in a “supplementary” role), and therefore the Ames test appears the bioassay of choice to identify direct DNA-reacting mutagens. As a precedent to this investigative model, ICH Guideline M7 (R1) on impurities in pharmaceuticals (ICH Citation2017) utilise a TTC-based approach. This Guideline focuses on DNA reactive substances that have a potential to directly cause DNA damage when present at low levels leading to mutations and therefore, potentially causing cancer. This type of mutagenic carcinogen is usually detected in a bacterial reverse mutation (mutagenicity) assay. Other types of genotoxicants that are non-mutagenic (clastogens and aneugens) typically have threshold mechanisms and are considered not to pose a carcinogenic risk in humans at the levels present as impurities. Therefore, an impurity negative in an appropriately conducted bacterial mutagenicity assay would be considered to be non-mutagenic, and no further genotoxicity assessments are recommended. Furthermore, ECHA Guidance for REACH testing of Annexe VII chemicals (1 to 10 tonnes per year) requires only a bacterial gene mutation test to satisfy requirements for genotoxicity testing (ECHA Citation2017). In their review of the TTC approach, EFSA and WHO concluded that if there are no structural alerts or chemical-specific genotoxicity data, such as Ames test results, indicating that the chemical has the potential to be a DNA-reactive carcinogen, based on the weight of evidence, TTC considerations for non-genotoxic compounds would apply (EFSA and WHO Citation2016).
Genotoxicity testing of mixtures
Genotoxicity bioassays, their standardised protocols (i.e. OECD 4713 and 4874) and the testing strategy were developed for pure substances and there is only limited scientific literature and very few guidelines discussing their suitability to address the genotoxic potential of complex mixtures. In this context, a mixture is defined as complex if it contains, besides chemically identified substances, a substantial fraction of unidentified compounds (Hardy et al. Citation2017). Although there is no formal acceptance of their adequacy for such purpose, the use of in vitro genotoxicity assays and testing strategies have been explored and recommended in a number of applications to address the safety of mixtures containing compounds with potentially unknown or not fully elucidated structures.
Environmental samples
Adapted protocols of standardised genotoxicity tests have been widely used for three decades to assess environmental compartments or ecosystems (geno-ecotoxicology as cited in Eisenbrand et al. Citation2002). As examples, Claxton et al. (Citation2004) and Elassouli et al. (Citation2007) reported results on air samples; White and Claxton (Citation2004) and Ohe et al. (Citation2004) reviewed the assessment of soil and water samples, respectively. Recently, Escher et al. (Citation2015) and Jia et al. (Citation2015) evaluated a large number of in vitro bioassays (up to 36) for water quality assessment and Escher et al. (Citation2015) proposed water quality guideline values based on the results of in vitro bioassays.
Medical devices
Similar to FCMs, the safety assessment of medical devices is a field where mixtures of substances transferred from the material into the product (or into the human recipient) have to be assessed. General safety requirements are similar to those applying to FCMs: medical devices shall not compromise the clinical condition or the health of patients, or the safety and health of users (Council Directive 93/42/CEEFootnote3). Biological evaluation is one part of the overall evaluation of medical devices supporting their safety in use (ISO 10993–1:2009Footnote4). It includes first a characterisation and quantification of material constituents as well as of extractables and leachables, and secondly considerations and assessment of toxicological endpoints including genotoxicity. As regard to genotoxicity endpoints, when the need to evaluate potential genotoxicity is established, a range of in vitro tests should be conducted to determine any gene mutations, changes in chromosome structure and number, and other DNA and gene toxicities caused by medical devices, materials, and/or their extracts (ISO 10993–1:2009). Tests should be performed on solutions, suspensions or extracts of the final device, unless otherwise justified (ISO 10993–3:2014Footnote5). The in vitro tests requested by ISO or recommended by the Centre for Devices and Radiological Health (CDRH) include a mutagenicity test in bacteria (OECD 4713) and either an in vitro mammalian chromosome aberration test (OECD 473Footnote6), or an in vitro mouse lymphoma tk assay (OECD 490Footnote7) or an in vitro mammalian cell micronucleus assay (OECD 4874) (ISO 10993–3:2014; FDA Citation2016). If the results of both in vitro tests are negative, further genotoxicity testing in animals is unnecessary. In the case of positive results in at least one of the in vitro tests, the ISO standard proposes a step-wise procedure to determine follow up with in vitro and/or in vivo tests (ISO 10993–3:2014). This includes consideration of confounding factors and elucidation of mechanism of action. In the case of a positive result in at least one assay, CDRH also recommends performing further investigation to identify the source of the genotoxicant; however “an in vivo genotoxicity assay is not recommended as a follow-up to rule out a positive in an in vitro assay because the amount of the chemicals in a device extract may be below the limit of detection of the in vivo assay” (FDA Citation2016). In the case of an equivocal result in an in vitro test, repetition of the same assay is recommended (FDA Citation2016).
Impurities in pharmaceuticals
Pharmaceuticals can be complex mixtures that contain not only active substances and excipients but also a number of known (e.g. starting materials) and unknown (e.g. byproducts of synthesis) impurities. The International Committee on Harmonisation (ICH) published the M7 guideline on the identification and control of mutagenic impurities in pharmaceuticals. The guideline takes into account that expected exposure to impurities is generally low. Therefore, the assessment focuses on direct DNA-reactive substances, which can already be of health concern at low concentrations. Genotoxic effects by other mechanisms (aneugens, clastogens) are considered of less importance as they typically exhibit threshold mechanisms for effect and if present as impurities at low levels, would not be expected to pose a carcinogenic risk in humans. Accordingly, impurities at low concentrations do not have to be evaluated for clastogenicity and aneugenicity. Consequently, it is stated that to assess the mutagenic potential of impurities, a single bacterial mutagenicity assay or an assessment of Structure–Activity Relationships (SARs) that focuses on bacterial mutagenicity predictions should be performed. The guideline gives no recommendations for testing of the whole mixture; however, it takes into account that for some impurities, isolation or synthesis might not be possible. In this case, bacterial mutagenicity testing could be carried out using a miniaturised assay format instead of the full OECD format to enable testing at higher concentrations. Although not specifically intended for this purpose, it is mentioned that the principles outlined in the guideline could also be applied to leachables of pharmaceutical packaging (ICH Citation2017).
Botanicals and botanical preparations
These types of materials are further examples of products that may be complex mixtures of unidentified substances requiring genotoxicity assessment. In its guidance (EFSA Citation2009), EFSA requires that tests performed cover genotoxic effects both at gene and chromosome levels referring to future guidance on genotoxicity testing for further details (European Food Safety Authority Scientific Committee Citation2011). Tests should be conducted according to internationally agreed protocols such as those described in OECD testing guidelines. The core set includes Ames test and an in vitro mammalian chromosome aberration test or an in vitro micronucleus assay; in addition, a mouse lymphoma tk assay may be needed. Deviation from this core set is possible if scientifically justified. Follow up of a positive in vitro test by an in vivo test is mentioned; however, attention should be paid to genotoxicant detection limits among other variables. Similarly, a guideline exists for the genotoxicity assessment of herbal medicines (EMEA/HPMC/107079/2007Footnote8). The testing strategy includes the Ames test and a decision tree for positive and negative results, including investigations based on in vivo approaches.
Novel foods
In vitro genotoxicity testing is also required as a basic component of hazard identification (EFSA Citation2016) of novel foods, most of them being complex, not fully characterised mixtures. In its guidance on novel foods (EFSA Citation2016), the EFSA Panel on Nutrition, Novel Foods and Food Allergens (NDA) refers to the EFSA guidance on genotoxicity testing (European Food Safety Authority Scientific Committee Citation2011), stressing that the approach “in principle applies to novel food”. However, the Panel recognises that for some complex mixtures “it may be necessary to focus on specific constituents of the novel food” and therefore deviations can be argued on a case-by-case basis.
European food safety authority opinion
The EFSA Scientific Committee recently reviewed methodologies regarding safety assessment of chemical mixtures. In this context, an approach for genotoxicity assessment has recently been developed (More et al. Citation2019). In brief, the first step of the approach involves to a thorough chemical characterisation of the mixture under investigation. For mixtures containing a substantial fraction of unidentified components, it is then recommended to first assess the genotoxic potential of the substances that can be chemically identified. If no genotoxic chemicals are found, then the unidentified fraction should be evaluated by testing either the fraction itself or, if not possible, the whole mixture. The possibility of fractionating the test material should be considered on a case by case basis, e.g. to remove inert, toxicologically irrelevant materials (e.g. polymers) and/or highly toxic chemicals which could interfere with the results of the test. The testing strategy for the whole mixture/fraction should follow standard guidelines as available for individual chemicals (Ames test and in vitro micronucleus assay; European Food Safety Authority Scientific Committee Citation2011). The top concentration may need to be greater than 5 mg/ml if cytotoxicity is absent. If the tests are clearly negative (no activity), the mixture is considered non-genotoxic. In the case of positive results, the significance of the data for risk assessment needs to be evaluated based on mechanistic, in vitro and possibly in vivo investigations.
Considerations on limit of detection (LOD)
General aspects and definitions
According to EFSA (More et al. Citation2019), the conclusion that a chemical mixture does not raise any genotoxic concern can be drawn from analytical and bioassay data indicating with confidence a low probability of the presence of individual genotoxic substances in the mixture investigated. The uncertainty associated with the conclusion is strongly dependent upon the detection capability of the tools applied, in other words on the limits of detection (LODs) of genotoxic chemicals by the methods used.
The LOD is a concept routinely applied in analytical chemistry. It is defined as the lowest concentration of an analyte in a sample that the analytical process can reliably detect, meaning that the signal of the analyte is statistically different from that of a blank. The term sensitivity is used together with LOD to describe the performance of an analytical method. A highly sensitive method refers to a method capable of detecting low levels of a chemical (sensitivity and LOD are inversely related) (Ekins and Edwards Citation1997). Although less frequently applied, a similar LOD concept has also been developed to characterise the performance of bioassay-based methods when used for biodetection purposes (Eichbaum et al. Citation2014; Schriks et al. Citation2015). In this context, it should be noted that the term sensitivity is a source of confusion. Indeed, in the bioassay field, sensitivity has a very different meaning than in analytical chemistry. For example, for a genotoxicity test, it is a performance indicator calculated as the ratio of the number of genotoxic substances that are correctly identified as genotoxic (true positives) and the sum of the correctly identified genotoxic substances and the genotoxic chemicals not identified (false negatives). It does not provide any insight into the limit of analytical detection at which a genotoxic substance can be reliably measured. With respect to FCM safety assessment, genotoxicity bioassays should be sensitive in both biological (detect the positives) and chemical (detect low level) senses. To avoid confusions and provide clarity, the term “sensitivity” will be avoided in the present manuscript. In addition, when related to a bioassay, LOD will be termed LOBD (limit of biological detection), while when related to analytical chemistry, LOD will be termed LOCD (limit of chemical detection) (see ).
Box 1. Terminology associated with the concept of limit of detection (LOD) as used in the present manuscript.
LOBDs for direct DNA-reactive mutagens in genotoxicity tests
Little information is available on LOBDs of genotoxicity tests. These bioassays were developed mainly for classification of pure substances, which are normally limited in concentration only by their solubility in the assay medium and their toxicity to cells. Assays are generally considered to be qualitative and not quantitative. In this context, the LOD did not have much relevance and therefore was not a major issue in assay development. However, in some specific situations, the question of the LOD has been raised and investigated.
For example, for pre-screening of pharmaceutical candidates available only in very small amounts, a number of different miniaturised Ames tests with lower sample requirement have been developed and comparisons of their performance with that of the standard Ames, using representative mutagens, have been undertaken (Escobar et al. Citation2013). The lowest effective concentration (LEC), defined as the lowest concentration that gave a positive response in the test, has been used to determine the LOBD. For example, in a systematic comparison of dilution curves from a wide variety of different mutagens, it was found that the Micro-Ames (µAmes) test, a 24-well plate version of the assay, and the standard Ames assay, provided approximately equivalent LECs, when experimental variations were taken into account (Proudlock and Evans Citation2016). The µAmes 24-well test requires 20-fold less test material than the standard assay. Importantly, in both tests, the pre-incubation method (20–60-min pre-incubation of test material with the bacterial suspension prior to plating) was associated with lower LECs as compared to the plate incorporation method (exposure of cells to material directly in the plate) (Proudlock and Evans Citation2016). This confirms previous findings (Escobar et al. Citation2013). A version of the µAmes assay with 96-well plates has also been developed and used in the early development of new pharmaceuticals (Escobar et al. Citation2013). It employs the 5 standard Salmonella tester strains.
Other miniaturisation approaches are based on a liquid format. The microplate fluctuation Ames test protocol (MPF) reduces sample requirement significantly. For a set of equivocal or weakly positive test compounds, it has been shown that the Ames MPF has equivalent or lower LECs than the standard Ames test (Fluckiger-Isler and Kamber Citation2012). However, overall, there are insufficient data available in the literature to conclude generally on differences in the LECs compared with those with the standard Ames test.
The issue of LOBDs has also been addressed in the fields of environmental and biological monitoring, where complex mixtures (e.g. water, air, urine) are analysed and the need for low LODs is well recognised (Wegrzyn and Czyz Citation2003). Variations of the Ames test were developed with the aim of obtaining more fit for purpose LOBDs for genotoxicants (e.g. Kado et al. Citation1983; McDaniels et al. Citation1990; Azuma et al. Citation1997; Takagi et al. Citation2011). Efforts have focused on modifying test conditions (e.g. S9 treatment, bacterial concentration, incubation temperature) as well as bacterial tester strains (Kado et al. Citation1983; McDaniels et al. Citation1990; Azuma et al. Citation1997; Takagi et al. Citation2011). A modified liquid-preincubation protocol, which uses higher concentrations of bacterial cultures and less liver homogenate, was developed and is referred to as a “microsuspension assay” (Kado et al. Citation1983), which has been applied extensively for testing biological and environmental samples. Lower LECs as compared to those of the standard Ames assay protocol have been reported for this variant of the Ames test when testing urine samples from cigarette smokers and four different mutagenic substances (Kado et al. Citation1983; Watanabe et al. Citation1995). Further studies report an enhanced response at lower concentrations for variations with an increased bacterial concentration, but did not directly evaluate the effect on the LECs (Agurell and Stensman Citation1992; Watanabe et al. Citation1995). Thus, modifications with higher bacterial concentrations seem to provide a promising approach to achieve more fit for purpose LOBDs, but the actual extent of the improvement is not clear and is likely to depend upon class of chemical and bacterial strains used. A miniaturised microsuspension assay in agar microplates has been recently developed and shown to exhibit similar performance and LECs as with the original format of the microsuspension test (Zwarg et al. Citation2018). A comparison between the microsuspension assay and microplate fluctuation method revealed similar LECs (Zwarg et al. Citation2018).
There is even less information on LOBDs for DNA-reactive genotoxicants in mammalian-based in vitro gene mutation assays. Information on LECs for tests using the Hprt and Xprt genes (OECD TG476Footnote9) could be found (Clive et al. Citation1979; Oberly et al. Citation1984). Lowest effective concentrations for DNA-reactive substances were such that the LOBDs were higher than the ones obtained with the Ames test (see for LOBD calculations). This holds true for newer genotoxicity tests in mammalian cells as well, such as the Greenscreen and the BlueScreen assays (data not shown, Pinter et al. in preparation).
Table 2. Calculated limits of biological detection (cLOBDs) for selected genotoxic chemicals in Ames plate incorporation test as compared to required target LOBDs (tLOBD) based on carcinogenic potency (TD50). For comparison, data derived from mammalian MLA hprp test are also provided.
Calculated LOBDs (cLOBDs) in FCM migrates
As mentioned above, LECs are available for a number of chemicals. Because LECs provide quantitative information on the limit at which a genotoxicity bioassay can detect genotoxic chemicals, they can be used to obtain insight on what the LOBDs (called calculated LOBDs, cLOBDs, in this paper) would be for genotoxic chemicals in FCM migrates, once the practicalities of the method have been taken into account (e.g. concentration, dilution). In the following sections, Ames test cLOBDs for selected representative genotoxic compounds are discussed from the perspective of the necessary LOBD (called target LOBD, tLOBD) for application of the TTC approach in the assessment of FCM migrates (see , ).
Figure 2. Comparison of calculated LOBD and target LOBD values for the Ames test in mg L-1 (simulant) for several mutagenic substances. The values are compared to a threshold of 0.01 mg L-1, corresponding to the current pragmatic management approach applied to prioritize unknown chemicals. The ratios between cLOBD and tLOBD are indicated on the top of respective bars.
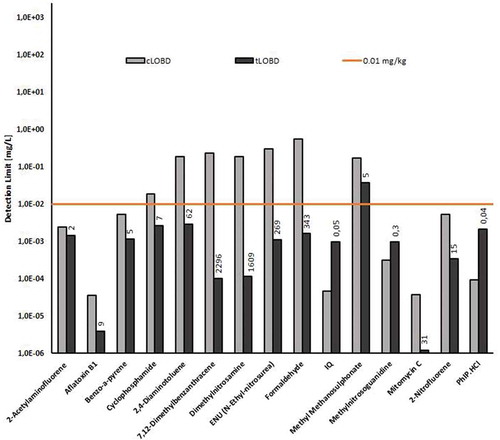
Rainer et al. (Rainer et al. Citation2018) developed an approach based on LECs in a standard Ames test to estimate cLOBDs in FCM migrates. This takes into account the concentration and dilution steps of the FCM migrate sample processing (Rainer et al. Citation2018). Briefly, to be tested in an Ames test, FCM migrates have first to be concentrated and then transferred into a bacteria-compatible solvent, in general DMSO, leading to a dilution of the sample. In the Rainer et al. paper (Rainer et al. Citation2018), a concentration factor of 1000 was considered and then a subsequent dilution factor of 25 applied (for a maximum tolerated DMSO concentration of 4%). This translates into an overall concentration factor of the migrate of 40. The cLOBDs are then obtained by dividing the LECs expressed in mass/L by a factor of 40. This overall factor of 40 is considered achievable in practice. Although the 1000 concentration factor may constitute a best case scenario, a dilution factor of 25 may be considered conservative since significantly higher DMSO concentrations (up to 14%) have been successfully used in the Ames test (Hakura et al. Citation1993). Examples of calculations are provided in using DNA-reactive genotoxic chemicals of diverse potency. It is acknowledged that relatively large variations may be observed in LECs generated in different studies. Based on the information available, it can be difficult to compare LEC values from different reports and it is not simple to understand how LEC values from different reports could be used. To obtain a rough approximation of how cLOBDs would relate to safety or other benchmarks, the analysis was limited to a few mutagens for which LECs were readily available. This study provides a wide range of LECs obtained in the standard Ames test (). When data were available, the same approach was applied to calculate cLOBDs based on the mammalian MLA hprt test. In this case, the DMSO concentration considered was the highest tolerated by mammalian cells (1%). This translated into a final concentration factor of 10 to be applied to LECs in mammalian cells in order to obtain cLOBDs. It has to be kept in mind that cLOBDs represent a best-case estimate because not only are the LECs employed low, but also because it is assumed that a 1000-fold concentrated migrate is transferred in DMSO without losses and that the matrix does not affect the bioassay results.
Interpretation of cLOBDs
Since genotoxic potencies/dose-responses of different direct DNA-reactive substances span several orders of magnitude, cLOBDs will vary accordingly, as illustrated in . It is therefore not possible to define a general cLOBD for genotoxicity bioassays such as the Ames test. cLOBDs always refer to specific substances.
There are several ways to interpret cLOBDs. First, they can be benchmarked against the TTC threshold of 0.0025 μg/kgbw/day (or 0.15 µg/60kg person) used to prioritise chemicals with a structural alert for genotoxicity (Kroes et al. Citation2004; EFSA Citation2016). Considering the exposure scenario currently used for assessing substances in plastic food contact materials, i.e. an adult of 60 kg bw consuming daily up to 1 kg of food in contact with material containing the substance (Silano et al. Citation2008), this TTC translates into a target generic LOBD of 0.00015 mg/L (0.15 µg in 1 L of migrate, see ), which can be compared with the cLOBDs of . With the exception of 3 substances, none of the calculated cLOBDs would be suitable to detect these genotoxins at this TTC-derived LOD.
It should be noted that the application of the TTC for chemicals that are potentially DNA-reactive mutagens results in conservative assessments (EFSA and WHO Citation2016). The establishment of this TTC value took into account data on potent genotoxic carcinogens to which a non-threshold, linear dose-response analysis was applied with a target lifetime cancer risk excess in exposed individuals of not greater than 1 × 10−6. It is important to note that EFSA determines the level of concern for direct DNA-reactive carcinogens from the margin of exposure (MoE) between the benchmark dose lower confidence limit for a 10% carcinogenic response (BMDL10) in in vivo carcinogenicity bioassays and exposure (Kenyon et al. Citation2007; EFSA Citation2012). Carcinogens associated with a MoE of at least 10000 are considered to be of low priority for action. Assuming a linear dose-response, a 10000 MoE over BMDL10 would correspond roughly to an excess risk of 1 × 10−5 (Boobis et al. Citation2017). This means that assessing a potential genotoxic substance using a TTC of 0.0025 μg/kg bw/day is more conservative than the approach applied for substantiated genotoxic substances.
Since genotoxic carcinogens can differ appreciably in their potency, the suitability of the Ames test cLOBDs to detect them at a defined level of risk was evaluated. This exercise was conducted for a few illustrative examples of potent genotoxic carcinogens, for which appropriate data were available (, ). Ratios between cLOBDs and target LOBDs (tLOBDs) as required to limit excess risk to 1 × 10−5 are provided in . This approach is very limited since it is not certain that the LECs used were actually the lowest available (significant variability is observed). In addition, the food intake scenario assumed in the calculation of the LOBDs (1 kg over lifetime) may be considered as relatively conservative and does not account for any consumption factors (Duffy et al. Citation2006), or possible exposure durations shorter than lifetime. Calculated cLOBDs/tLOBDs ratios were highly variable (, ), reflecting the known relatively weak correlation between mutagenic and carcinogenic potencies (McCann et al. Citation1988). All ratios except three were found to be >1 indicating than most of the cLOBDs are not adequate to detect a mutagen level corresponding to 1 in 105 excess cancer risk. It is difficult to generalise the conclusion drawn from this very limited dataset of mutagenic carcinogens and more research is necessary.
The analysis described above was on a group of relatively potent DNA-reactive genotoxicants and hence illustrates that for most known direct DNA-reactive mutagens, the LOBDs of the standard Ames test would most certainly be inadequate to detect levels in FCM migrates that would be compatible with an assurance of safety. In this context, it is important to note that the European regulation (European Commission Citation2011) on plastic states that provided that they are not carcinogenic, mutagenic or reprotoxic, non-authorised substances behind a barrier in a multilayer material may be used, but are not allowed to migrate into food. The minimum requirement for the analytical limit of detection is 0.01 mg/kg of food (or 0.01 mg/L of simulant). Similarly, for some authorised FCM substances, an SML of ‘not detectable’ at a detection limit of 0.01 mg/kg food is applied. Likewise, and in absence of regulation, the application of such a generic level of 0.01 mg/kg food (or L of simulant) has been extended, in particular within the industry, to unknown substances migrating into food. In practice, this 0.01 mg/L (10 ppb) limit is widely used as a pragmatic risk management cut-off level to prioritise unknown chemicals on which further determination work should be focused. To detect direct DNA-reactive mutagens at a level of <0.01 mg/L of simulant would provide additional safety assurance as compared to the current situation.
Data in / suggest that for 8/15 of the mutagenic substances considered, LOBDs could potentially be sufficient to detect levels <0.01 mg/L. Rainer et al. (Rainer et al. Citation2018) observed that 8 out of 16 direct DNA-reactive substances retrieved from the ECVAM genotoxin positive list (Kirkland et al. Citation2016) could potentially be detected at a level of 0.01 mg/L. Kenyon et al. (Kenyon et al. Citation2007) evaluated approximately 450 mutagens. Eighty five percent could be detected in the Ames test at 250 µg/plate (Kenyon et al. Citation2007) while only 22 percent could be detected at a level of 2.5 µg/plate corresponding to a cLOBD of about 0.02 mg/L. In addition, the Ames cLOBDs of 38 genotoxins that were identified in FCMs (Rainer et al. Citation2018) were calculated. Five (13%) substances could potentially be detected at the level of 0.01 mg/L.
In , calculated cLOBDs from a mammalian gene mutation assay are also presented and show that consistently higher values than the ones derived from the Ames test are obtained. This illustrates that mammalian tests are less sensitive than the Ames assay at detecting low levels of mutagens and consequently less suitable to detect such chemicals at adequate levels in complex mixtures.
In summary, the available data to estimate the suitability of the standard Ames test LOBDs for detecting direct DNA-reactive mutagens in complex mixtures such as FCM migrates are limited. They tend to indicate that in general, the standard Ames test is not entirely adequate to detect levels compatible with safety. The situation may be more favourable with the application of other formats of the Ames test such as the microsuspension based assay. However, data are still too scarce and limited to make any definitive statement. Indeed, more research is needed to address the question of LOBDs of DNA reactive mutagens in bioassays. Interestingly, for the most potent genotoxic carcinogens studied, even the standard Ames test may be capable of detecting levels lower than 0.01 mg/L of migrate (<10 ppb). This suggests that testing FCM migrates may provide additional safety assurance as compared to current practices and have a role in deciding on the use of the Cramer class-III TTC for unknown chemicals in FCM migrates.
Genotoxicity testing of FCM samples: currently available data
In vitro genotoxicity studies have been conducted on various types of FCM samples. Groh et al. (Groh and Muncke Citation2017) and Severin et al. (Severin et al. Citation2017) published comprehensive reviews regarding the use of bioassays for the assessment of FCMs including an overview of sample preparation and biological methods used for genotoxicity evaluation.
In general, genotoxicity testing of FCMs consisted of a sample preparation step prior to the execution of the bioassay. As food itself is not a suitable matrix for such analysis, samples were usually prepared by directly extracting the FCM with solvents or by conducting migration studies in food simulants under defined time and temperature conditions. Before testing, the extracts or migrates were in general concentrated through evaporation or solid phase extraction techniques and then transferred into a bioassay compatible solvent, most often DMSO or ethanol.
Sample preparation
For plastics, a number of extraction techniques have been applied, depending on the type of plastic and classes of substances to be investigated. They have included conventional extraction, microwave-assisted extraction, dissolution and precipitation techniques (Subramanian Citation2013; McCombie et al. Citation2016). However, there are no generally agreed standards for total extraction of plastics. Conditions for migration tests are listed in the European Commission Regulation (EU) No 10/2011 (European Commission Citation2011). In addition, technical guidelines to support Regulation (EU) No 10/2011 (European Commission Citation2011) have been drafted by the JRC – Joint Research Centre.
For non-harmonised Food Contact Materials and Articles (FCM&As) migration guidelines have been issued by some European Member States, or are pending. Several of the professional associations have published recommendations for their materials on their web sites.
For paper and board, there are two CEN-standards for extraction methods and two migration methods: EN 645:1993 (Cold-water extractables) and EN 647:1993 (Hot-water extractables), EN 14338:2003 (Conditions for determination of migration from paper and board using (MPPO) as a simulant) and EN 15519:2007 (Preparation of an organic solvent extract). Sample preparation for in vitro testing of paper and board was optimised as part of the “BIOSAFEPAPER” project (Bradley et al. Citation2008; Honkalampi-Hamalainen et al. Citation2010). Whilst methods for cytotoxicity testing have been included in accepted European standards (EN15845 and EN 16418), the methods for genotoxicity testing, that were developed/used in this project, have not yet been included in official guidelines.
Overall, sample preparation has been recognised as a challenging step, prone to systematic errors (Souza-Silva et al. Citation2015) such as loss of volatile chemicals. In addition, the potential for sample contamination from the laboratory environment has been recognised as being critical. Overall, there are no official and harmonised methods to prepare FCM samples for bioassay testing. This is different than for medical devices, which often consist of materials that are comparable to FCM (e.g. plastics and coatings), where extraction at exaggerated conditions is recommended (ISO 10993–3 2014). For FCMs it has been generally considered that total extraction can lead to much higher concentrations of target compounds than migration into foodstuffs (Begley et al. Citation2005; Franz and Welle Citation2008) and/or may result in the evaluation of substances that do not migrate at all. Migration experiments have been considered to provide more realistic estimates of migrating substances, although examples of over-estimated migration rate have been reported (Begley et al. Citation2005; Seiler et al. Citation2014; Driffield et al. Citation2018). The question on the relevance of extraction versus migration data to reflect actual migration in food is still debated and is likely to depend upon the material versus application considered.
In vitro testing of FCMs for DNA reactivity (Ames assay data)
The Ames test has been used by several authors on a wide-variety of FCM migrates or extracts either alone or in combination with other in vitro genotoxicity bioassays, providing some positive but mainly negative results as illustrated in two recent reviews by Severin et al. and Groh et al. (Groh and Muncke Citation2017; Severin et al. Citation2017) and summarised in .
Table 3. Ames test performed on FCM migrates and/or extracts. Table expanded and revised from Severin et al. (Citation2017) and Groh et al. (Citation2017).
In the study of Bradley et al. (Bradley et al. Citation2008), 19 food contact paper and board extracts (hot or cold water, ethanol or Tenax extraction) were tested for genotoxicity. The chosen tests were the standard Ames test in strains TA98, TA100, TA1535 and TA97 in the presence and absence of S9 and an in vitro comet assay in HepG2 cells. None of the extracts induced any positive response in either assay. Additionally, an ethanol extract from a paperboard not intended for food contact was shown to be mutagenic in the Ames test. Further investigation on this sample was performed to examine the role of identified substances from the sample in eliciting the mutagenic response (Honkalampi-Hamalainen et al. Citation2010). None of the substances identified in the extract could explain the mutagenic response and the reason for the observed mutagenicity remained unresolved. The authors concluded that in vitro bioassays could play a role in the safety assessment of FCM by identifying biological activities that are not predicted by chemical analysis alone.
In another study on paper and board, virgin and recycled paper extracts did not induce mutagenic activity in the Ames test with and without metabolic activation (Binderup et al. Citation2002). Two paper samples (with highest recycled fibre content) gave equivocal results in the first experiments; however, the weak activity could not be replicated using up to three times higher concentrations of the extracts in a second assay.
Several studies have investigated the mutagenic potential of plastic FCM extracts and migrates, most of them looking at water stored in PET bottles with and without exposure to sunlight. De Fusco et al. (De Fusco et al. Citation1990), Ubomba-Jaswa et al. (Ubomba-Jaswa et al. Citation2010), and Moraa (Citation2015) observed mutagenic activity in the Ames test after 1-month or 2-month storage, respectively, but not for longer storage times (3 months or longer). De Fusco et al. (De Fusco et al. Citation1990) and Ubomba-Jaswa et al. (Ubomba-Jaswa et al. Citation2010) observed higher mutagenic activity in samples exposed to sunlight compared to samples stored in the dark. Ubomba-Jaswa et al. (Ubomba-Jaswa et al. Citation2010) did not observe mutagenic activity either after 1-month storage or in daily-refill samples. Also, no activity was reported by Moraa (Moraa Citation2015) for samples stored for 15 and 30 days.
In a study similar to De Fusco et al. (De Fusco et al. Citation1990), Monarca et al. (Monarca et al. Citation1994) did not observe any mutagenicity in the Ames test for water samples stored for 1, 3 and 6-months in PET bottles. Bach et al. (Bach et al. Citation2013, Citation2014) did not observe mutagenic activity in water stored in PET bottles for 10 days at 60°C or exposed to sunlight.
Nakai et al. (Nakai et al. Citation2014) investigated the genotoxicity of styrene oligomers extracted from polystyrene with acetone using the Ames test with S. typhimurium TA100, TA1535, TA98, and TA1537, and E. coli WP2uvrA. No mutagenic activity was observed in the presence or absence of metabolic activation.
As part of the MIGRESIVE project, extracts from six laminates were tested in the Ames test, and none of them induced any mutagenic activity (Störmer and Franz Citation2009). Extracts from coating samples did not induce any mutagenicity in the Ames test as reported by Mittag et al. (Mittag et al. Citation2006) and Severin et al. (Severin et al. Citation2016).
The studies currently available were conducted in the context of genotoxicity assessment of migrates. Overall, very little was found on LOBDs and on the use of genotoxicity data to support the application of the Cramer class-III TTC for prioritising unknown NIAS. Interestingly, only a few samples were actually found to be positive in the Ames test. This might be because of the relatively high LOBDs for DNA-reactive mutagens in the Ames test. Alternatively, these data might reflect a low frequency of strong DNA-reactive mutagens amongst the NIAS migrating from FCMs. This possibility is supported by a recent EFSA analysis on FCM substances for which genotoxicity data were available (Bolognesi et al. Citation2017). Only 6 chemicals out of 214 substances were found to be positive in the bacterial reverse mutation test.
Good practices and way forward
As summarised above there are currently only a few data available on the assessment of FCM extracts/migrates for direct DNA-reactivity. The methods and experimental conditions vary significantly among the studies and are often not appropriately described. They are of very limited value in assessing the use of the Cramer class III TTC. In this situation, it is acknowledged that developing a harmonised approach accepted by all relevant stakeholders is an urgent need. However, because of the limitation of the information and experience available, proposing clear guidance is still a difficult task requiring more research. The following sections provide some recommendations, best practices and points to consider in the frame of testing FCM material for gene mutation activity, with the ultimate goal of supporting the use of the TTC approach for prioritisation of unknown NIAS in FCM migrates.
Recommendations for sample preparation
In order to run mutagenicity bioassays, it is necessary to first prepare a representative sample for testing. The aim of the sample preparation step is to produce a representative sample of substances from a food contact material, which could migrate into food. The extract prepared needs to be in a solvent suitable for application in bioassays. An overview of the proposed sample preparation is provided in .
Dialogue is advisable between those involved in the supply chain to decide upon the extraction/migration approach to be used. This may be FCM dependent. A control such as a procedural blank should be analysed in parallel, wherever possible. This can significantly facilitate the interpretation of the data, such as to link chemical and DNA reactivity data.
It is essential that any material investigated is representative. This requires understanding the variability and consistency within and across batches of the FCM, and between R&D and commercial products.
Determining the number of independent batches to be tested will depend upon analytical data and possibly preliminary information obtained with bioassays. For a thorough evaluation, it may be necessary to test more than one independent batch of the finished material. This could arise if GMP is not yet established or if it is a research sample, then multiple batches could be tested. If GMP is in place but there has been a process or ingredient change then more than one batch could be tested. If GMP is assured and/or chemical analysis demonstrates that the extracts from different batches are similar then a single batch may be tested.
It is essential to prevent the risk of adventitious external contamination during manufacturing, shipping and processing of the samples in order to avoid false positive results. Before shipping, FCM samples need to be wrapped in an inert foil such as aluminium. On receipt, they should be catalogued and stored in a clean, dry and cool environment. Direct contact with the food contact surface should be avoided. Samples should be processed as soon as possible.
The recommended procedure for sample preparation is as follows
Migration versus extraction
Migration and extraction conditions for chemical analysis are both considered relevant for testing in in vitro bioassays. Either extraction and/or migration could be performed. Migration protocols are not a primary scope of this review. For such studies, it is advised to follow available regulatory guidelines proposing relevant simulants and experimental conditions according to the types of foods to be covered. To deviate from such guidelines, scientific justification should be provided.
Extraction is considered important to provide information on which chemicals could potentially occur in FCM and consequently migrate into food. Extraction data are also useful to direct and focus further investigations using the migration approach. Data generated on extracts should always be interpreted with care and in comparison with migration data.
Different extraction solvents have been used (amongst others dichloromethane, acetonitrile and hexane/acetone) and protocols are available. Dichloromethane is genotoxic and might cause false-positive results in the bioassay if not removed completely (Jongen et al. Citation1978) but could be used for analytical approaches. Extraction conditions should be determined based on the physico-chemical properties (e.g. polarity) of the material studied. It is generally considered that extraction constitutes a worst-case scenario over migration. Such an assumption should be confirmed and documented.
To confirm that the extraction is complete and exhaustive, successive extractions can be used and the analytical profiles compared. If necessary, extremes of solvent polarities should be used until R&D experimental studies confirm a combination is suitable. This may mean that more than one extraction solvent or food simulant is required.
Blanks should always be run in order to exclude any influence of substances other than those of the FCM, this would include extraneous substances originating from laboratory chemical solutions and equipment/utensils used in the preparation of the samples.
Surface to volume ratio has to be determined and documented. For migration studies, it should represent a worst-case application of the tested sample. If this is not known, a surface to volume ratio corresponding to at least 20 dm2 per L of simulant should be chosen. In general FCM surface to solvent volume ratio and concentration factor should be optimised to obtain the highest possible concentration factor, although avoiding material precipitation and/or cytotoxicity, which could alter the detection of mutagenic activity. This might require conducting preliminary studies in order to determine the best suited testing conditions.
Concentration
Having obtained a migrate/extract, it will be necessary to concentrate (e.g. through evaporation) and transfer the concentrate to a solvent suitable for bioassays. Because of the substantial experience of its use in the in vitro field, its ability to increase the solubility of organic chemicals in physiological media and its relatively good cell tolerability, DMSO is recommended. Ethanol could be a suitable alternative, which might facilitate sample preparation and parallel chemical analysis. Depending on the solvent used in the extraction, a solvent swap step might be needed.
Migrate/extract concentration would normally range from a minimum of 100-fold up to 1000-fold. Concentration factors should be determined and documented.
When concentrating an extract (frequently a gentle stream of nitrogen and sometimes heat and/or vacuum is used), it is important to ensure that any volatile components are retained as best possible in the test mixture. Evaporation to dryness or near dryness ensures a good removal of the solvent, but can also lead to losses of volatile and semi-volatile compounds. To reduce the loss of volatiles, evaporation to dryness should be avoided and DMSO should be added to the solvent as a “keeper” prior to the final and complete evaporation of the solvent being removed if a solvent change is necessary.
If the solvent/simulant used for extracting the migrants is not particularly volatile (e.g. aqueous solutions), other concentration methods, such as SPE (solid phase extraction) or liquid-liquid extraction, should be considered. However, it has to be recognised that a single step SPE or liquid-liquid extraction method will not be able to concentrate the whole range of polarities known for genotoxic substances.
Extracts/migrates in water, or other aqueous media that are compatible with bioassays, can be applied at concentrations up to 90% in the bioassay (in combination with concentrated bioassay media), while the concentration of organic solvents is usually limited to a maximum of 4-10%. Therefore, for water extracts, there is a reduced requirement for sample concentration that might be compensated by increasing the surface to volume ratio during extraction/migration.
Chromatograms obtained from the analysis of the migrant extract/solution before and after the concentration step and transfer to a bioassay-compatible solvent should be compared to ensure that a representative sample is being tested.
Fractionation of the migrates/extracts to exclude substances of low genotoxic relevance but present at high concentrations (e.g. oligomers) could be considered in order to minimise solubility and cellular toxicity issues.
Different components of the migrant/extract solutions will have different solubility at each stage of the process. Concentrating and re-diluting the solution may partially fractionate the migrants or extracted chemicals.
It is advisable to validate the sample concentration method by spiking representative migrates/extracts (before the concentration steps) with model substances that reflect the critical physico-chemical properties (volatility, polarity) of genotoxic substances. The recovered amount of these spiked model substances can be determined analytically in the concentrated sample, to evaluate possible losses during sample concentration steps.
If there is a precipitate it should be ensured that the bioassay is not negatively affected by the precipitate. Precipitation during sample preparation may result in the loss of relevant substances and/or inhibition of the bioassay. If precipitation is unavoidable, spiking experiments can help to demonstrate that the in vitro bioassay is not inhibited by the precipitated material (see section 3.2). If necessary, fractionate then test the selected fractions as above. It is most likely that the precipitate will form when the DMSO is combined with the aqueous buffer prior to the application to the assay. It should be borne in mind that the keeper does not need to be DMSO, for example Tween80 could be used.
Bioassays
To support the application of the Cramer class III-TTC for unknown chemicals, an assay capable of identifying direct DNA-reactive mutagens is required. In addition, the bioassay needs to be capable of identifying true positives at low levels in mixtures (low LECs/LOBDs).
The Ames test is the most widely used and characterised short-term in vitro genotoxicity bioassay. It is the test currently most compliant with the criteria highlighted above. OECD-guidelines are available.
The use of the standard Ames pre-incubation method or agar plate assay (according to OECD 4713) with five different S. typhimurium strains is recommended. Tests should be run in triplicate. An important drawback is its high sample volume requirements.
Several miniaturised Ames test versions have been developed to cope with low availability of the material to be tested (Escobar et al. Citation2013). Many have been documented to exhibit performance similar to that of the standard Ames test. The development of an OECD Guideline for a number of miniaturised Ames tests is ongoing.
A microsuspension protocol using pre-incubation (90 min) of the sample with 10-fold more concentrated bacterial cultures may be suitable, as preliminary evaluations indicate possible lower LECs as compared to other tests such as the standard Ames assay (McDaniels et al. Citation1990; Wegrzyn and Czyz Citation2003; Fluckiger-Isler and Kamber Citation2012; Escobar et al. Citation2013; Proudlock and Evans Citation2016). A miniaturised version of this test is available (Zwarg et al. Citation2018). However, more data are required before a firm recommendation to use one of these miniaturised tests can be made.
Many direct DNA-reactive mutagens exert their genotoxic activity after phase-I biotransformation. Any assay used to test genotoxicity should be competent for such enzymatic reactions, either intrinsically or through addition of an enzyme-cofactor system. For the Ames test, the addition of S9 liver fraction from Aroclor 1254- or phenobarbital/β-naphthoflavone-treated rats is generally recommended.
The production of cytotoxicity in the bioassay is a key element in interpreting genotoxicity data. Indeed, cytotoxicity of some components may either mask the genotoxic activity of other components, or induce genotoxicity in some test systems (Kirkland et al. Citation2007). However, it is important to try to achieve cytotoxic concentrations if at all possible to verify that higher concentrations could not have been tested. Therefore, any genotoxicity assay should provide cytotoxicity information.
Criteria defining a positive response should be available. For the standard Ames test, to be considered positive, a sample has to induce >1.5–3.0-fold increase in the number of revertants over background depending on strainFootnote10, with a trend of dose-response (Mortelmans and Zeiger Citation2000). Additional dilutions with narrower steps may be necessary to be able to observe a dose-response in a positive sample.
In the standard Ames assay, it is widely recommended to test material up to a concentration of 5 mg/plate (if possible, according to solubility and cytotoxicity) (European Food Safety Authority Scientific Committee Citation2011). In its draft document on genotoxicity testing of mixtures, EFSA mentions using even higher concentrations, if feasible. Such high concentrations will in general not be feasible for FCM migrates/extracts (More et al. Citation2019). The highest level of migrate/extract tested could be estimated through the determination of overall migration (Standardization ECf. Citation2002).
DMSO is recommended as solvent for the tested material. Ethanol may also be used at up to 50 µg/plate, and may provide advantages in sample preparation. The treatment regimen should account for solvent tolerability in the bioassay used. Scientific literature is available providing information on DMSO and ethanol tolerability in different bioassays (Maron et al. Citation1981; Hakura et al. Citation1993, Citation2010; Di and Kerns Citation2006). This may be up to 14% for DMSO in the Ames test. Preliminary testing may be useful to further optimise experimental conditions.
The total concentration factor, resulting from the concentration of the migrate/extract and subsequent dilution of the concentrated extract with culture medium in the bioassay would normally range from a minimum of 10-fold up to 40-fold. In any migration or extraction studies, concentration factors should be determined and documented.
The dosing should be as high as possible, starting with the highest tolerated solvent concentration (e.g. 4-14% DMSO or 50 µg/plate ethanol in standard Ames test). Considering a maximum possible migrate/extract concentration factor of 1000 and taking into account the dilution necessary to prevent DMSO cytotoxicity, a total best case highest concentration factor of 40 can be anticipated. This may rarely be possible in practice, but an overall factor of at least 10 should be sought. In any migration or extraction studies, total concentration factors should be determined, traceable and documented.
The sample matrix can have significant effects on the mutagen LOBDs of the assay, for example if bacterial growth is inhibited. To test for matrix-interference, FCM-migrates/extracts (or samples in the bioassay compatible solvent) should be spiked with known genotoxicants, one directly active (e.g. 2-nitrofluorene or 4-nitroquinoline N-oxide) and one requiring metabolic activation (e.g. 2-aminoanthracene or benz[a]pyrene) to check the recovery of the activity and confirm that the predetermined LECs/LOBDs are conserved.
Data interpretation
As mentioned earlier, concluding on the mutagenicity of FCM migrates cannot rely only on bioassay data. To support the mutagenicity assessment of FCM-migrates, all available information and experimental chemical and biological data have to be collected, assembled, and then integrated through a weight of evidence approach (Kirkland et al. Citation2016). A review of the information and data from the perspectives of relevance and reliability needs to be performed as a first filter. Relevance is defined as the contribution a piece of evidence would make to answer the question, while reliability refers to the correctness and quality of the available data/information. For FCM-migrates, three relevant lines of evidence may be expected:
Information on chemistry, recipes and production of the FCM. This includes a list of the chemicals with technological function (IAS) such as monomers, antioxidants, and plasticizers. Chemical reactions involved in the manufacture of the FCM should be described, with particular emphasis on possible reaction products and oligomers. Data on impurities in starting raw materials should also be provided. Genotoxicity data should be collected for all individual chemicals cited. These could be either experimental data (e.g. available for IAS) or in silico predictions using both (Quantitative) Structure Activity Relationships (QSARs) and grouping/read across (Schilter et al. Citation2014). There are a number of models capable of predicting Ames mutagenicity with high accuracy (Benfenati et al. Citation2018; Honma et al. Citation2019). To increase confidence, it is recommended to integrate QSAR models based on numerical descriptors with rule-based expert systems. This approach has been successfully applied to food packaging chemicals (Manganelli et al. Citation2018). The quality of the retrieved information as well as its relevance to direct DNA-reactivity/mutagenicity should be established.
Analytical chemistry of FCM extracts and/or migrates. Analytical data based on GC- and LC–MS should be reviewed with emphasis on peak identity. The possibility of excluding the presence of specific substances (e.g. high potency chemicals in the cohort of concern) or classes of chemicals (e.g. organophosphorus compounds) should be highlighted and justified. Genotoxicity information, either experimental (if available) or predicted, should be provided for all individual chemicals identified.
Bioassay data. The quality/reliability of the data should be reviewed together with their relevance regarding detection of direct DNA-reactive chemicals. Possible interference with the test (e.g. cytotoxicity, inhibition of cell growth) should be identified and taken into account. Suitable information on the nature of the sample(s) tested (migrates, extracts) should be available as well as the concentration factor(s) involved. As mentioned earlier, the Ames test is the most relevant because of its ability to detect DNA-reactivity and its anticipated lower LOBD as compared to other (e.g. mammalian cell) testing procedures. Any other tests could be acceptable if they were shown to detect direct DNA-reactive chemicals and if their LOBDs for standard mutagens were at least similar to those obtained in the Ames test. If there is no evidence of mutagenicity in in vitro bioassay of extraction samples, such data alone might be sufficient. However, evidence should be provided that the extraction procedures represent a worst-case scenario and the bioassay is not inhibited by the sample extract.
The three lines of evidence should then be weighted and integrated in order to generate a conclusion on concern for mutagenicity. There is no formal approach to establish the weight of evidence. This should be based on expert judgement and should be transparently documented (Hardy et al. Citation2017). Several possible situations may emerge according to the degree of consistency across the lines of evidence available:
All lines consistently indicate a low concern for mutagenicity/DNA reactivity. In such situations, the Cramer class III TTC is considered applicable for prioritisation regarding identification of uncharacterized peaks. If there are no specific reasons otherwise, the TTC should be applied to individual peaks and not to the sum of all the unknown peaks of the forest. This implies that by default combined effects are not considered. This is justifiable by the fact that interactions (e.g. synergy) are documented to be very rare at exposure levels well below effect levels (Boobis et al. Citation2011). This is considered to be the case for packaging chemicals present in food at levels corresponding to the Cramer class III TTC or lower. Where migrates act through similar mode of action, dose addition after correction for potency, might be relevant. However, as suggested by Leeman et al. (Leeman et al. Citation2013), for chemicals at levels corresponding to the Cramer class III TTC or lower, the probability that the sum of doses reaches a level sufficient to produce toxicity is anticipated to be very low.
The migrate/extract is not mutagenic in the bioassay but chemical(s) that is/are known to be mutagenic are identified as/suspected to be present. Mutagenic chemical(s) is/are identified either from the review of the FCM manufacturing process and/or the chemical analysis of the FCM migrate and/or extract, but no mutagenicity is observed in the Ames test. In such a situation identified mutagenic chemicals have to be assessed by chemical-specific data, either available or to be generated. The first step is to characterise the migration of such chemical(s) in order to estimate exposure. This is particularly important if such chemical(s) are measured in an extract. Migration data in relevant food simulant are necessary and levels might also be checked in the actual food. Absence of migration may resolve the problem, but should be established using analytical methods with appropriate LOCD. Standard genotoxicity approaches may be used to exclude possible false positive and indirect mechanisms (e.g. oxidative stress). Additional data can be obtained to establish the in vivo significance (performing an in vivo test with the pure chemical) and human relevance (e.g. addressing differences in metabolism) of the chemical-specific in vitro data. If the migration of the chemical(s) raising concern for mutagenicity can be excluded or if strong evidence can be generated documenting that the concern for DNA reactivity is irrelevant, then the Cramer class III TTC is considered applicable for prioritisation regarding identification of uncharacterized peaks. If the requirements above are not met, then the Cramer class III TTC cannot be used.
The migrate/extract is mutagenic in the bioassay and chemical(s) that is/are known to be genotoxic are identified as/suspected to be present. In such a situation, considerations as highlighted in situation 2 above are applicable. If the migration of the known chemical(s) raising concern for mutagenicity can be excluded or if strong evidence can be generated documenting that the concern for mutagenicity is irrelevant (e.g. negative in an appropriate in vivo genotoxicity study), then a concordance analysis has to be conducted. This approach should ensure that the mutagenicity of the migrate is explained by the presence of a known substance(s) with mutagenic properties and not by the hidden presence of another, unidentified mutagenic chemical(s). It is acknowledged that concordance studies are often difficult and should be interpreted with caution. If the concordance analysis provides sufficient evidence to exclude the contribution of unidentified mutagenic chemical(s), then the Cramer class III TTC is considered applicable for prioritisation regarding identification of uncharacterized peaks. If the requirements above are not met, then the Cramer class III TTC cannot be used.
The migrate/extract is mutagenic in the bioassay but no chemical(s) that is/are known to be mutagenic is/are identified as/suspected to be present in the migrate/extract. In such a situation, the responsible chemical(s) need(s) to be identified. Concordance analysis is then required to ensure that the mutagenicity of the migrate is explained by the newly identified mutagenic chemical(s). The safety of these chemicals can be further assessed based on chemical-specific data, either available or to be generated. It is acknowledged that concordance studies are often difficult and should be interpreted with caution. This is particularly important when the mutagenicity data obtained with the concentrated migrate are borderline/equivocal (e.g. weak activity at the highest concentration only, only 1 batch or replicate weakly positive) and no chemical with an alert for genotoxicity is found. Identification of the chemical(s) will likely require applying a fractionation approach as part of a concordance analysis. If the migration of the identified chemical(s) raising concern for mutagenicity can be excluded (e.g. based on migration into food) or if strong evidence can be generated documenting that the genotoxic concern is irrelevant (e.g. negative in an appropriate in vivo genotoxicity study), then the Cramer class III TTC is considered applicable for prioritisation regarding identification of uncharacterized peaks. If the requirements above are not met, then the Cramer class III TTC cannot be used.
Research needs
Research is required to optimise the limit of detection of mutagenicity testing of FCM with bioassays. In addition to bioassay development and optimisation, there is also some potential to improve the limit of biological detection by optimising the sample preparation.
Sample preparation
It is necessary to confirm which solvent(s) are applicable to which combination of materials/migratable substances and determine whether a single solvent or a single mixture of solvents is applicable.
It is also necessary to confirm which extraction/test conditions are applicable to which combination of materials/migratable substances.
Development and validation of sample concentration methods
Genotoxic substances represent a large and heterogeneous group of chemicals with a wide range of different properties which cannot easily be concentrated without some losses being expected during sample preparation. Currently, few data are available on the concentration efficiency of sample preparation methods for genotoxic substances (Rainer et al. Citation2019; Rainer et al. Citation2018). Different sample preparation methods (e.g. evaporation, solid phase extraction, liquid-liquid-extraction) should be evaluated for their suitability to concentrate a broad spectrum of different genotoxic substances. The most promising methods should be optimised for the highest practical concentration of the most common migration solvents previously used (e.g. 95% ethanol, 50% ethanol, 3% acetic acid) and the best transfer to a bioassay compatible solvent, with as few losses as possible. This is especially relevant for less-volatile aqueous solvents (e.g. water, 50% ethanol, 3% acetic acid) for which no agreed upon method are currently available that allows efficiently concentrating the broad range of polarities observed for mutagens. To evaluate the recoveries for different genotoxic substances, migrates can be spiked with representative genotoxic substances, and the recovery can be determined by analytical determination of their concentration in the final concentrated extract. The report of Adahchour et al. (Adahchour and van Hattum Citation2001) provides an extensive list with physico-chemical properties of 156 different genotoxic organic substances, from which a set of representative substances can be selected, which represent the variety of genotoxic substances likely to be present in FCMs. However, there still remains a research need to confirm that during concentration of the migrant solution or extract, substances are not lost.
Transfer of extracts to bioassay compatible solvents
Most currently used sample concentration procedures involve transfer to DMSO or ethanol. Cell cultures can tolerate only low concentrations of DMSO or ethanol (Bradley et al. Citation2010). Therefore, substantial dilution with cell culture media is necessary, which results in higher limits of detection. If the migrates can be transferred to solvents, which do not require such high dilution factors, significantly better limits of bio-detection could be obtained. Different solvents should be compared, although, according to current knowledge, this would most probably require the use of aqueous solvents, as high concentrations of organic solvents are not compatible with bioassays. However, solubility of lipophilic substances in aqueous solvents could be limiting with this approach. Glucose or serum proteins, which are used in most bioassay media, could perhaps function as solubilizers, similar to DMSO in an aqueous solvent. If, for example, a 20% DMSO solution in water could be used instead of pure DMSO, the concentration factor could in theory be increased five-fold. Aqueous solvents containing bioassay-compatible solubilizers will not be able to dissolve the same amount of lipophilic FCM migrants as pure solvents (e.g. DMSO). However, solubility in this step of the sample preparation is not necessarily the limiting factor for the analysis, as in the final bioassay, aqueous solvents have to be used anyway, and only substances that are soluble in the final assay medium can be evaluated in a bioassay. It is necessary to confirm that substances are not lost during a solvent swap.
Fractionation
It is known that for many FCM, relatively high molecular weight chemicals comprising oligomers and derivatives may constitute the major quantitative fraction of the migrates/extracts. Although not a concern from a genotoxicity point of view, these components may interfere with the test, e.g. by reducing the concentration in the test media, or by inducing cellular toxicity, or by inhibiting bacterial growth. This may significantly alter the bioassay performance and contribute to a matrix effect, consequently impairing the accuracy and the LOBDs of the assay. To develop a routine approach to separate out the fraction >1000 Daltons would potentially bring significant improvement to genotoxicity testing of FCM migrates/extracts. It is necessary to investigate any impact of GPC (gel permeation chromatography)/membranes on the fractionation and removal of any chemicals present in the solution before fractionation. There is a research need to confirm that substances <1000 Daltons are not lost during the fractionation step.
Bioassays
Bacterial tests
There have been numerous efforts to modify the Ames test, with the main objectives of reducing the amount of material needed to be tested and of detecting genotoxins at lower LECs. Such methods may be of great relevance for FCM genotoxicity evaluation. Some of these methods use standard tester strains but with plating in multi-well format allowing significant reduction in the amounts of the components of the assay as compared to the standard Ames test (e.g. µAmes (Proudlock and Evans Citation2016)). Others employ a pre-incubation step with increased concentrations of bacteria (Agurell and Stensman Citation1992; Kado et al. Citation1983; Watanabe et al. Citation1995; Azuma et al. Citation1997). Such a test exists in standard and miniaturised formats (Zwarg et al. Citation2018). However, currently, insufficient data are available on the comparison of the limit of biological detection with these variations to the standard Ames test to judge their suitability for FCM safety assessment. LEC values, which can be used to estimate the limit of biological detection, are available for only a few compounds. To enable a useful comparison, a standard Ames test should be conducted in parallel to the modified variations, using the same batches of chemicals and the same tester strains. Further, a representative set of substances with different chemical and biological properties should be compared, as the improvement of the LEC values might be specific for certain compounds, and might lead to higher LEC values for other compounds.
The methods described above are modifications of the Ames test as described in the OECD-guideline 4713. Other screening tests have been developed, relying on different bacterial strains and principles. For example, some are based on measuring induction of genes associated with DNA damage and repair, including umu and SOS system components, with colorimetric or luminescence detection methods (McDaniels et al. Citation1990; Ates et al. Citation2016). Bacterial tester strains responding at low concentrations of certain classes of genotoxicants alone or when present in biological samples have also been designed or selected (Kado et al. Citation1983; Takagi et al. Citation2011) and constitute the basis of mutagenicity assays. To assess some of these test systems for bioassay of FCM mutagenicity, and specifically to address their ability to detect concentrations of mutagens necessary to ensure safety would be very valuable. Focus should be on both high and low potency DNA-reactive mutagens, including some that are FCM-relevant.
Other bioassay-based tests
Other new tests, based mainly on mammalian cells, are being developed. Efforts have focused mainly on providing tools based on cells of human origin, and/or on tests able to detect or differentiate multiple mechanisms of genotoxicity (Hughes et al. Citation2012; Ates et al. Citation2016). However (for understandable reasons), very little emphasis has been made on developing systems responding to very low concentrations of genotoxicants. Little information is available on possible LOBDs, although from the limited data available LECs are rather high and most are likely higher than the ones obtained with the Ames test. However, modifying experimental conditions and test settings may possibly lower LECs significantly and be worth investigating.
Planar chromatography coupled to bioassays
Recently, a method using planar chromatography using reversed phase high-performance thin-layer chromatography (HPTLC) plates with detection by specific microorganisms has been developed (Morlock and Klingelhöfer Citation2014). Briefly, chemical mixtures are separated by HPTLC and then a specific bioassay is run directly on the TLC plate. This is a procedure for detecting chemicals based on mechanism of action revealing only the biologically active chemicals. Such an approach may bring breakthrough advantages. Because the biological reaction is happening on the separated bands where the chemicals are concentrated, the LECs/LOBDs are expected to be much lower than with other methods in liquid media. This may require a smaller overall concentration of the samples without the need for transfer into a bioassay compatible solvent. Consequently, the issue of chemical losses during sample preparation would likely be significantly lower. In addition, because the chemicals are separated, potential matrix effects will be reduced. This approach has been applied to identify unknown bioactive molecules in complex mixtures such as foods, beverages and plant extracts (Morlock and Klingelhöfer Citation2014). Recently, this technique has been adapted to detect endocrine active chemicals (Klingelhofer and Morlock Citation2015), showing promising, high potential to detect very low levels. Attempts to combine the Ames test and SOS-umu test with TLC have been undertaken (Egetenmeyer and Weiss Citation2017; Weiss et al. Citation2017). Further work is necessary to assess the value of such an approach and to validate it against a number of potent and less potent direct DNA-reactive mutagens in complex mixtures such as FCM migrates/extracts.
New test validation and quality assurance
In order to ensure the usefulness and acceptance of new or modified bioassays for research, and particularly for regulatory purposes, requires that the assay is well defined, reproducible and that it accurately predicts the desired mechanism of action and/or toxic effect. The scientific validation of alternative methods aims to establish the relevance and reliability of a test method for a particular purpose and evaluates these aspects (Balls M. Citation1995; Hartung et al. Citation2004; OECD 2005Footnote11). Validation of genotoxicity tests should be undertaken using an appropriate battery of chemicals (Luijten et al. Citation2016) and control data ranges should be established. Note that establishing the validity of a method for the purpose proposed here (as a sensitive biodetection system) will involve different considerations than those necessary for validation for hazard identification (the typical use of a genotoxicity assay).
When applying a bioassay to test the genotoxicity of FCM migrates/extracts to support the application of the Cramer class-III TTC, the relevance relates to level of detection (low LOBDs, accounting for potential matrix effect) and mechanism (direct DNA-reactivity). The reliability of a new or modified test has to be characterised by evaluating its within-laboratory reproducibility, its transferability to other laboratories and its between-laboratory reproducibility. In addition, a test definition must be available comprising a standard protocol and prediction model, i.e. an algorithm used to convert the in vitro results into information that is fit for purpose such as, for example, prediction of the potential (non-)genotoxic effects.
The predictive capacity of the new/modified bioassay should be assessed with well characterised chemicals representing different chemical classes and can be compared to other reference tests predicting the same endpoint. Importantly, the predictive capacity might be specified for a defined applicability domain of the assay, which describes the types of chemicals and/or range of effects to which the test can be applied and provide reliable predictions.
The level of information required to ensure the scientific validity of an assay will depend on its developmental stage. A completely newly developed assay will require comprehensive information on reproducibility (within- and between-laboratories, and if relevant, also on predictive capacity such as required within the OECD Guidance Document 3411). To obtain historical control data is also of importance to ensure acceptability of new experiments and assist in data interpretation. A modified assay that is considered similar to a bioassay that has already gained regulatory acceptance may require only a limited amount of information to demonstrate that the new/modified assay provides similar performance as the scientifically valid one.
Conclusions
FCM migrates are complex mixtures containing some uncharacterized chemicals. With respect to safety evaluation, the unknowns constitute the major challenge. Identifying and testing all of them toxicologically is considered not feasible.
The application of the Cramer class III TTC is proposed to prioritise the unknown substances on which identification efforts should be focused.
However, application of the Cramer class III TTC requires excluding the presence of certain chemical categories, in the migrates under investigation.
These chemical categories include substances that are genotoxic. However, in line with internationally accepted guidance from ICH, EFSA and WHO amongst others, the concern for genotoxicity of substances present at low levels applies only to direct DNA-reactive mutagens. For other genotoxic modes of action (e.g. clastogenicity, aneugenicity), the considerations for non-genotoxic compounds would apply.
The present paper has reviewed the potential role of bioassays for identifying direct DNA-reactive genotoxicants in the assessment of packaging materials.
In this context, to be fit for purpose, an in vitro genotoxicity bioassay must be capable of detecting DNA-reactive mutagens at very low levels (low limits of biological detection, LOBDs). This will vary with the specific chemical.
No fully satisfactory bioassay was identified. In general, estimated LOBDs were too high to detect DNA-reactive mutagens at levels considered of low concern as derived from linear dose-response cancer risk extrapolation or acceptable margins of exposure.
However, the Ames assay was considered to provide valuable information. Indeed, it is capable of identifying DNA-reactive mutagens with high efficiency and accuracy. In addition, for high potency genotoxic carcinogens, detection levels lower than 0.01 mg/kg simulant (10 ppb) may be achieved. 10 ppb is the pragmatic cut-off limit widely applied in the EU, especially within the industry, to manage unknown chemicals in FCM-migrates. Applying a bioassay capable of detecting potent mutagens at levels <10 ppb would certainly bring additional benefits over the current practices in the safety evaluation of FCMs.
For a specific migrate, it is considered that in the absence of evidence of genotoxic concern based on:
Adequate information on composition and manufacturing of the FCM;
Analytical chemistry data on relevant extracts and migrates;
Ames test data;
The use of the Cramer class III TTC to support prioritisation of unknown chemicals is believed to be protective and applicable.
A review of the scientific literature indicates that various genotoxicity tests have been applied to FCM migrates. However, the available studies are often not well described and are difficult to interpret in the context of supporting the use of the Cramer class III TTC for prioritisation. Therefore, it is not yet possible to develop clear guidance. Instead, recommendations are proposed, as well as points to consider thoroughly when such studies are conducted. They concern mainly sample preparation (concentration, transfer in bioassay compatible solvent) and bioassay methods, with the aim to ensure the lowest possible LOBDs. Guidance on data interpretation has also been developed.
Several research avenues were identified focusing on the reduction of genotoxicity bioassay LOBDs. They involve improvements in both sample preparation and bioassay methods. They are considered to bring strong promise to improve the current situation.
The approach outlined in the present paper was reviewed in a workshop organised by ILSI Europe gathering 45 experts in relevant scientific fields including FCM manufacturing and composition, analytical chemistry, bioassay, toxicology and risk assessment. Although it was recognised that research is still necessary to address key issues such as LOBDs of mutagens, there was a broad acknowledgement that the developed approach as outlined in the present manuscript constitutes a significant improvement over currently applied methodologies and provides a relevant framework for integration of mutagenicity bioassays in the safety assessment of FCM. It was debated whether this new approach is applicable now or if some data gaps need to be filled first. Regardless, it was widely recognised that, although not perfect, the approach will already bring valuable benefits to reduce uncertainties in the risk assessment of FCMs.
It is important to note that the approach as described in the present paper is aimed at facilitating scientific safety assessment of FCMs. It is not intended to be a quality control tool. Although applicable to any FCM, it is viewed as particularly valuable in the frame of new packaging development, in line with safety by design principles. The information originating from the application of the approach should serve in the design of specifications, which can then be translated into a quality management plan.
Acknowledgments
This manuscript has been shared and debated during a workshop organised on 8-9 November 2018 in Brussels by the European branch of the International Life Sciences Institute, ILSI Europe, through the financial support from ILSI Europe’s Packaging Materials Task Force. We wish to thank all workshop participants for their valuable comments. The authors carried out the work that is, writing the scientific paper; separate to other activities of the task force.
Disclosure statement
No potential conflict of interest was reported by the authors.
Additional information
Funding
Notes
1. OECD Test No. 471: Bacterial Reverse Mutation Test.
2. OECD Test Guideline No. 487: In Vitro Mammalian Cell Micronucleus Test (MNvit).
3. European Commission, Council Directive 93/42/EEC of 14 June 1993 concerning medical devices.
4. ISO 10993–1:2009. Biological evaluation of medical devices – Part 1: Evaluation and testing within a risk management process.
5. ISO 10993–3:2014. Biological evaluation of medical devices – Part 3: Tests for genotoxicity, carcinogenicity and reproductive toxicity.
6. OECD Test No. 473: In vitro Mammalian Chromosome Aberration Test.
7. OECD Test No. 490: In Vitro Mammalian Cell Gene Mutation Tests Using the Thymidine Kinase Gene.
8. EMEA/HMPC/107079/2007: Committee on herbal medicinal products – Guideline on the assessment of genotoxicity of herbal substances/preparations.
9. OECD Test No. 476: In vitro Mammalian Cell Gene Mutation Test.
10. TA 1535, TA1537 1.5-fold; TA 1538, TA 98, TA100 2-fold; TA102 3-fold increases, respectively.
11. OECD Guidance Document No. 34 on The Validation and International Acceptance of New or Updated Test Methods for Hazard Assessment.
References
- Adahchour MV, van Hattum AGM 2001. Concentration techniques for genotoxicity testing of effluents. [RIVM] Institute for environmental studies. Amsterdam, The Netherlands: RIVM Report; No. E-01/08
- Agurell E, Stensman C. 1992. Salmonella mutagenicity of three complex mixtures assayed with the microsuspension technique. A WHO/IPCS/CSCM study. Mutat Res. 276(1–2):87–91.
- Ates G, Favyts D, Hendriks G, Derr R, Mertens B, Verschaeve L, Rogiers V, Doktorova TY. 2016. The Vitotox and ToxTracker assays: a two-test combination for quick and reliable assessment of genotoxic hazards. Mutat Res. 810:13–21.
- Azuma S-I, Kishino S, Katayama S, Akahori Y, Matsushita H. 1997. Highly sensitive mutation assay for mutagenicity monitoring of indoor air using salmonella typhimurium YG1041 and a microsuspension method. Mutagenesis. 12(5):373–377.
- Bach C, Dauchy X, Severin I, Munoz JF, Etienne S, Chagnon MC. 2013. Effect of temperature on the release of intentionally and non-intentionally added substances from polyethylene terephthalate (PET) bottles into water: chemical analysis and potential toxicity. Food Chem. 139(1–4):672–680.
- Bach C, Dauchy X, Severin I, Munoz JF, Etienne S, Chagnon MC. 2014. Effect of sunlight exposure on the release of intentionally and/or non-intentionally added substances from polyethylene terephthalate (PET) bottles into water: chemical analysis and in vitro toxicity. Food Chem. 162:63–71.
- Balls M. 1995. Practical aspects of the validation of toxicity test procedures. The report and recommendations of [ECVAM] European Union Reference Laboratory for alternatives to animal testing workshop 5. ATLA. 23:129–147.
- Bal-Price A, Crofton KM, Leist M, Allen S, Arand M, Buetler T, Delrue N, FitzGerald RE, Hartung T, Heinonen T, et al. 2015. International STakeholder NETwork (ISTNET): creating a developmental neurotoxicity (DNT) testing road map for regulatory purposes. Arch Toxicol. 89(2):269–287.
- Barlow S. 2005. Threshold of Toxicological Concern (TTC). ILSI Europe Concise Monographs Series.
- Begley T, Castle L, Feigenbaum A, Franz R, Hinrichs K, Lickly T, Mercea P, Milana M, O’Brien A, Rebre S, et al. 2005. Evaluation of migration models that might be used in support of regulations for food-contact plastics. Food Addit Contam. 22(1):73–90.
- Benfenati E, Golbamaki A, Raitano G, Roncaglioni A, Manganelli S, Lemke F, Norinder U, Lo Piparo E, Honma M, Manganaro A, et al. 2018. A large comparison of integrated SAR/QSAR models of the Ames test for mutagenicity. SAR QSAR Environ Res. 29(8):591–611.
- Binderup ML, Pedersen GA, Vinggaard AM, Rasmussen ES, Rosenquist H, Cederberg T. 2002. Toxicity testing and chemical analyses of recycled fibre-based paper for food contact. Food Addit Contam. 19(Suppl):13–28.
- Bolognesi C, Castoldi AF, Crebelli R, Barthélémy E, Maurici D, Wölfle D, Volk K, Castle L. 2017. Genotoxicity testing approaches for the safety assessment of substances used in food contact materials prior to their authorization in the European Union. Environ Mol Mutagen. 58(5):361–374.
- Boobis A, Brown P, Cronin MTD, Edwards J, Galli CL, Goodman J, Jacobs A, Kirkland D, Luijten M, Marsaux C, et al. 2017. Origin of the TTC values for compounds that are genotoxic and/or carcinogenic and an approach for their re-evaluation. Crit Rev Toxicol. 47(8):705–727.
- Boobis A, Budinsky R, Collie S, Crofton K, Embry M, Felter S, Hertzberg R, Kopp D, Mihlan G, Mumtaz M, et al. 2011. Critical analysis of literature on low-dose synergy for use in screening chemical mixtures for risk assessment. Crit Rev Toxicol. 41(5):369–383.
- Bovee TF, Hoogenboom LA, Hamers AR, Traag WA, Zuidema T, Aarts JM, Brouwer A, Kuiper HA. 1998. Validation and use of the CALUX-bioassay for the determination of dioxins and PCBs in bovine milk. Food Addit Contam. 15(8):863–875.
- Bradley EL, Honkalampi-Hamalainen U, Weber A, Andersson MA, Bertaud F, Castle L, Dahlman O, Hakulinen P, Hoornstra D, Lhuguenot JC, et al. 2008. The BIOSAFEPAPER project for in vitro toxicity assessments: preparation, detailed chemical characterisation and testing of extracts from paper and board samples. Food Chem Toxicol. 46(7):2498–2509.
- Bradley EL, Stammati A, Salkinoja-Salonen M, Andersson M, Bertaud F, Hoornstra D, Zucco F, Weber A, Turco L, Traussnig H, et al. 2010. Test procedures for obtaining representative extracts suitable for reliable in vitro toxicity assessment of paper and board intended for food contact. Food Addit Contam Part A. 27(2):262–271.
- Brand W, de Jongh CM, van der Linden SC, Mennes W, Puijker LM, van Leeuwen CJ, van Wezel AP, Schriks M, Heringa MB. 2013. Trigger values for investigation of hormonal activity in drinking water and its sources using CALUX bioassays. Environ Int. 55:109–118.
- Claxton LD, Matthews PP, Warren SH. 2004. The genotoxicity of ambient outdoor air, a review: salmonella mutagenicity. Mutat Res. 567(2–3):347–399.
- Clive D, Johnson KO, Spector JF, Batson AG, Brown MM. 1979. Validation and characterization of the L5178Y/TK± mouse lymphoma mutagen assay system. Mutat Res. 59(1):61–108.
- Connor TH, Barrie MD, Theiss JC, Matney TS, Ward JB Jr. 1983. Mutagenicity of formalin in the Ames assay. Mutat Res. 119(2):145–149.
- Couch DB, Forbes NL, Hsie AW. 1978. Comparative mutagenicity of alkylsulfate and alkanesulfonate derivatives in Chinese hamster ovary cells. Mutat Res. 57(2):217–224.
- De Fusco R, Monarca S, Biscardi D, Pasquini R, Fatigoni C. 1990. Leaching of mutagens into mineral water from polyethyleneterephthalate bottles. Sci Total Environ. 90:241–248.
- Dearfield KL, Gollapudi BB, Bemis JC, Benz RD, Douglas GR, Elespuru RK, Johnson GE, Kirkland DJ, LeBaron MJ, Li AP, et al. 2017. Next generation testing strategy for assessment of genomic damage: a conceptual framework and considerations. Environ Mol Mutagen. 58(5):264–283.
- Di L, Kerns EH. 2006. Biological assay challenges from compound solubility: strategies for bioassay optimization. Drug Discov Today. 11(9–10):446–451.
- Driffield M, Garcia-Lopez M, Christy J, Lloyd AS, Tarbin JA, Hough P, Bradley EL, Oldring PKT. 2018. The determination of monomers and oligomers from polyester-based can coatings into foodstuffs over extended storage periods. Food Addit Contam Part A. 35(6):1200–1213.
- Duffy E, Hearty AP, Gilsenan MB, Gibney MJ. 2006. Estimation of exposure to food packaging materials. 1: development of a food-packaging database. Food Addit Contam. 23(6):623–633.
- Egetenmeyer N, Weiss SC. 2017. Investigations for the detection of genotoxic substances on TLC plates. J Liq Chromatogr Relat Technol. 40(2):69–74.
- Eichbaum K, Brinkmann M, Buchinger S, Reifferscheid G, Hecker M, Giesy JP, Engwall M, van Bavel B, Hollert H. 2014. In vitro bioassays for detecting dioxin-like activity–application potentials and limits of detection, a review. Sci Total Environ. 487:37–48.
- Eisenbrand G, Pool-Zobel B, Baker V, Balls M, Blaauboer BJ, Boobis A, Carere A, Kevekordes S, Lhuguenot JC, Pieters R, et al. 2002. Methods of in vitro toxicology. Food Chem Toxicol. 40(2–3):193–236.
- Ekins R, Edwards P. 1997. On the meaning of “sensitivity”. Clin Chem. 43(10):1824–1831.
- Elassouli SM, Alqahtani MH, Milaat W. 2007. Genotoxicity of air borne particulates assessed by comet and the Salmonella mutagenicity test in Jeddah, Saudi Arabia. Int J Environ Res Public Health. 4(3):216–233.
- Eliopoulos P, Mourelatos D, Dozi-Vassiliades J. 1995. Comparative study on Salmonella mutagenicity and on cytogenetic and antineoplastic effects induced by cyclophosphamide and 3-aminobenzamide in cells of three transplantable tumours in vivo. Mutat Res. 342(3–4):141–146.
- Escher BI, Neale PA, Leusch FD. 2015. Effect-based trigger values for in vitro bioassays: reading across from existing water quality guideline values. Water Res. 81:137–148.
- Escobar PA, Kemper RA, Tarca J, Nicolette J, Kenyon M, Glowienke S, Sawant SG, Christensen J, Johnson TE, McKnight C, et al. 2013. Bacterial mutagenicity screening in the pharmaceutical industry. Mutat Res. 752(2):99–118.
- [EFSA] European Food Safety Authority. 2009. Guidance on safety assessment of botanicals and botanical preparations intended for use as ingredients in food supplements, on request of EFSA. Efsa J. 7(9).
- [ECHA] European Chemicals Agency. 2016. Guidance on information requirements and chemical safety assessment. Part D: Framework for exposure assessment.
- [ECHA] European Chemicals Agency. 2017 July. Guidance on information requirements and chemical safety assessment chapter R.7a: endpoint specific guidance. Version 6.0.
- European Commission. 2011. Commission regulation (EU) No 10/2011 of 14 January 2011 on plastic materials and articles intended to come into contact with food. E. C. (EC). Off J Eur Union. L12:50–89.
- [EFSA] European Food Safety Authority Scientific Committee. 2011. Scientific opinion on genotoxicity testing strategies applicable to food and feed safety assessment. Efsa J. 9(9):2379.
- [EFSA] European Food Safety Authority Scientific Committee. 2012. Scientific opinion on 'Statement on the applicability of the Margin of Exposure approach for the safety assessment of impurities which are both genotoxic and carcinogenic in substances added to food/feed'. EFSA Journal 10(3):2578.
- [EFSA] European Food Safety Authority. 2016. Guidance on the preparation and presentation of an application for authorisation of a novel food in the context of Regulation (EU) 2015/2283. EFSA J. 7(9):1249.
- [EFSA CEP Panel] European Food Safety Authority Panel on Food Contact Materials, Enzymes, Flavourings and Processing Aids. 2016. Recent developments in the risk assessment of chemicals in food and their potential impact on the safety assessment of substances used in food contact materials. EFSA J. 14(11):4594.
- [EFSA] European Food Safety Authority and [WHO] World Health Organisation. 2016. Review of the Threshold of Toxicological Concern (TTC) approach and development of new TTC decision tree. EFSA Supporting Publ. 2016:EN–1006.
- European Parliament. 2016. Report on the implementation of the food contact materials regulation ((EC) no 1935/2004) (with the cooperation of Christel Schaldemose). Committee on the Environment, Public Health and Food Safety 2016.
- [FDA] Food and Drug Administration. 2016. Use of international standard ISO 10993-1, “Biological evaluation of medical devices – part 1: evaluation and testing within a risk management process”. Guidance for Industry and Food and Drug Administration Staff. C. f. D. a. R. Health.
- Fluckiger-Isler S, Kamber M. 2012. Direct comparison of the Ames microplate format (MPF) test in liquid medium with the standard Ames pre-incubation assay on agar plates by use of equivocal to weakly positive test compounds. Mutat Res. 747(1):36–45.
- Franz R, Welle F. 2008. Migration measurement and modelling from poly(ethylene terephthalate) (PET) into soft drinks and fruit juices in comparison with food simulants. Food Addit Contam Part A. 25(8):1033–1046.
- Green MH, Muriel WJ, Bridges BA. 1976. Use of a simplified fluctuation test to detect low levels of mutagens. Mutat Res. 38(1):33–42.
- Grob K, Biedermann M, Scherbaum E, Roth M, Rieger K. 2006. Food contamination with organic materials in perspective: packaging materials as the largest and least controlled source? A view focusing on the European situation. Crit Rev Food Sci Nutr. 46(7):529–535.
- Groh KJ, Muncke J. 2017. In vitro toxicity testing of food contact materials: state-of-the-art and future challenges. Compr Rev Food Sci Food Saf. 16(5):1123–1150.
- Guidance from the Committee on Mutagenicity of Chemicals in Food, Consumer Products and the Environment (COM). 2010. Assessment of thresholds for in vivo mutagens. Published 1 April 2010.
- Gupta RS, Singh B. 1982. Mutagenic responses of five independent genetic loci in CHO cells to a variety of mutagens. Development and characteristics of a mutagen screening system based on selection for multiple drug-resistant markers. Mutat Res. 94(2):449–466.
- Hakura A, Mochida H, Yamatsu K. 1993. Dimethyl sulfoxide (DMSO) is mutagenic for bacterial mutagenicity tester strains. Mutat Res Lett. 303(3):127–133.
- Hakura A, Sugihara T, Hori Y, Uchida K, Sawada S, Suganuma A, Aoki T, Tsukidate K. 2010. Cytotoxic effect of dimethyl sulfoxide in the Ames test. Genes Environ. 32(1):1–6.
- Hakura A, Suzuki S, Sawada S, Sugihara T, Hori Y, Uchida K, Kerns WD, Sagami F, Motooka S, Satoh T. 2003. Use of human liver S9 in the Ames test: assay of three procarcinogens using human S9 derived from multiple donors. Regul Toxicol Pharmacol. 37(1):20–27.
- Hardy A, Benford D, Halldorsson T, Jeger MJ, Knutsen HK, More S, Naegeli H, Noteborn H, Ockleford C, Ricci A, et al. 2017. Guidance on the use of the weight of evidence approach in scientific assessments. EFSA Journal. 15(8):e0497.
- Hartung T, Bremer S, Casati S, Coecke S, Corvi R, Fortaner S, Gribaldo L, Halder M, Hoffmann S, Roi AJ, et al. 2004. A modular approach to the ECVAM principles on test validity. Altern Lab Anim. 32(5):467–472.
- Honkalampi-Hamalainen U, Bradley EL, Castle L, Severin I, Dahbi L, Dahlman O, Lhuguenot JC, Andersson MA, Hakulinen P, Hoornstra D, et al. 2010. Safety evaluation of food contact paper and board using chemical tests and in vitro bioassays: role of known and unknown substances. Food Addit Contam Part A. 27(3):406–415.
- Honma M, Kitazawa A, Cayley A, Williams RV, Barber C, Hanser T, Saiakhov R, Chakravarti S, Myatt GJ, Cross KP, et al. 2019. Improvement of quantitative structure-activity relationship (QSAR) tools for predicting Ames mutagenicity: outcomes of the Ames/QSAR international challenge project. Mutagenesis. 34(1):3–16.
- Hughes C, Rabinowitz A, Tate M, Birrell L, Allsup J, Billinton N, Walmsley RM. 2012. Development of a high-throughput Gaussia luciferase reporter assay for the activation of the GADD45a gene by mutagens, promutagens, clastogens, and aneugens. J Biomol Screen. 17(10):1302–1315.
- ICH 2017. Harmonised guidelines. Assessment and control of DNA reactive (mutagenic) impurities in pharmaceuticals to limit potential carcinogenic risk. March 2017.
- Jia A, Escher BI, Leusch FD, Tang JY, Prochazka E, Dong B, Snyder EM, Snyder SA. 2015. In vitro bioassays to evaluate complex chemical mixtures in recycled water. Water Res. 80:1–11.
- Jongen WM, Alink GM, Koeman JH. 1978. Mutagenic effect of dichloromethane on Salmonella typhimurium. Mutat Res. 56(3):245–248.
- Kaden DA, Hites RA, Thilly WG. 1979. Mutagenicity of soot and associated polycyclic aromatic hydrocarbons to salmonella typhimurium. Cancer Res. 39(10):4152–4159.
- Kado NY, Langley D, Eisenstadt E. 1983. A simple modification of the salmonella liquid-incubation assay. Increased sensitivity for detecting mutagens in human urine. Mutat Res. 121(1):25–32.
- Kawaguchi S, Nakamura T, Yamamoto A, Honda G, Sasaki YF. 2010. Is the comet assay a sensitive procedure for detecting genotoxicity? J Nucleic Acids. 2010:541050.
- Kenyon MO, Cheung JR, Dobo KL, Ku WW. 2007. An evaluation of the sensitivity of the Ames assay to discern low-level mutagenic impurities. Regul Toxicol Pharmacol. 48(1):75–86.
- Kirkland D, Kasper P, Martus HJ, Müller L, van Benthem J, Madia F, Corvi R. 2016. Updated recommended lists of genotoxic and non-genotoxic chemicals for assessment of the performance of new or improved genotoxicity tests. Mutat Res Genet Toxicol Environ Mutagen. 795:7–30.
- Kirkland D, Pfuhler S, Tweats D, Aardema M, Corvi R, Darroudi F, Elhajouji A, Glatt H, Hastwell P, Hayashi M, et al. 2007. How to reduce false positive results when undertaking in vitro genotoxicity testing and thus avoid unnecessary follow-up animal tests: report of an ECVAM workshop. Mutat Res. 628(1):31–55.
- Kirkland D, Reeve L, Gatehouse D, Vanparys P. 2011. A core in vitro genotoxicity battery comprising the Ames test plus the in vitro micronucleus test is sufficient to detect rodent carcinogens and in vivo genotoxins. Mutat Res. 721(1):27–73.
- Klingelhofer I, Morlock GE. 2015. Bioprofiling of surface/wastewater and bioquantitation of discovered endocrine-active compounds by streamlined direct bioautography. Anal Chem. 87(21):11098–11104.
- Koster S, Bani-Estivals M-H, Bonuomo M, Bradley E, Chagnon MC, Garcia ML, Godts F, Gude T, Helling R, Paseiro-Losada P, et al. 2015a. Guidance on best practices on the risk assessment of non-intentionally added substances (NIAS) in food contact materials and articles. ILSI Europe Report Series: 1–70.
- Koster S, Boobis AR, Cubberley R, Hollnagel HM, Richling E, Wildemann T, Würtzen G, Galli CL. 2011. Application of the TTC concept to unknown substances found in analysis of foods. Food Chem Toxicol. 49(8):1643–1660.
- Koster S, Leeman W, Verheij E, Dutman E, van Stee L, Nielsen LM, Ronsmans S, Noteborn H, Krul L. 2015b. A novel safety assessment strategy applied to non-selective extracts. Food Chem Toxicol. 80:163–181.
- Koster S, Rennen M, Leeman W, Houben G, Muilwijk B, van Acker F, Krul L. 2014. A novel safety assessment strategy for non-intentionally added substances (NIAS) in carton food contact materials. Food Addit Contam Part A. 31(3):422–443.
- Kroes R, Renwick AG, Cheeseman M, Kleiner J, Mangelsdorf I, Piersma A, Schilter B, Schlatter J, van Schothorst F, Vos JG, et al. 2004. Structure-based thresholds of toxicological concern (TTC): guidance for application to substances present at low levels in the diet. Food Chem Toxicol. 42(1):65–83.
- Leeman WR, Krul L, Houben GF. 2013. Complex mixtures: relevance of combined exposure to substances at low dose levels. Food Chem Toxicol. 58:141–148.
- Luijten M, Olthof ED, Hakkert BC, Rorije E, van der Laan JW, Woutersen RA, van Benthem J. 2016. An integrative test strategy for cancer hazard identification. Crit Rev Toxicol. 46(7):615–639.
- Moraa KA. 2015. Investigation of mutagenicity of solar disinfected (SODIS) water stored in plastic bottles [dissertation]. Nairobi (KE): University of Nairobi.
- Maisanaba S, Pichardo S, Jorda-Beneyto M, Aucejo S, Cameán AM, Jos Á. 2014. Cytotoxicity and mutagenicity studies on migration extracts from nanocomposites with potential use in food packaging. Food Chem Toxicol. 66:366–372.
- Manganelli S, Schilter B, Benfenati E, Manganaro A, Lo Piparo E. 2018. Integrated strategy for mutagenicity prediction applied to food contact chemicals. Altex. 35(2):169–178.
- Maron D, Katzenellenbogen J, Ames BN. 1981. Compatibility of organic solvents with the salmonella/microsome test. Mutat Res. 88(4):343–350.
- McCann J, Gold LS, Horn L, McGill R, Graedel TE, Kaldor J. 1988. Statistical analysis of salmonella test data and comparison to results of animal cancer tests. Mutat Res. 205(1–4):183–195.
- McCombie G, Hötzer K, Daniel J, Biedermann M, Eicher A, Grob K. 2016. Compliance work for polyolefins in food contact: results of an official control campaign. Food Control. 59:793–800.
- McDaniels AE, Reyes AL, Wymer LJ, Rankin CC, Stelma GN Jr. 1990. Comparison of the salmonella (Ames) test, umu tests, and the SOS chromotests for detecting genotoxins. Environ Mol Mutagen. 16(3):204–215.
- Mittag N, Förster S, Hoppe S, Simat TJ, Sehr A, Renz S, Vobach M, Kammann U. 2006. Bioassay guided analysis of migrants from can coatings. J Für Verbraucherschutz Und Lebensmittelsicherheit. 1(4):345–353.
- Monarca S, De Fusco R, Biscardi D, De Feo V, Pasquini R, Fatigoni C, Moretti M, Zanardini A. 1994. Studies of migration of potentially genotoxic compounds into water stored in PET bottles. Food Chem Toxicol. 32(9):783–788.
- More S, Bampidis V, Boesten J, Benford D, Bragard C, Halldorsson T, Hernandez‐Jerez A, Hougaard‐Bennekou S, Koutsoumanis K, Nielsen SS, et al. 2019. Genotoxicity assessment of chemical mixtures. EFSA Scientific Committee. Efsa J. 17(1):e05519.
- Morlock GE, Klingelhöfer I. 2014. Liquid chromatography-bioassay-mass spectrometry for profiling of physiologically active food. Anal Chem. 86(16):8289–8295.
- Mortelmans K, Zeiger E. 2000. The Ames Salmonella/microsome mutagenicity assay. Mutat Res. 455(1–2):29–60.
- Muller L, Blakey D, Dearfield KL, Galloway S, Guzzie P, Hayashi M, Kasper P, Kirkland D, MacGregor JT, Parry JM, et al. 2003. Strategy for genotoxicity testing and stratification of genotoxicity test results – report on initial activities of the IWGT expert group. Mutat Res. 540(2):177–181.
- Muncke J. 2009. Exposure to endocrine disrupting compounds via the food chain: is packaging a relevant source? Sci Total Environ. 407(16):4549–4559.
- Nakai M, Tsubokura M, Suzuki M, Fujishima S, Watanabe Y, Hasegawa Y, Oyama K, Ogura S. 2014. Genotoxicity of styrene oligomers extracted from polystyrene intended for use in contact with food. Toxicol Rep. 1:1175–1180.
- O’Neill JP, Machanoff R, San Sebastian JR, Hsie AW. 1982. Cytotoxicity and mutagenicity of dimethylnitrosamine in mammalian cells (CHO/HGPRT system); enhancement by calcium phosphate. Environ Mutagen. 4(1):7–18.
- Oberly TJ, Bewsey BJ, Probst GS. 1984. An evaluation of the L5178Y TK± mouse lymphoma forward mutation assay using 42 chemicals. Mutat Res. 125(2):291–306.
- Oberly TJ, Huffman DM, Scheuring JC, Garriott ML. 1993. An evaluation of 6 chromosomal mutagens in the AS52/XPRT mutation assay utilizing suspension culture and soft agar cloning. Mutat Res/Genet Toxicol. 319(3):179–187.
- Ohe T, Watanabe T, Wakabayashi K. 2004. Mutagens in surface waters: a review. Mutat Res. 567(2–3):109–149.
- Phillips BJ, Kranz E, Elias PS. 1980. An investigation of the genetic toxicology of irradiated foodstuffs using short-term test systems—II. Sister chromatid exchange and mutation assays in cultured Chinese hamster ovary cells. Food Cosmet Toxicol. 18(5):471–475.
- Proudlock R, Evans K. 2016. The micro-Ames test: a direct comparison of the performance and sensitivities of the standard and 24-well plate versions of the bacterial mutation test. Environ Mol Mutagen. 57(9):687–705.
- Rainer B, Mayrhofer E, Redl M, Dolak I, Mislivececk D, Czerny T, Kirchnawy C, Marin-Kuan M, Schilter B, Tacker M. 2019. An optimized test procedure to assess mutagenicity of food contact materials with the Ames MPF assay. Food Additives And Contaminants. 36(9):1419–1432.
- Rainer B, Pinter E, Czerny T, Riegel E, Kirchnawy C, Marin-Kuan M, Schilter B, Tacker M. 2018. Suitability of the Ames test to characterise genotoxicity of food contact material migrates. Food Addit Contam Part A. 35(11):2230–2243.
- Rennen MA, Koster S, Krul CA, Houben GF. 2011. Application of the threshold of toxicological concern (TTC) concept to the safety assessment of chemically complex food matrices. Food Chem Toxicol. 49(4):933–940.
- Schilter B, Benigni R, Boobis A, Chiodini A, Cockburn A, Cronin MT, Lo Piparo E, Modi S, Thiel A, Worth A. 2014. Establishing the level of safety concern for chemicals in food without the need for toxicity testing. Regul Toxicol Pharmacol. 68(2):275–296.
- Schilter B, Fussell K, Mollergues J, Latado H, Marin-Kuan M. 2018. In vitro toxicology, biodetection and risk assessment. Trends Tech Sci Res. 1:2.
- Schriks M, Baken K, Simon E, Besselink H, van der Linden S, Kienle C, van der Burg B 2015. Selection criteria to select in vitro bioassays for implementation and use. Deliverable report D41.2. EU FP7 project DEMEAU.
- Schug TT, Abagyan R, Blumberg B, Collins TJ, Crews D, DeFur PL, Dickerson SM, Edwards TM, Gore AC, Guillette LJ, et al. 2013. Designing endocrine disruption out of the next generation of chemicals. Green Chem. 15(1):181–198.
- Seiler A, Bach A, Driffield M, Paseiro Losada P, Mercea P, Tosa V, Franz R. 2014. Correlation of foodstuffs with ethanol-water mixtures with regard to the solubility of migrants from food contact materials. Food Addit Contam Part A. 31(3):498–511.
- Severin I, Lionti K, Dahbi L, Loriot C, Toury B, Chagnon MC. 2016. In vitro toxicity assessment of extracts derived from sol-gel coatings on polycarbonate intended to be used in food contact applications. Food Chem Toxicol. 93:51–57.
- Severin I, Souton E, Dahbi L, Chagnon MC. 2017. Use of bioassays to assess hazard of food contact material extracts: state of the art. Food Chem Toxicol. 105:429–447.
- Silano V, Bolognesi C, Castle L, Cravedi J-P, Engel K-H, Fowler P, Franz R, Grob K, Gürtler R, Husøy T, et al. 2008. Note for guidance for the preparation of an application for the safety assessment of a substance to be used in plastic food contact materials. EFSA panel on food contact materials, enzymes, flavourings and processing aids (CEP). Efsa J. 6(7):21r.
- Smith KE, Heringa MB, Uytewaal M, Mayer P. 2013. The dosing determines mutagenicity of hydrophobic compounds in the Ames II assay with metabolic transformation: passive dosing versus solvent spiking. Mutat Res. 750(1–2):12–18.
- Souza-Silva ÉA, Gionfriddo E, Pawliszyn J. 2015. A critical review of the state of the art of solid-phase microextraction of complex matrices II. Food analysis. TrAC Trends Anal Chem. 71:236–248.
- Speit G, Hartmann A. 1995. The contribution of excision repair to the DNA effects seen in the alkaline single cell gel test (comet assay). Mutagenesis. 10(6):555–559.
- Standardization ECf. 2002. Materials and articles in contact with foodstuffs – plastics – part 1: guide to the selection of conditions and test methods for overall migration. European standard, EN 1186-1.
- Störmer A, Franz R. 2009. MIGRESIVES: a research project on migration from adhesives in food-packaging materials in support of European legislation and standardization. Food Addit Contam Part A. 26(12):1581–1591.
- Subramanian MN. 2013. Plastic additives and testing. Hoboken: Scrivener Publishing, Wiley.
- Takagi, Y., Nakajima, D., Chengjun, S., Goto, S. 2011. Chapter 9: Application of the ultramicro forward-mutation assay to the monitoring of indoor and outdoor air mutagenicity – examples of Chengdu City and Tokyo. In: Moldoveanu A. editor. Advanced Topics in Environmental Health and Air Pollution Case Studies. Rijeka: InTech Publisher; p. 20.
- Tang Z-M, Wang Z-Y, Kang J-W. 2007. Screening of acetylcholinesterase inhibitors in natural extracts by CE with electrophoretically mediated microanalysis technique. Electrophoresis. 28(3):360–365.
- Thompson LH, Salazar EP, Brookman KW, Hoy CA. 1983. Hypersensitivity to cell killing and mutation induction by chemical carcinogens in an excision repair-deficient mutant of CHO cells. Mutat Res/DNA Repair Rep. 112(6):329–344.
- Thybaud V, Aardema M, Casciano D, Dellarco V, Embry MR, Gollapudi BB, Hayashi M, Holsapple MP, Jacobson-Kram D, Kasper P, et al. 2007a. Relevance and follow-up of positive results in in vitro genetic toxicity assays: an ILSI-HESI initiative. Mutat Res. 633(2):67–79.
- Thybaud V, Le Fevre AC, Boitier E. 2007b. Application of toxicogenomics to genetic toxicology risk assessment. Environ Mol Mutagen. 48(5):369–379.
- Ubomba-Jaswa E, Fernandez-Ibanez P, McGuigan KG. 2010. A preliminary Ames fluctuation assay assessment of the genotoxicity of drinking water that has been solar disinfected in polyethylene terephthalate (pet) bottles. J Water Health. 8(4):712–9.
- Ubomba-Jaswa E, Fernandez-Ibanez P, McGuigan KG. 2010. A preliminary Ames fluctuation assay assessment of the genotoxicity of drinking water that has been solar disinfected in polyethylene terephthalate (PET) bottles. J Water Health. 8(4):712–719.
- Valentin-Severin I, Le Hegarat L, Lhuguenot JC, Le Bon AM, Chagnon MC. 2003. Use of HepG2 cell line for direct or indirect mutagens screening: comparative investigation between comet and micronucleus assays. Mutat Res. 536(1–2):79–90.
- van der Burg B, Winter R, Man HY, Vangenechten C, Berckmans P, Weimer M, Witters H, van der Linden S. 2010a. Optimization and prevalidation of the in vitro AR CALUX method to test androgenic and antiandrogenic activity of compounds. Reprod Toxicol. 30(1):18–24.
- van der Burg B, Winter R, Weimer M, Berckmans P, Suzuki G, Gijsbers L, Jonas A, van der Linden S, Witters H, Aarts J, et al. 2010b. Optimization and prevalidation of the in vitro ERalpha CALUX method to test estrogenic and antiestrogenic activity of compounds. Reprod Toxicol. 30(1):73–80.
- Wambaugh JF, Hughes MF, Ring CL, MacMillan DK, Ford J, Fennell TR, Black SR, Snyder RW, Sipes NS, Wetmore BA, et al. 2018. Evaluating in vitro-in vivo extrapolation of toxicokinetics. Toxicol Sci. 163(1):152–169.
- Wangenheim J, Bolcsfoldi G. 1988. Mouse lymphoma L5178Y thymidine kinase locus assay of 50 compounds. Mutagenesis. 3(3):193–205.
- Watanabe T, Kohan MJ, Walsh D, Ball LM, DeMarini DM, Lewtas J. 1995. Mutagenicity of nitrodibenzopyranones in the Salmonella plate-incorporation and microsuspension assays. Mutat Res. 345(1–2):1–9.
- Wegrzyn G, Czyz A. 2003. Detection of mutagenic pollution of natural environment using microbiological assays. J Appl Microbiol. 95(6):1175–1181.
- Weiss SC, Egetenmeyer N, Schulz W. 2017. Coupling of in vitro bioassays with planar chromatography in effect-directed analysis. Adv Biochem Eng Biotechnol. 157:187–224.
- White PA, Claxton LD. 2004. Mutagens in contaminated soil: a review. Mutat Res. 567(2–3):227–345.
- Zamora PO, Benson JM, Marshall TC, Mokler BV, Li AP, Dahl AR, Brooks AL, McClellan RO. 1983. Cytotoxicity and mutagenicity of vapor-phase pollutants in rat lung epithelial cells and Chinese hamster ovary cells grown on collagen gels. J Toxicol Environ Health. 12(1):27–38.
- Zwarg J, Morales DA, Maselli BS, Brack W, Umbuzeiro GA. 2018. Miniaturization of the microsuspension salmonella/microsome assay in agar microplates. Environ Mol Mutagen. 59(6):488–501.