Abstract
The activity of crude extracts from Alaska Pollock muscle responsible for the hydrolysis of myofibrils and perimysia from beef Longissimus Dorsi muscle was investigated. The results showed that myosin heavy chain and myosin light chain were hydrolyzed completely after 12 h of incubation. Tropomyosin, α-actinin, and troponin-T disappeared after 24 h of incubation, whereas the actin was partially degraded. The collagen fibers were so loose that cross-linking among microfibrils occurred after treatment with the crude extracts. Meanwhile, the second structures of myofibrillar proteins were not changed markedly after incubation. The α-helices in perimysia treated with the crude extracts were partially transformed into β-sheets, while the β-turn and the random coil fractions were steady. It was concluded that myofibrils and perimysia from beef Longissimus Dorsi muscle were commendably hydrolyzed by the crude extracts from Alaska Pollock muscle, so that the crude extracts from Alaska Pollock muscle could be utilized as a potential meat tenderizer.
Se investigó la actividad de extractos no purificados de músculo de abadejo Alaska responsables de la hidrólisis de miofibrilos y perimisios de músculo Longissimus Dorsi de buey. Los resultados mostraron que las miosinas de cadena pesada (MHC) y las miosinas de cadena liviana (MLC) fueron hidrolizadas completamente tras 12 h de incubación. La tropomiosina, actinina αy troponina T desaparecieron tras 24 h de incubación, mientras la actina fue parcialmente degradada. Las fibras colágenas estaban tan sueltas que el entrecruzado entre microfibrilos tuvo lugar tras el tratamiento con los extractos no purificados. Mientras, las segundas estructuras de proteínas miofibrilares no mostraron un cambio marcado tras la incubación. Las hélices α en perimisios tratados con extractos no purificados fueron parcialmente transformados en láminas beta, mientras las fracciones de giro beta y de espiral aleatoria se mostraron constantes. Se concluyó que puesto que miofibrilos y perimisios procedentes de músculo Longissimus Dorsi de buey fueron remarcablemente hidrolizables con extractos no purificados de músculo de abadejo Alaska, estos extractos podrían ser utilizados como potencial ablandador de carne.
Introduction
Proteinases mainly existed in muscle fiber, cytoplasm, and extracellular matrix of the connective tissue surrounding the cells of fish muscle (Toyohara, Kinoshita, & Shimizu, Citation1990). Two main proteinases, cathepsins and calpains, played important roles in postmortem fish muscle. They were considered to hydrolyze myofibrillar proteins in the postmortem tenderization process and softening of fish gels (Jiang, Citation2007).
Cathepsin B and L as lysosomal cysteine proteinases existed in several different animals and tissues including liver (Mason, Taylor, & Etherington, Citation1984), brain (Marks & Berg, Citation1987), and skeletal muscle (Okitanil, Matsukura, Kato, & Fujimaki, Citation1980). Salmon muscle softening (Yamashita & Konagaya, Citation1991) and surimi degradation (An, Weerasinghe, Seymour, & Morrissey, Citation1994) have been attributed mostly to cathepsin B and L. Cathepsin B and L were active in the extracts of Alaska Pollock muscle (Porter, Koury, & Stone, Citation1996) and played an important role in gel softening (Luo, Kuwahara, Kaneniwa, Murata, & Yokoyama, Citation2001). All of these reports dealing with the cathepsins were focused on enzyme purification and characterization, as well as the postmortem fish muscle autolysis and gel softening, for further developing the processing technologies and improving the textural quality of surimi products. Cathepsin L was capable of hydrolyzing the major muscle structural proteins, such as connectin, nebulin, myosin, collagen, α-actinin, and troponins-T and -I in postmortem (Yamashita & Konagaya, Citation1991). Although cathepsin B from mackerel could strongly hydrolyze the myosin heavy chain (MHC) of actomyosin, actin was not hydrolyzed (Jiang, Lee, & Chen, Citation1996). Cathepsins B and L from rat liver also hydrolyzed collagen from rat tail tendon (Kirschke, Kembhavi, Bohley, & Barrett, Citation1982).
Calpains had been considered to participate in the postmortem muscle tenderization (Asghar & Bhatti, Citation1988; Koohmaraie, Babiker, Merkel, & Dutson, Citation1988). M-calpain contained in carp muscle and tilapia muscle (Jiang, Wang, & Chen, Citation1991) can rapidly hydrolyze muscle proteins at postmortem pH. Calpains from crustacean muscle could completely hydrolyze myofibrillar proteins (Jiang, Citation2007), but these from vertebrate muscle only selectively cleaved myofibrillar proteins into fragments (Ishioroshi, Samejima, & Yasui, Citation1982).
Myofibrillar components and intramuscular connective tissue had an influence on beef tenderness. The degradation of myofibrillar proteins may contribute to the meat tenderization (J. Han, Morton, Bekhit, & Sedcole, Citation2009), meanwhile extensive proteolysis of the collagen and elastin might appear to be the most likely change causing increased tenderness (Lawrie & Ledward, Citation2006). In recent research, plant proteolytic enzymes such as papain, actinidin (J. Han et al., Citation2009), liquid porcine pancreatin enzyme (Pietrasik, Aalhus, Gibson, & Shand, Citation2010), and microorganismal proteinases (Walsh et al., Citation2010) were effective agents on meat tenderizing. Unfortunately, there was little information available on the effects of natural extracts from fish on beef tenderness. The objectives of this work were to prepare crude extracts from the muscle of Alaska Pollock and to investigate how the crude extracts affect the myofibrils and perimysia from beef Longissimus Dorsi muscle with the aim to explore the feasibility of using as a commercial meat tenderizer.
Materials and methods
Materials
Alaska Pollock fillet (200–300 g/piece) which was caught from North Pacific was obtained from Metro market in Nanjing city, China. Beef Longissimus Dorsi muscle were bought from a local market near the campus of Nanjing Agricultural University. The fillet was packaged in vacuum and then stored at 0–4°C, and the beef samples were aged for 48 h at 0–4°C before the experiments. Benzyloxycarbonyl-arginylarginine-4-methyl-7-coumarylamide (Z-Arg-Arg-MCA), benzyloxycarbonyl-L-phenylalany-L-arginyl-4-methyl-7-coumarylamide (Z-Phe-Arg-MCA), and molecular weight marker were purchased from Sigma Chemical Co. (St. Louis, MI, USA). All the other chemicals used were of analytical grade.
Preparation of crude extracts
The crude extracts were prepared based on the procedure of Porter et al. (Citation1996). The Alaska Pollock fillet was frozen and homogenized with three volumes of 4°C extraction buffer (0.02 mol L−1 sodium acetate, pH 5.8, 1% NaCl, and 0.02% sodium azide) for 1.5 min in a Waring Blender (8010ES; Waring Commercial, New Hartford, NY, USA). The homogenate was left for 1 h at 4°C and then centrifuged for 20 min at 10,400 × g. The supernatant was then filtered to remove suspended fats to yield the crude extracts.
The optimum pH and temperature
The optimum pH and temperature of crude extracts were determined by the activity of cathepsin B and L. Enzyme activity was determined as described by Liu, Yin, Zhang, Li, & Ma (Citation2006). Briefly, Z-Arg-Arg-MCA and Z-Phe-Arg-MCA were used as substrates for cathepsin B and cathepsin L, respectively. Both the solutions were diluted to 500 μL by 0.1% Brij35. Either 250 μL of 0.4 mol L−1 phosphate buffer for cathepsin B or the same volume of 0.4 mol L−1 acetate buffer for cathepsin L was added to the crude extracts, respectively. The resulting solutions were preheated for 2 min followed by the addition of 250 μL of substrate with a concentration of 20 μmol L−1. The mixture was incubated for 10 min. Subsequently, 1 mL of stopping solution consisting of 0.1 mol L−1 acetate and 0.1 mol L−1 sodium chloroacetate buffer (pH 4.3) was added to the mixture for terminating the hydrolysis. A control tube was prepared in parallel for each test sample, but the stopping solution was added before the addition of the enzyme solution. The buffer pH was assayed in the range of 4.0–7.5; the determination of temperature activity was carried out in the temperature range from 20°C to 80°C. The relative fluorescence intensity of aminomethylcoumarin (AMC) liberated by hydrolysis was measured by a Microplate Spectrophotometer (SPECTRA max PLUS384; Molecular Devices Corp., Sunnyvale, CA, USA) with 380 nm of excitation wavelength and 460 nm of emission wavelength.
Extraction of myofibrillar proteins
Extraction of myofibrillar proteins was carried out as described by M. Han, Zhang, Fei, Xu, & Zhou (Citation2009). Beef Longissimus Dorsi muscle was ground, homogenized at 7000 rpm min−1 for 3 min, and washed using four volumes of a post-rigor extraction buffer (100 mmol L−1 Tris, 10 mmol L−1 EDTA, pH 8.3) in a Waring Blender (8010ES; Waring Commercial). Samples were then centrifuged 1000 × g for 20 min (Beckman Avanti J-E centrifuge; Beckman Coulter, Brea, USA). The myofibrillar pellet was then re-suspended and washed using a Ultra Turrax T25 BASIC (IKA Labortechnik, Staufen, Germany) at 5000 rpm min−1 for 1 min with four volumes of a standard salt solution (SSS) (100 mmol L−1 KCl, 20 mmol L−1 K2HPO4/KH2PO4, 2 mmol L−1 MgCl2, 1 mmol L−1 EGTA, 1 mmol L−1 NaN3, and pH 7.0) and then centrifuged at 1000 × g for 10 min. The procedure was repeated three times from the re-suspending and washing step. The pellet was re-suspended and washed with four volumes of SSS with 1% Triton X-100 and centrifuged for 10 min at 1500 × g, which was repeated two times. Then the pellet was re-suspended and washed with four volumes of SSS and centrifuged for 10 min at 1500 × g, and the process mentioned above was repeated twice from the pellet re-suspending and washing step with four volumes of SSS with 1% Triton X-100. The pellet was re-suspended and washed with four volumes of 0.1 mmol L−1 KCl and centrifuged for 10 min at 1500 × g to collect the pellet, which was repeated two additional times. The pellet was then re-suspended and washed in four volumes of 0.1 mmol L−1 NaCl and centrifuged for 10 min at 1500 × g. Then the myofibrillar proteins were obtained from the precipitate. The protein content of the final pellet was determined by the Biuret method (Gornall, Bardawill, & David, Citation1949) using bovine serum albumin (BSA) as standard. The beef myofibrillar protein (BMP) pellet was dissolved in 50 mM phosphate buffer (pH 6.5) containing 0.6 mol L−1 NaCl and stored at 4°C within 24 h.
Degradation of BMP
About 10 mg BMP dissolved in 50 mmol L−1 phosphate buffer (pH 6.5) was incubated for 1 h at the optimum temperature with concentrations of crude extracts (0.25%, 0.5%, 0.75%, and 1%; crude extract/protein, v/m). About 10 mg BMP dissolved in 50 mmol L−1 phosphate buffer (pH 6.5) was incubated for 1 h, 3 h, 6 h, 9 h, 12 h, and 24 h at the optimum temperature with 1% crude extracts. The protein concentration was finally adjusted to around 1 mg mL−1 using the same buffer solution and degradation of BMP was assayed by means of SDS-PAGE.
Degradation of the perimysia
The perimysia were prepared according to the method of Light & Champion (Citation1984). The beef Longissimus Dorsi muscle with epimysium away was cut into 1 cm cubes, homogenized with two volumes ice-cold 0.05M CaCl2 for 10 s in a Waring Blendor (8010ES; Waring Commercial). The homogenate was filtered and then re-homogenized in a further two volumes of ice-cold 0.05 mol L−1 CaCl2 and were re-filtered. This process was repeated two times, and the retained was the gross connective tissue, referring to as the perimysial fraction. About 1 g perimysia were incubated with concentrations (0.25%, 0.5%, 0.75%, and 1%, respectively) of crude extracts (crude extract/protein, v/m) at the optimum temperature for 1 h. About 1 g perimysia were incubated for 3 h, 6 h, 9 h, and 12 h with 1% crude extracts at the optimum temperature, respectively. Afterward, the perimysia were stored overnight at 0–4°C in a refrigerator for scanning electron microscopy (SEM) and Raman test.
Sodium dodecyl sulfate polyacrylamide gel electrophoresis (SDS-PAGE)
The SDS-PAGE was performed according to Laemmli (Citation1970) using a 12.5% acrylamide separating gel and a 4% acrylamide stacking gel in a Mini-PROTEAN® Tetra cell apparatus (BioRad, Beijing, China). Each sample containing about 1 mg protein was loaded on the gel. After electrophoretic development, gels were stained with Coomassie Brilliant Blue R-250 and destained overnight with a solution of 10% (v/v) methanol and 10% (v/v) acetic acid.
Circular dichroism spectrum
The protein concentration was adjusted to around 0.1 mg mL−1 using 50 mmol L−1 phosphate buffer (pH 6.5) solution, and secondary structures of BMP were assayed by circular dichroism spectrum. Circular dichroism spectrum was measured using Chirascan CD Spectrometer (Applied Photophysics Ltd., England).
Myofibrillar protein samples were transferred to a quartz cell with a 1 cm light-path length. Molecular ellipticity was measured in the range from 250 to 200 nm, and the scan rate was 100 nm min−1. Spectra were averaged over three scans and corrected for the buffer signal. A mean residue weight of 110 g mol−1 has been assumed. The percentages of α-helix, β-sheet, β-turn, and random coil structures were determined using the DICHROWEB Web site ( http://dichroweb.cryst.bbk.ac.uk/html/process.shtml) and as described by Whitmore & Wallace (Citation2008).
Scanning electron microscopy
The perimysia samples were cut into 2 × 2 mm, pre-fixed in 2.5% glutaraldehyde solution, and washed with 0.1 mol L−1 phosphate buffer (pH 7.2). Then, the samples were dehydrated in increasing concentrations of acetone (30, 45, 60, 75, 90, and 100%), critical-point-dried, and sputter-coated with 10 nm of gold. The micrographs were taken at a voltage of 15 kV with a Hitachi S-3000 N scanning electron microscope (Hitachi High-technologies Corporation, Tokyo, Japan). A large number of micrographs were taken to select representative micrographs.
Raman spectroscopy analysis
Raman experiments were conducted with a Jobin Yvon Labram HR800 spectrometer (Horiba/Jobin. Yvon, Longjumeau, France) and determined using the procedure described by Xu, Han, Fei, & Zhou (Citation2011). Spectra were smoothed, baseline corrected, and normalized against the phenylalanine band at 1003 cm−1 using Labspec version 3.01c (Horiba/Jobin. Yvon, Long-jumeau, France). In the most prominent band, the 1700–1600 cm−1 range has been assigned unambiguously to the amide I vibrational mode, the percentages of secondary structure in a protein can be estimated with accuracy and a good level of confidence from the frequency of the peak of the Raman Amide I band (Alix, Pedanou, & Berjot, Citation1988). Protein secondary structures were determined as percentages of α-helix, β-sheet, β-turn, and random coil using Alix's method (Alix et al., Citation1988).
Statistical analysis
All experiments were analyzed three times in duplicate. The results were obtained by using Statistical Analysis System (SAS8.12, SAS Institute Inc., Cary, NC, USA). Analysis of variance (ANOVA) was employed to determine the significance of main effects. Significant differences (p < 0.05) between means were identified using Duncan's multiple range tests.
Results and discussion
Effect of pH and temperature on crude extracts activity
As shown in , cathepsin B and L in crude extracts were active over a broad pH range, displaying maximum activity at pH 5.0. The finding was in agreement with Liu et al. (Citation2006), who reported that the optimum pH for silver carp cathepsin L was 5.0. Similar to our observation, Ogata, Aranishi, Hara, Osatomi, & Ishihara (Citation1998) showed that the carp cathepsin L had a potent hydrolytic action around pH 5.5. Ho, Chen, & Jiang (Citation2000) also found that the optimum conditions for the hydrolysis of MHC from mackerel surimi was pH 5.5–7.0 for cathepsin L and cathepsin L-like. While the optimum pH of cathepsin B was 6.0 in hepatopancreas of carp cyprinus carpio (Aranishi, Hara, Osatomi, & Ishihara, Citation1997a) and 6.5 in mackerel (Jiang, Lee, & Chen, Citation1994), which were more alkaline than that from Alaska Pollock muscle. At pH 5.5–6.5, the cathepsins in the crude extracts still remained 25%–90% of its maximum activity in the present study.
Figure 1. Effects of pH on cathepsin B and L in crude extracts activity.
Figura 1. Efectos del pH en catepsina B y L en la actividad de extractos no purificados.
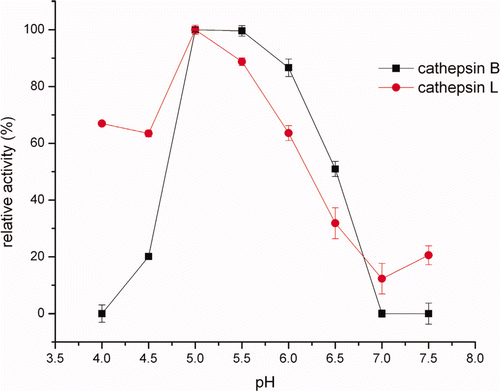
Cathepsin B and L in the crude extracts were found to have an optimum temperature of 50°C (). Furthermore, the relative activity increased from 20°C to 50°C and then dropped at higher temperatures (). Endogenous heat-stable cathepsins in some fish muscle lysosomes could be assumed as being involved in causing gel softening effect during gelation of surimi (An et al., Citation1994). Luo et al. (Citation2001) showed that Alaska Pollock surimi exhibited considerable gel softening in the course of heating, especially at 50°C. According to Kinoshita, Toyohara, Shimizu, & Sakaguchi (Citation1992), there was a modori-inducing protease (MIP) with an optimum temperature of 50°C in sarcoplasmic fluid of threadfin bream muscle. As a close comparison, the optimum temperature of cathepsin B from mackerel for the activity assay was 55°C (Jiang et al., Citation1994) and 45°C (Aranishi et al., Citation1997a), and cathepsin L from hepatopancreas of carp cyprinus carpio exhibited a maximum activity at 50°C (Aranishi, Ogata, Hara, Osatomi, & Ishihara, Citation1997b).
Degradation of BMP
Comparisons of the electropherograms of the BMP incubated with and without varying concentrations of crude extracts were showed in . As the concentration increased, the intensity of MHC (MW 200 kDa) on BMP in the crude extracts decreased. The degradation of the high MW component (MW > 250 kDa) was enhanced, with the concentrations of crude extracts. However, actin (MW 43 kDa) seemed to be resistant to proteolysis by the crude extracts. Obviously, it was suggested that crude extracts could strongly hydrolyze the MHC of BMP but did not hydrolyze actin after about 1 h of incubation.
Figure 3. Effect of concentrations of the crude extracts on the degradation of BMP after 1 h of incubation at 50°C. Lanes: a, protein standards; b, the control, BMP without crude extracts after 24 h of incubation at 0–4°C; c, with 0.25%; d, with 0.5%; e, with 0.75%; f, with 1% of crude extracts (v/m).
Figura 3. Efectos de concentraciones de los extractos no purificados en la degradación de proteínas miofibrilares de buey tras 1 h de incubación a 50°C. Carriles: a, proteína estándar; b, control; BMP sin extractos no purificados tras 24 h de incubación a 0–4°C; c, con 0.25%; d, con 0.5%; e, con 0.75%; f, con 1% de extractos no purificados (v/m).
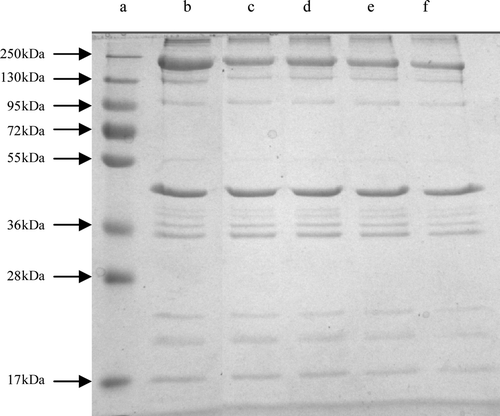
Degradation of BMP was observed on SDS-PAGE during 1 h, 3 h, 6 h, 9 h, 12 h and 24 h of incubation at 50°C (). Compared to the control, the MHC was degraded gradually as the incubation time was increased. The MHC and myosin light chain (MLC, MW 20 kDa) werecompletely hydrolyzed after 12 h of incubation; however, the actin bands were partially degraded. These results may be due to the action of proteinases in the crude extracts of Alaska Pollock muscle. Proteolytic activity in muscles is high at temperatures above 50°C and causes the rapid and severe degradation of myofibrillar proteins, particularly myosin (Wasson, Babbitt, & French, Citation1993). The results of the present study were similar to the work of Jiang, Lee, Tsao, & Lee (Citation1997), who showed that cathepsins B and L or purified cathepsin B of mackerel muscle not only degraded MHC but also partially degraded actin in pH 6.5 or 7.0 after 5 h of incubation at 55°C. Jiang et al. (Citation1996) indicated that actomyosin of mackerel surimi was incubated at 37°C for 12 h; degradation of MHC at pH 6.0–7.5 might be due to the action of calpains. However, no visible change was observed in electrophoretical profiles of actin until 12 h of incubation in the study. The proteinases can hydrolyze not only the fish proteins but also beef, suggesting that the crude extracts from Alaska Pollock muscle could act on proteins from the other species.
Figure 4. Effect of 1% the crude extracts (v/m) on the degradation of BMP. Lanes: a, protein standards; b, the control, BMP without crude extracts after 24 h of incubation at 0–4°C; c, after 1 h of incubation; d, after 3 h of incubation; e, after 6 h of incubation; f, after 9 h of incubation; g, after 12 h of incubation; h, after 24 h of incubation.
Figura 4. Efecto de 1% de extractos no purificados (v/m) en la degradación de proteínas miofibrilares de buey. Carriles: a, proteína estandar; b, control; BMP sin extractos no purificados tras 24 h de incubación a 0–4°C; c, tras 1 h de incubación; d, tras 3 h de incubación; e, tras 6 horas de incubación; f, tras 9 h de incubación; g, tras 12 h de incubación; h, tras 24 h de incubación.
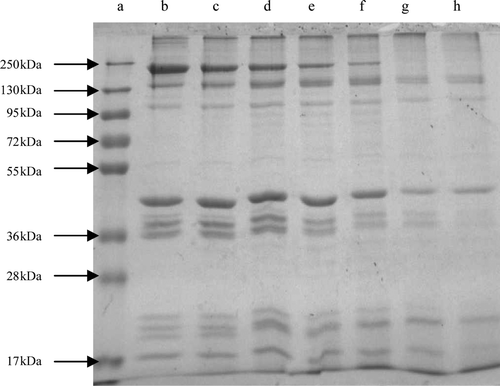
Disappearance of α-actinin (MW 95 kDa), troponin-T (MW 39 kDa), and tropomyosin (MW 36 kDa) was accompanied after 24 h of incubation. Yamashita & Konagaya (Citation1991) reported that the amounts of α-actinin and troponin-T markedly decreased after interaction with the salmon cathepsins B and L, along with the disappearance of myosin heavy and light chains. An et al. (Citation1994) also reported that rapid hydrolysis of troponin-T and tropomyosin was occurred by the Pacific whiting cathepsins L. These results were all generally corresponding with our experiment.
Changes of secondary structures of BMP
and showed the CD spectra and secondary structure fractions of BMP solutions incubated with concentrations of crude extracts for different hours. As shown in (b) and 6(b), α-helical and β-sheet fraction content in BMP was 29% and 18% at the control (4°C), respectively. Then, they were rapidly changed to 8% and 44% with 0.25% crude extracts incubated at 50°C. The changes of secondary structures were induced by the hydrolyzed activity and incubation temperature. The myofibrillar proteins were hydrolyzed with the proteinases in the crude extracts, indicating that approximately 17% of the α-helical portions of protein molecules unfolded. And many reports found that α-helical content of myosin was decreased with the high temperature (Liu, Zhao, Xiong, Xie, & Liu, Citation2007; Liu, Zhao, Xiong, Xie, & Qin, Citation2008; Ogawa, Ehara, Tamiya, & Tsuchiya, Citation1993), which was consistent with the present result. Otherwise, the MHC, actin, α-actinin, troponin-T, and tropomyosin were hydrolyzed into small fractions along with the concentrations of extracts and incubation time, but the secondary structure fractions of BMP was stable (p > 0.05). It was indicated that the content of secondary structure was not changed with the proteinase concentration and hydrolyzation time.
Figure 5. CD spectra (a) and secondary structures (b) of BMP as incubated with concentrations of the crude extracts.
Figura 5. Espectros de dicroismo circular (a) y estructuras secundarias (b) de proteínas miofibrilares de buey incubadas con concentraciones de extractos no purificados.
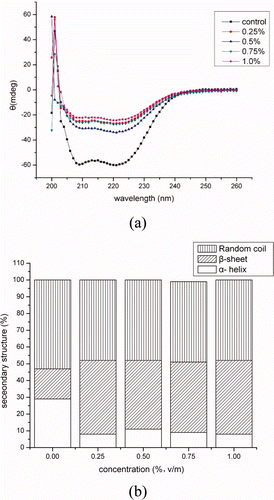
Figure 6. CD spectra (a) and secondary structures (b) of BMP as incubated with 1% crude extracts after 1 h, 3 h, 6 h, 9 h, 12 h and 24 h of incubation.
Figura 6. Espectros de dicroismo circular (a) y estructuras secundarias (b) de proteínas miofibrilares de buey incubadas con 1% de extractos no purificados tras 1 h, 3 h, 6 h, 12 h y 24 h de incubación.
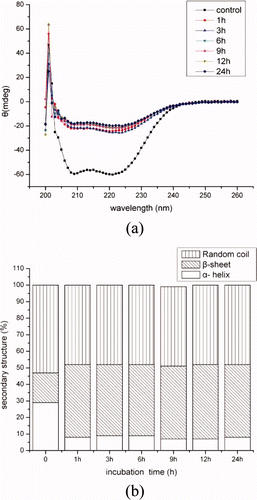
Scanning electron microscopy
The SEM images of perimysia incubated with the crude extracts were presented in and , respectively. The collagen fibers of the control appeared more closely than the treatments ((a) and 8(a)). The collagen fibers incubated with the 0.25% crude extracts became less crowded ((b)), and then they were dissolved into collagen macrofibrils after treating with 0.5% crude extracts ((c)). With the rising concentration of the crude extracts, collagen macrofibrils were loose and linkages between partial collagen microfibrils were formed ((d) and 7(e)). With more incubation time, the more linkages were formed ((b) and 8(c)), while intact collagen fibers and collagen macrofibrils were also found. After perimysia were incubated for 9 h ((d)) and 12 h ((e)), much more cross-linking was formed among collagen microfibrils, like a net. The SEM images of all treatments illustrated that the crude extracts of Alaska Pollock muscle efficiently hydrolyzed the perimysia from beef, likely caused by the cathepsins; however, how the linkages formed will be investigated further.
Figure 7. Scanning electron microscopy images of perimysia treatments: (a) control, (b) with 0.25%; (c) with 0.5%; (d) with 0.75%; and (e) with 1% of crude extracts (v/m) at 3000 × magnification.
Figura 7. Imagenes de Microscopia elctronica de barrido de tratamientos de perimisia: (a) control; (b) con 0.25%; (c), con 0.5%; (d) con 0.75%; (e) con 1% de extratos no purificados (v/m) a aumento 3000×.
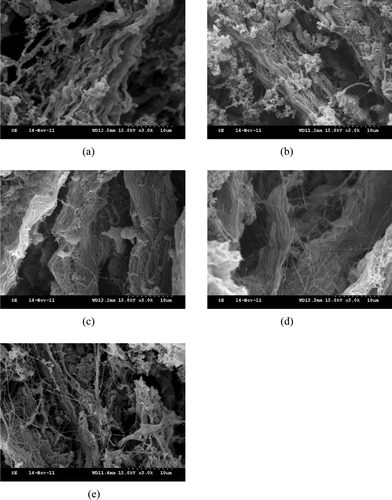
Figure 8. Scanning electron microscopy images of perimysia treated with 1% crude extracts: (a) control; (b) after 3 h of incubation; (c) after 6 h of incubation; (d) after 9 h of incubation; and (e) after 12 h of incubation at 3000 × magnification.
Figura 8. Imagenes de Microscopia elctronica de barrido de perimisios tratados con 1% de extractos no purificados: (a) control; (b) tras 3 h de incubación; (c) tras 6 h de incubación; (d) tras 9 h de incubación; (e) tras 12 h de incubación con aumento 3000×.
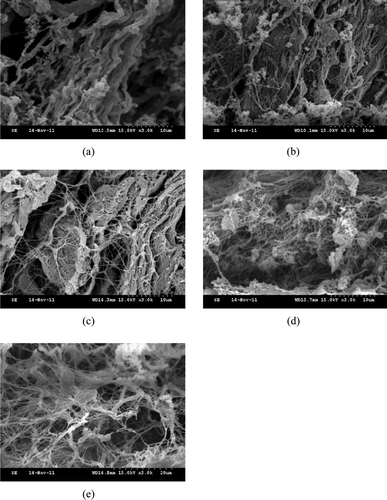
Changes of secondary structures of perimysia
According to Alix et al. (Citation1988), Tu (Citation1982), and Xu et al. (Citation2011), there was a correlation between the frequencies of the amide I band and the amount of the types of protein backbone conformation. The nature of the amide I band made it sensitive to change in the hydrogen bonding scheme involving the peptide linkages. The amide I band consisted of overlapped band components falling in the 1658–1650, 1680–1665, and 1665–1660 cm–1 range, which were attributable to α-helices, β-sheets, and random coil structures, respectively.
Raman spectra of perimysia treated with concentrations of the crude extracts for 3 h, 6 h, 9 h, and 12 h in the 400–2000 cm−1 region were shown in and . The results of quantitative estimation of the secondary structure fractions were shown as and . As the concentrations of the crude extracts increased from 0 to 0.75%, the α-helices content markedly decreased from 73.1% to 20%, the β-sheets fraction increased rapidly from 5.7% to 46.4%, and the β-turns and random coil fraction also tended to increase (). As shown in , the α-helices content showed a rapid decrease to 8.26% after 6 h of incubation. On the contrary, the β-sheets fraction was increased to 55.44% and then dropped with the incubation time. The work of Kirschke et al. (Citation1982), whose result was opposite to ours, which investigated the nature of the action of the cathepsin L on acid-soluble collagen from rat tail tendon for 30 min incubation at 24°C (pH 3.5) with SDS-PAGE electrophoresis, showed that cathepsin L, like cathepsin B, caused a decrease in the amount of the cross-linked β- and higher components, as compared with the control, and an increase in the uncross-linked α-chains. It may be due to the collagen and proteinase sources; in addition, the incubation temperature and time may also be responsible for the secondary structures.
Figure 9. Raman spectra in the 400–2000 cm−1 region from perimysia incubated with concentrations of the crude extracts: (a) the control; (b) with 0.25%; (c) with 0.5%; (d) with 0.75%; and (e) with 1% of crude extracts (v/m).
Figura 9. Espectros de Raman en la región de 400–2000 cm−1 de perimisios incubados con concentraciones de extractos no purificados: (a) control; (b) con 0.25%; (c); con 0.5%; (d) con 0.75%; (e) con 1% de extratos no purificados (v/m).
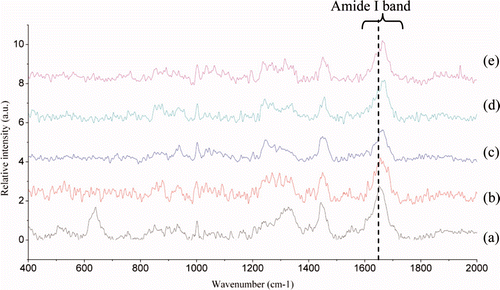
Figure 10. Raman spectra in the 400–2000 cm−1 region from perimysia treated with 1% crude extracts: (a) the control; (b) after 3 h of incubation; (c) after 6 h of incubation; (d) after 9 h of incubation; and (e) after 12 h of incubation.
Figura 10. Espectros de Raman en la región de 400–2000 cm−1 de perimisios tatrados con 1% de extractos no purificados: (a) control; (b) tras 3 h de incubación; (c) tras 6 h de incubación; (d) tras 9 h de incubación; (e) tras 12 h de incubación.
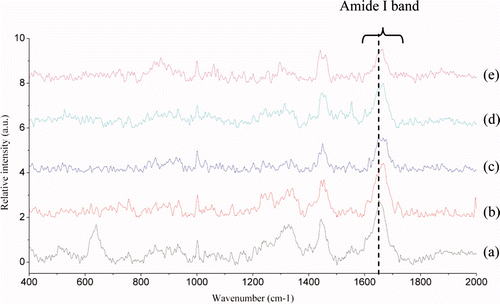
Figure 11. Secondary structure fractions estimated from the amide I band as a function of concentrations of the crude extracts.
Figura 11. Fracciones de estructura secundaria estimadas por la banda amida I como función de concentraciones de extractos no purificados.
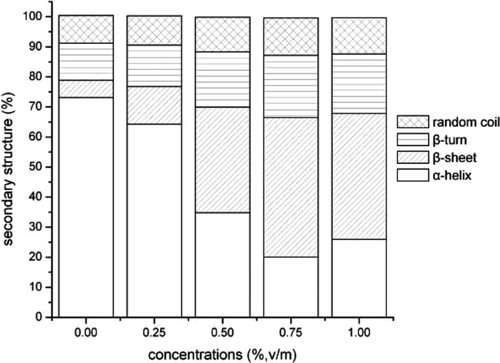
Figure 12. Secondary structure fractions estimated from the amide I band as a function of incubation time.
Figura 12. Fracciones de estructura secundaria estimadas por la banda amida I como función de tiempo de incubación.
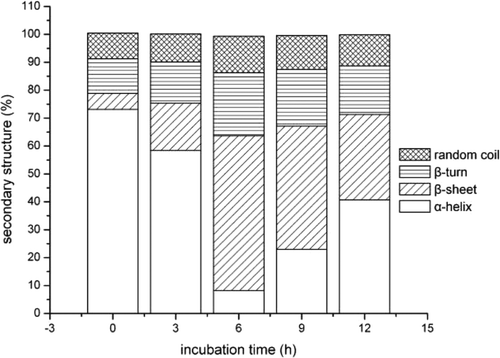
The changes of secondary structures might be due to hydrolysis of collagen by the crude extracts, which was supported by Marks & Berg (Citation1987), who reported that peptide bonds connected with hydrophobic amino acids such as Phe, Leu, Val, Trp, Tyr, or Met were cleaved, releasing Tyr-Gly and Gly-Phe-Leu (-Met), resulting in exposing much more protein branched chains; therefore, unfolded polypeptides had an innate tendency to form aggregates (Dobson, Citation2003) that were composed largely of β-sheets (Nelson et al., Citation2005) with the concentration and incubation time. Until 6 h of incubation, the protein chains were almost turned into β-sheets and then the hydrogen bonds between C˭O and N‒H on the terminal of non-helical peptides made it possible to form new α-helices. On the other side, the appearance of much more proline and glycin residues resulted in the decrease of β-sheets fraction with the incubation time, which was supported by Kim & Berg (Citation1993), who indicated that proline and glycin had the lowest propensity to form a β-sheet.
Conclusions
In conclusion, our findings showed that the crude extracts from Alaska Pollock muscle were very active in degradation of myosin, actin, α-actinin, troponin-T, and tropomyosin from beef, while they did not change the secondary structures as the concentration and incubation time. Incubation with the crude extracts let collagen fibers become collagen macrofibrils and microfibrils, the latter further cross-linked each other to form a net. During the course, α-helices and β-sheets of collagen from beef Longissimus Dorsi muscle transformed. This implied that the crude extracts from Alaska Pollock muscle would be a potential trend for tenderizing meat.
Acknowledgements
This research work was supported by the earmarked fund for modern Agro-industry Technology Research System (nycytx-38).
References
- Alix , A.J.P. , Pedanou , G. and Berjot , M. 1988 . Fast determination of the quantitative secondary structure of proteins by using some parameters of the Raman Amide I band . Journal of Molecular Structure , 174 : 159 – 164 . doi: 10.1016/0022-2860(88)80151-0
- An , H. , Weerasinghe , V. , Seymour , T.A. and Morrissey , M.T. 1994 . Cathepsin degradation of Pacific whiting surimi proteins . Journal of Food Science , 59 : 1013 – 1017 . doi: 10.1111/j.1365-2621.1994.tb08179.x
- Aranishi , F. , Hara , K. , Osatomi , K. and Ishihara , T. 1997a . Purification and characterization of cathepsin B from hepatopancreas of carp Cyprinus carpio . Comparative Biochemistry and Physiology Part B: Biochemistry and Molecular Biology , 117 : 579 – 587 . doi: 10.1016/s0305-0491(97)00191-0
- Aranishi , F. , Ogata , H. , Hara , K. , Osatomi , K. and Ishihara , T. 1997b . Purification and characterization of cathepsin L from hepatopancreas of carp Cyprinus carpio . Comparative Biochemistry and Physiology Part B: Biochemistry and Molecular Biology , 118 : 531 – 537 . doi: 10.1016/s0305-0491(97)00111-9
- Asghar , A. and Bhatti , A.R. 1988 . Endogenous proteolytic enzymes in skeletal muscle: Their significance in muscle physiology and during postmortem aging events in carcasses . Advances in Food Research , 31 : 343 – 451 . Retrieved from http://www.ncbi.nlm.nih.gov/pubmed/3328484
- Dobson , C.M. 2003 . Protein folding and misfolding . Nature , 426 : 884 – 890 . Retrieved from http://www.ncbi.nlm.nih.gov/pubmed/14685248
- Gornall , A.G. , Bardawill , C.J. and David , M.M. 1949 . Determination of serum proteins by means of the biuret reaction . The Journal of Biological Chemistry , 177 : 751 – 766 . Retrieved from http://www.jbc.org/content/177/2/751.short
- Han , J. , Morton , J.D. , Bekhit , A.E.D. and Sedcole , J.R. 2009 . Pre-rigor infusion with kiwifruit juice improves lamb tenderness . Meat Science , 82 : 324 – 330 . doi: 10.1016/j.meatsci.2009.02.003
- Han , M. , Zhang , Y. , Fei , Y. , Xu , X. and Zhou , G. 2009 . Effect of microbial transglutaminase on NMR relaxometry and microstructure of pork myofibrillar protein gel . European Food Research and Technology , 228 : 665 – 670 . doi: 10.1007/s00217-008-0976-x
- Ho , M.L. , Chen , G.H. and Jiang , S.T. 2000 . Effects of mackerel cathepsins L and L-like, and calpain on the degradation of mackerel surimi . Fisheries Science , 66 : 558 – 568 . doi: 10.1046/j.1444-2906.2000.00087.x
- Ishioroshi , M. , Samejima , K. and Yasui , T. 1982 . Further studies on the roles of the head and tall regions of the myosin molecule in heat-induced gelation . Journal of Food Science , 47 ( 1 ) : 114 – 120 . doi: 10.1111/j.1365-2621.1982.tb11040.x
- Jiang , S.T. 2007 . Effects of proteinases on the seafood quality . Journal of the Fisheries Society of Taiwan , 34 : 111 – 145 . Retrieved from http://catalog.digitalarchives.tw/item/00/32/dd/19.html
- Jiang , S.T. , Lee , B.L. , Tsao , C.Y. and Lee , J.J. 1997 . Mackerel cathepsins B and L effects on thermal degradation of surimi . Journal of Food Science , 62 : 310 – 315 . doi: 10.1111/j.1365-2621.1997.tb03991.x
- Jiang , S.T. , Lee , J.J. and Chen , H.C. 1994 . Purification and characterization of cathepsin B from ordinary muscle of mackerel (Scomber australasicus) . Journal of Agricultural and Food Chemistry , 42 : 1073 – 1079 . doi: 10.1021/jf00041a005
- Jiang , S.T. , Lee , J.J. and Chen , H.C. 1996 . Proteolysis of actomyosin by cathepsins B, L, L-like, and X from mackerel (Scomber australasicus) . Journal of Agricultural and Food Chemistry , 44 : 769 – 773 . doi: 10.1021/jf950250c
- Jiang , S.T. , Wang , J.H. and Chen , C.S. 1991 . Purification and some properties of calpain II from tilapia muscle (Tilapia nilotica × Tilapia aurea) . Journal of Agricultural and Food Chemistry , 39 : 237 – 241 . doi: 10.1021/jf00002a002
- Kim , C.A. and Berg , J.M. 1993 . Thermodynamic beta-sheet propensities measured using a zinc-finger host peptide . Nature , 362 : 267 – 270 . Retrieved from http://www.ncbi.nlm.nih.gov/pubmed/8459852
- Kinoshita , M. , Toyohara , H. , Shimizu , Y. and Sakaguchi , M. 1992 . Modori-inducing proteinase active at 50°C in threadfin bream muscle . Nippon Suisan Gakkaishi , 58 : 715 – 720 . Retrieved from http://www.journalarchive.jst.go.jp/english/jnlabstract_en.php?cdjournal=suisan1932&cdvol=58&noissue=4&startpage=715
- Kirschke , H. , Kembhavi , A.A. , Bohley , P. and Barrett , A.J. 1982 . Actin of rat liver cathepsin L on collagen and other substrates . Biochemistry Journal , 201 : 367 – 372 . http://www.ncbi.nlm.nih.gov/pmc/articles/PMC1163652/
- Koohmaraie , M. , Babiker , A.S. , Merkel , R.A. and Dutson , T.R. 1988 . Role of Ca+ + -dependent proteases and lysosomal enyzmes in postmortem changes in bovine skeletal muscle . Journal of Food Science , 53 : 1253 – 1257 . doi: 10.1111/j.1365-2621.1988.tb09251.x
- Laemmli , U.K. 1970 . Cleavage of structural proteins during the assembly of the head of bacteriophage T4 . Nature , 227 : 680 – 685 . doi: 10.1038/227680a0
- Lawrie , R.A. and Ledward , D.A. 2006 . Lawrie's meat science , (7th ed) , New York , Washington DC : Woodhead Publishing Limited and CRC Press LLC .
- Light , N. and Champion , A.E. 1984 . Characterization of muscle epimysium, perimysium and endomysium collagens . Biochemistry Journal , 219 : 1017 – 1026 . Retrieved from http://www.ncbi.nlm.nih.gov/pmc/articles/PMC1153576/
- Liu , H. , Yin , L. , Zhang , N. , Li , S. and Ma , C. 2006 . Purification and characterization of cathepsin L from the muscle of silver carp (Hypophthalmichthys molitrix) . Journal of Agricultural and Food Chemistry , 54 : 9584 – 9591 . doi: 10.1021/jf062038m
- Liu , R. , Zhao , S. m. , Xiong , S. b. , Xie , B. j. and Liu , H.m. 2007 . Studies on fish and pork paste gelation by dynamic rheology and circular dichroism . Journal of Food Science , 72 : 399 – 403 . doi: 10.1111/j.1750-3841.2007.00470.x
- Liu , R. , Zhao , S. m. , Xiong , S. b. , Xie , B. j. and Qin , L.h. 2008 . Role of secondary structures in the gelation of porcine myosin at different pH values . Meat Science , 80 : 632 – 639 . doi: 10.1016/j.meatsci.2008.02.014
- Luo , Y.K. , Kuwahara , R. , Kaneniwa , M. , Murata , Y. and Yokoyama , M. 2001 . Comparison of gel properties of surimi from alaska pollock and three freshwater fish species: Effects of thermal processing and protein concentration . Journal of Food Science , 66 : 548 – 554 . doi: 10.1111/j.1365-2621.2001.tb04600.x
- Marks , N. and Berg , M.J. 1987 . Rat brain cathepsin L: Characterization and differentiation from cathepsin B utilizing opioid peptides . Archives of Biochemistry and Biophysics , 259 ( 1 ) : 131 – 143 . doi: 10.1016/0003-9861(87)90478-4
- Mason , R.W. , Taylor , M.A.J. and Etherington , D.J. 1984 . The purification and properties of cathepsin L from rabbit liver . Biochemistry Journal , 217 : 209 – 217 . Retrieved from http://www.ncbi.nlm.nih.gov/pmc/articles/PMC1153198/
- Nelson , R. , Sawaya , M.R. , Balbirnie , M. , Madsen , A. , Riekel , C. , Grothe , R., and Eisenberg , D. 2005 . Structure of the cross-β spine of amyloid-like fibrils . Nature , 435 : 773 – 778 . doi:10.1038/nature03680
- Ogata , H. , Aranishi , F. , Hara , K. , Osatomi , K. and Ishihara , T. 1998 . Proteolytic degradation of myofibrillar components by carp cathepsin L . Journal of the Science of Food and Agriculture , 76 : 499 – 504 . doi: 10.1002/(sici)1097-0010(199804)76:4<499::aid-jsfa980>3.0.co;2-w
- Ogawa , M. , Ehara , T. , Tamiya , T. and Tsuchiya , T. 1993 . Thermal stability of fish myosin . Comparative Biochemistry and Physiology Part B: Comparative Biochemistry , 106 : 517 – 521 . doi: 10.1016/0305–0491(93)90126-p
- Okitanil , A. , Matsukura , U. , Kato , H. and Fujimaki , M. 1980 . Purification and some properties of a myofibrillar protein-degrading protease, cathepsin L, from rabbit skeletal muscle . Journal of Biochemistry , 87 : 1133 – 1143 .
- Pietrasik , Z. , Aalhus , J.L. , Gibson , L.L. and Shand , P.J. 2010 . Influence of blade tenderization, moisture enhancement and pancreatin enzyme treatment on the processing characteristics and tenderness of beef semitendinosus muscle . Meat Science , 84 : 512 – 517 . doi: 10.1016/j.meatsci.2009.10.006
- Porter , R. , Koury , B. and Stone , F. 1996 . Comparison of cathepsin B, D, H and L activity in four species of pacific fish . Journal of Food Biochemistry , 19 : 429 – 442 . doi: 10.1111/j.1745–4514.1995.tb00546.x
- Toyohara , H. , Kinoshita , M. and Shimizu , Y. 1990 . Proteolytic degradation of threadfin-bream meat gel . Journal of Food Science , 55 ( 1 ) : 259 – 260 . doi: 10.1111/j.1365–2621.1990.tb06068.x
- Tu , A.T. 1982 . Raman spectroscopy in biology: Principles and applications , New York : John Wiley .
- Walsh , H. , Martins , S. , O'Neill , E.E. , Kerry , J.P. , Kenny , T. and Ward , P. 2010 . The effect of sodium lactate, potassium lactate, carrageenan, whey protein concentrate, yeast extract and fungal proteinases on the cook yield and tenderness of bovine chuck muscles . Meat Science , 85 : 230 – 234 . doi: 10.1016/j.meatsci.2010.01.003
- Wasson , D.H. , Babbitt , J.K. and French , J.S. 1993 . Characterization of a heat stable protease from arrowtooth flounder . Journal of Aquatic Food Product Technology , 1 : 167 – 182 . doi: 10.1300/J030v01n03_11
- Whitmore , L. and Wallace , B.A. 2008 . Protein secondary structure analyses from circular dichroism spectroscopy: Methods and reference databases . Biopolymers , 89 : 392 – 400 . Retrieved from http://www.ncbi.nlm.nih.gov/pubmed/17896349
- Xu , X.L. , Han , M.Y. , Fei , Y. and Zhou , G.H. 2011 . Raman spectroscopic study of heat-induced gelation of pork myofibrillar proteins and its relationship with textural characteristic . Meat Science , 87 : 159 – 164 . doi: 10.1016/j.meatsci.2010.10.001
- Yamashita , M. and Konagaya , S. 1991 . Hydrolytic action of salmon cathepsins B and L to muscle structural proteins in respect of muscle softening . Nippon Suisan Gakkaishi , 57 : 1917 – 1922 . Retrieved from http://www.journalarchive.jst.go.jp/english/jnlabstract_en.php?cdjournal=suisan1932&cdvol=57&noissue=10&startpage = 1917