Abstract
Nixtamalized blue corn expanded extrudates were elaborated in a single screw extruder using feed moisture (FM) of 14, 15, 16, 16.5, 18.5 and 20.5%; ground corn with different particle size indexes (PSI, 83.97 and 94.15), and at a final extruder temperature of 130 and 140°C. The physicochemical properties of extrudates evaluated were moisture content, total anthocyanin and cyanidin 3-glucoside (C3G) content, color, density and expansion index. Extrudates processed at 16 and 16.5% of FM showed higher total anthocyanin and C3G contents. The extrudates processed with 16% FM, at 130°C and PSI of 83.97, showed the highest total anthocyanin content (211.1 mg · kg−1). Cyanidin 3-glucoside showed an increase (11.3%) in extrudates when compared with raw corn. The expansion index was higher for those made with FMs of 14, 15, 16 and 16.5%. The total anthocyanin and C3G contents were not correlated with any other parameter.
Se elaboraron extrudidos expandidos nixtamalizados de maíz azul con un extrusor de tornillo simple bajo las siguientes condiciones: humedad de alimentación: 14, 15, 16, 16,5, 18,5 y 20,5%, maíz con diferente índice de tamaño de partícula (ITP, 83,97 y 94,14); y temperatura final del extrusor: 130 y 140°C. Se evaluaron las siguientes propiedades fisicoquímicas en los extrudidos: humedad, contenido de antocianinas totales y contenido de cianidina 3-glucósido, color, densidad e índice de expansión. Los extrudidos procesados con humedad de alimentación 16 y 16,5%, mostraron los valores más elevados de antocianinas totales y cianidina 3-glucósido. El tratamiento con humedad de alimentación 16%, 130°C y un ITP de 83,97, presentó los valores más altos de antocianinas totales (211,1 mg · kg−1). La cianidina 3-glucósido mostró un incremento del 11,3% en los extrudidos comparados con el maíz crudo. El índice de expansión fue mayor a humedades de alimentación 14, 15, 16 y 16,5%. Las antocianinas totales y la cianidina 3-glucósido no correlacionaron con ninguna otra evaluación.
Palabras clave:
Introduction
Extrusion technology has been widely used by food industries to obtain a variety of products (Harper & Clark, 1979). Extruded products are commonly obtained from starchy materials, especially cereal grains such as corn, sorghum and rice. This process also has been used as an alternative method for the production of nixtamalized products (snacks and corn flours). Recently, many studies have been developed to produce healthier products to counteract chronic-degenerative diseases (Alavi, Karkle, Adhikari, & Keller, 2011; Gupta, Sing, & Dutt, 2008; Milan, Valdez, Gutierrez, Cardenas, & Mora, 2007; Nayak, Hai, Berrios, Tang, & Derito, 2011; Rocha, Gallegos, Gonzalez, Bello, & Delgado, 2008). To obtain food products with improved nutritional properties and health benefits, whole grains have been used. Phenolic compounds, mainly of the group of flavonoids denominated anthocyanins, are present in high concentrations in pigmented corns (De Pascual, Santos, & Rivas, 2002). These molecules are contained in the pericarp, aleurone layer and between the endosperm of the corn grains (Betran, Bockholt, & Rooney, 2001). These compounds give in the pigmented corns, products with additional nutritional value. The anthocyanins contained in blue corn are cyanidin 3-glucoside (C3G), cyanidin 3-galactoside, pelargonidin 3-glucoside and malvidin 3-glucoside (González et al., 2008).
In Mexico and Central America, corn is mostly processed by nixtamalization, a thermic-alkaline treatment that includes the addition of calcium hydroxide (lime) to improve the taste of the products (table tortillas, tortilla chips, tamales and snack foods). However, this process requires large amounts of water and long steeping times. Nixtamalized snacks are widely consumed in many Latin-American countries and in the United States. Nixtamalized extruded snacks and similar products are made from alkaline-treated flours (Platt et al., 2010). As an alternative method to obtain products with similar characteristics, extrusion has been used as a high-temperature short-time (HTST) process, offering many advantages such as the reduction of food nutrient loss, inactivation of certain undesirable compounds and starch gelatinization. There are many factors and variables affecting the extrusion process, and the most important include feed moisture (FM), barrel temperature and screw speed (Yu, Ramaswamy, & Boye, 2012). The process of milling corn grains results in materials with different particle sizes. However, to obtain expanded extrudate snacks, a fine particle size is desirable. Particle size has significant implications on the physicochemical, textural and functional properties of the final products obtained by extrusion (Martinez-Flores, Martinez, Figueroa, & Gonzalez, 1998).
Several studies have been reported using pigmented corn in the extrusion process. Del Pozo, Serna, Brenes, and Talcott (2007), made tortillas and chips produced with American and Mexican blue corn by traditional nixtamalization. They observed higher anthocyanin loss during the process in American blue corn (63% in tortillas and 81% in chips). This loss correlated with lower antioxidant activity. Mora et al. (2010) compared tortillas made by traditional nixtamalization and extrusion cooking. They found that blue corn tortillas lost more than 55% of their anthocyanins when they were processed by both technologies. The results indicated that the extrusion process retained higher levels of phytochemicals such as ferulic acid and antioxidants in all tortilla treatments. Zazueta, Martinez, Jacobo, Ordorica, and Paredes (2001), evaluated the effect of calcium hydroxide on some characteristics (expansion index and penetration force) of the extruded products obtained from blue corn. They concluded that it is possible to obtain good-quality extrudates fortified with calcium. Zazueta, Martinez, Jacobo, Ordorica, and Paredes (2002), suggested the utilization of calcium hydroxide in concentrations lower than 0.1% to obtain blue corn extruded products with a better expansion index and appearance.
There are many studies in which blue corn was used to obtain diverse products. However, none of them used whole blue corn to directly produce nixtamalized expanded extrudates with a higher anthocyanin content to take advantage of the natural and beneficial components of this cereal. The objective of this study was to establish the extrusion factors that have effects on the physicochemical, physical and textural properties of nixtamalized blue corn expanded extrudates to obtain a healthy product containing whole blue corn grains.
Materials and methods
Materials
Mexican dented blue corn (harvested in 2010) was obtained in a local market in Toluca, Mexico. The corn samples were placed in a clean apparatus (Clipper BLOUNT/Ferrell-Ross, Model M2BC; Bluffon Inc., USA) to remove foreign material. The clean kernels were stored in sealed polyethylene bags at 5°C in a refrigeration chamber until use. Commercial lime (Calhidra de Sonora, SA de CV, Hermosillo, Son. Mexico) and distilled water were used.
Characterization of blue corn
The moisture content (44–01), protein content (46–13), ash content (08–01) and crude fat content (30–25) were determined by AACC (2000) approved methods. The starch content was determined with the total starch kit assay procedure (Method 76–13 AACC, 2000, Megazyme International, Ireland). Three replicates were used for each determination.
Total anthocyanin content
This analysis was performed according to Abdel-Aal and Hucl (1999). Three grams of ground blue maize was weighed in a 50 mL centrifuge tube, and 24 mL of acidified ethanol (ethanol with HCl 1N, 85:15 v/v) was added. The solution was adjusted to pH 1 with 4N HCl and shaken for 15 min. A second adjustment was made to the same pH, and the solution was shaken again. After that, the tubes were centrifuged (Thermo Scientific, Model Heraes Biofuge Primo R., Germany) at 27 200g for 15 min; the supernatant was poured into a 50 mL centrifuge tube, and the volume was adjusted to 50 mL with acidified ethanol. The absorbance was measured at 535 nm (against blank) in a UV-visible spectrophotometer (Varian Australia PT LTD, Cary 50 CONC, Australia). All measurements were made in triplicate.
Analysis of cyanidin–3-glucoside
Samples of 400 mg of ground material were mixed with 1 mL methanol-HCl (10%, v/v) in a micro test tube and shaken at 4°C for 30 min. Test tubes were kept at 4°C for 12 h in the absence of light and shaken again for 1 h. Samples were centrifuged (Eppendorf AG, Model 5415D, Hamburg, Germany) at 2300g for 5 min, and the supernatant was centrifuged at 13,400g for 10 min. Extracts were kept in amber glass tubes until analysis.
The analysis of C3G was conducted on an HPLC with a UV detector and DAD (Varian ProStar, Model 410, Palo Alto, CA). Separation of C3G was accomplished on a reverse phase column (Waters, Model Spherisorb ODS–2, 5 μm, 250 × 46 mm, Ireland). The mobile phases were B, formic acid (5%) in water and C, methanol. The gradient used was as follows: 0–6 min, 15% B; 6–20 min, 15–22% B; and 20–35 min, 22–30% B. The flow rate was 1.5 mL/min; column temperature, 35°C; detection wavelength, 525 nm; injection, 20 μL; and postrun time, 5 min. All solvents were of HPLC grade. The analysis was performed in duplicate.
Physical evaluations of the blue corn kernel
Blue corn color parameters (L, a, b) were measured using a Hunter Lab Miniscan XE Plus (Hunter Association Laboratories, USA). To obtain a thousand-kernel weight (TKW), 1000 healthy grains were weighed on a scale (OHAUS triple beam series 700/800, Poland), and the result was recorded in grams. The hectolitric weight (HW) was obtained by method 44–11, AACC (2000). The length, width and thickness of the kernels were determined with a digital caliper (Mitutoyo Corp., Model CD-6 CS, Japan).
Blue corn grinding
Whole blue corn (7 kg) was ground 1 and 3 times on a six-blade mill with rubbed shell (Pulvex SA de CV, Model 200, serial 1030401, Mexico, DF) with a 0.8-mm mesh, to obtain ground corn (GC) with different particle sizes. Three kilograms of GC obtained in the first grinding was separated, placed into sealed polyethylene bags to avoid moisture loss and denominated ground corn from 1 milling (GCM1). The rest of the GC (3 kg) was reground two more times, placed into sealed polyethylene bags and denominated ground corn from three millings (GCM3). The GCM1 and GCM3 moisture contents (44-01 AACC 2000) were measured, and they were stored at 5°C until use.
The particle size distribution (PSD) in GCM1 and GCM3 samples was determined using a rotator-tapping sieve shaker (Rotachoc, Chopin Instruments, serial 46902, Villenueve-La-Garenne, France) by passing 50 g of the ground material through the following US sieves: No. 20 (841 μm), 40 (420 μm), 60 (250 μm), 80 (177 μm), 100 (149 μm) and pan (<149 μm) (Platt et al., 2010).
Particle size index (PSI) was measured according to Bedolla and Rooney (1984):
where SFN = sieve factor number and PSD (%) = particle size distribution.
Each factor number depends on the number of the US sieve (0.2, sieve No. 20; 0.4, sieve No. 40; 0.6, sieve No. 60; 0.8, sieve No. 80; 1.0, sieve No. 100 and pan).
Production of nixtamalized blue corn expanded extrudates
Samples of 250 g of ground corn (GCM1 and GCM3) were mixed for 10 min in a laboratory mixer (Kitchen Aid, Model MK45SSWH, St. Joseph, Michigan, USA) with calcium hydroxide (0.3% w/w), and distilled water was added to reach the following moisture contents: 14, 15, 16, 16.5, 18.5 and 20.5%. All treatments were refrigerated 12 h at 4°C to obtain better hydration of starch particles, and they were kept in darkness to avoid anthocyanin degradation.
Extrusion process
A single-screw extruder (Brabender Instruments, Model E 19/25 D, OHG Duisburg, Duisburg, Germany) was used. Its barrel was separated into four independently heated/cooled zones (1300 W each). The temperatures were 60, 80, 110°C in the first, second and third zones, respectively while the fourth zone was set at 130°C and 140°C. Screw number 3 (nominal compression ratio 3:1 and diameter 19 mm) was used. The screw speed was constant at 120 rpm, the extruder feed hopper was 50 rpm, and the die opening diameter was 4 mm. The operation conditions were selected from factorial combinations of the following processing factors: two final temperature zones (130 and 140°C), FM contents (14, 15, 16, 16.5, 18.5 and 20.5%) and two PSIs (83.97, CGM1 and 94.15, GCM3). The obtained extrudates, were cooled at room temperature for 1 h, then dried at 60°C in a tunnel dryer and stored at 5°C in darkness in sealed polyethylene bags.
Extrudates evaluation
AACC (2000) Approved Method 44-15 was applied to determine moisture content. Total anthocyanin content (TAC) and C3G were evaluated following the procedure applied to the blue corn kernels.
Physical properties
The color of the extrudates was measured using a Hunter Lab Miniscan XE Plus (Hunter Association Laboratories, USA), and the evaluated parameters were L, a, b. The apparent density was calculated as the ratio of weight (g) to volume (cm3) of individual extrudates. To obtain the volume, individual extrudates were weighed and measured (length and radius). It was assumed that the samples were geometrically similar to a cylinder, and the volume of each extrudate was computed. The expansion index was measured using a Digital Caliper (Mitutoyo Corp., Model CD–6 CS, Japan) and was calculated as the ratio of the mean diameter to the diameter of the extruder die. Ten replicates of each determination were performed.
Textural properties
Extrudates texture was evaluated as maximum stress, by a Texture Analyzer (TA-XT Plus, Texture Technology Corporation, Stable Micro Systems, Surrey, England). Individual extrudates were placed onto a plate and compressed using a Warner–Bratzel probe (Type P/75, Stable Micro Systems, Surrey, England). The test speed was set at 2.5 mm/s covering a compressed distance of 20 mm, and the results were expressed in terms of maximum shear stress (kPa). Ten replicates of each measurement were performed.
Experimental design and statistical analysis
A factorial design experiment of 6×2 × 2 was used. The factors were FM with six levels (14, 15, 16, 16.5, 18.5 and 20.5%); PSI with two levels (83.97 and 94.15); and temperature in the fourth zone of the extruder, with two levels (130 and 140°C). For all of the data gathered, an analysis of variance (ANOVA) was performed with a 95% level of confidence. Simple linear correlation coefficients (r) were obtained among the different evaluations carried in the extrudates. Statistical analyses were performed with the Statistical Analytical Systems software package Version 9.1.3 (SAS, Institute Cary, Inc., USA).
Results
Chemical and physical characteristics of blue corn
The chemical composition of blue maize is presented in . Starch content is an important indicator for the end use of corn; the amylose/amylopectin ratio influences the thermal and physical (especially expansion) properties and therefore the processing behavior of starch-based products (Taghvaei, Motiee, Shakeri, & Abbasian, 2010). The total starch content (TSC) of the blue corn was 68.39%, which is within the range presented for dented corn used for nixtamalization. A similar TSC (73.4%) was reported by Agama et al. (2004) for blue corn kernels. The protein content of blue corn was also similar to the dented (Serna, 1996) but higher than the values found by Agama, Salinas, Pacheco, and Bello (2011), for blue corn from two races (Tabloncillo and Chalqueño). The TAC in blue maize was similar to the values reported by Lopez, Parkin, and Garcia (2011) (631 mg · kg−1), and higher than the values presented by Dykes & Rooney (2007) (225 mg · kg−1) for this type of corn.
Table 1. Chemical composition of blue corn kernels.
Tabla 1. Composición química del maíz azul.
The physical characteristics of blue corn kernels () were similar to those of maize suitable for nixtamalization (Salinas, Martinez, Soto, Ortega, & Arellano, 2003). The color parameters were characteristic of this type of corn. The TKW in blue maize was higher than the value found by Milan et al. (2007) for QPM maize (260.2 g).
Table 2. Physical properties of blue corn kernels.
Tabla 2. Propiedades físicas del maíz azul.
Particle size distribution and particle size index of milled corn
The corn products obtained by extrusion mostly depend on the grinding characteristics of corn kernels, such as particle size, starch damage, type of mill and extruder conditions (Martinez-Flores et al., 1998). shows the PSD of ground corn from one milling and three millings. The moisture contents of GCM1 and GCM3 were 11.7 and 9.8%, respectively (data not shown). The reduction in the moisture content was most likely caused by the friction inside the mill and the slight increase in temperature between the millings (7.7°C). The accumulative fraction on sieves No. 20, 40, 60 and 80 (coarse particles) was 39.4% for GCM1 and 20.9% for GCM3. When these percentages were compared with the ground corn retained in sieves No. 100 and pan (fine particles), the results showed a marked difference between millings, with an accumulative fraction of 60.5% for GCM1 and 79.0% for GCM3.
Figure 1. Particle size distribution of blue maize milling fractions. Bars indicate standard deviation.
Figura 1. Distribución de tamaño de particula de las fracciones de maíz molidas. Las barras indican la desviación estándar.
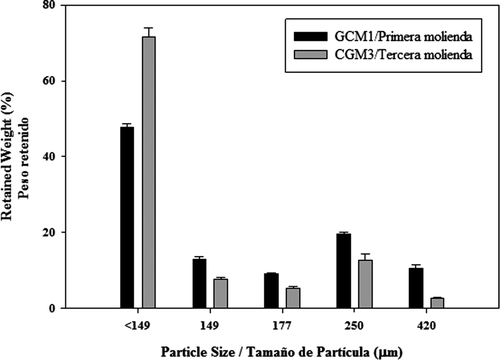
The PSI of GCM1 was 84.9 and that of GCM3 was 94.1. Higher PSI values denote higher proportions of fine particles. According to Mathew, Hoseney, and Faubion (1999), fine-ground corn produced extrudates with higher expansion values than medium and coarse-ground corn, and also depends of the extrusion variables.
Physicochemical properties of the extrudates
The results of the ANOVA showed () that the extrudates' moisture content, TAC and C3G content were highly affected (P < 0.01) by FM, temperature (T) and PSI and the interactions FM × T, FM × PSI and T × PSI.
Table 3. Mean valuesa of moisture content, total anthocyanins and C3G content of extrudates at different feed moistures, temperatures and PSIs.
Tabla 3. Valores mediosa de contenido de humedad, contenido total de antocianinas y cianidina 3-glucósido en extrudidos a diferentes humedades de alimentación, temperaturas e índices de tamaño de partícula (PSI).
shows the mean values of the moisture content in extrudates at different FMs, temperatures and PSIs. These values were lower as the FM decreased and were significantly different (P < 0.05) in almost all extrudates, except in those elaborated with FM of 14 and 15%. The extrusion temperature showed significant differences (P < 0.05) in moisture content of the extrudates processed at 130°C compared to those that were processed at 140°C.
The GCM1 extrudates, had significantly (P < 0.05) higher moisture content than the treatments obtained with GCM3. This effect is likely due to damage to the starch granules during the milling process, causing their water absorption index to increase. However, the GCM3 extrudates processed at 140°C did not show this increase in their moisture content, which can be explained by the processing at higher temperature and faster evaporation of water. Higher levels of FM resulted in more compact and darker materials, due to the change in the macromolecular structure of the starchy components of the extrudates that reduced the melt elasticity, thus decreasing the expansion, forming more compact materials (Ding, Ainsworth, Plunkett, Tucker, & Marson, 2006).
Changes in total anthocyanin content
Anthocyanins are stable compounds at acidic pH, and when lime (calcium hydroxide) is added, the anthocyanin content decreases (Salinas et al., 2003). shows the mean values of TAC in extrudates at different FMs, temperatures and PSIs. Extrudates retained between 17.8 and 38.5% of the TAC when compared with raw blue maize. The TAC was affected by FM, showing lower (P < 0.05) values in extrudates processed at higher FM (20.5, 18.5 and 16.5%). As it was expected, treatments processed at 130°C retained more anthocyanins than those made at 140°C. According to Brennan, Brennan, Derbyshire, and Tiwari (2011), high screw speed and temperature are the extrusion factors affecting polyphenols and the anthocyanin content of extruded foods by decarboxylation.
The milling process also affected the TAC in extrudates. Treatments elaborated with GCM1 presented the highest values of TAC. During corn milling, there was a slight increase in temperature and also an abrasive effect, which may have had an effect on the degradation of phenolic compounds. Extrudates processed at FM of 16%, 130°C and GCM1 showed the highest TAC. At FMs of 16 and 16.5%, the TAC was higher in all extrudates. At this moisture content, anthocyanins were most likely released from the cell matrix of the aleurone layer, forming monomers and dimers (Brennan et al., 2011). Extrudates elaborated under the following conditions: FM of 16%, temperature 130°C and GCM1 had the best TAC.
Even when the extrusion process affected the TAC, the results of this work demonstrated that partial anthocyanin losses still occurred. Mora et al. (2010) evaluated the effect of the extrusion process on the phenolic content (including anthocyanins) of tortillas produced from pigmented corn processed by conventional nixtamalization and extrusion cooking. Their results strongly indicated that the lime-cooking extrusion process retained higher levels of phytochemicals such as anthocyanins, especially in blue corn products. Similar findings were reported (Del Pozo et al., 2007) with the conclusion that blue corn extruded products showed an acceptable retention of anthocyanins when compared with those elaborated by the traditional nixtamalization process. This information was confirmed in this study.
Changes in cyanidin 3-glucoside content
Cyanidin–3-glucoside (and its malonated derivatives) is a type of anthocyanin contained in purple and blue corn (Yang et al., 2009). shows the mean values of C3G content in extrudates at different FMs, temperatures and PSIs. The C3G content showed statistically significant differences (P < 0.05) for all FM contents. This indicates that this parameter was highly affected by FM. Anthocyanins are water-soluble compounds, and it is likely that the presence of a certain amount of water leads to structural changes or the degradation of this compound. C3G was higher at FMs of 16 and 16.5%.
C3G content was higher (P < 0.05) for extrudates elaborated at 140°C than those made at 130°C. This effect was positive for the purpose of this study, and C3G was most likely not affected by the processing temperature, which indicates that C3G is a more stable anthocyanin (Brennan et al., 2011).
Extrudates produced with GCM1 showed higher C3G contents than those made with GCM3. In this study, whole blue corn was used, which means that higher amounts of pericarp and germ were present in ground corn. These anatomical parts of the corn kernels, most likely provided a positive effect by retaining and increasing the TAC and C3G levels. The lowest C3G value in extrudates () was 7.6 mg · kg−1, which corresponded to 4.5% of the C3G in raw corn kernels ().
However, the highest amount of C3G (186 mg · kg−1) was found in extrudates made at an FM of 16.5%, 140°C and GCM1, an increase of 11.3% when compared with the amount in raw blue corn kernels. This indicates that during extrusion process, some chemical and structural changes occurred to generate or released C3G. The correlation analysis showed that TAC did not correlate with C3G.
According to Salinas et al. (2003), the observed increase in C3G was most likely due to the degradation of acyl anthocyanins such as cyanidin 3-(3′′, 6′′ -malonylglucoside) and 3-(3″, 6″-dimalonylglucoside). Acyl anthocyanins have an ester bond between the glucoside and the malonyl group that is unstable at high temperatures and pH; breaking this bond leads to degradation into C3G.
Extrudates color
Changes in color during the extrusion process have important implications in the acceptance of extruded products by consumers. In this study it was expected that extrudates color were similar of that obtained in the corn kernels. The results of the ANOVA showed that the color parameters of the extrudates were significantly affected (P < 0.01) by FM and temperature as well as the interactions FM × T, FM × PSI and T × PSI. shows the mean values of Hunter Lab Color Values (L, a, b) in extrudates at different FMs, temperatures and PSIs.
Table 4. Mean valuesa of Hunter Lab color (L, a, b)b of extrudates at different feed moistures, temperatures and PSIs.
Tabla 4. Valores mediosa de parámetros de color Hunter Lab (L, a, b)b en extrudidos a diferentes humedades de alimentación, temperaturas e índices de tamaño de partícula (PSI).
The color parameter L indicates luminosity on a scale of 100 (white) to black (0) and was different for all FM contents. Blue corn kernels presented an L value of 37.8 (), which indicated a slight decrease in this parameter in the extrudates. As FM increased, the L value decreased and was different in all treatments. Extrudates processed at FM of 14%, 140°C and GCM1 presented the highest L value (37.4). However, the extrusion temperature had a significant effect on L values, extrudates processed at 140°C presented a higher L values (P < 0.05) than those processed at 130°C. As L values increased, FM was lower. These results were similar to those reported by Iio and Berghofer (1999), who demonstrated that L and a values of yellow corn extrudates were dependent on FM and barrel temperatures.
Positive and negative a values indicate red and green shades, respectively. The blue corn kernel a value was 0.4 (), which increased in all extrudates. This parameter significantly differed (P < 0.05) among all FM contents (). As a value increased, FM was lower in all extrudates. Durge, Sarkar, and Sinhgal (2011), evaluated changes in the color measurements of rice extrudates supplemented with anthocyanin extracts. They suggested that decreases in a values were a manifestation of pigment degradation by thermal effects during extrusion process, and a higher screw speed led to an increase in this value. Extrudates elaborated with FM of 15%, 140°C and GCM1 showed the highest a value (5.6). Higher positive values for a indicated more red shades in this treatment. The extrusion temperature had a significant effect (P < 0.05) on a, as the extrudates processed at 140°C showed the highest values.
Positive and negative b values indicated yellow and blue shades, respectively. The b value of blue corn kernels was 4.6 (). All extrudates showed a decrease in this parameter (). By visual examination, extrudates with higher FMs appeared more blue/purple and darker. The extrudates elaborated with FMs of 18.5%, 130°C and GCM1 showed the lowest b value (−0.5). At higher FMs, the b values became negative, an indication of changes in blueness. Extrusion temperature and PSI had a significant effect (P < 0.05) on b values, showing the highest values in extrudates processed at 140°C. Del Pozo et al. (2007) reported, from subjective color evaluations, a severe color loss when blue corn was nixtamalized at temperatures higher than 90°C. An alkaline pH causes the hydration of flavyl cation and increase the production of a colorless carbinol, reaching equilibrium with another open-form compound called chalcone (Fossen, Cabrita, & Anderson, 1998).
In general, changes in all color parameters were detected in the extrudates, some caused by processing and others caused by the effect of additives (calcium hydroxide). These effects were previously described by Martinez et al. (1998), who evaluated the effects of different calcium hydroxide concentrations on the L, a and b values of corn meal extrudates. They showed that this compound reacts with different pigments contained in the samples, interfering with browning reactions (Maillard reactions). They also reported that higher concentrations of calcium hydroxide affected the a values of extruded corn meals.
It was performed a correlation analysis among color parameters (L, a, b), CAT and C3G, and no significant correlations were founded.
Density
This evaluation is commonly used to define the physical characteristics and quality of extruded samples. shows the mean values of density, expansion index and maximum stress in extrudates at different FMs, temperatures and PSIs. The results of the ANOVA showed that FM, PSI and the interaction FM × Temperature had a very significant effect (P < 0.01) while the interaction of FM × PSI had a significant effect (P < 0.05) on this parameter.
Table 5. Mean valuesa of density, expansion index and maximum stress (σ) of extrudates at different feed moistures, temperatures and PSIs.
Tabla 5. Valores mediosa de densidad, índice de expansión y esfuerzo máximo (σ) en extrudidos a diferentes humedades de alimentación, temperaturas e índices de tamaño de partícula (PSI).
The density of the extrudates was different (P < 0.05) with higher FM. As the FM decreased, the density also decreased for all treatments. Lazou and Krokida (2010) measured the apparent density of corn-lentil extruded snacks obtained by extrusion and reported that density increased with higher moisture content and decreased with temperature. During extrusion, an increase in the temperature of processing decreases the melt viscosity and could improve bubble growth inside the material. However, in our extrudates, a decrease in density caused by higher temperatures was not found. Similar values of this parameter were obtained for the extrudates processed at 130 and 140°C. Treatments made with GCM3 presented lower values of density (P < 0.05) when compared with those elaborated with GCM1. This behavior could be an effect of milling process, the extrudates made with GCM3 showed lower values of density and moisture content. The main components of the grain: starch, proteins, non-starch polysaccharides (such as arabinoxilans and other gums contained in the pericarp) could change the absorption/desorption pattern due to the effects of milling and processing.
Expansion index
This is an important parameter of extrudates' quality in terms of the functionality and acceptability of the final product. The results of ANOVA showed that FM, PSI and their interaction temperature × PSI have a highly significant effect (P < 0.01) on this physical parameter. The extrusion temperature and the interaction FM × Temperature had a significant effect (P < 0.05).
The expansion index was reduced (P < 0.05) as FM increased (). The extrusion temperature also affected the expansion index; the extrudates processed at 130°C showed higher values (P < 0.05) than those processed at 140°C. These results were different than those reported previously by Maga and Liu (1993) who reported a better expansion index in blue corn extrudates processed at 140°C.
The addition of lime in the production of nixtamalized extruded snacks is necessary to develop a particular taste. The effects of calcium hydroxide and screw speed on the physicochemical characteristics of blue corn extrudates have been studied by Zazueta et al. (2001, 2002). These authors demonstrated that higher concentrations (0.2%) of this additive significantly reduced the expansion index. During the nixtamalization process, high pH levels are generated by the addition of this compound, causing the ionization of the hydroxyl radicals of the starch molecule. However, in lower concentrations (0.1%), the decrease in the expansion index is caused by a starch–calcium complex in which calcium ions are bound by charges on the starch hydroxyl groups and form a tight molecule. In this study, the addition of calcium hydroxide in higher concentrations (0.3%) on the extrudates, most likely decreases the expansion index.
The PSI of ground corn and twin-screw extrusion was studied by Garber, Hsieh, and Huff (1997). A larger PSI in ground corn does not absorb water like smaller PSI ground corn. This fact changes the viscosity and temperature inside the barrel, affecting the expansion index. This explains the higher values (P < 0.05) in the extrudates elaborated with GCM3. Treatments made with FM of 14%, 140°C and GCM3 presented the highest expansion index (2.64). Tempering time is also a factor affecting expansion. Zhang and Hoseney (1998) showed that a tempering time of ∼30 minutes for ground corn with coarse and fine particle size before extrusion produced a greater volumetric expansion index in corn meal extrudates. They also suggested that more uniform moisture absorption leads to better expansion.
To produce extruded snacks, corn flours are degermed to generate more expansion. To improve the nutritional profile of the extrudates, whole maize was milled (including germ). Lipids contained in blue maize kernel (4.3%) could act as lubricants, decreasing the friction, temperature of processing and cooking degree inside the barrel on the samples (Battacharya & Hanna, 1988), which could also affect the expansion index in the extrudates.
Textural properties (maximum shear stress)
To measure the texture of the extrudates, the maximum shear stress was calculated (). The ANOVA showed that FM, PSI and the interaction FM × Temperature have a very significant effect (P < 0.01) on this measure. In extruded snack foods, stress is mainly affected by FM, expansion, absorption, solubility and other factors such as the components of the raw materials (Anton & Luciano, 2007). Extrudates elaborated with FMs of 16, 15 or 14% showed similar maximum stress values. In this study, the differences in maximum stress might be dependent on liquid water diffusion and the particle size of GC. Zhang and Hoseney (1998) demonstrated that liquid water diffusion is different for large and small particles. Large particles have a porous structure, and their water adsorption is higher, leaving less water available for small particles that are plasticized during the extrusion process and therefore, affecting expansion index and therefore stress values.
Particle size index had an influence on extrudates made with GCM3, showing a high increase in maximum stress values. This increase most likely occurred because higher PSI leads to a major contact surface, and calcium ions could produce more starch–calcium bonds and create a more compact cellular architecture. Thus, higher calcium concentrations produced more rigid structures. Zazueta et al. (2001) demonstrated that higher concentrations of calcium hydroxide produced an increase the area under the force–deformation curve when blue maize extrudates were tested, meaning that a higher stress was required to penetrate the samples.
Conclusions
The PSI from GCM1 and GCM3 showed marked differences; GCM3 contained more fine particles (<140 μm). The TAC was higher in extrudates processed with FMs of 16 and 16.5%. Extrudates elaborated with FM of 16%, 130°C and GCM1 showed the highest TAC (211.1 mg · kg−1). Cyanidin 3-glucoside content showed an increase in extrudates elaborated with FM of 16.5%, 140°C and GCM1. Total anthocyanin and C3G contents were not associated. Color values (L, a, b) showed several changes between treatments. Density was highly affected by FM as well as the expansion index and maximum stress. Feed moisture was the extrusion factor that mostly affected the physicochemical, physical and textural properties of the extrudates. It is possible to retain C3G content in blue corn extrudates.
Acknowledgements
Escalante-Aburto is grateful to CONACyT for the doctoral scholarship provided. The authors thank SEP-PROMEP for the financial support of the Project “Application of Physical, Rheological and Biological Methods in Processing Corn”, through the Network: Conventional and Alternatives Technologies for Cereals Processing.
References
- AACC International . 2000 . Approved methods of analysis (10 th ed.) , St. Paul, MN : AACC .
- Abdel-Aal , M.E.S and Hucl , P. 1999 . A rapid method quantifying total anthocyanins in blue aleurone and purple pericarp wheats . Cereal Chemistry , 76 : 350 354 doi: 10.1094/CCHEM.1999.76.3.350
- Agama , A.E , Astrid , O.M , Farhat , M.I , Paredes , L.O , Ortiz , C.J and Bello , P.L.A. 2004 . Efecto de la nixtamalización sobre las características moleculares del almidón de variedades pigmentadas de maíz . Interciencia , 29 : 643 649
- Agama , A.E , Salinas , M.Y , Pacheco , V.G and Bello , P.L.A. 2011 . Características físicas y químicas de dos razas de maíz azul: Morfología del almidón . Revista Mexicana de Ciencias Agrícolas , 2 : 317 329
- Alavi , S , Karkle , E , Adhikari , K and Keller , L. 2011 . Extrusion research for addressing the obesity challenge . Cereal Foods World , 56 : 56 60 doi: 10.1094/CFW-56-2-0056
- Anton , A.A and Luciano , F.B. 2007 . Instrumental texture evaluation of extruded snack foods: A review . Ciencia y Tecnología Alimentaria , 5 : 254 251
- Battacharya , M and Hanna , M.A. 1988 . Effects of lipids on the properties of extruded products . Journal of Food Science , 53 : 1230 1231 doi: 10.1111/j.1365-2621.1988.tb13572.x
- Bedolla , S and Rooney , L.W. 1984 . Characteristics of U.S. and Mexican instant maize corn flours for tortilla and snack preparation . Cereal Foods World , 29 : 732 735
- Betran , F.J , Bockholt , A.J and Rooney , L. 2001 . “ Blue corn ” . In Specialty corns , Edited by: Hallauer , A.R . 293 337 Ames Iowa, USA : Iowa State University .
- Brennan , C , Brennan , M , Derbyshire , E and Tiwari , B.K. 2011 . Effects of extrusion on polyphenols, vitamins and antioxidant activity of foods . Trends in Food Science and Technology , 22 : 570 575 doi: 10.1016/j.tifs.2011.05.007
- Del Pozo , I.D , Serna , S.S.O , Brenes , H.C and Talcott , T.S. 2007 . Polyphenolics and antioxidant capacity of white and blue corns processed into tortillas and chips . Cereal Chemistry , 84 : 162 168 doi: 10.1094/CCHEM-84-2-0162
- De , Pascual T.S , Santos , B.C and Rivas , G.J.C. 2002 . LC-MS analysis of anthocyanins from purple corn cob . Journal of the Science of Food and Agriculture , 82 : 1003 1006 doi: 10.1002/jsfa.1143
- Ding , Q.B , Ainsworth , A , Plunkett , A , Tucker , G and Marson , H. 2006 . The effect of extrusion conditions on the functional and physical properties of wheat based expanded snacks . Journal of Food Engineering , 73 : 142 148 doi: 10.1016/j.jfoodeng.2005.01.013
- Durge , V.A , Sarkar , S and Sinhgal , S.R. 2011 . Stability of anthocyanins as pre-extrusion colouring of rice extrudates . Food Research International , doi: 10.1016/j.foodres.2011.05.017.
- Dykes , L and Rooney , L.W. 2007 . Phenolic compounds in cereal grains and their health benefits . Cereal Foods World , 52 : 105 111 doi: 10.1094/CFW-52-3-0105
- Fossen , T , Cabrita , L and Anderson , O.M. 1998 . Colour and stability of pure anthocyanins influenced by pH include the alkaline region . Food Chemistry , 63 : 435 440 doi: 10.1016/S0308-8146(98)00065-X
- Garber , W.B , Hsieh , F and Huff , E.H. 1997 . Influence of particle size on the twin-screw extrusion of corn meal . Cereal Chemistry , 74 : 656 661 doi: 10.1094/CCHEM.1997.74.5.656
- González , M.S , Pérez , A.J.J , Salinas , M.Y , Mateus , N , Silva , M.S.A , De , Freitas, V and Santos , B.C. 2008 . Flavanol-anthocyanin pigments in corn: NRM characterisation and presence in different purple corn varieties . Journal of Food Composition and Analysis , 21 : 521 526 doi: 10.1016/j.jfca.2008.05.009
- Gupta , M , Singh , B.A and Dutt , S.A. 2008 . Effect of barley flour on development of rice-based extruded snacks . Cereal Chemistry , 85 : 115 122 doi: 10.1094/CCHEM-85-2-0115
- Harper , J.M and Clark , J.P. 1979 . Food extrusion . Critical Reviews in Food Science and Nutrition , 11 : 155 215 doi: 10.1080/10408397909527262
- Iio , S and Berghofer , E. 1999 . Kinetics of colour changes during extrusion cooking of maize grits . Journal of Food Engineering , 39 : 73 80 doi: 10.1016/S0260-8774(98)00148-4
- Lazou , A and Krokida , M. 2010 . Structural and textural characterization of corn-lentil extruded snacks . Journal of Food Engineering , 100 : 392 408 doi: 10.1016/j.jfoodeng.2010.04.024
- Lopez , M.L.X , Parkin , K.L and Garcia , H.S. 2011 . Phase II-Inducing, polyphenols content and antioxidant capacity of corn (Zea mays L.) from phenotypes of white, blue, red, and purple colors processed into masa and tortillas . Plant Food for Human Nutrition , 66 : 41 47 doi: 10.1007/s11130–011-0210-z
- Maga , J and Liu , M.B. 1993 . Hopi blue corn extrusion . Developments in Food Science , 32 : 983 986
- Martinez , B.F , Chang , Y.K , Bannwart , A.C , Rodriguez , M.E , Guedes , P.A and Gaiotti , E.R. 1998 . Effects of calcium hydroxide and processing conditions on corn meal extrudates . Cereal Chemistry , 75 : 796 801 doi: 10.1094/CCHEM.1998.75.6.796
- Martinez-Flores , H.E , Martinez , B.F , Figueroa , C.J.D and Gonzalez , H.J. 1998 . Tortillas from extruded masa as related to corn genotype and milling process . Journal of Food Science , 63 : 130 133 doi: 10.1111/j.1365-2621.1998.tb15692.x
- Mathew , M.J , Hoseney , C.R and Faubion , M.J. 1999 . Effects of corn sample, mill type and particle size on curl and pet food extrudates . Cereal Chemistry , 76 : 621 624 doi: 10.1094/CCHEM.1999.76.5
- Milan , C.J , Valdez , A.C , Gutierrez , D.R , Cardenas , V.O.G and Mora , E.C. 2007 . Nutritional properties of quality protein maize and chickpea extruded based weaning food . Plant Food for Human Nutrition , 62 : 31 37 doi: 10.1007/s11130-006-0039-z
- Mora , R.S , Gutierrez , U.J.A , Serna , S.S.O , Sanchez , P.P , Reyes , M.C and Milan , C.J. 2010 . Phenolic content and antioxidant activity of tortillas produced from pigmented maize processed by conventional nixtamalization or extrusion cooking . Journal of Cereal Science , 52 : 502 508 doi: 10.1016/j.jcs.2010.08
- Nayak , B , Hai , L.R , Berrios , J.J , Tang , J and Derito , C. 2011 . Bioactivity of antioxidants in extruded products prepared from potato and dry pea flours . Journal of Agricultural and Food Chemistry , 59 : 8233 8243 doi. 10.1021/jf200732p
- Platt , L.L.C , Ramirez , W.B , Torres , C.P.I , Lopez , C.J , Sanchez , M.D.I , Reyes , M.C , Milan , C.J and Morales , R.I. 2010 . Improving textural characteristics of tortillas by adding gums during extrusion to obtain nixtamalized corn flour . Journal of Texture Studies , 41 : 736 755 doi: 10.1111/j.1745-4603.2010.00252.x
- Rocha , G.N.E , Gallegos , I.J.A , Gonzalez , L.R.F , Bello , P.A and Delgado , L.E. 2008 . Physical properties of extruded products from three Mexican common beans (Phaseolus vulgaris L.) cultivars . Plant Food for Human Nutrition , 63 : 99 104 doi: 10.1007/s11130-008-0076-x
- Salinas , M.Y , Martinez , B.F , Soto , H.M , Ortega , P.R and Arellano , V.J.L. 2003 . Effect of alkaline cooking process on anthocyanins in pigmented maize grain . Agrociencia , 37 : 617 628
- Serna , S.S.O. 1996 . Química, almacenamiento e industrialización de los cereales , México 11800, D.F : AGT(Ed.). S.A. .
- Taghvaei , G.A , Motiee , F , Shakeri , E and Abbasian , A. 2010 . Effect of amylose/amylopectin ratio on physico-mechanical properties of rubber compounds filled by starch . Journal of Applied Chemical Research , 4 : 53 60
- Yang , Z , Chen , Z , Yuan , S , Zhai , W , Piao , X and Piao , X. 2009 . Extraction and identification of anthocyanin from purple corn (Zea mays L.) . International Journal of Food Science and Technology , 44 : 2485 2492 doi: 10.1111/j.1365-2621.2009.02045.x
- Yu , L , Ramaswamy , H.S and Boye , J. 2012 . Twin-screw extrusion of corn flour and soy protein isolate (SPI) blends: A response surface analysis . Food Bioprocess Technology , 5 : 485 497 doi: 10.1007/s11947-009-0294-8
- Zazueta , M.J , Martinez , B.F , Jacobo , V.N , Ordorica , F.C and Paredes , L.O. 2001 . Effect of the addition of calcium hydroxide on some characteristics of extruded products from blue maize (Zea mays L.) using response surface methodology . Journal of Science and Food Agriculture , 81 : 1379 1386 doi: 10.1002/jsfa.951
- Zazueta , M.J , Martinez , B.F , Jacobo , V.N , Ordorica , F.C and Paredes , L.O. 2002 . Effects of calcium hydroxide and screw speed on physicochemical characteristics of extruded blue maize . Journal of Food Science , 67 : 3350 3358 doi: 10.1111/j.1365-2621.2002.tb09590.x
- Zhang , W and Hoseney , R.C. 1998 . Factors affecting expansion of corn meals with poor and good expansion properties . Cereal Chemistry , 75 : 639 643 zdoi: 10.1094/CCHEM.1998.75.5.639