Abstract
In this study, the effects of osmotic dehydration parameters (salt concentration, temperature, and ratio of solution to sample) on the dehydration rate and the quality of the dehydrated apricot were studied. Effective diffusivities were in the order of magnitude between 10−10 and 10−9 with an overall average of 1.37 × 10−9 m2/s for water loss and 1.15 × 10−9 m2/s for solid (salt) gain. The dehydration curves were constructed using Peleg's model. Increasing the solution volume increased the mass transfer rate and hence the solid gain increased rapidly. A ‘solution to sample’ ratio of 5:1 gave the best product quality. The best temperature and concentration that had a high water loss to solid gain ratio and an acceptable taste were 40 °C and 50 g/kg, respectively.
Se estudiaron los efectos de los parámetros de deshidratación osmótica (concentración salina, temperatura y relación solución a muestra) en el índice de deshidratación y la calidad del albaricoque deshidratado. Las difusividades efectivas se situaron en el orden de magnitud entre 10−10 y 10−9 con un promedio total de 1.37×10−9 m2/s para pérdida de agua y 1.12×10−9 m2/s para el aumento de sólido (sal). Las curvas de deshidratación se establecieron usando el modelo de Peleg. El aumento del volumen de solución incrementó el índice de transferencia de masa y por tanto el aumento de sólido se incrementó rápidamente. Un relación solución a muestra de 5:1 dio la mayor calidad de producto. La mejor temperatura y concentración que tuvo una mayor relación pérdida de agua a ganancia de sólido y un sabor aceptable fueron 40 °C y 50 g/kg, respectivamente.
Palabras clave:
Introduction
The quality of foodstuffs and their production cost are the most important factors to be considered when choosing a method for food preservation. Water being the one of the main food components, has a decisive direct influence on the organoleptic quality and durability of foodstuffs through its effects on many physicochemical and biological parameters. Water removal or making water hard to access for microbe development is the main task while preserving food (Lenart, Citation1996).
According to FAO, the annual production of apricot in Iran exceeds to 220,000 tons (making Iran as the world second producer). Nearly half of this amount was dried. This shows the importance of attempts for improving the quality of dried apricots. Traditional drying methods incorporate serious decreases of nutritive and sensorial values, by damaging the flavor, color, and nutrients of the product (Lenart, Citation1996; Lin, Durance, & Scaman, Citation1998). Osmotic drying is an interesting technique used as a pretreatment prior to air drying as it is supposed to obtain dry apricot ingredients, having a natural color, without sulfur dioxide, which could be suitable for different applications (Forni, Sormani, Scalise, & Torreggiani., Citation1997). This process has received considerable attention recently, to reduce the energy consumption and improve food quality (El-Aouar, Azoubel, & Murr, Citation2003).
Osmotic dehydration consists of the immersion of the fruit in a hypertonic aqueous solution leading to loss of water through the cell wall membrane of the fruit and subsequent flow along the inter-cellular space before diffusing into the solution (Sereno, Moreira, & Martinez, Citation2001). Solute transference to the fruit during osmosis causes an increase of soluble solids content of the fruit which decreases the phenolase activity and hence reducing the susceptibility of the fruit to the enzymatic browning during air dehydration (McBean, Joslyn, & Nury, 1971).
Khoyi and Hesari (Citation2007) reported on the kinetics of osmotic dehydration of apricot using sucrose solution. The osmotic solutions used there were sucrose solutions in high concentrations and hence were highly viscose. As a result using sucrose caused technical problems for mixing and furthermore it could increase the production costs. In a series of published papers (Corzo and Bracho, Citation2006; Khin, Zhou, & Perera, Citation2006; Mayor, Moreira, Chelno, & Sereno, Citation2006; Sutar and Gupta, Citation2007), the use of salt for osmotic dehydration has been proposed since it has low viscosity and its ions diffuse better than sucrose. Therefore, the aim of this article is to replace sucrose solution by salt.
Solid gain and water loss of the samples upon application of different salt concentrations at different temperatures were studied to evaluate the diffusion coefficient of the salt and water using Peleg and Crank's equations.
Materials and methods
Material
Apricots, cultivar Nasiry, picked at the fresh market maturity (the average refractive index and pH of apricots were 24 and 4.5, receptively), were purchased at a local market (Tabriz, Iran).
Equipment
The equipment used in the osmotic dehydration experiments consisted of a jacketed stainless steel chamber designed to work at atmospheric pressure (Corrêa, Pereira, Vieira, & Hubinger, Citation2010). The chamber presented internal diameter of 454 × 103 m and height of 500 × 103 m. The osmotic solution temperature was controlled using a thermostatic bath connected to the equipment. The solution was stirred by a controlled flow recirculation system using a sanitary pump.
Sample preparation and osmotic process
Apricots were cut into 10 mm slices and osmo-dehydrated. They were weighed and placed into 250 ml beakers (5 g for each beaker), containing 50, 100, or 150 g/kg salt solutions and osmo-dehydrated at temperatures of 30, 40, 50, or 60 °C. Dehydration times of 30, 60, 120, 180, 240, 300, or 360 min were used for each salt concentration and time. The duration of osmotic dehydration was based on previously published works (Corrêa et al., Citation2010; Mayor et al., Citation2006; Singh, Citation2001).
To find the best ‘solution to sample’ ratio three ratios were examined: 5:1, 10:1, and 15:1. In all experiments the weight ratio of solution to sample was 5:1 except of when studying the effect of solution to sample ratio. To determine the solid content of samples before and after the dehydration process, samples of the fruits were weighed and then oven dried. The average solid content of the samples was 160 g/kg (wb).
Referring to works done before (Azoubel and Murr, Citation2004; Corzo and Bracho, Citation2006; Palou, Lopez-Malo, Argaiz, & Welti, Citation1993; Park, Bin, Brod, & Park, Citation2002) Peleg's model was chosen to understand the mass transfer kinetics of osmotic dehydration:
To describe a plate of thickness 2L having the uniform initial water or solids amount MC(0), submitted to osmotic dehydration at constant conditions, Fick's unidirectional diffusion equation could be used (Ochoa-Martínez & Ayala-Aponte, Citation2005). As described in our previous work (Khoyi and Hesari, Citation2007) it becomes in three dimensions:
Allowing the calculation of effective diffusivity for solid gain and water loss based on the dimensionless amount of water loss (WA ) and solid gain (WS ):
Solid gain was calculated based on differences between solid content of the samples before and after processing. Water loss was obtained as weight loss of samples plus solid gain (EquationEquations (4) and Equation(5)). Then concerning the obtained experimental data's, curves of water loss and solid gain as a function of time were constructed and the average relative error for each set of data was calculated using EquationEquation (6)
Results
Determining the best ratio of ‘solution to sample’
The volume of the osmotic solution has a direct influence on the water loss and salt gain. Increasing the volume of the osmotic solution causes an increase in both water loss and salt gain (where the latter is undesirable). The results for different ‘solution to sample’ weight ratios are shown in and , which were obtained at 30 °C and 50 g/kg salt concentration. The highest water loss was obtained at 15:1 ratio which is accompanied by the highest salt gain. As shown in , the highest water loss to salt gain ratio were obtained when using a solution to sample ratio of 5:1. While water loss for this ratio was 12% lower, the WL/SG for 5:1 ratio was 46% more than 15:1 ratio. Therefore we used the 5:1 ratio that could have a better quality.
Evaluating of Peleg's equation parameters and effective diffusivity
Equation parameters and effective diffusivities were calculated for different temperatures and concentrations at solution to sample weight ratio of 5:1. It was assumed that equilibrium concentration in EquationEquation (4) equals to concentration at time of infinity which using Peleg's equation becomes 1/k 2. Then using EquationEquation (3), effective diffusivities were calculated. shows effective diffusivities for water loss and solid gain and amount of WL/SG.
Peleg's equation parameters, effective diffusivities, and WL/SG did not exhibit a trend with increase of temperature at the constant concentration, or with increase of concentration at the same temperature. The average relative error calculated varied from 1.5 to 12.3% for water loss and 1.4 to 3.1% for solid gain. As it is obvious, effective diffusivities were in the order of magnitude between 10−10 and 10−9 with an overall average of 1.37 × 10−9 m2/s for water loss and 1.15 × 10−9 m2/s for solid gain which was found similar for carrot, potato, and pumpkin dehydration in salt solution in previous works (Khin et al., Citation2006; Mayor et al., Citation2006; Singh, Kumar, & Gupta, Citation2007).
Effect of temperature and concentration variations on mass transfer rate
It is expected that increasing the concentration and temperature of the osmotic media would increase the mass transfer rate but the results shown in – did not exactly fulfill this expectation. As can be seen in , where the effect of concentration on water loss is shown, at temperatures of 30 °C and 40 °C increasing salt concentration from 50 to 150 g/kg increased the water loss. This effect was less clear for concentrations of 100–150 g/kg at temperatures of 50 °C and 60 °C. This might be explained by the detrimental effect of salt on relatively soft texture of the apricot which causes an overall mass loss at 150 g/kg concentration. Because of the aforementioned problems comparison between the results for 150 g/kg and other concentrations was not reliable. An increase in concentration from 50 to 100 g/kg causes a remarkable increase in water loss but more increase of concentration did not make such an increase in water loss. So without considering the taste of the product the best concentration is 100 g/kg but a selection between 50 g/kg and 100 g/kg depends on the taste that is to be desirable. Using 100 g/kg concentration results in a salty and sweet taste but when the salty taste is not desired then 50 g/kg concentration should be used. Furthermore the taste of products from 150 g/kg solution was very salty.
As is shown in increasing the temperature did not always cause an increase on the water loss. Approximately the highest water loss was observed at 40 °C. Increasing the temperature decreases the mass transfer resistance and at the same time increases the solubility of natural occurring sugars but at a different rate. As the salt concentration in the solution is a low concentration when temperature increases, the solution attends to absorb more solids from the apricots instead of absorbing water. Because of different increasing rates of mass transfer and solubility, increasing temperature from 30 to 40 °C caused an increase in water loss but when we reached 50 °C the overall result was a decrease in water loss and at 60 °C we saw an increase in water loss relative to 50 °C. Regarding these results it is preferable to work at 40 °C to obtain better quality except of when using 100 g/kg concentration where higher temperatures result in a similar amount of water loss.
To make a selection for temperature and concentration for the osmotic solution, between 50 g/kg and 40 °C, 100 g/kg and 40 °C or 100 g/kg and 50 °C, it is sufficient to compare the water loss to solid gain ratio for these sets of temperature and concentration. The highest value for that ratio occurred at 50 g/kg and 40 °C as stated in Supplementary Table 2. But if the dehydration rate is more important than the quality of the product, then 100 g/kg and 50 °C could be a good choice.
Discussion
Using salt solution as the osmotic media has some advantages over sucrose solution but has some disadvantages too. It has low viscosity and its ions diffuse better than sucrose (because they are smaller than sucrose molecules) but make a salty taste in the product. To obtain better quality low concentrations such as 50 g/kg should be used. Higher temperatures cause more migration of natural solids into osmotic media and higher concentrations cause more solid gain. This increasing temperature influence has been clearly shown in previous works (Khoyi and Hesari, Citation2007; Sereno et al., Citation2001; İspir & Türk Toğrul, Citation2009). The temperature has two different effects during dehydration: (i) temperature increases molecular agitation and thus enhances diffusion rates; (ii) the cellular material is quite sensitive to the temperature when it exceeds a critical value that depends on the cell species. For plant cells, this value is around 50–55 ˚C (Allali, Marchal, & Vorobie, Citation2010). Because of low concentration and low viscosity in the osmotic media the natural solids of the fruit can easily come in to the osmotic solution and be replaced with salt, so it is important to choose a temperature that improves water loss but limits the solids gain. In this work the optimum temperature and concentration set, was 40 °C and 50 g/kg.
tcyt_a_503279_sup_20983524.pdf
Download PDF (493.2 KB)References
- Allali , A. , Marchal , L. and Vorobie , E. 2010 . Effects of vacuum impregnation and ohmic heating with citric acid on the behavior of osmotic dehydration and structural changes of apple fruit . Biosystems Engineering , 106 ( 1 ) : 6 – 13 .
- Azoubel , P. M. and Murr , F. E.X. 2004 . Mass transfer kinetics of osmotic dehydration of cherry tomato . Journal of Food Engineering , 61 : 291 – 295 .
- Corrêa , J. L.G. , Pereira , L. M. , Vieira , G. S. and Hubinger , M. D. 2010 . Mass transfer kinetics of pulsed vacuum osmotic dehydration of guavas . Journal of Food Engineering , 96 : 498 – 504 .
- Corzo , O. and Bracho , N. 2006 . Application of Peleg model to study mass transfer during osmotic dehydration of sardine sheets . Journal of Food Engineering , 75 ( 4 ) : 535 – 541 .
- El-Aouar , A. A. , Azoubel , P. M. and Murr , F. E.X. 2003 . Dehydration kinetics of fresh and osmotically pretreated papaya (Carica papaya L.) . Journal of Food Engineering , 59 : 85 – 91 .
- Forni , E. , Sormani , A. , Scalise , S. and Torreggiani , D. 1997 . The influence of sugar composition on the color stability of osmodehydrated frozen intermediate moisture apricots . Food Research International , 30 : 87 – 94 .
- İspir , A. and Türk Toğrul , İ. 2009 . Osmotic dehydration of apricot: Kinetics and the effect of process parameters . Chemical Engineering Research and Design , 87 : 166 – 180 .
- Khin , M. M. , Zhou , W. and Perera , C. O. 2006 . A study of the mass transfer in osomotic dehydration of coated potato cubes . Journal of Food Engineering , 77 ( 1 ) : 84 – 95 .
- Khoyi , M. R. and Hesari , J. 2007 . Osmotic dehydration kinetics of apricot using sucrose solution . Journal of Food Engineering , 78 : 1355 – 1360 .
- Lenart , A. 1996 . Osmo-convective drying of fruits and vegetables: Technology and application . Drying Technology , 14 : 391 – 413 .
- Lin , T. M. , Durance , T. D. and Scaman , C. H. 1998 . Characteristics of vacuum microwave air and freeze dried carrot slices . Food Research International , 4 : 111 – 117 .
- Mayor , L. , Moreira , R. , Chelno , F. and Sereno , A. M. 2006 . Kinetics of osmotic dehydration of pumpkin with sodium chloride solutions . Journal of Food Engineering , 74 ( 2 ) : 253 – 262 .
- McBean , D. , Joslyn , M. A. and Nury , F. S. 1971 . “ Dehydrated fruit ” . In The biochemistry of fruit and their products , Edited by: Hulme , A. C. Vol. 2 , 623 – 652 . London : Academic Press .
- Ochoa-Martínez , C. I. and Ayala-Aponte , A. 2005 . Mathematical models of mass transfer in osmotic dehydration . CyTA – Journal of Food , 4 ( 5 ) : 330 – 342 .
- Park , K. J. , Bin , A. , Brod , F. P.R. and Park , T. H.K.B. 2002 . Osmotic dehydration kinetics of pear D'anjou (Pyrus communis L.) . Journal of Food Engineering , 52 : 293 – 298 .
- Palou , E. , Lopez-Malo , A. , Argaiz , A. and Welti , J. 1993 . Osmotic dehydration of papaya. Effect of syrup concentration . Revista Española de Ciencia Y Technologia de Alimentos , 33 ( 6 ) : 621 – 630 .
- Sereno , A. M. , Moreira , D. and Martinez , E. 2001 . Mass transfer coefficients during osmotic dehydration of apple single and combined aqueous solution of sugar and salts . Journal of Food Engineering , 47 : 43 – 49 .
- Singh , H. 2001 . Osmotic dehydration of carrot shreds for Gazraila preparation . Journal of Food Science and Technology , 38 : 152 – 154 .
- Singh , B. , Kumar , A. and Gupta , A. K. 2007 . Study of mass transfer kinetics and effective diffusivity during osmotic dehydration of carrot cubes . Journal of Food Engineering , 79 ( 2 ) : 471 – 480 .
- Sutar , P. P. and Gupta , D. K. 2007 . Mathematical modeling of mass transfer in osmotic dehydration of onion . Journal of Food Engineering , 78 ( 1 ) : 90 – 97 .
Supplementary material
Supplementary Figure 1. Water loss and solid gain vs. time for different solution to sample weight ratios.
Figura adicional 1. Pérdida de agua y aumento de sólido vs. tiempo para diferentes relaciones de solución a peso de muestra.
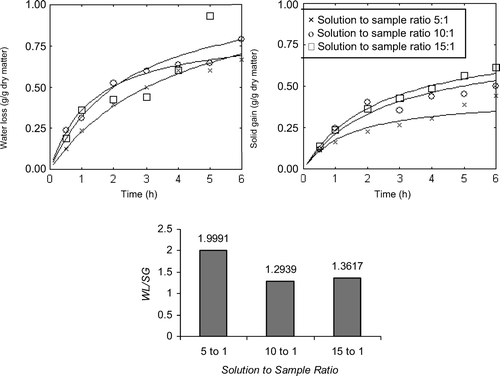
Supplementary Figure 2. Effect of concentration on water loss at 30, 40, 50 or 60 °C.
Figura adicional 2. Efecto de concentración en pérdida de agua a 30, 40, 50 o 60 °C.
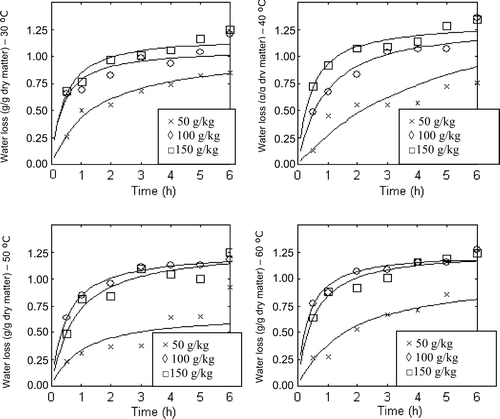
Supplementary Figure 3. Effect of temperature on water loss at 50, 100, 150 g/kg salt solutions.
Figura adicional 3. Efecto de la temperatura en la pérdida de agua a 50, 100, 150 g/kg de soluciones salinas.
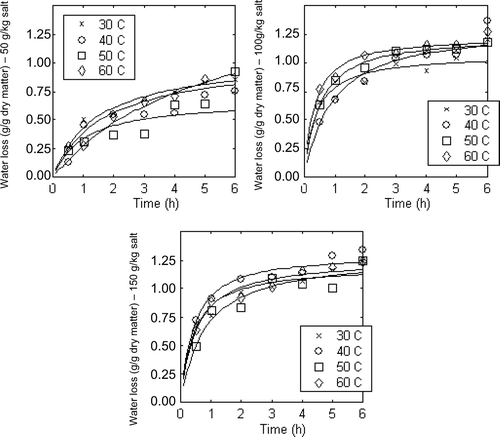
Supplementary Figure 4. Effect of temperature on solid gain at 50, 100, 150 g/kg salt solutions.
Figura adicional 4. Efecto de la temperatura en el aumento de sólido a 50, 100, 150 g/kg de solución salina.
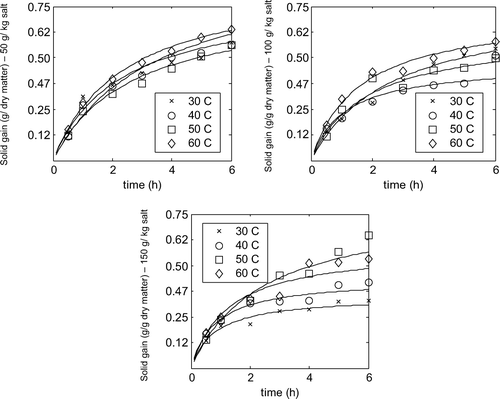