Abstract
Recent research, using model systems, has shown that enzymatic hydrolysis of food proteins can make them act as direct scavengers of diverse free radicals. This study aimed at the characterization of protein hydrolysates with antioxidant properties from Vigna unguiculata L. walp and hard-to-cook Phaseolus vulgaris L. protein concentrates. The maximum values obtained for all the assays were 15.1 mM/mg of protein for Trolox-equivalent-antioxidative capacity, 98.2% for percentage of 2,2-diphenyl-1-picrylhydrazyl (DPPH) discoloration, 70.1% and 71.4% for iron and copper chelating activities, respectively. The reducing power of hydrolysates was higher than butylated hydroxytoluene (BHT) (control). The antioxidant activity of the hydrolysates may be a result of enzymatic hydrolysis generating peptides that exhibit this type of activity. The hydrolysates showed an increase of amino acids that have been associated with the biological activity of peptides with antioxidant activity, such as hydrophobic residues: Ala, Phe and Ile; hydrophilic: Lys and neutral: Ser and Gly.
Estudios recientes han demostrado que los hidrolizados enzimáticos de proteínas alimentarias pueden actuar directamente sobre radicales libres. El objetivo del presente trabajo fue caracterizar las propiedades antioxidantes de hidrolizados proteínicos de Vigna unguiculata L. walp y Phaseolus vulgaris L. endurecido. Los valores máximos obtenidos para las determinaciones efectuadas fueron, 15,1 mM/mg de proteína de Capacidad Antioxidante Equivalente de Trolox, 98,2% de decoloración del radical DPPH, 70,1% y 70,4% de capacidad quelante de cobre y hierro, respectivamente. El poder reductor de los hidrolizados fue superior al del BHT (control). La capacidad antioxidante posiblemente se relacione conlos péptidos generados durante la hidrolisis, los cuales presentan dicha actividad. La composición aminoacídica de los hidrolizados mostró un incremento de aminoácidos hidrofóbicos (Ala, Fen e Ile), hidrofílicos (Lis) y neutros (Ser y Gli), los cuales han sido asociados a la secuencia de péptidos con actividad antioxidantes.
Palabras clave:
Introduction
Recently, there has been increasing interest in naturally occurring substances with antioxidative features. They are already used as natural antioxidants in the food industry, and there is increasing evidence that these substances retain their antioxidative effect within the human body. The ability of proteins to inhibit oxidation makes them an important component of antioxidant defense food systems and biological tissues (Loganayakia, Siddhurajub, & Maniana, Citation2011). Oxidation is a vital process in the organism, and oxidative metabolism is essential for cell survival. However, this metabolism produces free radicals, which can overwhelm protective enzymes such as superoxide dismutase, catalase and peroxidase, leading to cell damage and death through oxidation of membrane lipids, cellular proteins, DNA and enzymes. This oxidative stress plays a significant role in a number of age-specific diseases such as diabetes, cancer and atherosclerosis. Recent research, using model systems, has shown that enzymatic hydrolysis of food proteins can make them act as direct scavengers of diverse free radicals (Zhang, Wang, Xu, & Gao, Citation2009). The increased ability of protein hydrolysates to decrease the reactivity of a free radical is related to an increase in the exposure of the amino acids, which leads to increased peptide-free radical reactions as well as the ability of the peptide to decrease the energy of the scavenged free radical (Elias, Kellerbya, & Deckera, Citation2008).
The main protein sources for obtaining protein hydrolysates include casein, whey and egg. These animal proteins are considered highly nutritious because they contain essential amino acid proportions that meet particular human needs. Currently, restrictions are being proposed due to considerable concerns regarding sporadic viral outbreaks in meat animals, as well as traces of chemicals or drugs used in animal feed. This situation has brought about an increased use of plant proteins, mainly legumes and by-products of oil extraction industries such as defatted soybean meal, rapeseed and sunflower (Sewald & Jakubke, Citation2002). Legumes are widely consumed in southeastern Mexico as a major dietary protein source in both human and animal diets. Cowpea (Vigna unguiculata L. walp) is a major legume crop worldwide, particularly in tropical and subtropical areas (Freitas, Teixeira, & Ferreira, Citation2004). Common bean (Phaseolus vulgaris L.) has been a dietary staple in Mexico for millennia, although inadequate postharvest handling and storage techniques (i.e. temperature > 25°C and RH > 65%) produce the hard-to-cook (HTC) defect in beans, which negatively affects their nutritional quality and makes them less acceptable and marketable (Morales-De-León, Vázquez-Mata, Torres, Gil-Zenteno, & Bressani, Citation2007). Both legumes are high in protein (21–25%) which increases up to 70% when subjected to alkaline extraction to obtain protein concentrates.
The type of enzyme used determines hydrolysate characteristics and the peptides that can be obtained. Enzymes from bacterial and fungal origin are broad specificity proteases that produce a high degree of hydrolysis values (Tovar-Pérez, Guerrero-Legarreta, Farrés-González, & Soriano-Santos, Citation2009). In contrast, specific enzymes such as Pepsin and Pancreatin (trypsin, chymotrypsin and elastase) act as endopeptidases that produce a low degree of hydrolysis. However, using sequential systems of this type of enzymes, it is possible to improve the degree of hydrolysis (Sewald & Jakubke, Citation2002). Extensive hydrolysis of V. unguiculata and HTC P. vulgaris protein concentrates with commercial and digestive enzymes could therefore produce a number of peptides with a myriad of potential applications; for example, as natural-source therapeutic elements in medical treatments and/or as an ingredient in functional foods. Taking this into account, the aim of the present study was to modify enzymatic protein concentrates of V. unguiculata and HTC P. vulgaris, evaluate the antioxidant properties of the hydrolysates and relate the biological activity to their amino acid compositions.
Materials and methods
Seeds and chemicals
Cowpea V. unguiculata L. walp seeds were obtained from the February 2007 harvest in Yucatan, Mexico. Common black beans (P. vulgaris L.), var. Jamapa, were used. Reagents were analytical grade and purchased from J.T. Baker (Phillipsburg, NJ, USA), Sigma (Sigma Chemical Co., St. Louis, MO, USA), Merck (Darmstadt, Germany) and Bio-Rad (Bio-Rad Laboratories, Inc. Hercules, CA, USA). Alcalase® (EC 3.4.21.62) 2.4L FG and Flavourzyme® (EC 3.4.21.4) 500MG (Novo Nordisk, Bagsvaerd, Denmark). Pepsin (EC 3.4.23.1) from porcine gastric mucosa (Sigma, P7000−100G) and pancreatin (mixture of trypsin EC 3.4.21.4, chymotrypsin 3.4.21.1 and elastase 3.4.21.37) from porcine pancreas (Sigma, P3292−100G).
Flour preparation
Selected seeds were processed in a disk mill (model 4-E Quaker, Mill Straub Co., Philadelphia, PA, USA) until flour was obtained. The flours were then sifted through 4.76 and 2.38 mm screens and the hulls removed with a fluidizing air bed (Generatoris, model PS-LF-160, Distrito Federal, Mexico). Further milling was carried out, first in a Mykros impact mill, with subsequent sifting through a 20-mesh screen (0.85 mm) followed by milling in a Cyclotec1093 (Tecator, Sweden) mill and sifting through a 60-mesh screen (0.24 mm).
Protein concentrates
A single extraction was performed with 10 kg of both cowpea and common bean seeds. The flours were processed using a wet fractionation method (Betancur-Ancona, Gallegos-Tintoré, & Chel-Guerrero, Citation2004). In brief, whole flour was suspended in distilled water at a 1:6 (w/v) ratio, pH was adjusted to 11 with 1M NaOH, and the dispersion was stirred for 1 h at 400 rpm with a mechanical agitator (Caframo Rz-1, Heidolph, Schwabach, Germany). This suspension was wet-milled with a Kitchen-Aid® food processor, and the fiber solids were separated from the starch and protein mix by straining through 80-and 150-mesh sieves and washing the residue five times with distilled water. The protein–starch suspension was allowed to sediment for 30 min at room temperature to recover the starch and protein fractions. The pH of the separated solubilized proteins was adjusted to the isoelectric point (4.5) with 1N HCl. The suspension was then centrifuged at 1317 × g for 12 min (Mistral 3000i, Curtin Matheson Sci.), the supernatants were discarded, and the precipitates were freeze-dried at −47°C and 13 × 10−3 mbar until use.
Enzymatic hydrolysis
Hydrolysis of the protein concentrates was performed using a totally randomized design. Treatments were Alcalase®, Flavourzyme®, the sequential enzymatic systems Alcalase®-Flavourzyme® and Pepsin-Pancreatin. The response variable was degree of hydrolysis (DH). Hydrolysis was carried out under controlled conditions (temperature, pH and stirring) in a 1000 mL reaction vessel equipped with a stirrer, thermometer and pH electrode. The hydrolysis with the Alcalase®, Flavourzyme® and Alcalase®-Flavourzyme® sequential system was performed according to Pedroche et al. (Citation2002). Protein concentrates were suspended in distilled water to produce a 10% (w/v) protein solution which was equilibrated at optimum temperature and pH for each protease before adding the respective enzyme. Protease was then added to the solution at a ratio of 0.3 AU/g for Alcalase® and 50 LAPU/g for Flavourzyme®. Hydrolysis conditions were 90 min at 50°C for each enzyme and 45 min for each enzyme for the sequential system, using pH 8.0 for Alcalase® and pH 7.0 for Flavourzyme®. The pH was kept constant by adding 1.0 M NaOH during hydrolysis. The hydrolysis with the sequential pepsin-pancreatin system was done for 90 min: predigestion with pepsin for 45 min followed by incubation with pancreatin for 45 min. Hydrolysis parameters were substrate concentration 4%; enzyme/substrate ratio 1:10; pH 2.0 for pepsin; pH 7.5 for pancreatin; and 37°C hydrolysis temperature (Megías et al., Citation2004; Yang, Marczak, Yokoo, Usui, & Yoshikawa, Citation2003). In both treatments, the reaction was stopped by heating to 80°C for 20 min, followed by centrifuging at 1317 x g for 12 min (Mistral 3000i, Curtin Matheson Sci.) to remove the insoluble portion.
Degree of hydrolysis
Degree of hydrolysis was calculated by determining free amino groups with o-phthaldialdehyde: DH = (H/H tot)100; where H tot is the total number of peptide bonds per protein equivalent, and H is the number of hydrolyzed bonds (Nielsen, Petersen, & Dammann, Citation2001).
Antioxidant activity
ABTS•+ decolorization assay
The ABTS•+ radical cation was produced by reacting 2, 2′-azino-bis (3-ethylbenzothiazoline-6-sulphonic acid) (ABTS) with potassium persulfate (Pukalskas et al., Citation2002). To prepare the stock solution, ABTS was dissolved at a 2 mM concentration in 50 mL phosphate-buffered saline (PBS) prepared from 4.0908 g NaCl, 0.1347 g KH2PO4, 0.7098 g Na2HPO4, and 0.0749 g KCl dissolved in 500 mL ultrapure water. If pH was lower than 7.4, it was adjusted with NaOH. A 70 mM K2S4O8 solution in ultrapure water was prepared. The ABTS radical cation was produced by reacting 10 mL ABTS stock solution with 40 μL K2S4O8 solution and allowing the mixture to stand in darkness at room temperature for 16–17 h before use. The radical was stable in this form for more than 2 days when stored in darkness at room temperature. Antioxidant compound content in the hydrolysates was analyzed by diluting the ABTS•+ solution with PBS to an absorbance of 0.800 ± 0.030 AU at 734 nm. After adding 990 μL diluted ABTS•+ solution (A 734 nm = 0.800 ± 0.030) to 10 μL of 6-hydroxy-2,5,7,8-tetramethylchroman-2-carboxylic acid (TROLOX) standard in PBS, absorbance was read at room temperature exactly 6 min after initial mixing. All determinations were carried out in triplicate. The Trolox equivalent antioxidant coefficient was calculated using 20 mg of each hydrolysate. The percentage decrease in absorbance at 734 nm was calculated and plotted as a function of the antioxidant concentration of Trolox for the standard reference data. To calculate the Trolox equivalent antioxidant coefficient (TEAC), the slope of the absorbance inhibition percentage vs. antioxidant concentration plot was divided by the slope of the Trolox plot. This produces the TEAC at a specific point in time (Pukalskas et al. Citation2002).
DPPH free radical-scavenging assay
The scavenging effect on α,α-diphenyl- β-picrylhydrazyl (DPPH) free radical was measured as described by Shimada, Fujikawa, Yahara, and Nakamura (Citation1992). With some modifications, a hydrolysate solution (1.5 mL) with 20 mg was added to 1.5 mL of 0.1 mM DPPH in 95% ethanol. The mixture was shaken and left for 30 min at room temperature. Absorbance was measured at 517 nm. The lower absorbance (A517) represents higher DPPH scavenging activity, which is expressed as [(blank A517-sample A517)/blank A517] × 100%.
Chelating of metal ions Cu2+ and Fe2+
Cu2+-chelating activity was determined using the pyrocatechol violet reagent according to Saiga, Tanabe, and Nishimura (Citation2003). Briefly, 1.0 mL of sodium acetate buffer (100 mM, pH 4.9), 100 μL of Cu (II) standard solution (1.0 μg/μL), and 100 μL of sample (containing 200 μg of protein) were mixed in a test tube. The mixture was allowed to react for 5 min at room temperature and 25 μL of a pyrocatechol violet solution (4.0 mM) was then added. Absorbance was determined at 632 nm. Copper chelating activity was calculated as follows: Chelating activity (%) = (1 − sample absorbance at 632 nm/control absorbance at 632 nm) × 100. Fe2+-chelating activity was determined by measuring the formation of the Fe2+-ferrozine complex according to Carter (Citation1971). Briefly, 1.0 mL of sodium acetate buffer (100 mM, pH 4.9), 100 μL of Fe(II) standard solution (1.0 μg/μL), and 100 μL of sample (containing 200 μg of protein) were mixed in a test tube. The mixture was allowed to react for 5 min at room temperature and 50 μL of a ferrozine solution (40 mM) was then added. Absorbance was determined at 562 nm. Iron chelating activity was calculated as follows: Chelating activity (%) = (1 − sample absorbance at 562 nm/ control absorbance at 562 nm) × 100.
Reducing power
This method is based on the reduction of potassium ferricyanide (Fe3+) in the presence of an antioxidant to (Fe2+) forming the blue complex K[FeII(CN6)], which absorbs at 700 nm (Yen & Chen, Citation1995). First, 200 μL of sample (containing 1 mg of protein), 500 μL of phosphate buffer (0.2 M, pH 6.6), and 500 μL of potassium ferricyanide (1%) were mixed in a test tube. The test tube was then incubated at 50°C for 20 min. Subsequently, 500 mL of trichloroacetic acid (10%) were added, and the tube was centrifuged at 3000 × g for 10 min. An aliquot of 500 μL of the supernatant was dissolved in an equal amount of distilled water, immediately 500 μL of ferric chloride (0.1%) were added. Absorbance was determined at 700 nm. Samples were tested in a range of concentrations from 200 to 1000 mg/mL. Butylated hydroxytoluene was used as control in the same range of concentrations.
Amino acid composition
Amino acid composition was determined for the protein concentrates and hydrolysates by high performance liquid chromatography (Alaiz, Navarro, Giron, & Vioque, Citation1992). Samples (4mg of protein) were treated with 4 mL of HCl 6.0 N, placedin hydrolysis tubes and gassed with nitrogen at 110°C for 24 h. They were then dried in a rotavapor (Büchi, Rotavapor R-215, Flawil, Switzerland) and suspended in sodium borate buffer (1.0 M, pH 9.0). Derivatization was performed at 50°C using diethyl ethoxymethylenemalonate. Amino acids were separated using high-performance liquid chromatography (HPLC) with a reversed-phase column (300 × 3.9 mm, Nova Pack C18, 4 mm; Waters), and a binary gradient system with sodium acetate containing 25 mM (A) 0.02 g/L sodium azide at pH 6.0, and (B) acetonitrile as solvent. The flow-rate was 0.9 mL/min, and the elution gradient was: time 0.0–3.0 min, linear gradient A:B (91:9) to A–B (86:14); time 3.0–13.0 min, elution with A–B (86–14); time 13.0–30.0 min, linear gradient A–B (86:14) to A–B(69:31); time 30.0–35.0 min, elution with A–B (69:31).
Statistical analysis
All results were analyzed using descriptive statistics with a central tendency and dispersion measures. One-way ANOVAs were run to evaluate protein concentrate hydrolysis data and in vitro antioxidant activities. The least significant difference (LSD) multiple range test was used to determine differences among treatments. All analyses were done according to Montgomery (Citation2004) and processed with the Statgraphics Plus version 5.1 software.
Results and discussion
Protein concentrate hydrolysis
The crude protein content of the concentrates was 65.57 and 73.03% for Vigna unguiculata and HTC Phaseolus vulgaris, respectively. These contents are similar to those reported for other legumes, such as Canavalia ensiformis (73.75%) (Chel-Guerrero, Pérez-Flores, Betancur-Ancona, & Dávila-Ortíz, Citation2002) and Phaseolus lunatus (71.13%) (Betancur-Ancona et al., Citation2004), which are used for their functional properties and biological activity as alternative sources for the development of nutraceutical products. Therefore, the seed proteins of the legumes V. unguiculata and HTC P. vulgaris can be an alternative for obtaining hydrolysates with functional and biological activity. Hydrolysis with Alcalase® Flavourzyme® and sequential systems Alcalase®-Flavourzyme® (AF) and Pepsin-Pancreatin (PP) produced extensive hydrolysis V. unguiculata and HTC P. vulgaris protein concentrates (). Enzymatic hydrolysis is widely used to release bioactive peptides.
Table 1. Degree of hydrolysis obtained with Alcalase®, Flavourzyme®, Alcalase®-Flavourzyme® (AF), and Pepsin-Pancreatin (PP).
Tabla 1. Grados de hidrólisis obtenidos con Alcalase®, Flavourzyme®, Alcalase®-Flavourzyme® y Pepsina-Pancreatina.
In the present study, hydrolysis of V. unguiculata protein concentrate with Flavourzyme® resulted in a maximum DH of 58.8%, and hydrolysis of HTC P. vulgaris with Alcalase® resulted in a minimum DH of 13.5%, at 90 min hydrolysis time and stable pH conditions. Degree of hydrolysis values were higher than those reported for hydrolysis of legumes such as V. unguiculata (23.61%) with Alcalase® for 60 min (Espinosa, Chel, & Betancur, Citation2006), chickpea (27%) with Flavourzyme® for 180 min (Clemente, Vioque, Sánchez-Vioque, Pedroche, & Millán, Citation1999) and soy with pepsin for 60 min (11%) and 180 min (17%) (Qi, Hettiarachychy, & Kalapathy, Citation1997). In general, enzymes from microbial and fungal origins such as Alcalase® and Flavourzyme® have a higher catalytic activity compared to enzymes from gastric and intestinal origins like pepsin and pancreatin. This is consistent with the results observed in this study, since the hydrolysates with Alcalase® and Flavourzyme® exhibited the greatest degrees of hydrolysis for V. unguiculata, and the Alcalase®-Flavourzyme® system for P. vulgaris (). HTC P. vulgaris had lower degrees of hydrolysis than V. unguiculata, probably due to the changes that occur in proteins of P. vulgaris during hardening. Storage at high temperature and relative humidity increases endogenous protease activity and consequent hydrolysis of P. vulgaris storage protein. This would apparently increase overall protein digestibility, but it also causes increased interactions with digestibility-limiting agents such as high molecular mass tannins, which are 1.64 times greater in HTC P. vulgaris (Morales-De-León et al. Citation2007).
Antioxidant activity
To evaluate the antioxidant activity, the hydrolysates with the greatest degrees of hydrolysis for each legume were selected. These were V. unguiculata obtained with Alcalase® (VA), Flavourzyme® (VF) and Pepsin-Pancreatin (VPP), and HTC P. vulgaris obtained with Alcalase®-Flavourzyme® (PAF) and Pepsin-Pancreatin (PPP).
ABTS•+ decolorization assay
The ABTS assay is very useful for the assessment of total antioxidant capacity. It is based on the inhibition by antioxidants of the absorbance of the radical cation 2,2-azinobis-(3-ethylbenzothiazoline-6-sulphonate), which has a characteristic long-wavelength absorption spectrum showing main absorption maxima at 415 nm, and secondary absorption maxima at 660, 734, and 820 nm. The use of Trolox as a standard allows the assay to be called TEAC. Antioxidant activity of the protein concentrates and hydrolysates was quantified and calculated as mM/mg sample. Protein concentrates had no bioactivity while hydrolysates presented antioxidant activity (). The enzymatic hydrolysis released antioxidant peptides that were inactive when included within the intact protein. Other authors have reported that accessibility to the oxidant–antioxidant test systems is greater for small peptides and amino acids than for large peptides and proteins (Moosmann & Behl, Citation2002). The development of antioxidant activity following enzymatic hydrolysis of V. unguiculata and HTC P. vulgaris protein concentrates is in line with previous reports about antioxidant activity of protein hydrolysates (Gómez-Ruiz, López-Expósito, Pihlanto, Ramos, & Recio, Citation2008).
Figure 1. Trolox equivalent antioxidant capacity (TEAC)in hydrolysates of Vigna unguiculata obtained with Alcalase® (VA), Flavourzyme® (VF) and Pepsin-Pancreatin (VPP), and hard-to-cook Phaseolus vulgaris obtained with Alcalase®-Flavourzyme® (PAF) and Pepsin-Pancreatin (PPP). Data are presented as means (n = 3). Different letters indicate significant differences (p < 0.05).
Figura 1. Capacidad antioxidanteequivalente de Trolox (TEAC) de los hidrolizados de Vigna unguiculata obtenidos con Alcalase® (VA), Flavourzyme® (VF) y Pepsina-Pancreatina (VPP), y de Phaseolus vulgaris endurecido obtenidos con Alcalase®-Flavourzyme® (PAF) and Pepsina-Pancreatina (PPP). Los datos corresponden al promedio de 3 determinaciones. Letras diferentes indican diferencia significativa (p < 0,05).
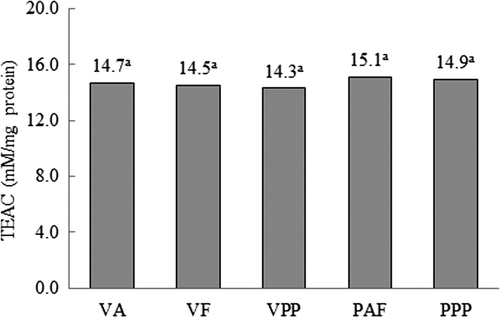
Trolox equivalent antioxidant coefficient values did not differ between hydrolysates () indicating that the ability of protein hydrolysates to act as antioxidants cannot be attributed to the degree of hydrolysis, but rather may be given by the amino acid composition of hydrolysates, which in turn depends on the proteolytic activity of the enzyme system used. The TEAC values of the hydrolysates ranged from 14.3 to 15.1 mM/mg of sample, these values were higher than those reported by Moosmann and Behl (Citation2002) for hydrolysates of ovine casein (1.63 mM/mg of sample) obtained with trypsin and chymotrypsin.
DPPH free radical-scavenging assay
The DPPH (2,2-diphenyl-1-picrylhydrazyl) free radical is a stable free radical, which has been widely accepted as a tool for estimating free radical-scavenging activities of antioxidants. Proton radical scavenging has been reported to be an important mechanism for antioxidation. The assay for assessment of proton-radical-scavenging activity with DPPH is simple and quite reproducible, with this compound having been reported to be stoichiometrically decolorized by antioxidants. The reduction in the concentration of the DPPH is allowed to monitor the decrease in its absorbance at a characteristic wavelength (517 nm) when it encountered proton-radical scavengers. The color change from purple to yellow after the reduction can be quantified by its decrease in absorbance. Radical scavenging activity increases with increasing percentage of free radical inhibition. The percentages of DPPH remaining in the presence of the hydrolysates at a concentration of 100 mg/mL are shown in .
Figure 2. Percentage of DPPH discoloration after addition of the hydrolysates of Vigna unguiculata obtained with Alcalase® (VA), Flavourzyme® (VF) and Pepsin-Pancreatin (VPP), and hard-to-cook Phaseolus vulgaris obtained with Alcalase®-Flavourzyme® (PAF) and Pepsin-Pancreatin (PPP). Data are presented as means (n = 3). Different letters indicate significant differences (p < 0.05).
Figura 2. Porcentaje de decoloración de DPPH después de la adición de los hidrolizados de Vigna unguiculata obtenidos con Alcalase® (VA), Flavourzyme® (VF) y Pepsina-Pancreatina (VPP), y Phaseolus vulgaris endurecido obtenidos con Alcalase®-Flavourzyme® (PAF) and Pepsina-Pancreatina (PPP). Los datos corresponden al promedio de 3 determinaciones. Letras diferentes indican diferencia significativa (p < 0,05).
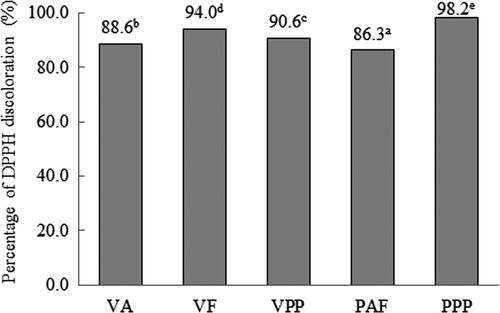
The scavenging activity of the hydrolysates was higher than the values of 63% and 76% reported by Park, Murakami, Mori, and Matsumura (Citation2005) for soy peptides (25 mg/mL). The hydrolysates differed significantly in radical-scavenging activity (p < 0.05). For the hydrolysates of V. unguiculata, the most active sample was the hydrolysate obtained with Flavourzyme® (98.2%); for the hydrolysates of HTC P. vulgaris, the most active sample was the hydrolysate obtained with the sequential system Alcalase®-Flavourzyme® (94.0%). Legume protein-derived peptides have been reported to exhibit radical-scavenging activity. Chen, Muramoto, Yamauchi, and Nokihara (Citation1996) reported that a total of 22 peptides deriving from proteolytic digest of a soybean protein, β -conglycinin, exhibited some DPPH radical-scavenging. In this study, the DPPH radical-scavenging activity of V. unguiculata and HTC P. vulgaris hydrolysates may be attributable to the peptides produced by enzymatic proteolysis.
Chelating of metal ions Fe2+ and Cu2+
Iron and copper are abundant transition-metal ions in humans, both may work as catalysts for the generation of reactive oxygen species in pathological conditions. The reduced forms of both potentiate oxygen toxicity by converting, via the Fenton reaction, the less reactive hydrogen peroxide to the more reactive oxygen species, the hydroxyl radical. Therefore, it is not surprising that iron and copper overloads have been shown to be associated with carcinogenesis and cardiovascular disease. It has been assumed that the strict regulation of iron and copper assimilation prevents an excess of free intracellular content of both metals, which leads to oxidative stress (Chen et al. Citation1996). The iron ion chelating activities in hydrolysates of V. unguiculata obtained with Alcalase® (VA), Flavourzyme® (VF) and Pepsin-Pancreatin (VPP), and HTC P. vulgaris obtained with Alcalase®-Flavourzyme® (PAF) and Pepsin-Pancreatin (PPP) are shown in . For V. unguiculata hydrolysates, VA had the highest chelating activity of Fe2+, followed by VF, and VPP. For HTC P. vulgaris hydrolysates, PAF had the highest chelating activity of Fe2+, followed by VPP. The chelating activity of Fe2 + differed significantly between hydrolysates (p < 0.05).
Figure 3. Iron chelating activity in hydrolysates of Vigna unguiculata obtained with Alcalase® (VA), Flavourzyme® (VF) and Pepsin-Pancreatin (VPP), and hard-to-cook Phaseolus vulgaris obtained with Alcalase®-Flavourzyme® (PAF) and Pepsin-Pancreatin (PPP). Data are presented as means (n = 3). Different letters indicate significant differences (p<0.05).
Figura 3. Actividad quelante de hierro de los hidrolizados de Vigna unguiculata obtenidos con Alcalase® (VA), Flavourzyme® (VF) y Pepsina-Pancreatina (VPP), y de Phaseolus vulgaris endurecido obtenido con Alcalase®-Flavourzyme® (PAF) and Pepsina-Pancreatina (PPP). Los datos corresponden al promedio de 3 determinaciones. Letras diferentes indican diferencia significativa (p < 0,05).
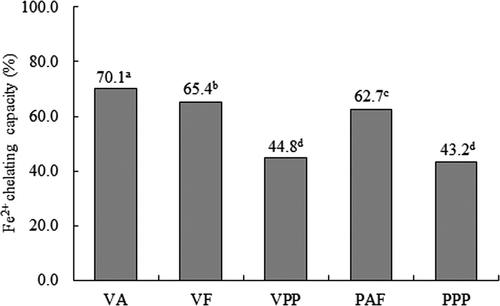
The copper ion chelating activities in hydrolysates of V. unguiculata obtained with Alcalase® (VA), Flavourzyme® (VF) and Pepsin-Pancreatin (VPP), and HTC P. Vulgaris obtained with Alcalase®-Flavourzyme® (PAF) and Pepsin-Pancreatin (PPP) are shown in . For V. unguiculata hydrolysates, VA had the highest chelating activity of Cu2+, followed by VF, and VPP. For HTC P. vulgaris hydrolysates, PAF had the highest chelating activity of Fe2+, followed by VPP. The chelating activity of Cu2+differed significantly between hydrolysates (p < 0.05).
Figure 4. Copper chelating activity in hydrolysates of Vigna unguiculata obtained with Alcalase® (VA), Flavourzyme® (VF) and Pepsin-Pancreatin (VPP), and hard-to-cook Phaseolus vulgaris obtained with Alcalase®-Flavourzyme® (PAF) and Pepsin-Pancreatin (PPP). Data are presented as means (n = 3). Different letters indicate significant differences (p<0.05).
Figura 4. Actividad quelante de cobre de los hidrolizados de Vigna unguiculata obtenidos con Alcalase® (VA), Flavourzyme® (VF) and Pepsina-Pancreatina (VPP), y de Phaseolus vulgaris endurecido obtenido con Alcalase®-Flavourzyme® (PAF) and Pepsina-Pancreatina (PPP). Los datos corresponden al promedio de 3 determinaciones. Letras diferentes indican diferencia significativa (p < 0,05).
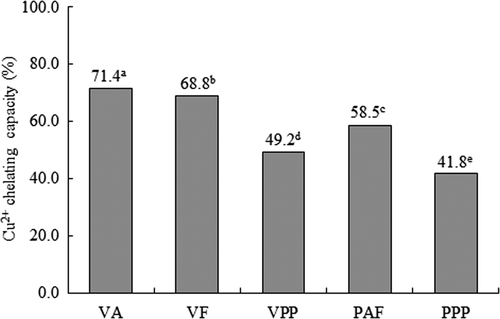
Protein hydrolysis led to an enhanced Fe2+ and Cu2+ chelating ability. According to Liu, Chen, and Lin, (Citation2005), proteolysis may increase the concentration of carboxylic acid groups and an increased exposure of metal-chelating amino acid residues such as histidine. Hydrolysates obtained with Alcalase®, Flavourzyme® and sequential Alcalase®-Flavourzyme® of both legumes exhibit a greater chelating capacity, these enzyme systems probably generated more exposure of metal-chelating amino acid residues than the gastrointestinal enzymes Pepsin and Pancreatin.
Reducing power
The reducing capacity of a compound may serve as a significant indicator of its potential antioxidant activity. According to Chang, Kim, and Hwang (Citation2002), the antioxidant activities of antioxidants have been attributed to various mechanisms, such as prevention of chain initiation, binding of transition metal ion catalysts, decomposition of peroxides, prevention of continued proton abstraction, and radical scavenging. Different studies have indicated that antioxidative activity and reducing power are related (Chang et al. Citation2002). The reducing power of the V. unguiculata and HTC P. vulgaris hydrolysates are shown in and . Reduced butylated hydroxytoluene (BHT) was used as the positive control. The different hydrolysates from the two legumes exhibited a dose dependent reducing power activity within the concentration range 0, 200, 400, 600, 800, and 1000 μg/mL.
Figure 5. Determination of reducing power of the hydrolysates of Vigna unguiculata obtained with Alcalase® (VA), Flavourzyme® (VF) and Pepsin-Pancreatin (VPP), BHT was the positive control. Data are presented as means (n = 3).
Figura 5. Determinación del poder reductor de los hidrolizados de Vigna unguiculataobtenidos con Alcalase® (VA), Flavourzyme® (VF) and Pepsina-Pancreatina (VPP), se empleó BHT como control positivo. Los datos corresponden al promedio de 3 determinaciones.
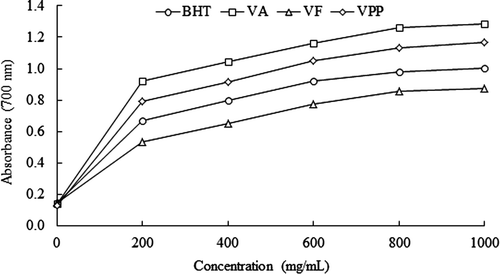
Figure 6. Determination of reducing power of the hydrolysates of Phaseolus vulgaris obtained with Alcalase®-Flavourzyme® (PAF) and Pepsin-Pancreatin (PPP), BHT was the positive control. Data are presented as means (n = 3).
Figura 6. Determinación del poder reductor de los hidrolizados de Phaseolus vulgaris obtenidos con Alcalase®-Flavourzyme® (PAF) and Pepsina-Pancreatina (PPP), se empleó BHT como control positivo. Los datos corresponden al promedio de 3 determinaciones.
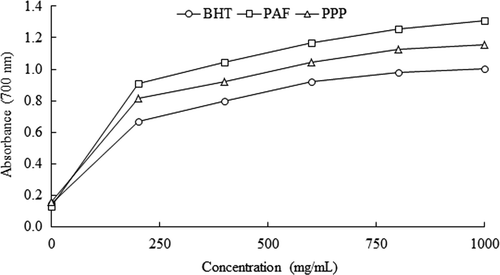
For V. unguiculata hydrolysates, VA had the highest reducing power, followed by VPP and VF. For HTC P. vulgaris hydrolysates, PAF had the highest reducing power, followed by VPP. The reducing power did not differ significantly between VA, VF, PAF, and PPP (p < 0.05). At the same concentration, the reducing power of VA, VPP, PAF, and PPP was higher than the positive control (BHT). In contrast, the reducing power of VF was lower than the BHT. The reducing power is a method to determine the mechanism of radical reduction of the hydrolysates. According to Xue et al. (Citation2012), the reducing power of a compound may be attributed to its hydrogen-donating ability.
Amino acid composition
The amino acid compositions of the hydrolysates are presented in . The five hydrolysates presented differences in the composition. They were rich in aspartic acid, glutamic acid, serine, arginine, valine, isoleucine, leucine, phenylalanine, and lysine. In addition, the total hydrophobic amino acids’ (THAA) content in hydrolysates was higher than its counterpart in protein concentrates. For V. unguiculata hydrolysates, VA had the highest THAA content, followed by VF and VPP. For HTC P. vulgaris hydrolysates, PAF had the highest THAA content, followed by VPP.
Table 2. Amino acid composition of Vigna unguiculata and hard-to-cook Phaseolus vulgaris protein concentrates and hydrolysates.
Tabla 2. Composición de aminoácidos de los concentrados e hidrolizados proteínicos de Vigna unguiculata y Phaseolus vulgaris endurecido.
For protein hydrolysates, the increase in hydrophobicity will increase their solubility in lipid which in turn results in the enhancement of the antioxidant activity. In fact, peptides derived from many protein sources with increased hydrophobicity have been reported to be related to antioxidative properties (Li, Jiang, Zhang, Mu, & Liu, Citation2008). Some amino acids, including tyrosine, histidine, proline, alanine, and leucine, have been reported to contribute to scavenging free radicals (Elias et al. Citation2008). For V. unguiculata hydrolysates VA these residues account for 22.3%, followed by VF (21.6%), and VPP (21.2%). For HTC P. vulgaris hydrolysates PAF these residues account for 16.7%, followed by VPP (15.7%). According to Elias et al (Citation2008), the presence of certain amino acids in the sequence of peptides with antioxidant activity may be related to the hydrolytic activity of the enzymes used. It should be pointed out that neither the structure–activity relationship nor the antioxidant mechanism of peptides is fully understood. In general, the scavenging of free radicals was attributed to the donation of hydrogen/electron. Some amino acids were widely believed to be direct radical scavengers due to their special groups in side chains, such as His (imidazole group) (Gómez-Ruiz et al. Citation2008) Trp (indolic group), and Tyr (phenolic group) (Guo, Kouzuma, & Yonekura, Citation2009). These groups could act as hydrogen donors. Additionally, Met is prone to oxidation of the Met sulfoxide and Cys donates the sulfur hydrogen (Hernández-Ledesma, Dávalos, Bartolomé, & Amigo, Citation2005). Aromatic amino acids (Tyr and Phe) are generally considered to be effective radical scavengers because they can donate protons easily to electron deficient radicals while at the same time maintaining their stability via resonance structures (Hernández-Ledesma et al., Citation2005).
Conclusions
For the first time, antioxidant activities have been recorded in V. unguiculata and HTC P. vulgaris protein hydrolysates produced with commercial enzymes Alcalase® and Flavourzyme®, gastrointestinal enzymes pepsin and pancreatin, and sequential enzymatic systems, Alcalase®-Flavourzyme® and pepsin-pancreatin. Hydrolysates from both commercial and gastrointestinal enzymes exhibit antioxidant capacity. Hydrolysates obtained with commercial enzymes exhibit a greater antioxidant capacity; these enzyme systems probably generated more exposure of amino acid residues, which can act as effective radical scavengers, in comparison with the gastrointestinal enzymes. Although further research is needed on purification steps and peptide sequences, hydrolysates from both legumes are promising natural antioxidants and potential ingredients in functional food systems.
Acknowledgments
This publication forms part of the projects “Actividad biológica de fracciones péptidicas derivadas de la hidrólisis enzimática de proteínas de frijoles lima (Phaseolus lunatus) y caupí (Vigna unguiculata)” funded by CONACYT-México (project 153012), and “Purificación y caracterización de péptidos bioactivos obtenidos por hidrólisis enzimática de proteínas de Fuentes vegetales subutilizadas (Red Temática: Bioactividad de péptidos e hidrolizados),” funded by Programa de Mejoramiento al Profesorado-PROMEP-SEP. The authors thank the Industria Mexicana Coca-Cola for the financial support.
References
- Alaiz , M. , Navarro , J.L. , Giron , J. and Vioque , E. 1992 . Amino acid analysis by high-performance liquid chromatography after derivatization with diethylethoxymethylenemalonate . Journal of Chromatography , 591 : 181 – 186 .
- Betancur-Ancona , D. , Gallegos-Tintoré , S. and Chel-Guerrero , L. 2004 . Wet fractionation of Phaseolus lunatus seeds: partial characterization of starch and protein . Journal of Science of Food and Agriculture , 84 : 1193 – 1201 .
- Carter , P. 1971 . Spectrophotometric determination of serum iron at the submicrogram level with a new reagent (ferrozine) . Analytical Biochemistry , 40 : 450 – 458 .
- Chang , W.C. , Kim , S.C. and Hwang , S.S. 2002 . Antioxidant activity and free radical scavenging capacity between Korean medicinal plants and flavonoids by assay guided comparison . Plant Science , 163 : 1161 – 1168 .
- Chel-Guerrero , L. , Pérez-Flores , V. , Betancur-Ancona , D. and Dávila-Ortíz , G. 2002 . Functional properties of flours and protein isolates from Phaseolus lunatus and Canavalia ensiformis Seeds . Journal of Agricultural and Food Chemistry , 50 : 584 – 591 .
- Chen , H.M. , Muramoto , K. , Yamauchi , F. and Nokihara , K. 1996 . Antioxidant activity of designed peptides based on the antioxidative peptide isolated from digests of a soybean protein . Journal of Agricultural and Food Chemistry , 44 : 2619 – 2623 .
- Clemente , A. , Vioque , J. , Sánchez-Vioque , R. , Pedroche , J. and Millán , F. 1999 . Production of extensive chickpea (Cicer arietinum L.) protein hydrolysates with reduced antigenic activity . Journal of Agricultural and Food Chemistry , 47 : 3776 – 3781 .
- Elias , R.J. , Kellerbya , S.S. and Deckera , E.A. 2008 . Antioxidant activity of proteins and peptides . Critical Reviews in Food Science and Nutrition , 48 : 430 – 441 .
- Espinosa , L. , Chel , L. and Betancur , D. 2006 . Hidrólisis enzimática de proteínas como una alternativa para la obtención de alimentos funcionales . Revista de la Facultad de Ingeniería Química, Universidad Autónoma de Yucatán , 42 : 16 – 24 .
- Freitas , R.L. , Teixeira , A.R. and Ferreira , R.B. 2004 . Characterization of the proteins from Vigna unguiculata seeds . Journal of Agricultural and Food Chemistry , 52 : 1682 – 1687 .
- Gómez-Ruiz , J.A. , López-Expósito , I. , Pihlanto , A. , Ramos , M. and Recio , I. 2008 . Antioxidant activity of ovine casein hydrolysates: identification of active peptides by HPLC-MS/MS . Milchwissenschaft , 60 : 41 – 44 .
- Guo , H. , Kouzuma , Y. and Yonekura , M. 2009 . Structures and properties of antioxidative peptides derived from royal jelly protein . Food Chemistry , 113 : 238 – 245 .
- Hernández-Ledesma , B. , Dávalos , A. , Bartolomé , B. and Amigo , L. 2005 . Preparation of antioxidant enzymatic hydrolysates from α-lactalbumin and β-lactoglobulin. Identification of active peptides by HPLC-MS/MS . Journal of Agricultural and Food Chemistry , 53 : 588 – 593 .
- Li , Y. , Jiang , B. , Zhang , T. , Mu , W. and Liu , J. 2008 . Antioxidant and free radical-scavenging activities of chickpea protein hydrolysate (CPH) . Food Chemistry , 106 : 444 – 450 .
- Liu , J.R. , Chen , M.J. and Lin , C.W. 2005 . Antimutagenic and antioxidant properties of milk-kefir and soymilk-kefir . Journal of Agricultural and Food Chemistry , 53 : 2467 – 2474 .
- Loganayakia , N. , Siddhurajub , P. and Maniana , S. 2011 . A comparative study on in vitro antioxidant activity of the legumes Acacia auriculiformis and Acacia ferruginea with a conventional legume . Cajanuscajan. CyTA-Journal of Foods , 9 : 8 – 16 .
- Megías , C. , Yust , M.M. , Pedroche , J. , Lquari , H. , Girón-Calle , J. , Alaiz , M. … and Vioque , J. 2004 . Purification of an ACE inhibitory peptide after hydrolysis of sunflower (Helianthus annuus L.) protein isolates . Journal of Agricultural and Food Chemistry , 52 : 1928 – 1932 .
- Montgomery , D. 2004 . Diseño y análisis de experimentos , México, D.F , , México : Limusa-Wiley .
- Moosmann , B. and Behl , C. 2002 . Secretory peptide hormones are biochemical antioxidants: Structure-activity relationship . Molecular Pharmacology , 61 : 260 – 268 .
- Morales-De-León , J.C. , Vázquez-Mata , N. , Torres , N. , Gil-Zenteno , L. and Bressani , R. 2007 . Preparation and characterization of protein isolate from fresh and hardened beans . (Phaseolus vulgaris L.). Journal of Food Science , 72 : 96 – 102 .
- Nielsen , P. , Petersen , D. and Dammann , C. 2001 . Improved method for determining food protein degree of hydrolysis . Journal of Food Science , 66 : 642 – 643 .
- Park , E.Y. , Murakami , H. , Mori , T. and Matsumura , Y. 2005 . Effects of protein and peptide addition on lipid oxidation in powder model system . Journal of Agricultural and Food Chemistry , 53 : 137 – 144 .
- Pedroche , J. , Yust , M.M. , Girón-Calle , J. , Alaiz , M. , Millán , F. and Vioque , J. 2002 . Utilization of chickpea protein isolates for the production of peptides with angiotensina I converting enzyme inhibitory activity . Journal of Science of Food and Agriculture , 82 : 960 – 965 .
- Pukalskas , A. , Van Beek , T. , Venskutonis , R. , Linssen , J. , Van Veldhuizen , A. and Groot , A. 2002 . Identification of radical scavengers in sweet grass (Hierochloeodorata) . Journal of Agricultural and Food Chemistry , 50 : 2914 – 2919 .
- Qi , M. , Hettiarachychy , N. and Kalapathy , U. 1997 . Solubility and emulsifying properties of soy protein isolates modified by pancreatin . Journal of Food Science , 62 : 1110 – 1115 .
- Saiga , A. , Tanabe , S. and Nishimura , T. 2003 . Antioxidant activity of peptides obtained from porcine myofibrillar proteins by protease treatment . Journal of Agricultural and Food Chemistry , 51 : 3661 – 3667 .
- Sewald , N. and Jakubke , H.D. 2002 . Peptides: Chemistry and biology , Weinheim , , Germany : Wiley-VCH .
- Shimada , K. , Fujikawa , K. , Yahara , K. and Nakamura , T. 1992 . Antioxidative properties of xanthan on the antioxidation of soybean oil in cyclodextrin emulsion . Journal of Agricultural and Food Chemistry , 40 : 945 – 948 .
- Tovar-Pérez , E. , Guerrero-Legarreta , I. , Farrés-González , A. and Soriano-Santos , J. 2009 . Angiotensin I-converting enzyme-inhibitory peptide fractions from albumin 1 and globulin as obtained of amaranth grain . Food Chemistry , 116 : 437 – 444 .
- Xue , T. , Qiuping , W. , Guowei , L. , Jiao , W. , Kaijian , Y. and Yonghui , S. 2012 . Structural and antioxidant modification of wheat peptides modified by the heat and lipid peroxidation product malondialdehyde . Journal of Food Science , 71 : H16 – H22 .
- Yang , Y. , Marczak , D.E. , Yokoo , M. , Usui , H. and Yoshikawa , M. 2003 . Isolation and antihypertensive effect of angiotensin I-converting enzyme (ACE) inhibitory peptides from spinach rubisco . Journal of Agricultural and Food Chemistry , 51 : 4897 – 4902 .
- Yen , G.C. and Chen , H.Y. 1995 . Antioxidant activity of various tea extracts in relation to their antimutagenicity . Journal of Agricultural and Food Chemistry , 43 : 27 – 32 .
- Zhang , S.B. , Wang , Z. , Xu , S.Y. and Gao , X.F. 2009 . Purification and characterization of a radical scavenging peptide from rapeseed protein hydrolysates . Journal of the American Oil Chemists’ Society , 86 : 959 – 966 .