Abstract
Chile is considered the largest tea consumer in America, so a broad variety of tea types and brands are available in Chilean markets. Thirty-four commercial teas (white, green, red, oolong, and black) commonly found in Chile were evaluated according to their phenolic profiles, antioxidant capacity, and in vitro inhibition against α-amylase relevant for hyperglycemia control. Multivariate tools were used to evidence patterns among samples respect their biochemical parameters. Green and white tea groups showed higher total phenolic contents than red and black teas. All tea samples inhibited the 2,2-diphenyl-1-picrylhydrazyl free radical and black teas significantly inhibited the α-amylase enzyme. Major phenolics detected by liquid chromatography in green and white teas were epigallocatechingallate and epicatechingallate, whereas gallic acid was common in black, red, and oolong teas. Caffeine was found across all samples. The multivariate analysis evidenced a high variability among analyzed tea and even from brand to brand.
Chile es considerado el mayor consumidor de té en América y una amplia variedad en tipos y marcas de té existen en el mercado chileno. Un total de treinta y cuatro muestras de té (blanco, verde, rojo, oolong y negro) fueron evaluadas de acuerdo a sus perfiles de compuestos fenólicos, capacidad antioxidante e inhibición in vitro de la α-amilasa, relevante en el control de la hiperglicemia. Se utilizaron herramientas multivariantes para evidenciar patrones entre las muestras respecto a sus parámetros bioquímicos. Los grupos de té verdes y blancos presentaron los valores más altos en fenólicos totales. Todas las muestras inhibieron el radical libre 2,2-difenil-1-picrilhidracilo, en cambio sólo los tés negros inhibieron significativamente la enzima α-amilasa. Los principales compuestos fenólicos detectados por cromatografía líquida en té verde y blanco fueron la epigalocatequina-galato y epicatequina-galato, mientras que ácido gálico fue detectado en las muestras de té negro, rojo y oolong. Se detectó cafeína en todas las muestras evaluadas. El análisis multivariante demostró una alta variabilidad entre las muestras de té y aún entre las diferentes marcas procedentes de un mismo tipo de té.
Introduction
Tea is the most popular drink after water worldwide and is made from leaves of Camellia sinensis (Theaceae family), a plant native from the southeastern China and cultivated across the world mainly in tropical and subtropical areas (Sultana et al., Citation2008). Several countries have adopted tea as a traditional drink in spite of the fact that many of them are not considered tea producers. This is the case of Chile, where tea consumption is significantly high and plays an important role on Chilean culture. Recent data show that tea intake in Chile is around 319.5 cups per capita, the largest among American countries, only followed by the United States, Colombia, and Brazil (150.2, 11.3, and 9.1 cups, respectively) ( http://www.oficinascomerciales.es/icex/cda/controller/page Ofecomes/0,5310,5280449_5282927_5284940_4237341_CL,0 0.html). This situation explains the large amounts of tea importations, especially black and semi-fermented teas (17,780.8 tons in 2010) coming mainly from Argentina and Sri Lanka ( http://www.odepa.gob.cl). Although all tea types arise from the same plant (C. sinensis), differences in tea chemical composition are mainly related to their manufacture conditions, growth conditions, and area of cultivation. Based on processing and the harvested leaf development, tea types are classified into: white (harvested leave buds with white trichomes, non-fermented or semi-fermented), green teas (non-fermented), oolong teas (semi-fermented), and black teas which are fully fermented. Pu-erh or red teas are postfermented teas as opposed to fermented teas, such as black tea (Gonzalez de Mejia, Ramirez, & Puangpraphant, Citation2009; Ku, Kim, Park, Liu, & Lee, Citation2010). Phenolic compounds present in fresh tea leaves, mainly flavanol monomers and gallates, go through important chemical changes during the fermentation process. Thus, catechins are enzymatically oxidized or condensed to a wide array of complex products, such as the polymers theaflavins and thearubigins (Kim, Goodner, Park, Choi, & Talcott, Citation2011). These phenolic compounds are responsible for certain black tea characteristics, such as color and taste (Gonzalez de Mejia et al., Citation2009).
Besides the cultural aspect, the overall increase in tea consumption may also be due to the numerous studies which have shown evidence about the potential health benefits linked to tea. Tea phenolic compounds, such as catechins are thought to play a role on preventing or reducing the risk of oxidation-linked chronic diseases, such as diabetes, cardiovascular diseases, and likely cancer according to recent studies (González-Manzano et al., 2011; Tabassum, Siddiqui, & Rizvi, Citation2010). The antioxidant properties of tea phenolic compounds have also been documented and different mechanisms have been proposed (Frei & Higdon, Citation2003). Further, the inhibitory action of tea polyphenols against mammalian α-amylases has been reported (Koh, Wong, Loo, Kasapis, & Huang, Citation2010). This property could lead to the reduction of starch hydrolysis potentially reducing the postprandial hyperglycemia. Therefore, tea could be considered a healthy drink with bioactive molecules and high antioxidant capacity (Gonzalez de Mejia et al., Citation2009).
A wide variety of tea types and brands are available in Chilean markets, however, few studies exist about their chemical composition and potential health-linked properties. Such information would be helpful to consumers who are continuously looking for healthier food and beverage choices, especially in Chile where the emergence of obesity-linked chronic diseases is reaching high rates as in developed countries (Albala, Vio, Kain, & Uauy, Citation2002). Also, information based on biochemical parameters would allow the evaluation of the overall quality of tea products available for Chilean consumers. From above rationale, the objective of this study was to characterize and compare 34 tea products (water infusions from black, red, green, oolong, and white teas) commercialized in Chile respect their chemical composition (phenolic profiles) and some functional properties, such as antioxidant capacity and α-amylase inhibitory activity using in vitro models.
Materials and methods
Samples and infusion preparation
Different tea types (black, green, oolong, red, and white) of 34 commercially available bagged teas (Camellia sinensis) were purchased from a local supermarket in Valparaiso, Chile. The characteristics of analyzed samples are summarized in .
Table 1. Sample description.
Tabla 1. Descripción de muestras.
Tea infusions were prepared following the traditional home preparation: 2 g of tea were mixed with 200 mL of distilled water at 100°C and soaked for 10 min under agitation. Next, tea extracts were centrifuged for about 15 min at 6037 × g and the supernatant was recovered and stored at <5°C until analysis. For each commercial tea sample, three bags were analyzed and the extraction procedure was run in triplicate.
Chemicals
Porcine pancreatic α-amylase (EC 3.2.1.1), the 2,2-diphenyl-1-picrylhydrazyl (DPPH) radical, the Folin–Ciocalteu reagent, and the pure HPLC standards [gallic acid (GA), (−)-epigallocatechingallate (EGCG), (−)-epicatechingallate (ECG), and caffeine (CA)] were purchased from Sigma Chemical Co. (St Louis, MO, USA). Unless noted, all chemicals were HPLC grade for the HPLC analysis.
Total phenolics
The analysis was performed according to Shetty, Curtis, Levin, Wikowsky, and Ang (Citation1995). Briefly, 0.5 mL of tea extract was transferred into a test tube and mixed with 1 mL of 95% ethanol and 5 mL of distilled water. To each sample, 0.5 mL of Folin–Ciocalteu (1N) was added and mixed. After 5 min at room temperature, 1 mL of sodium carbonate (Na2CO3) solution was added to the reaction mixture and allowed to stand for 1 h in a dark place. The absorbance was read at 725 nm. The standard curve was built using various concentrations of GA in 95% ethanol, and the results were expressed as mg of gallic acid equivalents (GAE) per g of sample weight.
Antioxidant capacity by the DPPH radical inhibition assay
The antioxidant capacity was determined by the DPPH radical scavenging method by an assay modified by Kwon, Vattem, and Shetty (Citation2006). To 1 mL of 60 μM DPPH in 95% methanol, 200 μL of each tea extract was added, and the decrease in absorbance was measured after 1 min at 517 nm. A control (distilled water instead tea extract) was also included. The percentage of inhibition was calculated according to the Equation (1):
α-Amylase inhibition assay
The α-amylase inhibitory activity was determined by an assay modified from the Worthington Biochemical Corp. (1993). A total of 500 μL of each sample extract and 500 μL of 0.02 M sodium phosphate buffer (pH 6.9 with 0.006 M NaCl) containing α-amylase solution (0.5 mg/mL) were incubated at 25°C for 10 min. After preincubation, 500 μL of a 1% starch solution in 0.02 M sodium phosphate buffer (pH 6.9 with 0.006 M NaCl) was added to each tube at timed intervals. The reaction mixtures were then incubated at 25°C for 10 min. The reaction was stopped with 1.0 mL of dinitrosalicylic acid color reagent. The test tubes were then incubated in a boiling water bath for 10 min and cooled to room temperature. The reaction mixture was then diluted after adding 15 mL of distilled water, and absorbance was measured at 540 nm. The absorbance of sample blanks (buffer instead of enzyme solution) and a control (buffer in place of sample extract) were recorded as well. The final extract absorbance (A540 extract) was obtained by subtracting its corresponding sample blank reading. The α-amylase inhibitory activity was calculated according to the Equation (2), as follows:
High performance liquid chromatography analysis
Tea infusions were filtered (pore size 0.2 μm) and the HPLC analysis was performed according to Zuo, Chen, and Deng (Citation2002) with some modifications as follows. A HPLC system series 200 with UV/Vis detector (PerkinElmer Inc., Shelton, CT, USA) equipped with a binary pump, an autosampler and controlled by the TotalChrom software (Perkin Elmer Inc., Shelton, CT, USA) was used. The analytical column was a HibarLiChrospher 100 RP-18 (5 μm) (Merck, Darmstadt, Germany). The injection volume was 10 μL, the flow rate was 0.5 mL/min, and the eluates were monitored at 280 nm. The solvents used for gradient elution were: A – water/acetic acid (97:3) and B – 100% methanol. The methanol concentration was increased to 40% over the first 6 min, then to 60% for the next 16 min, then to 70% within the next 2 min, and to 100% over the next 2 min. Finally, the gradient was changed to initial conditions and maintained for 4 min (total run time 30 min). Phenolic compounds were identified using pure HPLC standards based on their retention times. Co-chromatography was used when necessary. Concentrations of GA, EGCG, ECG, and CA were determined according to their calibration curves (peak area versus concentration) obtained for each compound (R 2 ≥ 0.999). The results were expressed in mg/g of tea.
Statistical analysis
Extractions were run in triplicate and all analyses were carried out in triplicate. Results were expressed as mean ± standard deviation. Data were subjected to one-way analysis of variance (ANOVA) with the HSD Tukey test for multiple comparisons. Variance normality and homogeneity were evaluated with the Kolmogorov–Smirnov and Levene test (Montgomery, Citation2001). The significance level was α = 0.05. All the univariate statistics were performed using the Statgraphics Centurion XVI (StatPoint Inc., Rockville, MD, USA). Multivariate analysis was performed by principal component analysis (PCA) and the partial least square discriminant analysis (PLS-DA) according to Saavedra et al. (Citation2011), using SIMCA-P + 12 (Umetrics AB, Umea, Sweden). Data were centered and scaled to variance one prior to the multivariate analysis. All analyses were validated by a full cross-validation routine to minimize the predicted residual sum of squares function thus avoiding model over fitting (Cen, He, & Huang, Citation2007).
Results and discussion
Total phenolic contents
According to , a high variability was observed in the total phenolic contents among all analyzed tea types and brands (p < 0.05). Total phenolics ranged from 37 ± 1 to 112 ± 0.1 mg GAE/g. The Twinings white tea and the Akbar green tea showed the highest values among all samples (112 ± 0.1 and 111 ± 1 mg GAE/g, respectively), whereas the Twinings Lapsang Souchang black tea had the lowest level (37 ± 1 mg GAE/g). Overall, white and green tea infusions showed higher total phenolic contents than the black tea group with the exception of the Twinings Darjeeling black tea which had a different trend (104 ± 4 mg GAE/g). Hilal and Engelhardt (Citation2007) pointed out that, in general, black tea from Darjeeling may contain up to 10% more catechins than some green teas.
Table 2. α-Amylase inhibitory activities, DPPH radical scavenging capacity, and total phenolic contents in tea infusions.
Tabla 2. Actividad inhibidora de α-amilasa, capacidad de barrido de radical DPPH y contenidos totales de fenólicos en infusiones de té.
Difference in the total phenolic contents in tea samples can be influenced by several factors, such as the diversity of used raw material (sinensis or assamic ecotypes), the geographic origin, type of processing (fermented or non-fermented), and the extraction conditions for their analysis. Considering the effect of manufacture conditions, it is well known that during the fermentation process, the quantity of simple catechins, commonly found at high levels in non-fermented teas, decreases due to oxidation reactions catalyzed by natural polyphenol oxidases. These reactions lead to the increase of larger phenolic molecules, such as theaflavins and thearubigins in black teas (Ankolekar et al., Citation2011). In this way, Deetae, Parichanon, Trakunleewatthana, Chanseetis, and Lertsir (Citation2012) found that total catechin contents were significantly higher in green teas than in oolong (semi-fermented) and black teas (fermented). Also, other studies revealed that green teas have more total phenolic compounds than black teas (Moraes de Souza, Oldoni, Reginato, & Alencar, Citation2008).
Anesini, Ferraro, and Filip (Citation2008) reported that total polyphenol concentration was higher in green teas (non-fermented) than in black teas (fermented) from Argentina. Levels varied from 211 to 143 mg GAE/g in green teas, and from 176 to 84 mg GAE/g in black teas. These ranges are higher than values obtained for green and black teas in current study and may be related to the fact that above authors used methanol for phenolics extraction. The analysis of water soluble phenolic compounds in tea infusions would give more physiologically relevant results for overall potential phenolic-linked health benefits.
Some Pu-erh red teas are produced by ripening unoxidized tea leaves for several months using some yeast species prior to being pressed for further postfermentation process. Previous studies have shown that microbes in Pu-erh teas may grow at the expense of several benzene compounds (Ku et al., Citation2010). This fact could also explain why analyzed red teas showed overall low total phenolic contents (from 47 ± 6 to 53 ± 7 mg GAE/g) when compared to non-fermented teas and black teas in this study.
2,2-Diphenyl-1-picryhydrazyl (DPPH) radical scavenging capacity
The antioxidant capacities of analyzed samples are shown in . In general, white and green teas exhibited higher DPPH free radical scavenging capacity than red and black teas. However, variability among samples was lower when compared to that observed among the total phenolic results. White teas, such as the Butterfly white and Supremo white showed a 64 ± 1% and 64 ± 2% of inhibition, respectively. In case of the green tea group, the Dilmah Sencha green tea showed the highest value (64 ± 3%) and the Dilmah oolong tea (semi-fermented) significantly inhibited the DPPH radical (63 ± 2%). In contrast, the lowest DPPH inhibition was found in the Twinings Pu-erh red tea (49 ± 3%) and in the Twinings Darjeeling (50 ± 6%) and Club Seleccion (49 ± 7%) black teas. Results from current work are consistent with other studies where green teas also showed the highest free radical scavenging capacities, closely followed by black teas (Deetae et al., Citation2012).
Several factors, such as those influencing the total phenolic contents may explain variability observed in analyzed samples. As mentioned above, the oxidation degree of tea leaves determines the characteristic phenolic profiles and this likely influences the free radical scavenging-linked antioxidant properties in teas. Non-fermented teas are generally rich in flavonoids, such as catechin (C), epicatechin (EC), epigallocatechin (EGC), epicatechingallate (ECG) and epigallocatechingallate (EGCG) (Gonzalez de Mejia et al., Citation2009). Karori, Washira, Wanyoko, and Ngure (Citation2007) found that high levels of EGCG, EC, EGC, C, and ECG in green tea extracts strongly correlated to high DPPH radical inhibition. The presence of a trihydroxyphenyl B-ring in EGCG and EGC would explain the potent free radical scavenging properties of such gallocatechins (Shang et al., Citation2002).
Black teas also showed the capacity to inhibit the DPPH free radical though in a lesser extent than white teas. Polymeric theaflavins found in fermented teas contain additional hydroxyl groups which make them able to donate protons due to resonance delocalization. Further, GA is another common phenolic compound detected in black teas and may also be associated to their antioxidant activities (Karori et al., Citation2007).
𝛂-Amylase inhibitory potential
The control of postprandial hyperglycemia is critical in the early therapy for Type 2 diabetes. One therapeutic approach to decrease hyperglycemia is to delay glucose absorption through the inhibition of carbohydrate-hydrolyzing enzymes, such as α-amylases and α-glucosidases at intestinal level (Ankolekar et al., Citation2011; Chen, Qu, Fu, Dong, & Zhang, Citation2009). Green, black, and oolong teas have shown potential antidiabetic properties and therefore may play a role on lowering blood glucose and preventing hyperglycemia (Buyukbalci & El, Citation2008).
The in vitro inhibition of porcine pancreatic α-amylase significantly varied among analyzed samples (). Almost all black teas inhibited the α-amylase, and the inhibitory activities ranged from 12 ± 1% to 80 ± 2%. The Lipton Royal black tea had 80 ± 2% of inhibition, followed by Dilmah Ceylon (57 ± 4%), Teekanne Gold (56 ± 4%), and Akbar Ceylon (56 ± 3%). A different trend was shown by the Club Red Label black tea from Argentina, since no inhibitory activity against α-amylase was observed. The α-amylase inhibition was not significant in case of all red teas, the Supremo white tea, and most of the green teas. The Akbar green tea, which also showed a high total phenolic content (111 ± 1 mg GAE/g), moderately inhibited the α-amylase (30% ± 3).
Polymeric phenolic compounds, such as theaflavins and thearubigins have been associated to the anti-hyperglycemia properties observed in black teas. Previous in vitro and in vivo studies have shown that isolated theaflavins have higher α-amylase inhibitory activities than catechins due to their affinity for protein-like enzymes (Honda, Nanjo, & Hara, Citation1994). Koh et al. (Citation2010) reported that less quantity of black tea is necessary to inhibit the α-amylase and α-glucosidase enzymes than in case of green teas and also Ankolekar et al. (Citation2011) found that fully fermented teas had the highest α-amylase inhibitory activities (84.1–71.6%). Also, McDougall et al. (2005) found that fruits rich in tannin compounds exhibited high α-amylase inhibitory properties than green teas (poor in tannin compounds). This rationale would explain why overall all black teas (fermented) strongly inhibited the α-amylase among all analyzed samples in this study.
Koh et al. (Citation2010) indicated that tannin's inhibitory action would be attributed to non-covalent interactions, hydrogen bonding, and π–π interactions between the tannin aromatic ring and proteins.
HPLC analysis of phenolic compounds and CA
Specific phenolics and CA contents detected by HPLC are shown in , and shows some characteristic HPLC profiles for green, red, black, and oolong tea infusions.
Figure 1. HPLC chromatograms of tea infusions at 280 nm. (a) Oolong tea (Dilmah), (b) green tea (Akbar), (c) black tea (Twining Lapsang Souchang) and (d) pu-erh red tea (Dilmah). GA, gallic acid; EGCG, epigallocatechingallate; ECG, epicatechingallate; CA, caffeine.
Figura 1. HPLC de infusiones de té a 280 nm. (a) té oolong (Dilmah), (b) té verde (Akbar), (c) té negro (Twining Lapsang Souchang) y (d) té rojo Pu-erh (Dilmah). GA, ácido gálico; EGCG, epigalocatequina galata; ECG, epicatequina galata; CA, cafeína.
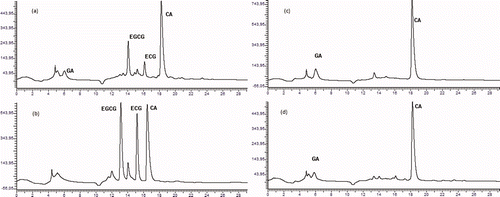
Table 3. Compounds detected at 280 nm by HPLC in tea infusions.
Tabla 3. Componentes detectados a 280 nm por HPLC (Cromatografía líquida de alta eficacia) en infusiones de té.
Significant differences were observed among phenolic profiles and were likely related to the type of tea and brand. Overall, EGCG and ECG were the major phenolic compounds detected in white and green teas. Among green teas, contents of EGCG and ECG ranged from 35 ± 5 to 56 ± 4 mg/g and from 9 ± 1 to 39 ± 3 mg/g, respectively. In case of white teas, the levels of EGCG and ECG varied from 30 to 60 mg/g and from 13 to 35 mg/g, respectively. The Twinings white tea showed the highest EGCG content (60 ± 9 mg/g), whereas the Akbar green tea was remarkable due to its high concentration of ECG (39 ± 3 mg/g) and EGCG (56 ± 4 mg/g). Similar concentrations were found by previous studies. Rusak, Komes, Likic, Horzic, and Kovac (Citation2008) and Cheong, Park, Kang, Ko, and Seo (Citation2005) reported that contents of EGCG and ECG in green tea infusions ranged from 51.5 to 88.3 mg/g and from 7.5 to 18.9 mg/g, respectively. Further, contents of 65 and 14 mg/g for EGCG and ECG, respectively, were also reported in white tea infusions (Rusak et al., Citation2008).
Major phenolic compounds found in black teas were GA and ECG, and concentrations ranged from 1.1 ± 0.1 to 5 ± 1 mg/g and from 3 ± 0.4 to 22 ± 2 mg/g, respectively. No EGCG was detected in black teas with exception of the Twinings Darjeeling black tea (42 ± 3 mg/g). Similarly, a different trend related to its total phenolic content was observed in this brand as mentioned above. Gallic acid, ECG, and EGCG were simultaneously found in the semi-fermented Dilmah oolong tea, thus showing a different phenolic profile among all tea types. Trihydroxylflavan-3-ols, such as EGCG and EGC are generally oxidized faster during the fermentation phase of black tea processing and GA is most likely produced from the degradation of EGCG (Lee et al., Citation2008). This would explain differences observed in the HPLC phenolic profiles of analyzed tea types in current work; however, the effect of the brand was also important.
The non-phenolic compound CA was found in all samples (). The Twinings Pu-erh red tea had the highest CA content (41 ± 3 mg/g), whereas the Supremo green tea showed the lowest amount (17 ± 1 mg/g). In black teas, variability among CA concentrations was lower than that observed in white, green, and red teas. Previous studies reported similar CA levels ranging from 29.2 to 56.3 mg/g in green tea infusions by using the HPLC analysis (Reto, Figueira, Filipe, & Almeida, Citation2007).
Statistical analysis
The ANOVA and following mean multiple comparisons using the Tukey HSD intervals (α = 0.05) of all data revealed a wide and high variability between each type of tea and within each group of tea (Data not shown). This confirms what was stated above regarding observed variability of analyzed parameters among tea samples. Manning and Roberts (Citation2003) and Pongsuwan et al. (Citation2007) also found similar statistical variability when analyzed different commercial green tea products.
The PCA was performed to clarify and better analyze obtained results. PCA reduces the data dimensionality and allows their visualization retaining as much as possible the variability present in the original data (Martens & Martens, Citation2001).
The first PCA model retained two principal components (t1 and t2) which explained 83.8% of the total variability of dataset. As shown in , t1 (57.6% of explained variance) arranges samples (left to right) according to their high total phenolics, EGCG, ECG, and GA concentrations; meanwhile component t2 (26.2% of explained variance) arranges samples (top to bottom) according to their high α-amylase inhibitory activities and pH values. These results reveal that analyzed tea samples showed similar patterns depending on their manufacture conditions (type of tea) and brand. Overall, black and red teas formed two properly defined groups (Groups A and B, respectively), however, a wider dispersion was observed within the black tea group (A), possibly associated to the differences in fermentation conditions, origin, or agronomic factors. The Twinings Darjeeling black tea (Group G) was the only sample with a different and a unique pattern compared to the rest of analyzed black teas and even to the other tea groups. Therefore, this specific brand of black tea likely corresponds to a new type of tea. This sample also showed the highest total phenolic content and had a different HPLC phenolic profile among black teas. Such observed trend could be difficult to explain and may be related either to its origin or to a not declared mixture of teas. However, more studies would be necessary to confirm this.
Figure 2. Score plot for PCA model.
Figura 2. Gráfica de resultados de modelo PCA (Análisis de componentes principales).
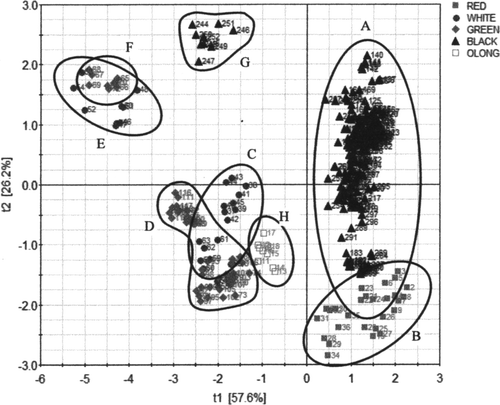
No defined groups were observed among green and white teas (C and D groups and E and F groups). Only the Akbar green (Group F) and the Twinings white teas (Group E) showed different patterns. This would be associated to their high total phenolic contents as discussed above. The Dilmah oolong tea showed a particular pattern close to the green group (Group H). In this case, the manufacture conditions (semi-fermentation) determine the oolong tea differentiation.
The supervised pattern recognition technique PLS-DA was applied to confirm relationships suggested by the PCA. In case of current data, four groups were designated: class 1 (black teas), class 2 (red teas), class 3 (green teas), and class 4 (white teas). Since the Twinings Darjeeling black tea showed a different pattern, data from this sample were excluded in the analysis.
The PLS-DA model () fitted four PLS-components which explained 96.6% of X variance, while the explained variance for Y vector reached over 65.2%. Further, the cumulative overall cross-validated Q2 was 62.5%. The PLS-DA model (t1 versus t2) confirmed the separation of black and red teas classes, while green and white classes were not well discriminated. However, the PLS-DA model properly classified the 100% of black teas, 88.89% of green teas, 69.44% of red teas, and only 26.3% of white teas (85.62% weighted average by sample size). This would be related to the overlapping pattern observed in white and green tea classes.
Conclusions
The current study revealed a high variability among analyzed tea products commercialized in Chile. Overall, high total phenolic contents and DPPH radical-linked antioxidant capacities were related to white and green teas. Black teas showed a significant in vitroα-amylase inhibitory activity indicating potential anti-hyperglycemic properties. Major catechins found in white and green teas were EGCG and ECG, whereas GA was mainly detected in fully fermented teas. Independently of the type of tea, CA was found in all tea samples. According to the PCA and PLS-DA multivariate analysis of all data, black and red teas formed two defined groups whereas green and white teas did not exhibited a clear separation. Such patterns were significantly related to the total phenolic contents, HPLC phenolic profiles, and α-amylase inhibitory activities, which in turn would indicate both influence of manufacture conditions (type of tea) and brand. This is the first time that tea products commercialized in Chile are evaluated considering their phenolic profiles, CA contents, and some health-relevant in vitro properties.
Acknowledgement
We thank the Direction of Research and Innovation from the Pontificia Universidad Catolica de Valparaiso for the grant No. 037.225/2009 to L.G.R.
References
- Albala , C. , Vio , F. , Kain , J. and Uauy , R. 2002 . Nutrition transition in Chile: Determinants and consequences . Public Health Nutrition , 5 : 123 – 128 .
- Anesini , C. , Ferraro , G.-E. and Filip , R. 2008 . Total polyphenol content and antioxidant capacity of commercially available tea (Camellia sinensis) in Argentina . Journal of Agricultural and Food Chemistry , 56 : 9225 – 9229 .
- Ankolekar , C. , Terry , T. , Johnson , K. , Johnson , D. , Barbosa , A.C.L. and Shetty , K. 2011 . Anti-hyperglycemia properties of tea (Camellia sinensis) bioactives using in vitro assay models and influence of extraction time . Journal of Medicinal Food , 14 : 1190 – 1197 .
- Buyukbalci , A. and El , S.-N. 2008 . Determination of in vitro antidiabetic effects, antioxidant activities and phenol contents of some herbal teas . Plant Foods for Human Nutrition , 63 : 27 – 33 .
- Cen , H. , He , Y. and Huang , M. 2007 . Combination and comparison of multivariate analysis for the identification of orange varieties using visible and near reflectance spectroscopy . European Food Research and Technology , 225 : 699 – 705 .
- Chen , H. , Qu , Z. , Fu , L. , Dong , P. and Zhang , X. 2009 . Physicochemical properties and antioxidant capacity of 3 polysaccharides from green tea, Oolong tea, and black tea . Journal of Food Science , 74 : 469 – 474 .
- Cheong , W. , Park , M. , Kang , G. , Ko , J. and Seo , Y. 2005 . Determination of catechins compounds in Korean green tea infusions under various extraction conditions by high performance liquid chromatography . Bulletin of the Korean Chemical Society , 26 : 747 – 754 .
- Deetae , P. , Parichanon , P. , Trakunleewatthana , P. , Chanseetis , C. and Lertsir , S. 2012 . Antioxidant and anti-glycation properties of Thai herbal teas in comparison with conventional teas . Food Chemistry , 133 : 953 – 959 .
- Frei , B. and Higdon , J.V. 2003 . Antioxidant activity of tea polyphenols in vivo: Evidence from animal studies . The Journal of Nutrition , 133
- Gonzalez de Mejia , E. , Ramirez , M.-V. and Puangpraphant , S. 2009 . Bioactive components of tea: Cancer, inflammation and behavior . Brain Behavior and Immunity , 23 : 721 – 731 .
- González-Manzano , S. , Dueñas , M. , Surco-Laos , F. , Hidalgo , M. , González-Paramas , A. , Santos-Buelga , C. and Sonia de Pascual , T. 2011 . In vitro evaluation of the antioxidant and anti-inflammatory activities of sulphated metabolites of catechins . CYTA – Journal of Food , 9 : 257 – 264 .
- Hilal , Y. and Engelhardt , U. 2007 . Characterization of white tea-comparison to green and black tea . Journal of Consumer Protection and Food Safety , 2 : 414 – 421 .
- Honda , M. , Nanjo , F. and Hara , Y. 1994 . “ Inhibition of saccharide digestive enzymes by tea polyphenols ” . In Food phytochemicals for cancer prevention II: Teas, spices and herbs , Edited by: Ho , C.-T. , Osawa , T. , Huang , M.-T. and Rosen , R.-T. 83 – 89 . Oxford , , UK : University Press .
- Karori , S. , Washira , F. , Wanyoko , J. and Ngure , R. 2007 . Antioxidant capacity of different types of tea products . African Journal of Biotechnology , 6 : 2287 – 2296 .
- Kim , Y. , Goodner , K.L. , Park , J.-D. , Choi , J. and Talcott , S.T. 2011 . Changes in antioxidant phytochemicals and volatile composition of Camellia sinensis by oxidation during tea fermentation . Food Chemistry , 129 : 1331 – 1342 .
- Koh , L. , Wong , L. , Loo , Y. , Kasapis , S. and Huang , D. 2010 . Evaluation on different teas against starch digestibility by mammalian glycosidases . Journal of Agricultural and Food Chemistry , 58 : 148 – 154 .
- Ku , K. , Kim , J. , Park , H.-J. , Liu , K.-H. and Lee , C. 2010 . Application of metabolic in the analysis of manufacturing type of Pu-erh tea and composition changes with different postfermentation year . Journal of Agricultural and Food Chemistry , 58 : 345 – 352 .
- Kwon , Y. , Vattem , D. and Shetty , K. 2006 . Evaluation of pepper for management of diabetes and hypertension . Asia Pacific Journal of Clinical Nutrition , 15 : 107 – 118 .
- Lee , S. , Dou , J. , Chen , J. , Lin , S. , Lee , R. and Tzen , T. 2008 . Massive accumulation of gallic acid and unique occurrence of myricetin, quercetin, and kaempferol in preparing old oolong tea . Journal of Agricultural and Food Chemistry , 56 : 7950 – 7956 .
- Manning , J. and Roberts , J.C. 2003 . Analysis of catechins content of commercial green tea products . Journal of Herbal Pharmacotheraphy , 3 : 19 – 32 .
- Martens , H. and Martens, M . 2001 . “ Analysis of one data table X: Principal components analysis ” . In Multivariate Analysis of Quality , Edited by: H. Martens & M. Martens . pp. 93–110 Chichester : John Wiley .
- McDougall , G. , Shapiro , F. , Dobson , P. , Smith , P. , Blake , A. and Stewart , D. 2005 . Different polyphenolic components of soft fruits inhibit alpha-amylase and alpha-glucosidase . Journal of Agricultural and Food Chemistry , 53 : 2760 – 2766 .
- Montgomery , D. 2001 . “ Experiment with a single factor: The analysis of variance ” . In D. Montgomery (Ed.) Design and analysis of experiments , pp. 10–20 New York : John Wiley .
- Moraes de Souza , R. , Oldoni , T. , Reginato , M. and Alencar , S. 2008 . Antioxidant activity and phenolic composition of herbal infusions consumed in Brazil . CYTA – Journal of Food , 6 : 41 – 47 .
- Pongsuwan , W. , Fukusaki , E. , Bamba , T. , Yonetani , T. , Yamahara , T. and Kobayashi , A. 2007 . Prediction of Japanese green tea ranking by gas chromatography/mass spectrometry-based hydrophilic metabolite fingerprinting . Journal of Agricultural and Food Chemistry , 55 : 231 – 236 .
- Reto , M. , Figueira , E. , Filipe , H. and Almeida , C. 2007 . Chemical composition of green tea (Camellia sinensis) infusions commercialized in Portugal . Plant Foods for Human Nutrition , 62 : 139 – 144 .
- Rusak , G. , Komes , D. , Likic , S. , Horzic , D. and Kovac , M. 2008 . Phenolic content and antioxidative capacity of green and white tea extracts depending on extraction conditions and the solvent used . Food Chemistry , 110 : 852 – 858 .
- Saavedra , J. , Fuentealba , C. , Yañez , L. , Bravo , M. , Quiroz , W. , Lukacsy , G. and Carot , J. 2011 . Chemometric approaches for the zoning of Pinot Noir wines from the Casablanca valley, Chile . Food Chemistry , 127 : 1842 – 1847 .
- Shang , S. , Cheng , X. , Stark , R. , Rosen , R. , Yang , C.H. and Ho , CH.-T. 2002 . Chemical studies on antioxidant mechanism of tea catechins: Analysis of radical reaction products of catechins and epicatechin with 2,2-diphenyl-1-picrilhydrazyl . Bioorganic and Medicinal Chemistry , 10 : 2233 – 2237 .
- Shetty , K. , Curtis , O. , Levin , R. , Wikowsky , R. and Ang , W. 1995 . Prevention of verification associated with in vitro shoot culture oregano by Pseudomonas spp . Journal of plant Physiology , 147 : 447 – 451 .
- Sultana , T. , Stecher , G. , Mayer , R. , Troyer , L. , Nasimullah , M. , Abel , G. … and Karl , G. 2008 . Quality assessment and quantitative analysis of flavonoids from tea samples of different origins by HPLC-DAD-ESI-MS . Journal of Agricultural and Food Chemistry , 56 : 3444 – 3453 .
- Tabassum , I. , Siddiqui , Z.N. and Rizvi , S.J. 2010 . Protective effect of Ocimum sanctum and Camellia sinensis on stress-induced oxidative damage in the central nervous system of rattusnorvegicus . Research Journal of Pharmaceutical, Biological and Chemical science , 14 : 120 – 134 .
- Worthington Biochemical Corp . 1993 . “ Alpha amylase ” . In Worthington enzyme manual , Edited by: Worthington , V. 36 – 41 . Freehold , NJ : Author .
- Zuo , Y. , Chen , H. and Deng , Y. 2002 . Simultaneous determination of catechins, caffeine and gallic acids in green, Oolong, black and pu-erh teas using HPLC with a photodiode array detector . Talanta , 57 : 307 – 316 .