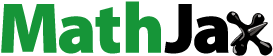
ABSTRACT
A physiologically based pharmacokinetic (PBPK) model was developed to investigate the production-specific factors involved in the transfer of α-hexabromocyclododecane (α-HBCDD) to broiler meat. The model describes growth and lipid deposition in tissues of fast- (FG) and slow- (SG) growing broilers from hatching to slaughter and simulates the exposure through the ingestion of contaminated feed or expanded polystyrene insulation material. Growth parameters were obtained from the literature while parameters relative to uptake, distribution, and elimination of α-HBCDD were adjusted using results of a previous experiment involving broilers exposed through feed throughout the rearing period or allowed to depurate before slaughter. The model was used to compare the two main edible tissues, breast and leg meat, as well as skin, and to investigate the variability within strain. Between strains and within strain, α-HBCDD assimilation efficiency (AE) is higher when the animals are slaughtered young or heavy. However, increasing slaughter age will lower α-HBCDD concentration in tissues, due to dilution. Based on fresh weight, the concentration of α-HBCDD in breast muscles and skin tends to be lower in SG than in FG broilers (−30 to +10%), while it is 10% to 80% higher in leg muscles. Compared to breast muscles, consuming leg muscles would elicit an exposure 9 and 16 times higher in FG and SG broilers, respectively. The consumption of skin together with muscles would multiply the exposure by up to 36 times compared to breast muscle alone. In case of acute exposure, the α-HBCDD concentration in tissues increased sharply, all the more since the animals are lighter in weight, and then decreased rapidly. In FG broilers, dilution through growth contributed for up to 37%, 28% and 97% to the decontamination of breast muscles, leg muscles and skin, respectively, depending on the duration of depuration before slaughter.
Introduction
Hexabromocyclododecane (HBCDD) is a brominated aliphatic cyclic hydrocarbon with three main isomers α, β and γ (representing 1–12%, 10–13% and 75–89% of the “technical” HBCDD, respectively). This compound is used as a flame retardant additive to reduce ignition in thermal insulation materials and textiles (Marvin et al. Citation2011; Koch et al. Citation2015). Due to its bioaccumulative, persistent and toxic characteristics, HBCDD has been listed in Annexe A of the Stockholm Convention on Persistent Organic Pollutants in November 2014, resulting in a mid-term ban on its manufacture and use. In Europe, the main application of HBCDD (around 80%) has been in extruded (XPS) and expanded (EPS) polystyrene (PS) used in building and construction (ECHA, Citation2009), which remains the only use exempted in the Stockholm Convention. Thus, as the life span of polystyrene foam insulation in buildings is estimated to be 30 to 50 years and as the use of HBCDD in EPS and XPS began in the 1980s, the release of HBCDD into the environment is expected to continue for a long time and even increase from 2025 onwards (Li et al. Citation2016).
Due to its lipophilicity, food and especially fatty food of animal origin is the main route of human exposure to HBCDD (Marvin et al. Citation2011; Koch et al. Citation2015). Overall levels of HBCDD concentrations in animal-derived foodstuffs, below 1 ng g−1 lipid weight (lw), may be considered acceptable, and the median chronic dietary exposure of 0.43 ng kg−1 d−1 in European adults is deemed to be of no public health concern (EFSA Citation2011). This compound is recognised to induce endocrine and behavioural effects in animals but its effects on human health are still not fully elucidated (Lyche et al. Citation2015). Nevertheless, human exposure assessment is still ongoing. Moreover, the level of food contamination still requires investigations (Venisseau et al. Citation2018).
In this respect, as a major meat consumed worldwide, the quality of broiler meat is worth investigation. As pointed out by several authors, the α-isomer predominates in the environment, animal tissues and foodstuffs (Marvin et al. Citation2011; Koch et al. Citation2015). Thus, this isomer dominates in the profile of the matrices to which rearing animals may be exposed and of their tissues (Berntssen et al. Citation2011; Cariou et al. Citation2014). Like other meats, poultry meat displays overall low levels of HBCDD (Riviere et al. Citation2014; Dervilly-Pinel et al. Citation2017). However, national monitoring plans conducted in France have revealed concentrations in some samples of poultry eggs and meat reaching 3,000 ng g−1 lw (DGAL Citation2009). Such high levels are generally ascribed to the ingestion of fragments of XPS used for insulating hen houses (Hiebl and Vetter Citation2007; Jondreville et al. Citation2017a). Besides this accidental contamination, variability in broiler meat HBCDD concentration may arise from the variability of production systems (breed, animal performance, outdoor access, etc.). Indeed, in addition to fast-growing (FG) broilers, which are an international standard, consumers demand products from alternative systems such as Label Rouge or organic systems involving slow-growing (SG) animals having access to outdoor runs. SG broilers are raised twice as long as FG broilers, they ingest more feed for an often lower market weight, resulting in a lower feed efficiency, and display different tissue growths and lipid content (Quentin et al. Citation2003). A recent survey conducted in France reported similar average concentrations of HBCDD in conventional, Label Rouge and organic breast meat samples (0.21 ± 0.14, 0.63 ± 0.80, and 0.47 ± 0.40 ng HBCDD g−1 lw, respectively). However, the median concentration was slightly higher for alternative systems (respectively, 0.17, 0.34 and 0.33 ng HBCDD g−1 lw) (Dervilly-Pinel et al. Citation2017). In addition to the access to an outdoor run, the aforementioned production-specific factors may affect the concentration of α-HBCDD in tissues of exposed broilers. The rearing duration is longer and the buildings are very often older compared to those used for FG chickens, possibly allowing animal access to insulation materials. The exposure risk for consumers could be therefore higher with SG chickens compared to FG chickens. Indeed, in an experiment involving broilers exposed through feeds with similar α-HBCDD concentrations, Jondreville et al. (Citation2017b) observed slightly higher concentrations in the tissues of SG compared to FG broilers at market age. This experiment also revealed that, even expressed on lipids, the concentration of α-HBCDD in leg muscles was 2.8 times higher than in breast muscles, although they are both referred to as ‘meat’. Nevertheless, this single experiment does not allow assessment of the impact of several factors, such as the duration of exposure or the variability within each production type of age and body weight at slaughter, on the concentration of α-HBCDD in tissues at market age.
As physiologically based pharmacokinetic (PBPK) modelling is a relevant tool for incorporating information on the various production-specific factors, we developed a PBPK model allowing simulation of the fate of ingested α-HBCDD in FG and SG broilers. Once the model was calibrated and validated using the results of the aforementioned studies (Omer et al. Citation2017; Jondreville et al. Citation2017b), it was used to estimate the α-HBCDD concentration in meat (breast and leg muscles) and skin according to different scenarios of exposure and rearing characteristics.
Materials and methods
Model description
Model overview
The PBPK model allows the description of time-dependent growth and lipid deposition in tissues of FG and SG broilers from birth to slaughter. The considered compartments are the main edible muscles (Breast and Leg), the fatty tissues which play a role in α-HBCDD storage (Skin and separable fat depots, SFD), Liver, which is involved in α-HBCDD metabolism, Blood, which distributes α-HBCDD to tissues and the rest of the body referred to as ‘Other tissues’ (). Ingested α-HBCDD enters the body through intestinal absorption and is directed to the liver, where it is partly metabolised and subsequently eliminated. The distribution of α-HBCDD is limited by blood perfusion in all tissues except in fatty tissues (Skin and SFD), which are described with two sub-compartments, one being perfused and the other described as a diffusion-limited one (). In all the simulations, α-HBCDD is ingested as a racemic mixture (Omer et al. Citation2017), and the fate of α-HBCDD in tissues thus refers to the sum of the two enantiomers (+) and (−). All model parameters were considered to be identical for both enantiomers, except for the rate of hepatic metabolism, for which two specific intrinsic clearance values were considered. The PBPK model was implemented using Python™ version 2.7 (www.python.org) using “numpy”, “matplotlib”, “math”, and “csv” packages. Full code of the model is available from the corresponding author on request.
Figure 1. Simplified representation of the PBPK model simulating the absorption/metabolism/elimination (solid grey), blood distribution (solid black), direct uptake from liver through lipid deposition (dotted black), and passive diffusion in tissues (dotted grey) of α-HBCDD in growing broilers.
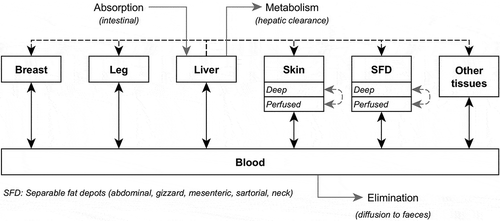
The parameterisation of the model for growth and lipid deposition, as well as tissue perfusion, is based on a literature review presented in Supplemental Material S1. For each strain (FG and SG), males (M), females (F) and as hatched (M + F) broilers are considered separately. The main features of the model are presented below and the list of parameters is presented in .
Table 1. Parameters of the model for fast-(FG) and slow-(SG) growing broilers. In brackets, parameter values after optimisation.
Bodyweight, feed ingestion, tissues’ growth and lipid deposition
Bodyweight (BW, kg) follows a modified Gompertz function (Méda et al. Citation2014), based on two pairs of initial and final BW (kg) and Age (d) pairs (Age1/BW1 and Age2/BW2, respectively) and on a precocity parameter (Bgomp, d−1) describing the shape of the growth curve (1). Ages and body weights at hatching and slaughter were taken from data provided by breeders for Cobb 500 (Cobb-Vantress Citation2007) and JA 657 (Willems Citation2017) for FG and SG broilers, respectively, but can be modified to simulate different growth trajectories. Bgomp was adjusted using those data as well. The amount of feed ingested daily (D Feed Ing, kg) is related to BW according to an exponential relationship (2), whose parameters were adjusted using the same data as for BW.
The weight of the liver, the blood and the muscles of breast and of leg (W tissue, kg) (3), as well the weight of body lipids (W BL, kg) (4) are allometric functions of BW. Storage fat combines Skin and SFD (i.e. abdominal, mesenteric, sartorial, neck, gizzard fat depots; Crespo and Esteve-Garcia Citation2002). Unlike other tissues, the weight of storage fat (W storagefat, kg) is related to W BL (5). The contribution of skin to storage fat (prop Skin) is considered constant (66%), and consequently so is the contribution of SFD. The weight of the ‘Other tissues’ compartment (W other, kg) is the difference between BW and the sum of weights of aforementioned tissues.
The lipid dynamics is then described as follows. For each tissue, the corresponding lipid weight is given by the multiplication of tissue weight by its lipid concentration (Tissue lipid conc, kg kg−1) (6), except for the weight of lipids in the ‘Other tissues’ compartment, calculated as the difference between W BL and the sum of the weights of lipids in each of the depicted tissues (7). The concentration of lipids is constant in all tissues except in SFD, for which the daily deposition (W SFD gain, kg d−1) linearly increases in lipid concentration from an initial value (SFD gain lipid conc ini, kg kg−1) to a constant value (SFD gain lipid conc fin, kg kg−1) reached at the age of 14 and 28 days (SFD lipid conc age, d) in FG and SG broilers, respectively (8). The concentration of neutral lipids (sum of triglycerides and esterified cholesterol) in each tissue (Tissue neut lipid conc, kg kg−1) is proportional to its lipid concentration, and the proportion of neutral lipids in each tissue lipids (a tissue neut lipid) is constant (9).
Ingestion, absorption, distribution and elimination of α-HBCDD
Broilers may be exposed to α-HBCDD through feed with a given α-HBCDD concentration (Diet HBCDD conc, µg kg−1) and/or through accidental ingestion of insulated material such as XPS (NonDiet HBCDD intake, µg d−1). The amount of HBCDD daily absorbed (HBCDD abs, µg d−1) is the product of the amount ingested and the absorption coefficient (HBCDD abs coef), set at 0.9 following the results obtained in mouse by Szabo et al. (Citation2011) (10).
The distribution of α-HBCDD is governed by the tissue irrigation and the partition coefficient between plasma and the tissue (Kp tissue, L kg−1). Cardiac blood flow is an allometric relationship of BW (Dzialowsli and Crossley Citation2015) and cardiac plasma flow (TQ, L d−1) is calculated accounting for haematocrit (Ht) (11). The daily plasma flow to each tissue (Q tissue, L d−1) is the product of its weight (W tissue, kg) by a specific perfusion rate (Tissue perf, L d−1 kg−1) (12), except in ‘Other tissues’ compartment, where it is the difference between cardiac plasma flow and the sum of the flows to each depicted tissue (13).
Since α-HBCDD is a lipophilic and non-polar compound, Kp tissue is assumed to be equal to the ratio of neutral lipids concentration in the tissue to their concentration in plasma (Haddad et al. Citation2000) (14). The net flow of α-HBCDD from the plasma to each tissue (HBCDD plasma tissue, μg d−1) is the difference between its arterial and venous flows (15).
In addition to this perfusion-limited distribution, a distribution limited by diffusion is represented in Skin and SFD. Thus, each of these tissues is divided into two sub-compartments, a perfused and a deep one (Tissueperf and Tissuedeep, respectively), Prop Storagefatperf being the proportion of perfused tissue (Levitt Citation2007). The two sub-compartments exchange by passive diffusion (HBCDD TissuePerf TissueDeep, μg d−1), according to a diffusive transfer parameter (Diff Storagefat coef, d kg−1) (MacLachlan and Bhula Citation2009; MacLachlan Citation2010) (16). For a compound with a log Kow of 5.75, this parameter is set at 200 according to McLachlan (Citation1994). In addition, in order to account for the almost exclusive hepatic origin of the fatty acids accumulating in adipose tissues of broilers (Griffin et al. Citation1992), each tissue uptakes α-HBCDD directly from the liver (HBCDD tissue uptake, μg d−1) through neutral lipids deposition (i.e. W Tissue Gain × Tissue Gain neut lipid conc) (17).
As a lipophilic compound, α-HBCDD is mainly cleared through hepatic metabolism, with first-order kinetics (MacLachlan and Bhula Citation2009). Hepatic metabolic α-HBCDD flow (HBCD CL, μg d−1) is the product of the amount of α-HBCDD present in the liver (W HBCD liver, μg) and the intrinsic clearance (CLi, d−1) (18). To account for the enrichment of tissues in (−)-α-HBCDD in broilers exposed to a racemic mixture of α-HBCDD (Omer et al. Citation2017), values of 0.5 for CLip and 0.4 for CLin were ascribed to the intrinsic clearance of (+)-α-HBCDD and (−)-α-HBCDD, respectively.
Finally, a passive “return” flow from plasma to faeces (HBCDD Plasma Faeces, g d−1) was also considered (19) according to a diffusive transfer parameter (Diff Faeces coef, d kg−1) set at 200 (McLachlan Citation1994), HBCDD concentration in plasma (HBCDD Plasma conc, µg L−1), and to plasma and faeces lipid concentrations (Plasma lipid conc, kg L−1; Faeces lipid conc, kg kg−1). Faeces lipid concentration (20) is calculated using dry matter and lipid concentrations in feed (Feed DM conc and Feed lipid conc, kg kg−1), digestibilities of feed dry matter and lipids (Dig DM and Dig lipid), and dry matter content of faeces (Faeces DM conc).
Sensitivity analysis, optimisation and evaluation of the model
A local sensitivity analysis was implemented to evaluate the relative importance of some model parameters on assimilation efficiencies. The absolute value of the normalised sensitivity coefficient (NSC) was calculated to distinguish the relative importance of model parameters (21):
where p is the model parameter, Δp is the variation in the model parameter, r is the model output, and Δr is the variation in the model output. In this study, parameters varied according to different ranges selected by the expertise of the authors. A parameter was considered influential if NSC reached a minimum absolute value of 0.25 (Leavens et al. Citation2012). A positive value of NSC indicates that an increase (or decrease) in the parameter implies an increase (or decrease) of the output.
Optimisation and validation of the model were achieved by means of the results of the aforementioned experiment, which involved FG and SG broilers exposed to α-HBCDD via the ingestion of a contaminated diet (Omer et al. Citation2017; Jondreville et al. Citation2017b). Exposure lasted from hatching to slaughter at 42 and 84 days of age in FG and SG broilers, respectively. In addition, some SG broilers were offered a control non-contaminated diet from 43 to 84 days of age, allowing investigation of decontamination of the tissues. Throughout the exposure and depuration periods, the kinetics of tissue weight (liver, breast muscles, leg muscles, plasma, abdominal fat), total lipids concentrations and concentration by classes of lipids (triglycerides, free cholesterol, esterified cholesterol, phospholipids), as well as α-HBCDD concentrations were reported by Jondreville et al. (Citation2017b) while Omer et al. (Citation2017) reported the results of the isoform analyses of α-HBCDD. The observed data relative to body weight, feed ingested, tissue weight total and neutral lipid concentrations, as well as (+)-α-HBCDD and (−)-α-HBCDD concentrations in tissues were introduced in the model prior to optimisation and evaluation.
Optimisation was performed using the data collected in SG broilers exposed through feed during 42 days and decontaminated until 84 days. The optimised parameters concern the absorption of α-HBCDD (HBCDD abs coef), the elimination of α-HBCDD by hepatic metabolism (CLip, CLin, Liver Perf), the accumulation of α-HBCDD in storage fatty tissues (Skin perf, SFD perf, Dif Storagefat coef, Prop Storagefatperf) and the proportion of neutral lipids in the plasma (a plasma neut lip), which are all influenced all the Kps. The proportions of neutral lipids in the lipids of Liver, Breast and ‘Other tissues’ were also adjusted. Once these parameters were adjusted, the model was evaluated by comparing its outputs to experimental data from FG and SG broilers exposed through feed throughout their life (i.e. from hatching to slaughter).
Simulations
The outputs of the model are the concentrations of α-HBCDD in each tissue or in the entire body based on fresh weight (HBCDD Tissue conc, µg kg−1) or on lipid weight (HBCDD Tissue conc lip, µg kg−1) and the assimilation efficiency (AE), which is the accumulated amount of α-HBCDD divided by the ingested amount. When broilers are exposed through feed, the accumulation ratio of α-HBCDD in each tissue is calculated as the ratio of its concentration in the tissue to its concentration in feed. This ratio is referred to as AR Tissue and ARlip Tissue when the concentration in the tissue is based on fresh or lipid weight, respectively. Due to the short lifetime of broilers compared to the time required for the complete elimination and decontamination of α-HBCDD, the calculation of half-life is difficult. Therefore, we calculated the mean daily rate of variation of α-HBCDD burden (kw, which refers to W HBCDD tissue, µg) and concentration in tissue lipids (kc, which refers to HBCDD Tissue conc lip, µg kg−1) during decontamination. The first-rate (kw, d−1) accounts for elimination only, while the second one (kc, d−1) also accounts for the dilution of α-HBCDD through lipid deposition. The contribution of growth to decontamination is calculated as 1-kw/kc. These linear relationships greatly differ from the kinetics usually adjusted to decontamination data and do not allow estimation of elimination or decontamination half-lives. Nevertheless, they are appropriate to estimate the expected concentration in tissues at a fixed slaughter age.
The first set of simulations was aimed at comparing the two strains and the tissues within each strain for the kinetics of tissues contamination in animals exposed through feed (38 µg α-HBCDD kg−1) from hatching to slaughter. First, as hatched FG- and SG-broilers with default age and BW at slaughter (i.e. 42 days and 2.9 kg vs. 84 days and 2.2 kg) were compared (). At a second step, the two strains were compared accounting for the variability in animal performance reported in French industry statistics provided by one of the co-authors of this paper (A. Travel, ITAVI). According to these statistics, FG-broilers at slaughter are aged 36–54 days and weigh 1.8–3.4 kg, while SG-broilers are aged 81–95 days and weigh 1.9–3.5 kg; their feed efficiency (FE) ranges between 0.44 and 0.69 and between 0.26 and 0.47, respectively (). To compare the two strains, graded values of the pair Age and BW at slaughter were introduced in the model. The pairs leading to FE outside the range were discarded.
Table 2. Fast- (FG) and slow-growing (SG) broilers exposed to α-HBCDD through a contaminated feed (38 µg α-HBCDD kg−1) from hatching to slaughter: main characteristics of broilers, assimilation efficiency (AE) and accumulation ratio (AR) of α-HBCDD at slaughter.
Second set of simulations was aimed at investigating the rate of decontamination of tissues. Firstly, the two strains and the tissues within strain were compared by means of FG and SG broilers with default age and BW at slaughter exposed through feed during the first half of their life (up to 21 and 42 days, respectively) and then provided a non-contaminated feed until slaughter. Secondly, we assessed the impact of the date and duration of exposure on α-HBCDD concentration in tissues and the rate of decontamination. In this aim, the model was run with as hatched FG broilers raised during 42 days and ingesting the same total amount of 172 µg α-HBCDD either by ingesting a contaminated feed (38 µg α-HBCDD kg−1) throughout rearing (Feed) or 57.3 µg α-HBCDD daily during three consecutive days from 12 to 14 (XPS-A), 19 to 21 (XPS-B) or 26 to 28 (XPS-C) days of age. In a previous experiment, Jondreville et al. (Citation2017a) observed that laying hens may voluntary ingest far higher amounts of α-HBCDD, reaching 7500 µg daily. These hens had access to XPS of which they ingested up to 400 mg daily. With a similar XPS, broilers in the current simulations would ingest far lower amounts of 3 mg daily, which is very plausible to simulate an accidental contamination.
Results and discussion
Sensitivity analysis
Full results of sensitivity analysis are given in Supplemental Material S2. The model was found to be sensitive to various “growth” parameters including body weight at slaughter (BW2), total and neutral lipid concentrations in body and in tissues. In particular, BW2, a BL, Liver lipid conc, a Liver neut lipid, and a Storagefat neut lipid were found to influence simultaneously AE and ARlip of several tissues. This is logical as these parameters either concern body growth, liver which is the first tissue contaminated and the one “exporting” HBCDD to other tissues (through the synthesis of contaminated fatty acids) or Storagefat which can represent up to more than 50% of the lipids in body when birds are close to slaughter (data not shown). On the contrary, parameters that are specific to tissues like Breast or Leg only influence outputs for this same tissue. As expected, model outputs were found very sensitive to absorption coefficient (HBCDD abs coef) and to hepatic metabolism (i.e. intrinsic clearances Clin and Clip), with NSC about 1 and −0.28, respectively, but not sensitive to irrigation parameters (i.e. perfusion).
Optimisation and evaluation of the model
Optimisation
The optimised parameters are presented in . The optimised perfusion rates of Skin, SFD and Liver are in the range of literature data (Supplemental Material S1). Similarly, the optimised coefficient of diffusion of α-HBCDD within storage fat (Diff Storagefat coef) is consistent with literature data for lipophilic compounds (McLachlan Citation1994) as well as its absorption coefficient (Kelly et al. Citation2004). The optimisation of the proportion of neutral lipids in lipids of Breast, Liver and Other tissues, and most of all, of Plasma (a tissue neut lip) challenges the hypothesis of the diffusion of α-HBCDD within tissue neutral lipids (Haddad et al. Citation2000). Particularly, due to the adjustment of a plasma neut lip at the value of 0.20 instead of 0.36, the partition coefficients of α-HBCDD to SFD, Skin and Leg increase by 80% (1120 vs. 620 for SFD, 500 vs. 275 for Skin, 33 vs. 18 in Leg of FG broilers and 52 vs. 29 in Leg of SG broilers). In contrast, as the optimised values of a Breast neut lip and a Liver neut lip are decreased compared to initial values (0.23 vs. 0.62 and 0.38 vs. 0.55, respectively), the partition coefficients of α-HBCDD to these tissues are only slightly modified (4 vs. 5 and 25 vs. 20, respectively). Simulated α-HBCDD concentrations in tissues after optimisation vs. data used for parameter optimisation are presented in ).
Figure 2. Observed and simulated α-HBCDD concentration (ng g−1 lipid weight)1 and enantiomeric fraction (EF)2 in tissues of broilers exposed to a contaminated feed (38 µg α-HBCDD kg−1). (a): slow-growing broilers exposed during 42 days and then decontaminated during 42 days (used for optimisation); (b): slow-growing broilers exposed during 84 days; (c): fast-growing broilers exposed during 42 days.
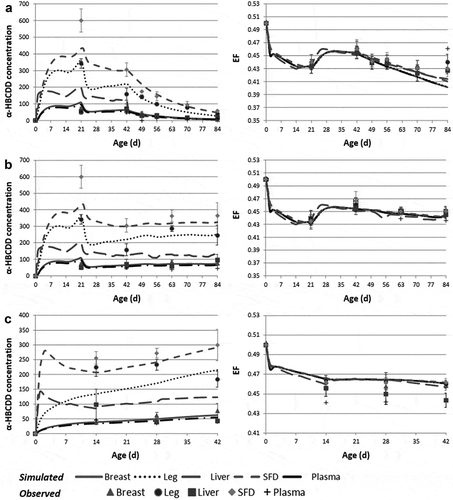
Evaluation
The model was challenged against data obtained in SG- and FG-broilers exposed through feed up to 42 and 84 days of age, respectively (Omer et al. Citation2017; Jondreville et al. Citation2017b) (, Supplemental Material S3). Globally, the model fails to properly predict the concentration of α-HBCDD in Liver and in Plasma (not intended for human consumption), with an overestimation of 7% to 83% compared to observed data. Nevertheless, the concentration of α-HBCDD in the two main edible muscles (the most intended for human consumption), Breast and Leg, is fairly well predicted, with an underestimation of 18 ± 5 and 21 ± 16%, respectively, in FG broilers and an overestimation of 5 ± 9 and 6 ± 8%, respectively, in SG broilers. The concentration of α-HBCDD in SFD is slightly underestimated by 10 ± 6% in the two strains. In all these tissues, the enantiomeric fraction (EF) is very well predicted with overestimation and underestimation below 3% in FG and SG broilers, respectively.
Simulations
Exposure up to slaughter
Default values
At slaughter, SG broilers have ingested 40% more feed than FG broilers (6.37 vs. 4.53 kg), whereas their body weight is 26% lower (2.20 vs. 2.90 kg) so that their feed efficiency (FE) is 46% lower (0.35 vs. 0.64). Despite a slightly higher body lipid concentration at slaughter (136 vs. 130 g kg−1 BW), SG broilers accumulate 40% less lipids than FG broilers per kg feed ingested (47 vs.78 g) and 38% less neutral lipids. In accordance, α-HBCDD AE in SG broilers is 38% lower than in FG broilers (0.22 vs. 0.36). As previously reported (MacLachlan Citation2010), the concentration of α-HBCDD in tissues increases sharply during the first week of life and then remains steady or slightly decreases due to dilution through growth (). At slaughter, the two strains display quite similar tissue α-HBCDD concentrations based on lipids: SFD and Skin are 2% less concentrated in FG than in SG broilers, whereas Breast and Leg are 8% and 5% more concentrated, respectively. However, these concentrations differ between tissues. Skin and SFD lipids are the most concentrated (ARlip = 5.9–6.1), followed by Leg and Breast lipids (ARlip = 4.2–4.4 and 1.2–1.3). When α-HBCDD concentrations are expressed relative to fresh weight (fw; as consumed), SFD is 5.4 more concentrated than feed; Skin is 2.3 less concentrated than SFD and Breast is by far the least concentrated tissue, around 342 times less concentrated than SFD (). Due to its high lipid concentration, Leg in SG broilers is 1.7 times more concentrated in α-HBCDD than in FG broilers. When Breast is consumed together with Skin, α-HBCDD concentration is multiplied by 22 and 20 in FG and SG broilers, respectively. When it is consumed with Leg, the concentration is multiplied by 3.3 and 2.2, respectively.
Variability within strain
Within each strain, FE improves as the animals are slaughtered earlier and heavier (0.44 to 0.69 and 0.26 to 0.47, in FG and SG broilers, respectively). Similarly, AE always increases when animals are slaughtered younger or heavier. Due to their faster growth, the impact of lengthening the rearing period in FG broilers is almost twice as high as in SG broilers, while the impact of increasing BW at slaughter is quite similar in the two strains (). However, due to dilution through growth, AR () and ARlip (Supplemental Material S4) in tissues vary oppositely to AE: they are higher as the animals are slaughtered late and light. Thus, within strain, improving FE limits the contamination of edible tissues, the concentration of which may vary by a factor 1.4 to 1.6 in FG broilers and by 1.5 to 1.7 in SG broilers.
Figure 4. Assimilation efficiency (AE)1 and accumulation ratio (AR)2 of α-HBCDD in tissues of fast- (FG) and slow-growing (SG) broilers3 exposed through feed (38 µg α-HBCDD kg−1) from hatching to slaughter according to their age (Age, d) and body weight (BW, kg) at slaughter.
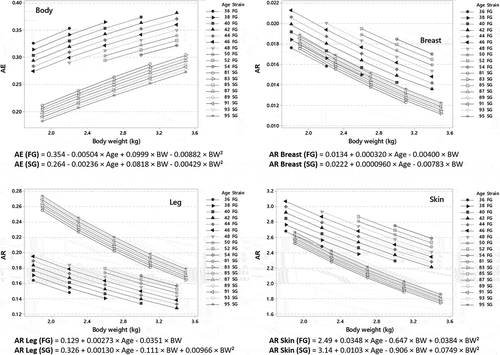
Based on lipids, the concentration of α-HBCDD in tissues is overall lower in SG than in FG broilers, especially when BW at slaughter is high. Thus, depending on the age at slaughter, ARlip in tissues of SG broilers maybe 0.80 to 1.07 times those of FG broilers when BW at slaughter is 2.2 kg and 0.68 to 0.98 times when BW at slaughter is 3.0 kg (Supplemental Material S4). This result fits with the outputs of the model developed by MacLachlan (Citation2010), who estimated that body lipids in SG broilers would be 20% less concentrated in lipophilic pesticides than in FG broilers. However, the current model allows fine-tuning of the impact of production characteristics. Besides, the current estimates clearly do not corroborate the slight increase in α-HBCDD concentration in breast muscles of SG compared to FG broilers reported by Dervilly-Pinel et al. (Citation2017). Thus, characteristics other than those linked to growth and body characteristics such as access to an outdoor run (Jondreville et al. Citation2013), or the use of PS for building insulation (Cariou et al. Citation2014) may be involved.
As Breast and Skin display similar lipid concentration in the two strains, the differences between strains are similar when α-HBCDD concentration is expressed on fresh weight or on lipid weight. In contrast, due to its high lipid concentration, Leg in SG broilers displays higher α-HBCDD concentration based on fresh weight than in FG broilers, and the difference between the two strains is accentuated when body weight at slaughter decreases. Thus, AR in Leg of SG broilers maybe 1.3 to 1.8 times those in SG broilers when BW at slaughter is 2.2 kg and 1.1 to 1.5 times when BW at slaughter is 3.0 kg ().
Decontamination
The model also allows calculation of α-HBCDD concentrations that can be expected at slaughter when animals are allowed to decontaminate after they have been exposed either through feed or accidental ingestion of insulated material such as XPS.
Default values
The example presented in and relates to FG and SG broilers fed a clean feed during the second half of the rearing period, i.e. during 21 and 42 last days, respectively. Over this period, the mean decontamination rates (kc) are around twice as high in FG than in SG broilers (0.044 to 0.045 vs. 0.022 d−1) and does not differ between tissues within each strain. Consequently, over the 21- and 42-day depuration period, α-HBCDD concentration in tissue lipids decreases to a very similar extent in the two strains. This decrease in concentration results from elimination and from dilution through lipid deposition. In contrast to the decontamination rate, the elimination rate (kw) varies between tissues, being higher in muscles than in fatty tissues, especially in FG broilers, which deposit large amounts of separable fat by the end of the rearing period. Overall, fatty tissues, which develop by the end of growth, act as a sink of α-HBCDD and slowdown elimination. However, their development accelerates dilution, so that the resulting decontamination rate does not differ between tissues. Due to their faster growth, dilution contributes to a higher extent to the decontamination of the tissues in FG than in SG broilers (39 vs. 13% in Breast, 27 vs. 14% in Leg, and 85 vs. 51% in Skin; ; 95 vs. 59% in SFD; data not shown).
Table 3. Final concentrations, mean decontamination and elimination rates of α-HBCDD in tissues of fast- (FG) and slow-growing (SG) broilersa contaminated either through feedb or extruded polystyrenec (XPS) consumption.
Figure 5. Simulated kinetics of α-HBCDD burden (µg) (a) and accumulation ratio (ARlip)1 (b) in tissues of fast- (FG) and slow-growing (SG) broilers2 exposed through feed (38 µg α-HBCDD kg−1) from hatching to 21 and 42 days, respectively. Mean decontamination (kc) and elimination (kw) rates are presented in .
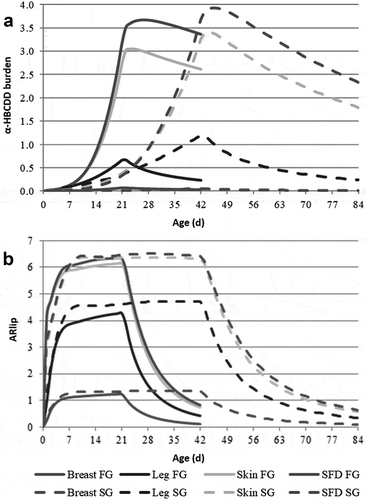
Impact of the duration of α-HBCDD exposure
The scenarios correspond to a 3-day exposure of FG broilers to α-HBCDD through the accidental ingestion of XPS, resulting in the ingestion of 172 µg α-HBCDD. After exposure, animals decontaminate during 28 (A), 21 (B) or 14 (C) days before slaughter. The scenario referred to as Feed, taken as reference, corresponds to broilers chronically exposed α-HBCDD through feed ingestion (38 µg α-HBCDD kg−1 feed) from hatching to slaughter, as presented in and . In case of a 3-day exposure, the concentration of α-HBCDD in tissues sharply increases all the more since broilers are young and light, reaching a maximum of 2,900 (A), 1,300 (B) and 660 (C) µg kg−1 lw in Skin (). Although high, these concentrations remain at the same order of magnitude as those reported in French surveys (DGAL Citation2009) and below the 10,400 µg kg−1 lw recorded in eggs of laying hens after a 3-day exposure through XPS ingestion (Jondreville et al. Citation2017a). This is far higher than the maximum 230 µg kg−1 lw observed for the ‘Feed’ scenario. After the exposure has ceased, these concentrations sharply decrease. The final concentration is the result of initial concentration, duration of depuration and mean decontamination rate. Within each tissue, this latter increases with the age at which broilers are exposed, especially in muscles (). This increase results partly from an enhanced contribution of dilution through lipid deposition in all tissues for older animals. This example also highlights the differences between tissues. In muscles, significant elimination rates are calculated and dilution explains 19% to 37% of the decontamination (). In contrast, the α-HBCDD burden in Skin barely decreases during depuration, because of the redistribution of α-HBCDD to fatty tissues after exposure has ceased, especially in case of late exposure (Figure S4.1). In this case, dilution through growth is almost the only way of decontamination before slaughter.
Figure 6. Simulated kinetics of α-HBCDD concentration (µg kg−1 lw) in breast, leg and skin of fast-growing broilers1 ingesting 172 µg α-HBCDD according to different modalities of exposure: 42-day exposure through feed containing 38 α-HBCDD µg kg−1 (Feed) or exposure through the daily ingestion of 57.3 µg α-HBCDD (extruded polystyrene) during 3 days from 12 to 14 (a), 19 to 21 (b) or 26 to 28 (c) days of age2,3.
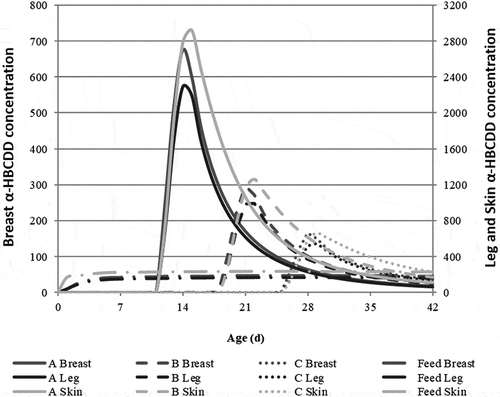
Ingestion of α-HBCDD through broiler meat consumption
As reported by Dervilly-Pinel et al. (Citation2017), breast meat exhibits a mean concentration of 0.60 ng α-HBCDD g−1 lw, irrespective of the strain. Accounting for 93% α-HBCDD (Riviere et al. Citation2014) and a lipid content of 1.27 g kg−1 (), this tissue would contain 0.00709 ng g−1 fw. Considering that animals are exposed through feed throughout the rearing period, the concentration in leg muscles would be 9.3 and 15.6 times higher in FG and SG broilers, respectively (i.e. 0.0680 and 0.108 ng α-HBCDD g−1 fw). Such increases are worth taking into account in human exposure assessment. Similarly, the consumption of skin together with muscles elicits a significant increase in human exposure. Indeed, on a fw basis, Skin is 160 and 150 times more concentrated in α-HBCDD than Breast in FG and SG broilers, respectively. Thus, breast with skin is 22 and 20 more concentrated than breast muscle, respectively, while leg with skin is 30 and 34 times more concentrated, respectively. Accounting for the slow decontamination of skin compared to muscles, Skin may be up to 200 times more concentrated than Breast on fw. In that case, breast + skin and leg + skin are 27 and 36 times more concentrated than breast, respectively. The exposure level for consumers will thus be lower for chickens sold under cut products compared to whole carcases. Indeed, the skin is usually removed from the breast whereas the whole carcases are usually roasted and during this cooking procedure, the subcutaneous lipids may migrate towards the muscle tissue (Rabot Citation1998).
Conclusions
Risk-assessment strongly relies on modelling tools. To that purpose, the current study proposed a PBPK model for the study of the absorption and fate of α-HBCDD in broiler tissues, according to broiler strain and rearing characteristics (age and weight at slaughter). In particular, simulation results showed that the consumption of skin associated to muscle meat (breast or leg) could tremendously increase the exposure to this persistent organic pollutant. Finally, since this model account for lipid dynamics in the bird, it could constitute a generic framework for the study of other lipophilic compounds.
Supplemental Material
Download MS Word (294.4 KB)Acknowledgments
This work was co-funded by the French Ministry for Agriculture and Fisheries in the framework of the “Compte d’Affectation Spéciale Développement Agricole et Rural” (CASDAR project BrAviPorc, number 1256) and by the “Réseau Mixte Technologique QUASAPROVE”. It was carried out within the framework of the Technological Mixed Unit BIRD associating ITAVI and INRA on applied research projects. The authors wish to thank Ronan Cariou (Laboratoire d’Étude des Résidus et Contaminants dans les Aliments) for the analysis of α-HBCDD concentration in samples used for the development and validation of the simulation model.
Supplementary Material
Supplemental data for this article can be accessed on the publisher’s website.
Additional information
Funding
References
- Berntssen MHG, Valdersnes S, Rosenlund G, Torstensen BE, Zeilmaker MJ, van Eijkeren JCH. 2011. Toxicokinetics and carry-over model of alpha-hexabromocyclododecane (HBCD) from feed to consumption-sized Atlantic salmon (Salmo salar). Food Addit Contam Part A. 28:1274–1286.
- Cariou R, Venisseau A, Amand G, Marchand P, Marcon M, Huneau A, Le Bouquin S, Dervilly-Pinel G, Travel A, Le Bizec B. 2014. Identification of hexabromocyclododecane in building material. Organohalog Compd. 76:1521–1524.
- Cobb-Vantress. 2007. Cobb 500 broiler performance and nutrition supplement. https://www.cobb-vantress.com/assets/Cobb-Files/product-guides/bdc20a5443/70dec630-0abf-11e9-9c88-c51e407c53ab.pdf
- Crespo N, Esteve-Garcia E. 2002. Dietary polyinsaturated fatty acids decrease fat deposition in separable fat depots but not in remainder carcass. Poult Sci. 81:512–518.
- Dervilly-Pinel G, Guérin T, Minvielle B, Travel A, Normand J, Bourin M, Royer E, Dubreil E, Mompelat S, Hommet F, et al. 2017. Micropollutants and chemical residues in organic and conventional meat. Food Chem. 232:218–228.
- [DGAL] Direction Générale de l’Alimentation, Ministère de l’Alimentation de l’Agriculture et de la Pêche. 2009. Bilan des plans de surveillance et de contrôle mis en oeuvre par la DGAL en 2008 dans le domaine de la sécurité sanitaire des aliments. [Accessed 2019 Jul]. https://agriculture.gouv.fr/telecharger/82487?token=3c27f66a37a348e7ef29ec1e3544f93d.
- Dzialowsli EM, Crossley DA. 2015. The cadiovascular system. In: Scanes CG, editor. Sturkie’s avian physiology. Sixth ed. New York, NY: Elsevier Academic Press; p. 193–284.
- [ECHA] European Chemicals Agency. 2009. Background document for hexabromocyclododecane and all major diastereomers identified (HBCDD). [accessed 2019 Jul]. http://echa.europa.eu/documents/10162/13640/hbcdd_en.pdf.
- [EFSA] European Food Safety Authority. 2011. Scientific opinion on hexabromocyclododecanes (HBCDDs) in food. Efsa J. 9:2296–2414.
- Griffin HD, Guo K, Windsor D, Butterwith SC. 1992. Adipose tissue lipogenesis and fat deposition in leaner broiler chickens. J Nutr. 122:363–368.
- Haddad S, Poulin P, Krishnan K. 2000. Relative lipid content as the sole mechanistic determinant of the adipose tissue: blood partition coefficients of highly lipophilic organic chemicals. Chemosphere. 40:839–843.
- Haitook T. 2006. Study on chicken meat production for small-scale farmers in northeast Thailand. J Agric Rural Dev Tropics Subtropics. 87(Suppl):1–164.
- Hiebl J, Vetter W. 2007. Detection of hexabromocyclododecane and its metabolite pentabromocyclododecene in chicken egg and fish from the official food control. J Agric Food Chem. 55:3319–3324.
- Jondreville C, Cariou R, Meda B, Dominguez-Romero E, Omer E, Dervilly-Pinel G, Le Bizec B, Travel A, Baéza E. 2017b. Accumulation of alpha-hexabromocyclododecane (alpha-HBCDD) in tissues of fast- and slow-growing broilers (Gallus domesticus). Chemosphere. 178:424–431.
- Jondreville C, Cariou R, Travel A, Belhomme L-J, Dervilly-Pinel G, Le Bizec B, Huneau-Salaün A, Le Bouquin-Leneveu S. 2017a. Hens can ingest extruded polystyrene in rearing buildings and lay eggs contaminated with hexabromocyclododecane. Chemosphere. 186:62–67.
- Jondreville C, Fournier A, Jurjanz S, Engel E, Ratel J, Marchand P, Travel A. 2013. Contamination of poultry products by environmental pollutants. XV European Symposium on the Quality of Eggs and Egg Products, XXI European Symposium on the Quality of Poultry Meat; Sept 15–19; Bergamo, Italy. p. 12.
- Kelly BC, Gobas FAPC, McLachlan MS. 2004. Intestinal absorption and biomagnification of organic contaminants in fish, wildlife, and humans. Environ Toxicol Chem. 23:2324–2336.
- Koch C, Schmidt-Kötters T, Rupp R, Sures B. 2015. Review of hexabromocyclododecane (HBCD) with a focus on legislation and recent publications concerning toxicokinetics and -dynamics. Environ Pollut. 199:26–34.
- Leavens T, Tell L, Clothier K, Griffith R, Baynes R, Riviere J. 2012. Development of a physiologically based pharmacokinetic model to predict tulathromycin distribution in goats. J Vet Pharmacol Ther. 35:121–131.
- Levitt DG. 2007. Heterogeneity of human adipose blood flow. BMC Clin Pharmacol. 7:1–21.
- Li L, Weber R, Liu J, Hu J. 2016. Long-term emissions of hexabromocyclododecane as a chemical of concern in products in China. Environ Int. 91:291–300.
- Lyche JL, Rosseland C, Berge G, Polder A. 2015. Human health risk associated with brominated flame-retardants (BFRs). Environ Int. 74:170–180.
- MacLachlan DJ. 2010. Physiologically based pharmacokinetic (PBPK) model for residues of lipophilic pesticides in poultry. Food Addit Contam Part A. 27:302–314.
- MacLachlan DJ, Bhula R. 2009. Transfer of lipid-soluble pesticides from contaminated feed to livestock, and residue management. Anim Feed Sci Technol. 149:307–321.
- Marvin CH, Tomy GT, Armitage JM, Arnot JA, McCarty L, Covaci A, Palace V. 2011. Hexabromocyclododecane: current understanding of chemistry, environmental fate and toxicology and implications for global management. Environ Sci Technol. 45:8613–8623.
- McLachlan MS. 1994. Model of the fate of hydrophobic contaminants in cows. Environ Sci Technol. 28:2407–2414.
- Méda B, Fortun-Lamothe L, Hassouna M. 2014. Prediction of nutrient flows with potential impacts on the environment in a rabbit farm: a modelling approach. Anim Prod Sci. 54:2042–2051.
- Omer E, Cariou R, Huneau-Salaun A, Dervilly-Pinel G, BaézaBaéza E, Travel A, Le Bouquin S, Jondreville C, Le Bizec B. 2017. Enantiomer-specific accumulation and depuration of alpha-hexabromocyclododecane (alpha-HBCDD) in chicken (Gallus gallus) as a tool to identify contamination sources. Chemosphere. 180:365–372.
- Quentin M, Bouvarel I, Berri C, Le Bihan-Duval E, Baéza E, Jego Y, Picard M. 2003. Growth, carcass composition and meat quality response to dietary concentrations in fast-, medium- and slow-growing commercial broilers. Anim Res. 52:65–77.
- Rabot C. 1998. Vitesse de croissance et caractéristiques lipidiques et sensorielles des muscles de poulet Ph.D. thesis. Paris, France: Institut National Agronomique Paris-Grignon. p. 156.
- Riviere G, Sirot V, Tard A, Jean J, Marchand P, Veyrand B, Le Bizec B, Leblanc JC. 2014. Food risk assessment for perfluoroalkyl acids and brominated flame retardants in the French population: results from the second French total diet study. Sci Total Environ. 491–492:176–183.
- Shahin KA, Abd Elazeem F. 2005. Effects of breed, sex and diet and their interactions on carcass composition and tissue weight distribution of broiler chickens. Archiv Für Tierzucht. 48:612–626.
- Szabo DT, Diliberto JJ, Hakk H, Huwe JK, Birnbaum LS. 2011. Toxicokinetics of the flame retardant hexabromocyclododecane alpha: effect of dose, timing, route, repeated exposure, and metabolism. Toxicol Sci. 121:234–244.
- Venisseau A, Bichon E, Brosseaud A, Vaccher V, Lesquin E, Larvor F, Durand S, Dervilly-Pinel G, Marchand P, Le Bizec B. 2018. Occurrence of legacy and novel brominated flame retardants in food and feed in France for the period 2014 to 2016. Chemosphere. 207:497–506.
- Willems SARL. 2017. Performances de poulets de souches JA567. http://poussins-willems.com/performances657.htm.