ABSTRACT
Pyrrolizidine alkaloids (PAs) and their corresponding N-oxides (PANOs) have been determined in food and feed at levels relevant for consumer health. More than 660 different PAs have been detected, but few are available as reference substances for analytical demands. In the context of the European legislation on maximum levels of PAs in food products, a defined analytical scope of 21 PAs for determination has been suggested. An expansion of the scope from 21 to 35 PAs, including 14 structural isomers, is currently under discussion. In the present study, a target screening method was established for a comprehensive characterisation of PA profiles of the species Echium vulgare, Heliotropium europaeum, Cynoglossum officinale and Symphytum spp. to assess whether an expansion of the analytical scope is required to quantitatively cover the total PA contents of Boraginaceae species. The scope of the method comprised known and unknown PAs previously screened and confirmed in the respective plant extracts. A total of 176 PAs and PANOs were detected. The toxic 1,2-unsaturated PAs represent the predominant PA type with about 98% of the mean total content. This PA profiling demonstrates that an expansion of the scope from 21 to 35 PAs is required to adequately cover the mean total PA contents of Cynoglossum officinale and H. europaeum, whereas in the case of Symphytum spp. and Echium vulgare an expansion would not be necessary.
Abbreviations
Pyrrolizidine alkaloid: PA, Pyrrolizidine alkaloid N-oxide: PANO, European Food Safety Authority: EFSA, German Federal Institute for Risk Assessment: BfR, ultra-high performance liquid chromatography: UHPLC, high-resolution: HR, tandem mass spectrometer: MS/MS, multiple reaction monitoring: MRM, data-independent MS2: ddms2, electrospray ionisation: ESI, limit of detection: LOD, limit of quantification: LOQ, gas chromatography coupled with mass spectrometry: GC-MS, fragmentation (data): MS2 (data), full scan: MS1, variable data-independent acquisition: vDIA, monoester esterified with a necic acid at position C9 of the necine base: O9-monoester, relative proportion: rel. prop., intraperitoneal: i.p., intravenous: i.v., higher-energy C-trap dissociation: HCD, all-ion fragmentation: AIF, parallel reaction monitoring: PRM
Introduction
Pyrrolizidine alkaloids (PAs) are a widespread group of toxic secondary plant metabolites that serve as a defence mechanism against herbivores. They are primarily formed by the plant families Boraginaceae (all genera), Asteraceae (tribes Eupatorieae and Senecioneae) and Fabaceae (mainly the genus Crotalaria) (Hartmann and Witte Citation1995). Up to now, several hundred different PAs have been identified and their presence is assumed in over 6000 different plant species worldwide (Smith and Culvenor Citation1981; Stegelmeier et al. Citation1999; Roeder Citation2000). Recently, El-Shazly and Wink (Citation2014) reviewed more than 190 PAs in about 220 species of the family Boraginaceae. The PA content of a plant varies between plant species, plant organ, geographical origin and growth state and is also influenced by environmental factors (e.g. climate and soil conditions) (Johnson et al. Citation1985; O’Dowd and Edgar Citation1989; Kirk et al. Citation2010).
PAs comprise a necine base esterified with one or two necic acids (). Depending on the degree of esterification, PAs are divided into monoesters, macrocyclic esters and open-chain diesters () (Hartmann and Witte Citation1995; Wiedenfeld et al. Citation2008). The necine base is composed of two fused five-membered rings with a nitrogen atom at the bridgehead. The ring system can be saturated or unsaturated at the 1,2-position. In general, the necine base includes a hydroxymethyl group at C-1 and/or a hydroxyl group at C-7 (). The necic acids are mono- or dicarboxylic acids with branched carbon chains containing hydroxy-, alkoxy-, epoxy- and carboxyester groups (Wiedenfeld et al. Citation2008). In , typical necic acids of the Boraginaceae species are shown. In plants, PAs are naturally found in the form of tertiary bases and/or their corresponding N-oxides (PANOs), where the nitrogen is oxidised. PANOs serve as the transport and storage form in PA-producing plants (Hartmann and Witte Citation1995). The structural diversity of both necine bases and necic acids leads to a wide range of different structures and numerous stereoisomers (Smith and Culvenor Citation1981; Mattocks Citation1986; Rizk Citation1991). With regard to the structural distribution of PAs, there are differences between the plant families Boraginaceae, Asteraceae and Fabaceae. Plant species of the family Boraginaceae predominantly produce PA monoester and open-chain diester, whereas the macrocyclic ester type is characteristic for the tribe Senecioneae of the Asteraceae and for species of the Fabaceae (Hartmann and Witte Citation1995).
Figure 1. Common chemical structure of PA (comprising necine base and necic acid) and PA structures of different ester types.
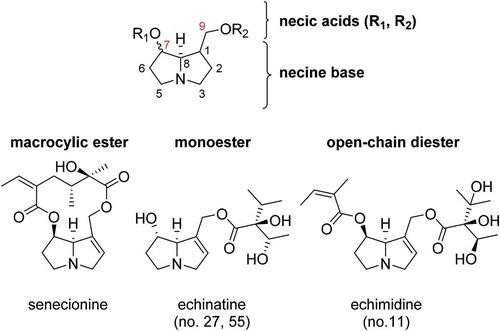
Figure 2. a) Structures of common necine bases of the plant family Boraginaceae. b) Structures of typical necic acids occurring in PAs of the plant family Boraginaceae.
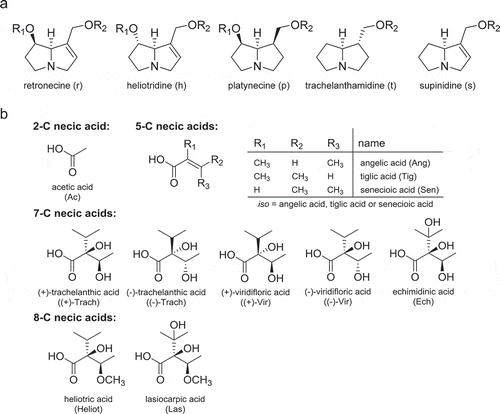
PAs are assumed to be toxic if the necine base is unsaturated at the 1,2-position and esterified with a branched necic acid on at least the C7 or C9 position of the necine base (Mattocks Citation1986). The ingestion of PAs can cause toxic effects in humans and livestock, with the liver being the main target organ (Stegelmeier et al. Citation1999; Fu et al. Citation2004). Acute intoxication is associated with liver dysfunction, especially hepatic veno-occlusive disease (HVOD) (Bras et al. Citation1954; Tandon et al. Citation1976; Kakar et al. Citation2010). Moreover, some PAs have proven to be genotoxic carcinogens in animal studies (NTP Citation1978, Citation2003; Fu et al. Citation2004). Recent studies of animal- and plant-derived food from the European market have shown that humans are mainly exposed to PAs through traditional medicine and foods contaminated by PA-producing plants like tea, herbal infusion, honey, dietary supplements and spices (Bodi et al. Citation2014; Mädge et al. Citation2015; Mulder et al. Citation2018; BfR Citation2019). Based on a comprehensive survey across Europe, the European Food Safety Authority (EFSA) concluded that there is a potential risk for human health related to the exposure to PAs (EFSA Citation2017). The potential health risk of PAs in food is assessed on the basis of the sum of quantified PA and PANO content.
Due to the high diversity of naturally-occurring PAs, a standardised definition of the analytical scope is essential for the comparability of quantitative results with regard to risk assessment and the introduction of legislation on maximum levels of PAs in different food products (European Commission Citation2019). In recent years, the EFSA (EFSA Citation2017) and the German Federal Institute of Risk Assessment (BfR) have published preliminary recommendations for the scope of analysis. The BfR has recommended the investigation of at least 21 individual PAs based on their availability as reference substances in 2013/2014 and their contents determined in (herbal) tea (BfR Citation2013, Citation2015). In the view of these recommendations, the European Commission and European Working Group of Agricultural Contaminants have proposed an analytical scope of 21 individual PAs (scope of 21 PAs) for the legislation on PAs in food products. Since some of these PAs occur naturally as isomers and a chromatographic separation of these PAs cannot be guaranteed by using most of the common analytical methods, the expansion of the scope from 21 to 35 PAs (including 14 structural isomers – scope of 35 PAs) () is currently under discussion (EC Working Group of Agricultural Contaminants Citation2020).
Table 1. PAs and PANOs of the scope of 21 PAs proposed for legislation on PAs in food and their corresponding naturally occurring isomers (given in the same row) discussed for an expansion of the scope to 35 PAs.
For the control of PAs in food and feed, most of the common analytical methods used are target methods based on liquid chromatography in combination with triple quadrupole mass spectrometry (LC-MS/MS) operating in the multiple reaction-monitoring (MRM) mode (Bodi et al. Citation2014; Mulder et al. Citation2018). Due to the high number of PAs and PANOs identified in different plant species so far (Hartmann and Witte Citation1995; El-Shazly and Wink Citation2014), it has to be examined whether the application of the proposed analytical scope of 21 PAs may lead to an underestimation of PA levels in food and feed. Therefore, methodologies have to be developed that allow qualitative and semi-quantitative determination of PAs unavailable as reference substances.
Recently, target screening approaches using high-resolution mass spectrometry (LC-HR-MS) have been increasingly applied to determine PA regardless of their availability as reference substances (Avula et al. Citation2015; Skoneczny et al. Citation2015; Zhu et al. Citation2016; Kast et al. Citation2018). Target screening approaches can represent a full scan mass acquisition with parallel i) fragmentation of a distinct precursor ion selection (ddms2, PRM), ii) fragmentation of precursor ions from consecutive isolation windows covering the entire full scan mass range (vDIA) or iii) fragmentation of all precursor ions of the entire full scan mass range (AIF). For the identification of target PA, the required exact mass of the precursor ion and the respective fragmentation pattern is derived from the sum formula and chemical structure published in literature. The formation of PA fragmentation patterns is characteristic and predictable depending on the necine base, necic acid, ester type and the N-oxidation of the bicyclic ring system (Lin et al. Citation1998; Li et al. Citation2008; Ruan et al. Citation2012). The high-resolution modes enable a sensitive and selective detection and estimation of an almost unlimited number of known PAs simultaneously in a single chromatographic run. Published target screening methods have been mainly focused on the analysis of selected retronecine and/or heliotridine PAs and PANOs from literature (Jeong and Lim Citation2019; Wang et al. Citation2019). A previous selection of PAs from literature also limits the analytical scope of a method. The limitation may lead to an exclusion of unknown retronecine and/or heliotridine type PAs and especially PAs from the necine bases supinidine, trachelanthamidine and platynecine, which in turn would result in an underestimation of the total PA content.
Therefore, a comprehensive profiling of PAs and PANOs in Echium vulgare, Symphytum spp., Cynoglossum officinale and Heliotropium europaeum was performed using mass spectrometric analyses. In the first step, known and unknown PAs and PANOs were detected by screening and confirmation experiments of respective plant extracts based on evaluated characteristic fragmentation patterns of the 1,2-unsaturated retronecine, heliotridine and supinidine type PAs and the 1,2-saturated trachelanthamidine and platynecine type PAs. Secondly, an LC-HR-MS target screening method comprising all confirmed PAs and PANOs was developed and established for qualitative and quantitative PA profiling. Finally, the PA profiles obtained were compared with the proposed analytical scope of 21 PAs in order to assess whether the proposed scope of 21 PAs is appropriate or whether the discussed 14 structural isomers need to be included in the scope (scope of 35 PAs) of common analytical methods. The profiles provide a better understanding of the PA distribution, content and related toxic potential. Structural differences can be observed in the distribution of major and minor compounds of the four species. Statistically verified PA profiles may enable the identification of the PA-producing plant source (genera/plant species) of contaminated food or feed.
Material & methods
Chemicals and reference substances
Methanol, acetonitrile and water were obtained in LC-MS grade from Merck (Darmstadt, Germany). Ammonium formate (VWR, Germany), formic acid (Honeywell, Germany) and zinc dust (Sigma-Aldrich, Seelze, Germany) were purchased in analytical quality. The PA standard substances senecionine, senecionine N-oxide seneciphylline, seneciphylline N-oxide, retrorsine N-oxide, monocrotaline, monocrotaline N-oxide, senkirkine, erucifoline, erucifoline N-oxide, jacobine, jacobine N-oxide, echimidine, intermedine, intermedine N-oxide, lycopsamine, lycopsamine N-oxide, lasiocarpine, europine hydrochloride, europine N-oxide, 7-acetyllycopsamine and 7-acetylintermedine were purchased from PhytoLab (Verstenbergsgreuth, Germany) and echinatine, echinatine N-oxide, heliosupine, heliosupine N-oxide, rinderine and rinderine N-oxide from Phytoplan (Heidelberg, Germany). Retrorsine was purchased from AppliChem (Darmstadt, Germany) and heliotrine N-oxide and lasiocarpine N-oxide from Cfm Oskar Tropitzsch (Markredwitz, Germany). Heliotrine and trichodesmine were obtained from Latoxan (Portes-les-Valence, France) and echimidine N-oxide from Carl Roth (Karlsruhe, Germany). Trachelanthamine was purchased from BOC Sciences (New York, USA). Depending on their solubility, the PAs were dissolved in either methanol or acetonitrile. Stock solutions were stored at 4°C. For the calibration curves, multi-reference mixtures in acetonitrile (1 µg each PA/mL) prepared from individual PA stock solutions and a heliotrine stock solution in methanol (0.509 mg/mL) were used.
Plant materials
Echium vulgare, Symphytum spp. and Cynoglossum officinale were cultivated in 2017 under field conditions in northern Germany, Schleswig-Holstein, and were kindly supplied by the Botanical Institute of the Christian-Albrechts-Universität zu Kiel (Kiel, Germany). A single sample of seeds of Heliotropium europaeum was kindly provided by the Experimental Botanical Garden of the Georg-August-University Göttingen (Göttingen, Germany). For screening and confirmation of PAs and PANOs the air-dried above-ground parts of Echium vulgare, Symphytum spp. and Cynoglossum officinale were milled with dry ice by an ultra-centrifugal mill (1 mm ring sieve, ZM 200, Retsch, Germany). Materials were homogenised with a drum hoop mixer (J. Engelsmann AG, Ludwigshafen am Rhein, Germany). Seeds of Heliotropium europaeum were ground with mortar.
For qualitative and semi-quantitative profiling of PAs and PANOs, a representative number of inflorescences were sampled. Inflorescences of 80 individual plants of Echium vulgare and Cynoglossum officinale and inflorescences of 27 individual plants of Sympyhtum spp. were analysed. Unlike these plant materials, Heliotropium europaeum is not sufficiently represented (n = 1). The plant materials were ground to powder using a coffee grinder. Hay and Hypochaeris radicata were sampled in Brandenburg and Berlin, respectively, and were milled with dry ice by an ultra-centrifugal mill (1 mm ring sieve, ZM 200, Retsch, Germany). All plant materials were stored at room temperature in the dark until sample preparation.
Screening and confirmation of PAs and PANOs
Extract preparation
One gram of the above-ground parts of Echium vulgare, Symphytum spp. and Cynoglossum officinale as well as 1 g of seeds of Heliotropium europaeum were extracted with 40 mL extraction solution (25% methanol with 2% formic acid in water v/v) by vortex mixing and ultrasonic bath for 15 min. The sample was centrifuged (20°C, 4000 rpm, 10 min), the supernatant was passed through a 0.20 µm nylon membrane filter (VWR, Germany) and stored at −20°C until analysis. For the reduction of N-oxides in the plant extracts a 20 mL aliquot was mixed with 4.5 g zinc dust for 3.5 h. After centrifugation (20°C, 4000 rpm, 10 min) the supernatant was filtered through a 0.20 µm nylon membrane filter (VWR, Germany) and stored at −20°C until analysis.
LC-MS/MS and LC-HR-MS parameters
For chromatographic separation, an UltiMate RS 3000 Ultra-High-Performance Liquid Chromatography (UHPLC) system (Dionex/Thermo Fisher Scientific, Waltham, USA) was used. Chromatographic analyses were carried out using a C18 Hypersil Gold column (1.9 µm particle size, 150 × 2.1 mm, Thermo Fisher Scientific, Waltham, USA) including a pre-column cartridge of the same material. The column temperature was maintained at 40°C and 2 µL of the reduced and non-reduced plant extracts were injected. Solvent A was prepared from 0.1% formic acid (v/v) and 5 mM ammonium formate in water. Solvent B was prepared from 0.1% formic acid, 0.5% water (v/v) and 5 mM ammonium formate in methanol. The analysis was performed with the following gradient elution, adjusted to a flow rate of 300 µL/min: 0–0.5 min (5% B), 0.5–7 min (5–50% B), 7–7.5 min (50–80% B), 7.5–7.6 min (80–98% B), 7.6–10.1 min (98% B), 10.1–10.7 min (98–5% B), 10.7–15.0 (re-equilibration 5% B).
Mass spectrometric analyses were performed with a MS/MS (TSQ QuantivaTM, Thermo Fisher Scientific, Waltham, USA) and a high-resolution hybrid quadrupole – Orbitrap mass spectrometer (LC-HR-MS) (Q ExactiveTM Focus, Thermo Fisher Scientific, Waltham, USA), both coupled to the described UHPLC system. All mass spectrometric analyses were performed in electrospray ionisation (ESI) positive mode. The LC-MS/MS was used for screening and confirmation experiments of non-reduced and reduced plant extracts and the following settings were applied: ion spray voltage: 3500 V, capillary temperature: 333°C, vaporiser temperature: 350°C. Sheath gas pressure was adjusted to 40 arbitrary unit, while an aux gas flow of 12 arbitrary units was used; CID gas: 2 mTorr. Precursor ion scans with a scan range from 150 to 600 m/z were acquired via LC-MS/MS for all structure-specific fragmentation patterns, m/z values are given in , with collision energies from 25 to 34 eV. Constant neutral loss scans were performed for Δ amu 28, 60. For confirmation of the compounds detected in the screening experiments, product ion scans with a scan range from 80 to 600 m/z were performed for various m/z values of precursor ions. For identification of sum formulas of detected PAs and PANOs and their further confirmation, full scan and highly resolved product ion spectra using LC-HR-MS were recorded in the full MS/data-dependent MS2 (ddms2) mode with the following settings applied: ion spray voltage: 4000 V, capillary temperature: 275°C, sheath gas flow rate: 45 arbitrary unit and sweep gas flow rate: 2 arbitrary unit. The full scan experiments were acquired in the mass range of 150–600 m/z followed by ddms2 using collision energies from 25 to 33 eV. The resolution was set to 70,000 FWHM (at 200 m/z) for the full scan and 17,500 FWHM (at 200 m/z) for fragmentation. Data acquisition and evaluations for all MS experiments were achieved with Xcalibur software Version 4.0 (Thermo Fisher Scientific, Waltham, USA). All obtained mass spectrometric information of confirmed PAs and PANOs were implemented in target screening lists. These lists contained the sum formula of confirmed precursor ions, the accurate masses of fragment ions, the retention time, the name of the compound if already known and the name of the plant species in which the compound was detected.
Table 2. Characteristic mass spectrometric fragment ions [m/z] and respective relative intensity ratios [%] used for screening and confirmation of PAs and PANOs of different necine base and ester types ( and ). Base peak ions are shown in red.
Qualitative and semi-quantitative profiling of PAs and PANOs
Extract preparation
The extract preparation is described in detail in section Screening and confirmation of PAs and PANOs. Note that inflorescences were extracted instead of above-ground parts of Echium vulgare, Symphytum spp. and Cynoglossum officinale. A reduction of the N-oxides was not carried out. The extracts of C. officinale and H. europaeum were diluted 1:10 and 1:500 with the extraction solution.
LC-HR-MS parameters
The same LC-HR-(ESI)-MS device as described before was used for qualitative and semi-quantitative profiling of PAs and PANOs. The LC and HR-MS settings applied are given in detail in the section Screening and confirmation of PAs and PANOs. High-resolution mass spectrometric analysis was carried out in the variable data-independent acquisition (vDIA) mode. The mass range was 150 to 560 m/z for the full scan. This mass range was divided into three consecutive isolation windows using the quadrupole: 150–330 m/z, 325–400 m/z, 395–560 m/z. All precursor ions in each isolation window were fragmented in the higher-energy C-trap dissociation (HCD) cell applying a collision energy of 30 eV. The resolution was set to 70,000 FWHM (at 200 m/z) for the full scan and 17,500 FWHM (at 200 m/z) for all mass windows. Data were acquired and processed with Thermo Scientific TraceFinderTM software Version 4.1 (Thermo Fisher Scientific, Waltham, USA). The method specifications for the identification and confirmation of PAs and PANOs were based on the guidance document SANTE/11813/2017 with respect to the chapter D (SANTE/11813/2017).
Validation parameters
For the investigation of matrix effects and for finding a PA representative for calibration, three 6-point calibration curves (5, 50, 150, 300, 450 and 600 ng/mL) were prepared from the multi-reference solutions (1 µg each PA/mL) in (a) extraction solution, in (b) extract of hay and (c) extract of Hypochaeris radicata. The extracts of hay (b) and Hypochaeris radicata (c) were prepared as described in the section Screening and confirmation of PAs and PANOs. Heliotrine was used as representative for semi-quantification. In order to calibrate a concentration range from 1.5 to 20000 ng/mL, three overlapping calibration curves with equidistant concentrations were prepared in extraction solution. Three individual calibration curves (a, b, c) ranging from 1.5 to 20000 ng/mL were prepared and linear or quadratic regression with weighting factor of 1/x2 were used. Curve a, with 10 concentration level, ranged from 1.5 to 200 ng/mL (R2 = 0.9944). Curve b contained 16 concentration levels from 150 to 2000 ng/mL (R2 = 0.9978). Curve c with a concentration in the range of 1500 to 20000 ng/mL was described by a quadratic function (R2 = 0.9968). The detection limit (LOD = 0.020 mg/kg) and the limit of quantitation (LOQ = 0.056 mg/kg) were determined for the representative heliotrine according to the established DIN 32645 (DIN ISO 32645:Citation2008-11 2008). LOD and LOQ values were used representative for all detected PAs and PANOs.
Calculations
The concentrations of PAs and PANOs were calculated by Thermo Scientific TraceFinderTM software Ver. 4.1 (Thermo Fisher Scientific, Waltham, USA). Concentrations below or equal to the limit of detection (LOD) were set to 0 ng/mL. If concentrations were stated greater than the LOD and less than the limit of quantitation (LOQ), concentrations were set to half of LOQ.
PAs and PANOs were only included in the PA profile if at least 95% of the individual samples contained the compound. An exception represented the profile of H. europaeum for which only one sample material could be analysed. The content of a compound (sum of PA, corresponding PANO and structural isomers, if detected) was calculated as the averaged content of all individual samples. The mean total PA content of E. vulgare, Symphytum spp., C. officinale and H. europaeum was calculated as the sum of all mean PA contents.
Result and discussion
Analyses for PA profiling
Screening and confirmation of PAs and PANOs
The fragmentation behaviour of different PA structure types was studied by recording and evaluating product-ion spectra of numerous PA reference substances. The fragmentation data (MS2 data), given in , demonstrate that PAs form predictable and characteristic fragment ions with specific ion ratios, depending on their structure (Lin et al. Citation1998; Boppré et al. Citation2005; Li et al. Citation2008; Ruan et al. Citation2012; These et al. Citation2013). The characteristic fragmentation patterns were utilised for LC-MS/MS screening and confirmation experiments of PAs and PANOs in plant extracts of Echium vulgare, Symphytum spp., Cynoglossum officinale and Heliotropium europaeum. For the determination of the accurate molecular mass of confirmed precursor ions and of the fragment ions formed in MS2, the plant extracts were analysed using LC-HR-MS in the mode of combined full MS and product ion scan. These data allowed the derivation of the sum formula and a proposal of the chemical structure.
The characterisation of PAs from 1,2-unsaturated retronecine and heliotridine type is generally based on the detection of the fragment ions m/z 120 and 138. The fragments represent the core structure of the necine base and were detected for all PA ester types irrespectively of the esterifying necic acid(s). In order to distinguish between the different types of esters, further fragment ions have to be considered. Thus, the additional observation of fragment ions m/z 94 and 156 provided evidence for monoester PA, esterified with a necic acid at position C9 of the necine base (O9-monoester) (m/z 111 and 172 for the corresponding N-oxide). Open-chain diesters with esterification at C7 with acetic acid show an additional fragment ion at m/z 180; the respective N-oxide exhibits an ion at m/z 214. The fragment ion m/z 220 is observed for open diester esterified with angelic acid or one of its configurationally isomeric tiglic or senecioic acid at C7, and m/z 254 for the corresponding N-oxide (Boppré et al. Citation2005; Colegate et al. Citation2005). The differentiation of PAs and PANOs from the 7R-configured retronecine or 7S-configured heliotridine necine base was deduced from different intensity ratios of certain characteristic fragment ions at m/z 83, 94, 96 and 138 () (Colegate et al. Citation2018). In the 1,2-unsaturated supinidine type the hydroxyl group at position C7 is missing and can therefore not occur as diester. This also applies to the 1,2-saturated trachelanthamidine type. Due to the absence of supinidine type reference substances, the characteristic fragmentation pattern of tertiary PAs was derived from previously published mass spectrometric data (GC-MS data) (Culvenor Citation1954) combined with recorded product ion spectra of H. europaeum extracts. The fragmentation pattern of the corresponding N-oxide exhibited characteristic fragment ions at m/z 122 and 158. This pattern was exclusively derived from product ion spectra of the H. europaeum extracts. The 1,2-saturated platynecine type PAs are generally characterised by the detection of the fragment ions m/z 122 and 140. Both fragments represent the core structure of the necine base platynecine. Additional characteristic fragment ions for further characterisation are m/z 96, 158 for O9-monoesters and m/z 113, 174 for the corresponding N-oxides. PAs of the 1,2-saturated trachelanthamidine type show the characteristic fragment ions m/z 96, 124 and 142. The latter fragment is formed by the cleavage of the weak allylic ester bond at C9 and represents the necine base trachelanthamidine. In comparison to the respective 1,2-unsaturated necine structures, the described 1,2-saturated compounds differ in their elemental composition in two hydrogen atoms and thus in 2 amu of their corresponding fragment ions and fragmentation patterns, respectively.
For the confirmation of detected tertiary bases and their corresponding N-oxides, zinc powder was added to plant extracts for selective reduction of N-oxides. Re-analysis of these reduced plant extracts was performed to demonstrate the absence of N-oxides and the formation or increase of the signal of the corresponding tertiary base.
Where possible, the identities of PAs and PANOs were confirmed on the basis of chromatographic and mass spectrometric comparison with reference substances. In the absence of reference substances, PAs and PANOs could only be tentatively identified. Therefore, general plausibility checks were performed for each result and structural proposal, respectively. This included the comparison of the received MS2 data with published mass spectrometric data for PAs and PANOs. The obtained retention time was compared with the expected retention time based on polarity or knowledge on similar structures within the same plant family. Moreover, biogenetic considerations from literature were also taken into account with respect to stereochemistry. All characterised PAs and PANOs of Echium vulgare, Symphytum spp., Cynoglossum officinale and Heliotropium europaeum were implemented in target screening lists used for reconstruction of targeted analyte chromatogram from LC-HR-MS data.
Qualitative and semi-quantitative profiling of PAs and PANOs
An LC-HR-MS target screening method was performed for qualitative and semi-quantitative profiling of PAs and PANOs previously detected and confirmed by screening and confirmation studies. The Boraginaceae species investigated are widely distributed in Europe and can play a crucial role as contaminants in food and feed. For the PA profiling, inflorescences of Echium vulgare (n = 80), Cynoglossum officinale (n = 80) and Sympyhtum spp. (n = 27) were analysed, since several studies have reported flowers and inflorescences as the main organ of PA accumulation in plant species (Catalfamo et al. Citation1982; van Dam et al. Citation1995; Kruse et al. Citation2017; Stegemann et al. Citation2018). Additionally, the qualitative and semi-quantitative PA profile of a single sample of seeds from Heliotropium europaeum (n = 1) was studied. The target screening lists of the method comprised a total of 176 PAs and PANOs, of which a considerable number of PAs were not commercially available as reference substance. As individual PA and PANO had different response factors under ESI-MS conditions and thus, calibration curves could not be generated for each PA and PANO, a semi-quantification approach had to be applied. In order to find a pragmatic and appropriate semi-quantification approach, the full scan (MS1) responses of reference substances were determined. The analysed reference substances represent known monoesters, open-chain diesters and corresponding N-oxides of the plant family Boraginaceae (El-Shazly and Wink Citation2014) and are included in the proposed scope of 21 PAs for legislation. The analyte closest to the medium MS1 response, heliotrine, was selected as representative for semi-quantification. Hence, all PAs and PANOs regardless of their structure were quantified via heliotrine-precursor ion as reference. Studies with different matrix-matched and solvent calibration curves demonstrated that matrix effects could be neglected and a solvent calibration could be applied instead (Figure S2 of the supplementary material) (SANTE/11813/Citation2017 2018). Quantitative studies of PA-producing plant species have shown that individual PA contents ranged from ppm contents for major PAs to trace amounts for minor compounds (These et al. Citation2013). In order to cope with this broad range, a calibration curve covering a concentration range from 1.5 to 20000 ng/mL was applied and divided into three sections.
PA profiles
Mean total PA content
Since reference substances are not available for all PAs and PANOs found in the four Boraginaceae species, the PA contents were estimated semi-quantitatively using heliotrine as reference substance. The calculated mean of PA contents of 1,2-saturated and 1,2-unsaturated PAs and PANOs ranged from 357 to 32428 mg/kg, corresponding to 0.04% and 3.24% dry mass, respectively (). The highest mean total contents were detected in Cynoglossum officinale (32428 mg/kg) and Heliotropium europaeum (15736 mg/kg). These contents are more than 10 times higher than those found in Echium vulgare (1330 mg/kg) and Sympyhtum spp. (357 mg/kg). The total contents are in the same order of magnitude as described in the literature for Cynoglossum officinale, Heliotropium europaeum, Echium vulgare and different species of the genus Sympyhtum (Pfister et al. Citation1992; Roeder Citation1995; Boppré et al. Citation2005; Shimshoni et al. Citation2015; Stegemann et al. Citation2018).
Detected PAs and their structural characteristics
The characterisation of PA profiles in the four Boraginaceae species yielded a total of 176 PAs and PANOs, including isomers. The majority of detected alkaloids, 99 PAs and PANOs, are already known from the literature. Of these, 18 PAs and PANOs are included in the discussed analytical scope of 35 PAs (). So far 77 PAs and PANOs have not yet been described for the respective plant species, genera and plant family, respectively. A comprehensive compilation of all PAs found in this study is given in the Tables S1 to S4 in the supplementary material. The tables present the (tentatively) identified compounds listed with an internal number, the accurate mass of the quasi molecular ions [M + H]+, the sum formulas, the number of detected isomers, the structural characteristics related to the necine base and the esterified necic acid(s) at C7 and/or C9 as well as the individual contents.
A comparison of the four plant species indicates that the species Cynoglossum officinale and Heliotropium europaeum exhibit a higher structural diversity than Echium vulgare and Symphytum spp. due to a higher number of different necine base types and corresponding PAs and PANOs (). Cynoglossum officinale’s PA profile comprised 57 PAs and PANOs, of which 35 previously unknown PAs and PANOs were tentatively identified for the species. The majority of alkaloids were assigned as PAs of the heliotridine type, further PAs consisted of the 1,2-saturated necine bases trachelanthamidine and platynecine ( and S3). The profiling of H. europaeum yielded 60 PAs and PANOs, including 29 previously undescribed compounds for the species. Most alkaloids consisted of the necine base heliotridine, whereby six PAs were assigned to the trachelanthamidine and supinidine types ( and S4). In the inflorescences of Echium vulgare, 46 PAs and PANOs were found of which 35 alkaloids were already reported while 11 PAs and PANOs were previously unknown for E. vulgare. With the exception of one PA consisting of the 1,2-saturated platynecine base, all other detected PAs were derived from the 1,2-unsaturated retronecine base ( and S1). For the plant material Symphytum spp. the characterisation resulted in the detection of 13 1,2-unsaturated PAs and PANOs from the retronecine type, whereby two previously unknown PANOs for the genus Symphytum were tentatively identified ( and S2). The observed distribution of the necine base types heliotridine and retronecine is comparable to literature data (Hartmann and Witte Citation1995; El-Shazly and Wink Citation2014) and suggests that PA biosynthesis of the genera Echium and Symphytum is more similar whereas PA biosynthesis of Heliotropium rather resembles that of the genus Cynoglossum.
Table 3. Number of detected PAs and PANOs from each necine base type and their relative proportions (rel. prop.) of the mean total PA content in the four Boraginaceae species.
Two compounds of the 1,2-saturated platynecine type (7-angeloylplatynecine (no. 3, Table S1) and megalanthonine N-oxide (no. 29, and S3)) were tentatively identified in extracts of Echium vulgare and Cynoglossum officinale. Their presence in these species has not been previously described; however, 7-angeloylplatynecine has been detected in several Senecio species of the plant family Asteraceae (Witte et al. Citation1993) and megalanthonine N-oxide in the Boraginaceae species Heliotropium megalanthum (Reina et al. Citation1998). In comparison, PAs of the 1,2-saturated trachelanthamidine type were found more abundant in number and quantities but were produced exclusively by the analysed plant species H. europaeum and C. officinale (). For both plant species, previously unknown PAs and PANOs of the trachelanthamidine type were identified (floridimine and an unknown isomer (no. 28, and S3), unknown PA (no. 50, and S4) and trachelanthamine or viridiflorine (no. 51, and S4)). According to literature, PAs no. 28 and 51 have been detected in various species of the plant family Boraginaceae (Reina et al. Citation1997; El-Shazly and Wink Citation2014), while PA no. 50 has not yet been described.
Table 4. The 10 most abundant alkaloids (sum of PAs and PANOs) of the plant species Echium vulgare. The bold alkaloids are included in the analytical scope of 21 PAs proposed for the determination of maximum levels while alkaloids given in italics are representatives of the 14 structural isomers discussed for the expansion of the scope to 35 PAs.
Table 5. The most abundant alkaloids (sum of PAs and PANOs) and PA profile, respectively, of the plant material Symphytum spp. The bold alkaloids are included in the analytical scope of 21 PAs proposed for the determination of maximum levels while alkaloids given in italics are representatives of the 14 structural isomers discussed for the expansion of the scope to 35 PAs.
Table 6. The 10 most abundant alkaloids (sum of PAs and PANOs) of the plant species Cynoglossum officinale. The bold alkaloids are included in the analytical scope of 21 PAs proposed for the determination of maximum levels while alkaloids given in italics are representatives of the 14 structural isomers discussed for the expansion of the scope to 35 PAs.
Table 7. The 10 most abundant alkaloids (sum of PAs and PANOs) of the plant species Heliotropium europaeum. The bold alkaloids are included in the analytical scope of 21 PAs proposed for the determination of maximum levels while alkaloids given in italics are representatives of the 14 structural isomers discussed for the expansion of the scope to 35 PAs.
The qualitative and quantitative distribution of 1,2-unsaturated necine base types compared to the saturated necine base types ( and ) indicated that Boraginaceae species almost exclusively contain PAs of the unsaturated structure types, mainly retronecine and heliotridine, as confirmed in previous studies (Hartmann and Witte Citation1995; El-Shazly and Wink Citation2014). 1,2-unsaturated type PAs accounted for approximately 92 to 100% of the total PA contents. Cynoglossum officinale formed the highest relative proportion of 1,2-saturated PAs covering about 8% (2521 mg/kg) of the mean total content, while the relative proportion of 1,2-saturated PAs in the other plant species was below 0.1% (0 to 11 mg/kg) ().
PA and PANO
A general comparison of the composition of tertiary PAs and PANOs showed that N-oxides constituted the major part in E. vulgare (95%), Symphytum spp. (92%), C. officinale (95%) and H. europaeum (92%). Several studies identified the N-oxides as the dominating form in plants, e.g. Heliotropium europaeum (>90%), Echium rauwolfii and Echium horridum (90%), Cynoglossum officinale (60 – 90%) (O’Dowd and Edgar Citation1989; Pfister et al. Citation1992; El-Shazly et al. Citation1999; Shimshoni et al. Citation2015).
Ester types
The data further indicate that E. vulgare, Symphytum spp., C. officinale and H. europaeum only produce PAs of the structure types monoester and open-chain diester, which is closely consistent with the literature reviewed by El-Shazly and Wink (Citation2014). Although a smaller number of monoester structures than diesters were found, the relative proportion of detected monoesters accounted for more than 50% of the mean total PA content except for Echium vulgare. The highest relative proportion of monoesters was found in H. europaeum (83% of the mean total PA content, 13026 mg/kg), followed by Symphytum spp. (77%, 276 mg/kg), C. officinale (57%, 18522 mg/kg) and E. vulgare (31%, 414 mg/kg). Further structural comparisons of the profiles demonstrate that detected PAs are mainly esterified with trachelanthic acid, viridifloric acid or echimidinic acid at C9. An esterification with necic acids like heliotridic acid or lasiocarpic acid was only detected in the profile of the species H. europaeum. Open-chain diesters with esterification at C7 with a typical 5-C necic acid (e.g. angelic acid, tiglic acid or senecioic acid) occurred more frequently in number and content than acetylated diesters (Figure S1 of the supplementary material). A necic acid at C9 position additional esterified with acetic acid was found predominantly in the plant species compared to an esterification with a 5-C necic acid. These highly branched compounds were detected exclusively in Echium vulgare, Cynoglossum officinale and Heliotropium europaeum (Figure S1).
Alkaloid distribution and their coverage rate with respect to the analytical scope proposed for legislation on PAs
The 10 most abundant PAs and PANOs of the PA profiles of investigated species ( to ) were compared to reported studies from literature and to the analytical scope of 21 PAs and the scope of 35 PAs, including the 14 structural isomers, discussed for the legislation on PAs in food.
Echium vulgare
PA profiling of inflorescences from Echium vulgare showed that echimidine and the corresponding N-oxide (no. 11, ) were the most abundant alkaloids, accounting for 59.1% of total PA content. Echimidine N-oxide has already been reported as a major alkaloid with high amounts in E. vulgare and further Echium species like E. setosum, E. rauwolfii and E. horridum (42 – 63%) (El-Shazly et al. Citation1996a, Citation1999; Kast et al. Citation2018). In addition, the monoesters vulgarine and vulgarine N-oxide (no. 12, ) as well as echimplatine and its diastereomer leptanthine and the corresponding N-oxides (no. 5, ) were determined as main alkaloids, accounting for 10% and 19% of the mean total content, respectively. In structural terms, the (tentatively) identified three most abundant alkaloids of Echium vulgare consist of a retronecine base esterified at C9 with echimidinic acid or hydroxylated (+)-trachelanthic acid. Further abundant PAs and PANOs of these structure types are echivulgarine (3.8%, no. 18, ), 7-2-methylbutyryl-9-echimidinylretronecine (0.5%, no. 14, ) and uplandicine (0.4%, no. 7, ). Skoneczny et al. (Citation2015) published the latest comprehensive PA profiling of leaves from Australian E. vulgare and reported echimidine N-oxide, echimiplatine N-oxide and, contrary to our data, uplandicine N-oxide as major compounds. In pollen of E. vulgare from Germany and Switzerland high concentrations of echivulgarine, vulgarine, acetylechimdine, echimidine and the corresponding N-oxides were observed (Boppré et al. Citation2005; Lucchetti et al. Citation2016; Kast et al. Citation2018). The major alkaloids echimidine, echimidine N-oxide (no.11, ) and the diastereomers intermedine, lycopsamine and the corresponding N-oxides (no. 4, ) are included in the scope of 21 PAs and 35 PAs, respectively. Both scopes would cover 60% of the mean total PA content of E. vulgare, while further abundant alkaloids like vulgarine (no. 12, ) and echimiplatine/leptanthine (no. 5, ) would remain undetected due to their unavailability as reference substances.
Symphytum spp
In Symphytum spp., the stereoisomers intermedine and lycopsamine and their corresponding N-oxides were found to be the most abundant compounds with 72% of the mean total PA content (no. 19, ), followed by echimidine N-oxide (15%, no. 23, ). The acetylated derivate of lycopsamine, 7-acetyllycopsamine and the corresponding N-oxide (no. 21, ), accounted for 1% of the PA content. The presence of the tentatively identified isomers echimiplatine N-oxide and leptanthine N-oxide (no. 20, ) with about 5% of the total content, has not been previously published for the genus Symphytum. The PA profile obtained could be assigned to the genus Symphytum, albeit a specific assignment to a plant species was not possible due to the similar PA profiles of plant species from the genus Symphytum (Roeder Citation1995; El-Shazly and Wink Citation2014). However, S. officinale can be excluded, due to the fact that echimidine N-oxide (no. 23, ), symlandine and/or a respective isomer and the corresponding N-oxide (no. 22, ) have (tentatively) been identified in relevant amounts in the plant extracts (28% of the mean total content). Both PAs have previously been observed in significant amounts in S. asperum, S. tuberosum and various cytotypes of S. x uplandicum, a hybrid of S. officinale and S. asperum, whereas S. officinale contains no echimidine and symlandine at all (Mütterlein and Arnold Citation1993; HMPC Citation2015). The major PAs echimidine N-oxide (no. 23, ), lycopsamine, intermedine and the corresponding N-oxides (no. 19, ) of the investigated plant material are in the discussed scope of 21 PAs and 35 PAs, respectively. An application of the scopes would cover 87% of the mean total PA content.
Cynoglossum officinale
In accordance with previous studies, the most abundant PAs and corresponding N-oxides found in C. officinale were heliosupine (no. 40, ) and the isomers echinatine and rinderine (no. 27, ) (El-Shazly et al. Citation1996b). These compounds corresponded to 90% of the mean total content. Additionally, the diastereomers of echimiplatine and leptanthine, 5-hydroxyrinderine and a further detected isomer (no. 31, ) were tentatively identified in C. officinale (0.4%). These PAs and PANOs together with other minor compounds (0.7% of the total content) have not been previously described for C. officinale ( and S3). However, none of the identified PAs and PANOs are listed in the analytical scope of 21 PAs. Only the discussed expansion of the scope by the 14 structural isomers (scope of 35 PAs) would guarantee the detection of a contamination of food with Cynoglossum officinale.
Heliotropium europaeum
Seeds of H. europaeum mainly contained the PAs heliotrine (76%, no. 57, ), lasiocarpine (14%, no. 73, ), europine (6%, no. 60, ) and the corresponding PANOs. These alkaloids, esterified with heliotridic or lasiocarpic acid at position C9 of the necine base heliotridine, represented 96% of the mean total PA content, hence confirming previous studies (O’Dowd and Edgar Citation1989; Shimshoni et al. Citation2015). These previous studies have investigated the PA profile of aerial plant parts of H. europaeum grown in Israel and south-eastern Australia and reported europine (38% and 43% of the total content), heliotrine (38% and 37%) and lasiocarpine (14% and 17%) as the most abundant PAs (sum of PAs and PANOs). Further tentatively identified PAs and PANOs, shown in , exhibited relative proportions less than or equal to 1%. The majority of these alkaloids are the PAs and corresponding PANOs heliosupine, rinderine/echinatine and their derivatives, which have been already reported for H. europaeum and further species of the genus Heliotropium (El-Shazly and Wink Citation2014; Shimshoni et al. Citation2015). The analytical scope of 21 PAs includes the three major alkaloids and their corresponding N-oxides (no. 57, 60 and 73, ). An expansion of the scope to 35 PAs would cover further abundant alkaloids, like echinatine, rinderine, heliosupine and their N-oxides (no. 55 and 72, ) and would increase the coverage rate from 96% to 97% of the mean total PA content of H. europaeum.
The findings of the present study indicate that the discussed scope of 35 PAs for the legislation on PA is appropriate to quantitatively cover the mean total PA content of Symphytum spp. (87%) and H. europaeum (97%). For the species Echium vulgare, the rate of coverage amounted for 60%. In the plant species C. officinale, the monoesters echinatine and/or rinderine and the open-chain diesters heliosupine and their corresponding N-oxide, which are not included in the proposed scope of 21 PAs, were most abundantly detected (). However, these PAs represent heliotridine type isomers of the retronecine type PAs intermedine, lycopsamine and echimidine and are in the expanded scope of 35 PAs. The application of the scope of 35 PAs would improve the coverage rate from 0% to 90% of the mean total PA content.
Consequently, the expansion of the analytical scope from 21 to 35 PAs is appropriate to cover the mean total PA content in three of the four investigated Boraginaceae species and up to 60% in Echium vulgare ().
Marker compounds of the PA profiles
The alkaloids (PAs/PANOs) most abundantly detected in the plant species were considered as marker compounds of the four plant species (–).
The majority of these marker compounds, approximately 69%, were formed by a single plant species. Their contents varied in the range from 4.0 to 12005.7 mg/kg. In particular, the marker alkaloids of the plant species Cynoglossum officinale and Heliotropium europaeum were found in high contents ( and ). The three most abundant marker alkaloids of each plant species represent at least 89% of the mean total PA content (–). For instance, the three most abundant alkaloids of the plant species C. officinale, echinatine/rinderine, heliosupine and trachelanthamine and their corresponding PANOs (no. 27, 40 and 25, ), account for 98% (31617 mg/kg) of the mean total PA content; H. europaeum: 96% (15149 mg/kg), Symphytum spp.: 94% (335 mg/kg) and E. vulgare: 89% (1178 mg/kg). The alkaloid heliosupine, the isomers echinatine/rinderine and their PANOs (no. 72 and 55, ) were also detected as marker compounds in H. europaeum. In E. vulgare and Symphytum spp. the respective diastereomeric isomers, intermedine/lycopsamine and echimidine, were found as marker compounds (no. 11, 4, and no. 19, 23, ). These alkaloids are important marker compounds and isomer groups, respectively, due to their widespread distribution among the species studied and their increased contents. Their presence has already been reported in several different plant species of the family Boraginaceae (El-Shazly and Wink Citation2014).
Observed differences in the contents and structures of the marker alkaloids are sufficiently variable to distinguish the four investigated plant species. This is advantageous, e.g. to identify the plant source of PA contamination in food or feed.
PA profiles and their implications for the toxic potentials of Boraginaceae species
In principle, all 1,2 unsaturated PAs are suspected to be hepatotoxic and genotoxic carcinogens, since the double bond in 1,2-position of the pyrrolizidine moiety is considered to be a structural precondition for the formation of reactive pyrrolic metabolites that damage biomolecules (Allgaier and Franz Citation2015; Stegelmeier et al. Citation1999; Wiedenfeld et al. Citation2008; Fu et al. Citation2017). PAs of the 1,2-saturated necine bases platynecine and trachelanthamidine cannot be activated to pyrrolic esters and thus are considered to be non-toxic (Ruan et al. Citation2014; Louisse et al. Citation2019). In previous studies, PAs were tested for their acute toxicity in rodents after intraperitoneal (i.p.) or intravenous (i.v.) application. Diesters showed in general higher potencies compared to monoesters (Merz and Schrenk Citation2016). This also seems the consensus of recently published in vitro studies testing different hepatotoxic and genotoxic endpoints (Allemang et al. Citation2018; Geburek et al. Citation2019; Lester et al. Citation2019; Louisse et al. Citation2019; Rutz et al. Citation2019). However, due to the limited toxicological data (Merz and Schrenk Citation2016), no generally accepted quantitative structure–activity relationship can be derived. Hence, in the framework of current risk assessment, individual 1,2-unsaturated PAs, regardless of their structure, are considered as a group of equivalent substances with cumulative effects in respect to their carcinogenic potential (EFSA Citation2017; BfR Citation2019). Therefore, differences in the contents of 1,2-unsaturated PAs should be considered to be the main factor for the toxic potential of plants. The toxic potential of Cynoglossum officinale is assumed to be the highest among the investigated plant species since the highest total content of 1,2-unsaturated type PAs was estimated (29907 mg/kg, 92.2% of the mean total PA content); followed by Heliotropium europaeum (15711 mg/kg, 99.8%), Echium vulgare (1330 mg/kg, 99.99%) and Symphytum spp. (357 mg/kg, 100%).
As already discussed, the present data confirm that the four Boraginaceae species exclusively produce monoesters and open-chain diesters (Hartmann and Witte Citation1995; El-Shazly and Wink Citation2014). Considering the determined content of open-chain diesters which are assumed to have a higher toxic potential the following order can be observed: Cynoglossum officinale (13906 mg/kg, corresponding to 43% of the mean total PA content), followed by Heliotropium europaeum (2710 mg/kg, 17%), Echium vulgare (917 mg/kg, 69%) and Symphytum spp. (82 mg/kg, 23%).
Conclusion
A comprehensive qualitative and quantitative PA profiling of Echium vulgare, Cynoglossum officinale, Heliotropium europaeum and Symphytum spp. was performed using LC-MS/MS and LC-HR-MS analyses. PAs and PANOs were (tentatively) identified by screening and confirmation experiments of the four plant extracts and subsequently analysed by a target screening method using LC-HR-MS. A total of 176 PAs and PANOs were determined in the four Boraginaceae species (Tables S1 to S4). The PA profiles of Echium vulgare, Cynoglossum officinale and Symphytum spp. were statistically verified by a representative sample size. Characteristic mass spectrometric fragmentation patterns were studied in order to identify PAs and PANOs of the 1,2-unsaturated retronecine, heliotridine and supinidine type and the 1,2-saturated platynecine and trachelanthamidine type (). Tentative structural assignments were suggested for each compound based on mass spectrometric data, literature data and biogenetic considerations.
The characterised PA profiles of the four Boraginaceae species differ in their number of detected PAs and PANOs, in their (tentatively) identified structures and contents. Thus, the PA profiles of Cynoglossum officinale and Heliotropium europaeum comprise a higher number of different necine base types, esterified necic acids and corresponding PAs and PANOs, than the profiles of Echium vulgare and Symphytum spp. The last-mentioned plant species primarily produced PAs and PANOs of the 1,2-unsaturated retronecine type, while Cynoglossum officinale and Heliotropium europaeum mainly formed PAs of the heliotridine type. These types accounted for approximately 92% to 100% of the mean total PA contents of the four plant species (); the estimated total contents ranging from 357 to 32428 mg/kg herb. The contents of individual PAs are not equally distributed among the detected PAs of the profiles, e.g. the three most abundant marker PAs and PANOs account for at least 89% of the mean total content of each plant species studied (–). N-oxide was found to be the predominant form in all plant species. The plant species Cynoglossum officinale is assumed to have the highest toxic potential among the plant species studied, due to the comparatively high contents of 1,2-unsaturated PAs and open-chain diesters. However, the major alkaloids found in this plant species are not included in the scope of 21 PAs proposed for legislation on PAs in food products. The currently discussed expansion of the scope from 21 to 35 PAs would improve the coverage rate from 0% to 90% of the mean total PA content. Therefore, only the discussed scope of 35 PAs is appropriate to quantitatively cover the mean total PA content in three of the four investigated Boraginaceae species and up to 60% of the PAs detected in Echium vulgare. For an improvement of the coverage rate of Echium vulgare further PA reference substances are necessary.
Declaration of interest statement
The authors declare no conflict of interest.
Supplemental Material
Download MS Word (276.6 KB)Acknowledgments
The authors would like to express their gratitude to Mr. Andreas Stock for his assistance in sampling, grinding and extraction of the plant materials.
Supplementary materials
Supplemental data for this article can be accessed on the publisher’s website.
References
- Allemang A, Mahony C, Lester C, Pfuhler S. 2018. Relative potency of fifteen pyrrolizidine alkaloids to induce DNA damage as measured by micronucleus induction in HepaRG human liver cells. Food and Chemical Toxicology. 121:72–81. doi:10.1016/j.fct.2018.08.003.
- Allgaier C, Franz S. 2015. Risk assessment on the use of herbal medicinal products containing pyrrolizidine alkaloids. Regul Toxicol Pharm. 73(2):494–500. doi:10.1016/j.yrtph.2015.09.024.
- Avula B, Sagi S, Wang YH, Zweigenbaum J, Wang M, Khan IA. 2015. Characterization and screening of pyrrolizidine alkaloids and N-oxides from botanicals and dietary supplements using UHPLC-high resolution mass spectrometry. Food Chem. 178:136–148. doi:10.1016/j.foodchem.2015.01.053.
- [BfR] German Federal Institute for Risk Assessment. 2013. Pyrrolizidine alkaloids in herbal teas and teas. BfR Opinion No 018/2013.
- [BfR] German Federal Institute for Risk Assessment. 2015. Provisional BfR recommendations on the analysis of pyrrolizidine alkaloids (PA) in herbal tea and tea (analyte spectrum and sampling method). BfR Communication No 002/2016.
- [BfR] German Federal Institute for Risk Assessment. 2019. Pyrrolizidine alkaloid levels in dried and deep-frozen spices and herbs too high. BfR Opinion No 017/2019.
- Bodi D, Ronczka S, Gottschalk C, Behr N, Skibba A, Wagner M, Lahrssen-Wiederholt M, Preiss-Weigert A, These A. 2014. Determination of pyrrolizidine alkaloids in tea, herbal drugs and honey. Food Addit Contam A. 31(11):1886–1895. doi:10.1080/19440049.2014.964337.
- Boppré M, Colegate SM, Edgar JA. 2005. Pyrrolizidine alkaloids of Echium vulgare honey found in pure pollen. J Agr Food Chem. 53(3):594–600. doi:10.1021/jf0484531.
- Bras G, Jelliffe DB, Stuart KL. 1954. Veno-occlusive disease of liver with nonportal type of cirrhosis, occurring in Jamaica. A.M.A. Arch Pathol. 57(4):285–300.
- Catalfamo JL, Martin WB, Birecka H. 1982. Amino alcohols of pyrrolizidine alkaloids and their necines in Heliotropium curassavicum, Heliotropium spathulatum and Heliotropium indicum. Phytochemistry. 21(11):2669–2675. doi:10.1016/0031-9422(82)83096-3.
- Colegate SM, Edgar JA, Knill AM, Lee ST. 2005. Solid-phase extraction and HPLC-MS profiling of pyrrolizidine alkaloids and their N-oxides: a case study of Echium plantagineum. Phytochem Anal. 16(2):108–119. doi:10.1002/pca.828.
- Colegate SM, Upton R, Gardner DR, Panter KE, Betz JM. 2018. Potentially toxic pyrrolizidine alkaloids in Eupatorium perfoliatum and three related species. Implications for herbal use as boneset. Phytochem Analysis. 29(6):613–626. doi:10.1002/pca.2775.
- Culvenor CCJ. 1954. The alkaloids of Heliotropium europaeum L. II. Isolation and structures of the 3rd major alkaloid and 2 minor alkaloids, and isolation of the principal N-oxides. Aust J Chem. 7(3):287–297. doi:10.1071/CH9540287.
- [DG SANTE] European Commission Directorate-General for Health and Food Safety/11813/2017. 2018. Guidance document on analytical quality control and method validation procedures for pesticide residues and analysis in food and feed. Document No SANTE/11813/2017.
- [DIN] German Institute for Standardization ISO 32645:2008-11. 2008. Chemische Analytik – nachweis-, Erfassungs- und Bestimmungsgrenze unter Wiederholbedingungen – begriffe, Verfahren, Auswertung. ISO 32645. 1994–1995
- [EC] European Commission Working Group of Agricultural Contaminants. 2020. Draft commission regulation amending regulation (EC) 1881/2006 as regards maximum levels of pyrrolizidine alkaloids in certain foods - from protocol.
- [EFSA] European Food Safety Authority. 2017. Risks for human health related to the presence of pyrrolizidine alkaloids in honey, tea, herbal infusions and food supplements. EFSA J. 15(7):1–34.
- El-Shazly A, Abdel Aziz E, Tei A, Wink M. 1999. Pyrrolizidine alkaloids from Echium rauwolfii and Echium horridum (Boraginaceae). Verlag Der Zeitschrift Für Naturforschung. 54c(5–6):295–300. doi:10.1515/znc-1999-5-601.
- El-Shazly A, Sarg T, Ateya A, Abdel Aziz E, El-Dahmy S, Witte L, Wink M. 1996a. Pyrrolizidine alkaloids from Echium setosum And Echium vulgare. J Nat Prod. 59(3):310–313. doi:10.1021/np9600661.
- El-Shazly A, Sarg T, Ateya A, Abdel Aziz E, Witte L, Wink M. 1996b. Pyrrolizidine alkaloids of Cynoglossum officinale and Cynoglossum amabile (family Boraginaceae). Biochem Syst Ecol. 24(5):415–421. doi:10.1016/0305-1978(96)00035-X.
- El-Shazly A, Wink M. 2014. Diversity of pyrrolizidine alkaloids in the Boraginaceae structures, distribution, and biological properties. Diversity. 6(4):188–282. doi:10.3390/d6020188.
- European Commission. 2019. Summary report of the standing committee on plants, animals, food and feed held in Brussels on 29 Mar 2019 (Section novel food and toxicological safety of the food chain). [cited 2020 Feb 23]. https://ec.europa.eu/food/sites/food/files/safety/docs/reg-com_toxic_20190329_sum.pdf: European Commission: Health and Food Safety Directorate General.
- Fu PP, Xia Q, He X, Barel S, Edery N, Beland FA, Shimshoni JA. 2017. Detection of pyrrolizidine alkaloid DNA adducts in livers of cattle poisoned with Heliotropium europaeum. Chem Res Toxicol. 30(3):851–858. doi:10.1021/acs.chemrestox.6b00456.
- Fu PP, Xia Q, Lin G, Chou MW. 2004. Pyrrolizidine alkaloids-genotoxicity, metabolism enzymes, metabolic activation, and mechanisms. Drug Metab Rev. 36(1):1–55. doi:10.1081/DMR-120028426.
- Geburek I, Preiss-Weigert A, Lahrssen-Wiederholt M, Schrenk D, These A. 2019. In vitro metabolism of pyrrolizidine alkaloids - Metabolic degradation and GSH conjugate formation of different structure types. Food Chem Toxicol. 135:110868.
- Hartmann T, Witte L. 1995. Chemistry, biology and chemoecology of the pyrrolizidine alkaloids. In: Pelletier SW, editor. Alkaloids: chemical and biological perspectives. Oxford (UK): Pergamon Press; p. 155–233.
- [HMPC] Herbal Medicinal Product Committee. 2015. Assessment report on Symphytum officinale L., radix. EMA/HMPC/572844/2009.
- Jeong WT, Lim HB. 2019. Determination and chemical profiling of toxic pyrrolizidine alkaloids in botanical samples with UPLC–Q-TOFMS. Chromatographia. 82(11):1653–1664. doi:10.1007/s10337-019-03785-y.
- Johnson AE, Molyneux RJ, Merrill GB. 1985. Chemistry of toxic range plants. Variation in pyrrolizidine alkaloid content of Senecio, Amsinckia, and Crotalaria species. J Agric Food Chem. 33(1):50–55. doi:10.1021/jf00061a015.
- Kakar F, Akbarian Z, Leslie T, Mustafa ML, Watson J, van Egmond HP, Omar MF, Mofleh J. 2010. An outbreak of hepatic veno-occlusive disease in Western afghanistan associated with exposure to wheat flour contaminated with pyrrolizidine alkaloids. J Toxicol. 2010:313280. doi:10.1155/2010/313280.
- Kast C, Kilchenmann V, Reinhard H, Droz B, Lucchetti MA, Dubecke A, Beckh G, Zoller O. 2018. Chemical fingerprinting identifies Echium vulgare, Eupatorium cannabinum and Senecio spp. as plant species mainly responsible for pyrrolizidine alkaloids in bee-collected pollen. Food Addit Contam A. 35(2):316–327. doi:10.1080/19440049.2017.1378443.
- Kirk H, Vrieling K, Van Der Meijden E, Klinkhamer PG. 2010. Species by environment interactions affect pyrrolizidine alkaloid expression in Senecio jacobaea, Senecio aquaticus, and their hybrids. J Chem Ecol. 36(4):378–387. doi:10.1007/s10886-010-9772-8.
- Kruse LH, Stegemann T, Sievert C, Ober D. 2017. Identification of a second site of pyrrolizidine alkaloid biosynthesis in Comfrey to boost plant defense in floral stage. Plant Physiol. 174(1):47–55. doi:10.1104/pp.17.00265.
- Lester C, Troutman J, Obringer C, Wehmeyer K, Stoffolano P, Karb M, Xu Y, Roe A, Carr G, Blackburn K, et al. 2019. Intrinsic relative potency of a series of pyrrolizidine alkaloids characterized by rate and extent of metabolism. Food Chem Toxicol. 131:110523. doi:10.1016/j.fct.2019.05.031.
- Li SL, Lin G, Fu PP, Chan CL, Li M, Jiang ZH, Zhao ZZ. 2008. Identification of five hepatotoxic pyrrolizidine alkaloids in a commonly used traditional Chinese medicinal herb, Herba Senecionis scandentis (Qianliguang). Rapid Commun Mass Spectrom. 22(4):591–602. doi:10.1002/rcm.3398.
- Lin G, Zhou KY, Zhao XG, Wang ZT, But PPH. 1998. Determination of hepatotoxic pyrrolizidine alkaloids by on-line high performance liquid chromatography mass spectrometry with an electrospray interface. Rapid Commun Mass Sp. 12(20):1445–1456. doi:10.1002/(SICI)1097-0231(19981030)12:20<1445::AID-RCM356>3.0.CO;2-G.
- Louisse J, Rijkers D, Stoopen G, Holleboom WJ, Delagrange M, Molthof E, Mulder PPJ, Hoogenboom RLAP, Audebert M, Peijnenburg AACM. 2019. Determination of genotoxic potencies of pyrrolizidine alkaloids in HepaRG cells using the gamma H2AX assay. Food and Chemical Toxicology. 131:110532.
- Lucchetti MA, Glauser G, Kilchenmann V, Dubecke A, Beckh G, Praz C, Kast C. 2016. Pyrrolizidine Alkaloids from Echium vulgare in honey originate primarily from floral nectar. J Agr Food Chem. 64(25):5267–5273. doi:10.1021/acs.jafc.6b02320.
- Mädge I, Cramer L, Rahaus I, Jerz G, Winterhalter P, Beuerle T. 2015. Pyrrolizidine alkaloids in herbal teas for infants, pregnant or lactating women. Food Chem. 187:491–498. doi:10.1016/j.foodchem.2015.04.067.
- Mattocks AR. 1986. Chemistry and Toxicology of Pyrrolizidine Alkaloids. London, UK: Academic Press.
- Merz KH, Schrenk D. 2016. Interim relative potency factors for the toxicological risk assessment of pyrrolizidine alkaloids in food and herbal medicines. Toxicol Lett. 263:44–57. doi:10.1016/j.toxlet.2016.05.002.
- Mulder PPJ, Lopez P, Castellari M, Bodi D, Ronczka S, Preiss-Weigert A, These A. 2018. Occurrence of pyrrolizidine alkaloids in animal- and plant-derived food: results of a survey across Europe. Food Addit Contam Part A Chem Anal Control Expo Risk Assess. 35(1):118–133. doi:10.1080/19440049.2017.1382726.
- Mütterlein R, Arnold C-G. 1993. Investigations concerning the content and the pattern of pyrrolizidine alkaloids in Symphytum officinale L. Pharmazeutische Zeitung Wissenschaft. 138:119–125.
- [NTP] National Toxicology Program. 1978. Bioassay of lasiocarpine for possible carcinogenicity. National Cancer Institute technical report series.
- [NTP] National Toxicology Program. 2003. Toxicology and carcinogenesis studies of riddelliine (CAS No. 23246-96-0) in F344/N Rats and B6C3F1 Mice (Gavage Studies). National Toxicology Program technical report series.
- O’Dowd DJ, Edgar JA. 1989. Seasonal dynamics in the pyrrolizidine alkaloids of Heliotropium europaeum. Australian J Ecol. 14(1):95–105. doi:10.1111/j.1442-9993.1989.tb01011.x.
- Pfister JA, Molyneux RJ, Baker DC. 1992. Pyrrolizidine alkaloid content of houndstongue (Cynoglossum officinale L). J Range Manage. 45(3):254–256. doi:10.2307/4002973.
- Reina M, GonzalezColoma A, Gutierrez C, Cabrera R, Henriquez J, Villarroel L. 1997. Bioactive saturated pyrrolizidine alkaloids from Heliotropium floridum. Phytochemistry. 46(5):845–853. doi:10.1016/S0031-9422(97)00354-3.
- Reina M, Gonzalez-Coloma A, Gutierrez C, Cabrera R, Henriquez J, Villarroel L. 1998. Pyrrolizidine alkaloids from Heliotropium megalanthum. J Nat Prod. 61(11):1418–1420.
- Rizk A-FM. 1991. The pyrrolizidine alkaloids: plant sources and properties. In: Rizk A-FM, editor. Naturally occurring pyrrolizidine alkaloids. Boca Raton (FL): CRC Press; p. 1–89.
- Roeder E. 1995. Medicinal plants in Europe containing pyrrolizidine alkaloids. Pharmazie. 50(H.2)):83–98.
- Roeder E. 2000. Medicinal plants in China containing pyrrolizidine alkaloids. Pharmazie. 55(10):711–726.
- Ruan JQ, Li N, Xia QS, Fu PP, Peng SY, Ye Y, Lin G. 2012. Characteristic ion clusters as determinants for the identification of pyrrolizidine alkaloid N-oxides in pyrrolizidine alkaloid-containing natural products using HPLC-MS analysis. J Mass Spectrom. 47(3):331–337. doi:10.1002/jms.2969.
- Ruan JQ, Yang MB, Fu P, Ye Y, Lin G. 2014. Metabolic activation of pyrrolizidine alkaloids: insights into the structural and enzymatic basis. Chem Res Toxicol. 27(6):1030–1039. doi:10.1021/tx500071q.
- Rutz L, Gao L, Schrenk D. 2019. Structure-dependent cytotoxicity of different pyrrolizidine alkaloids in primary rat hepatocytes. Zeitschrift Für Phytotherapie. 40:S 01–S25.
- Shimshoni JA, Mulder PP, Bouznach A, Edery N, Pasval I, Barel S, Abd-El Khaliq M, Perl S. 2015. Heliotropium europaeum poisoning in cattle and analysis of its pyrrolizidine alkaloid profile. J Agric Food Chem. 63(5):1664–1672. doi:10.1021/jf5052199.
- Skoneczny D, Weston PA, Zhu X, Gurr GM, Callaway RM, Weston LA. 2015. Metabolic profiling of pyrrolizidine alkaloids in foliage of two Echium spp. invaders in Australia-A case of novel weapons? Int J Mol Sci. 16(11):26721–26737. doi:10.3390/ijms161125979.
- Smith LW, Culvenor CCJ. 1981. Plant sources of hepatotoxic pyrrolizidine alkaloids. J Nat Prod. 44(2):129–152. doi:10.1021/np50014a001.
- Stegelmeier BL, Edgar J, Colegate SM, Gardner DL, Schoch TK, Coulombe RA, Molyneux RJ. 1999. Pyrrolizidine alkaloid plants, metabolism and toxicity. J Nat Toxins. 8(1):95–116.
- Stegemann T, Kruse LH, Brutt M, Ober D. 2018. Specific distribution of pyrrolizidine alkaloids in floral parts of Comfrey (Symphytum officinale) and its implications for flower ecology. J Chem Ecol. 45:1–8.
- Tandon BN, Tandon RK, Tandon HD, Narndranathan M, Joshi YK. 1976. An epidemic of veno-occlusive disease of liver in Central India. Lancet. 2(7980):271–272. doi:10.1016/S0140-6736(76)90727-3.
- These A, Bodi D, Ronczka S, Lahrssen-Wiederholt M, Preiss-Weigert A. 2013. Structural screening by multiple reaction monitoring as a new approach for tandem mass spectrometry: presented for the determination of pyrrolizidine alkaloids in plants. Anal Bioanal Chem. 405(29):9375–9383. doi:10.1007/s00216-013-7365-4.
- van Dam NM, Witte L, Theuring C, Hartmann T. 1995. Distribution, biosynthesis and turnover of pyrrolizidine alkaloids in Cynoglossum Officinale. Phytochemistry. 39(2):287–292. doi:10.1016/0031-9422(94)00944-O.
- Wang T, Frandsen HL, Christiansson NR, Rosendal SE, Pedersen M, Smedsgaard J. 2019. Pyrrolizidine alkaloids in honey: quantification with and without standards. Food Control. 98:227–237. doi:10.1016/j.foodcont.2018.11.033.
- Wiedenfeld H, Roeder E, Bourauel T, Edgar JA. 2008. Pyrrolizidine alkaloids - Structure and Toxicity. Göttingen, Germany: V&R unipress GmbH.
- Witte L, Rubiolo P, Bicchi C, Hartmann T. 1993. Comparative analysis of pyrrolizidine alkaloids from natural sources by gas chromatography-mass spectrometry. Phytochemistry. 32(1):187–196. doi:10.1016/0031-9422(92)80130-7.
- Zhu L, Ruan JQ, Li N, Fu PP, Ye Y, Lin G. 2016. A novel ultra-performance liquid chromatography hyphenated with quadrupole time of flight mass spectrometry method for rapid estimation of total toxic retronecine-type of pyrrolizidine alkaloids in herbs without requiring corresponding standards. Food Chem. 194:1320–1328. doi:10.1016/j.foodchem.2014.11.093.