Abstract
Restarter yeast strains are used to consume residual sugar (mainly fructose) in stuck wine fermentations. Forty-three yeast strains were evaluated initially. Strain ITD-00068 showed the highest values for the maximum growth rate, maximum fructose consumption rate, and maximum ethanol production rate (0.143, 0.268, and 0.231 h−1, respectively). Therefore, it was selected for further molecular and kinetic analyses. RFLP analysis identified this isolate as Saccharomyces cerevisiae. Strain ITD-00068 consumed 100% and 36% of the fructose present (at 20°C and 30°C, respectively), when cultured in medium with 12% (v/v) ethanol. The fructose consumption rate was reduced by 97% at 30°C, when ethanol was initially present in the media. S. cerevisiae ITD-00068 was tested in stuck fermentation conditions and was able to complete fermentation in 144 h in a commercial red wine, demonstrating that S. cerevisiae ITD-00068 is a potential candidate for use as a restarter in wine stuck fermentation at 20°C.
Las cepas de levadura reiniciadoras se utilizan para consumir los azúcares residuales (principalmente fructosa) en fermentaciones de vino estancadas. La selección de cepas inició a partir de 43 cepas de levadura. La cepa ITD-00068 mostró los valores más altos de las tasas máximas de crecimiento, consumo de fructosa y producción de etanol (0,143, 0,268, and 0,231 h−1, respectivamente). Por lo tanto, fue seleccionada para los posteriores análisis moleculares y cinéticos. Una vez saleccionada, la cepa fue identificada por medio RFLP’s como Saccharomyces cerevisiae. La cepa ITD-00068 consumió 100% y 36% de la fructosa presente (a 20°C y 30°C, respectivamente) cuando se cultivó en un medio con una concentración inicial de 12% (v/v) de etanol. La tasa de consumo de fructosa se redujo en un 97% a 30°C, cuando el etanol estuvo presente desde el inicio en el medio de cultivo. S. cerevisiae ITD-00068 se ensayó en condiciones de fermentación estancada y fue capaz de completar la fermentación en 144 h en un vino tinto comercial, lo que demuestra que S. cerevisiae ITD-00068 es un candidato potencial para su uso como un reiniciador en la fermentación estancada a 20°C.
Palabras claves:
Introduction
Wine production is a complex process where yeasts (principally Saccharomyces cerevisiae) transform sugars present in grape juice into ethanol, CO2, and other important metabolites associated with organoleptic qualities (Pérez-Coello, Briones-Pérez, Ubeda-Iranzo, & Martin-Alvarez, Citation1999). One of the most common problems associated with wine production is stuck fermentation that results when yeast stop metabolic activities and sugars present in grape juice are not completely fermented (Bisson, Citation1999). Unmetabolized sugars result in lower ethanol yields and contamination of the final product with nocive microorganisms. In addition, a sweet flavor is undesirable in dry wines (Berthels, Cordero-Otero, Bauer, Thevelein, & Pretorius, Citation2004).
Several factors including nutrient deficiencies and the presence of toxic compounds have been identified as causes leading to stuck fermentation and may act synchronously in yeast cells (Alexandre & Charpentier, Citation1998; Bisson, Citation1999; Dombek & Ingram, Citation1986; Ingram & Buttke, Citation1984; Mauricio, Guijo, & Ortega, Citation1991; Pampulha & Loureiro-Dias, Citation1990; Salmon, Citation1989). Ethanol toxicity is one of the most important causes of this problem (Alexandre & Charpentier, Citation1998; Bisson, Citation1999). As fermentation proceeds, ethanol concentrations increase and some yeast strains may be unable to survive under these conditions. At the same time, ethanol results in decreased sugar consumption (Ansanay-Galeote, Blondin, Dequin, & Sablayrolles, Citation2001). During wine fermentation, wine yeasts co-ferment glucose and fructose (sugars present in grape juice). Yeasts usually prefer glucose instead of fructose (Berthels, Cordero-Otero, Bauer, Pretorius, & Thevelein, Citation2008; Berthels et al., Citation2004; Tronchoni, Gamero, Arroyo-López, Barrio, & Querol, Citation2009), and accumulating fructose concentrations result in the development of stuck fermentation (Bisson & Butzke, Citation2000). It is known that in S. cerevisiae glucose and fructose are transported into the cell by the same proteins (Reifenberger, Freidel, & Ciriacy, Citation1995; Perez, Luyten, Michel, Riou, & Blondin, Citation2005) and these sugar transporters have a lower affinity for fructose than for glucose (Reifenberger, Boles, & Ciriacy, Citation1997). In addition, ethanol favors a shift in tautomeric equilibrium from fructopyranose to fructofuranose that further diminishes the affinity of the transporter for fructose (Flood, Johns, & White, Citation1996).
When stuck fermentation occurs, reinoculation is carried out to complete the fermentation process (Cavazza, Poznanski, & Trioli, Citation2004; Varela, Pizarro, & Agosin, Citation2004); however, the yeast strain used for this purpose must be capable of consuming residual sugars, mostly fructose, in the presence of elevated ethanol concentrations and other metabolites (Bisson & Butzke, Citation2000). Yeasts capable of adapting to high ethanol concentration are able to survive and continue to consume sugars in later stages of alcoholic fermentation (Ivorra, Pérez-Ortín, & Del Olmo, Citation1999; Pretorius, Citation2000). Non-Saccharomyces species could be used for reinoculation purposes such as Zygosaccharomyces bailii, a fructophilic yeast (prefers fructose instead of glucose) tolerant to high ethanol concentrations (Pina, Goncalves, Prista, & Loureiro-Dias, Citation2004; Sousa, Miranda, Côrte-Real, & Leão, Citation1996). Torulaspora delbrueckii, a S. cerevisiae related species, is used to improve the organoleptic quality of wine and is also tolerant to high ethanol concentrations (Ciani & Maccarelli, Citation1998). Santos et al. (Citation2008) reported that Z. bailii and T. delbrueckii had higher survival rates in the presence of 18% (v/v) ethanol compared with S. cerevisiae. However, fructose consumption occurred more effectively in the presence of S. cerevisiae in medium containing 12% (v/v) ethanol.
Saccharomyces cerevisiae has been used extensively in wine production and for other alcoholic fermentation processes (Fiore, Arrizon, Gschaedler, Flores, & Romano, Citation2005; Torresi, Frangipane, & Anelli, Citation2011) and shown to effectively carry out fermentation in high ethanol concentrations (Pérez-Coello et al., Citation1999; Pretorius, Citation2000). Saccharomyces cerevisiae is a glucophilic yeast (prefers glucose over fructose), but during stuck wine fermentation, fructose becomes the principal sugar in grape must (Guillaume, Delobel, Sablayrollles, & Blondin, Citation2007). The ability of yeast to consume this sugar at high ethanol concentrations (and in the presence of other metabolites) is crucial for wine dryness (Bisson & Butzke, Citation2000). This species has been used for restarting stuck fermentations (Cavazza et al., Citation2004).
On the other hand, little attention has been paid to the use of S. cerevesiae isolated from a high-fructose concentration environments such those present in agave juice. Fermentable sugars (fructose and glucose) are obtained by cooking agave to hydrolyze fructans, which has a glucose/fructose ratio of 1/9 in Agave tequilana (López, Mancilla-Margalli, & Mendoza-Díaz, Citation2003). The glucose/fructose ratio reported for Agave salmiana juice was 1/8 (Michel-Cuello, Juarez-Flores, Aguirre-Rivera, & Pinos-Rodriguez, Citation2008), while this ratio was 1/9 in Agave durangensis juice (Martell-Nevárez et al., Citation2011), which means that fructose represents over 90% of the fermentable sugars in the agave must. Cooking process also causes nitrogen loss by Maillard reactions, leading to low levels of nitrogen in the agave musts (Mancilla-Margalli and López, Citation2002).
Our group isolated 43 yeast strains from the alcoholic fermentation of agave juice used in the production of mezcal (a Mexican distilled alcoholic beverage). This juice contains high concentrations of ethanol in combination with a fructose–glucose ratio of 9:1. Since agave has a high fructose content, combined with low nitrogen content, and taking in to account that stuck fermentations in winemaking are often limited by exactly these two factors; the testing of agave strains for their finishing capabilities is a very logical approach. Therefore, the aim of the present work was to identify and characterize strains that could serve as potential restarter yeasts for stuck wine fermentation.
Materials and methods
Strains used
Strains (n = 43) isolated from the alcoholic fermentation of agave juice for the production of mezcal were maintained in 30% (v/v) glycerol at –20°C. Yeast strains were reactivated in yeast-peptone-dextrose-agar (YPDA) medium: yeast extract, 10 g L−1; peptone, 20 g L−1; dextrose, 20 g L−1; and agar, 20 g L−1. For inoculum preparation, yeast strains were grown in YPD medium (YPDA medium without agar) at a controlled temperature of 30°C with continuous orbital shaking (120 r.p.m.) for 12 h. The commercial wine strain Fermichamp (DSM Food Specialties B.V., The Netherlands) was used as reference strain.
Fermentation conditions for strain selection
Respective strains were screened by carrying out fermentations in 30 mL glass flasks containing 15 mL of modified SF medium containing: yeast extract, 10 g L–1; peptone, 20 g L–1; fructose, 25 g L−1; ethanol, 6% (v/v); and acetic acid 0.03% (v/v). The strains that were able to consume 90–100% of the fructose present in this medium, within the first 48 h, were cultivated in SF medium containing: yeast extract, 10 g L−1; peptone, 20 g L−1; fructose, 25 g L−1; ethanol, 12% (v/v); and acetic acid 0.06% (v/v) as reported by Santos et al. (Citation2008). The amino nitrogen concentration of these media was close to 1000 mg L−1, which comes from the yeast extract and peptone. Medium was inoculated with 1 × 106 cells mL−1, in all cases. All experiments were carried out in triplicate at a controlled temperature of 30°C without agitation. Biomass concentrations were determined by measuring dry weight, while fructose and ethanol concentrations were measured by the Agilent 1200 series high performance liquid chromatography (HPLC) system (Agilent Technologies, CA, USA).
Molecular identification
Identification of the selected strain was performed as follows: DNA of the selected strain was purified from overnight YEPD cultures as described by Querol, Barrio, & Ramón, (Citation1994). The ITS1-5.8S-ITS2 region was amplified using primers ITS1 (5′-TCCGTAGGTGAACCTGCGG-3′) and ITS4 (5′-TCCTCCGCTTATTGATATG-3′) as described by Esteve-Zarzoso, Belloch, Uruburu, & Querol, (Citation1999). Polymerase chain reaction (PCR) products were digested with the restriction endonucleases CfoI, HaeIII, and HinfI (Boehringer Mannheim) as described previously (Esteve-Zarzoso et al., Citation1999; Guillamón, Sabaté, Barrio, Cano, & Querol, Citation1998). PCR products and restriction fragments were separated on 1.4% agarose gels that were subsequently stained with ethidium bromide, visualized under UV light and photographed (Image Master, Pharmacia Biotech, Uppsala, Sweden). Fragment sizes were estimated using DNA markers (100 bp ladder, Gibco-BRL MD, USA). The Yeast-Id database (http://www.yeast-id.com/) was queried with the restriction patterns to identify the selected yeast strain.
Kinetic characterization of the selected strain
Fermentations with the selected strain were conducted in 30 mL glass flasks containing 15 mL SF medium (Santos et al., Citation2008) with 12% (v/v) ethanol and 0.06% (v/v) acetic acid. Medium was inoculated with 1 × 106 cells/mL. Fermentations were carried out in triplicate at controlled temperatures of 20°C and 30°C without agitation. Fermentations without ethanol and acetic acid were also conducted as a control. Fructose and ethanol concentration were measured by HPLC.
Stuck fermentation
A commercial red wine (12% (v/v) ethanol, pH 3.17) was used as the basis to obtain a culture medium that simulates a stuck fermentation. Concentration of glucose and fructose present in the commercial wine were measured by HPLC, obtaining 4.78 and 5.13 g L−1, respectively. Fructose was added to reach a sugar concentration of 15 g L−1, whereby the proportion of fructose was approximately 70% of the sugars at the beginning of the kinetics. Nitrogen was supplied by adding 75 mg L−1 of (NH4)2SO4. Two fermentations were performed in this medium, in order to compare the sugar consumption kinetics of the strain S. cerevisiae ITD-00068 against the wine strain Fermichamp. Fermentations were carried out in 30 mL glass flasks containing 15 mL medium inoculated with 2 × 107 cells mL−1. Fermentations were carried out in triplicate at a controlled temperature of 20°C for 144 h without agitation. Reducing sugar concentrations were measured by the dinitrosalicylic acid method (Miller, Blum, Glennon, & Burton, Citation1960).
Analytical methods
Fructose and ethanol concentrations were measured using the Agilent 1200 series HPLC system equipped with a refractive index detector. Standard curve was derived from duplicate readings of 10 fructose solutions ranging in concentration from 1 to 60 g L−1 and ethanol concentrations ranging from 0.12 to 13.2% (v/v). The retention time was 13.89 min for fructose and 25 min for ethanol. Samples were filtered through a 0.45 µm nylon membrane and analyzed using a 300 × 7.8 mm Rezex Roa Organic Acid H+ (8%) ionic exchange column (Phenomenex Inc., CA, USA) at 20°C. The injection volume was 5 µL and the mobile phase was 0.005 N H2SO4 in distilled water at 0.5 mL min−1.
Statistical analysis
Data were analyzed with Statistica software version 7 (Statsoft Inc., OK, USA). Tukey’s least-significant differences test was used to define differences between means at the 5% significance level (P < 0.05).
Table 1. Fructose consumed after 48 h incubation by the 43 yeast strains cultured in modified SF medium containing 6% (v/v) ethanol.
Fructosa consumida después de 48 h de incubación por las 43 cepas de levadura cultivadas en medio SF modificado, el cual contenía 6% (v/v) de etanol.
Results
Strain selection
Strain selection was performed using a two-stage strategy. During the first stage, selection was carried out by growing 43 yeast strains in modified SF medium in duplicate and fructose concentrations determined every 24 h. Twenty-four strains consumed all of the fructose present in the medium (25 g L−1) in the presence of 6% (v/v) ethanol. Only strains ITD-00068, ITD-00056, ITD-00115, ITD-00220, ITD-00204, and ITD-00205 consumed 90–100% of the fructose present in the medium within the first 48 h () and therefore chosen for further analysis.
During the second-stage, selected strains were grown in SF medium by triplicate. Fructose, ethanol, and biomass concentrations were determined every 6 h. Strain ITD-00068 completely consumed the available fructose after 48 h of fermentation (), whereas strains ITD-00056, ITD-00115, ITD-00205, ITD-00204, and ITD-00220 consumed 92%, 74%, 73%, 71%, and 63% of the available fructose, respectively.
Figure 1. Fructose consumption (A) and ethanol production (B) of the tested strains in SF medium in the presence of 12% (v/v) ethanol at 30°C. ITD-00056 (▼), ITD-00220 (∆), ITD-00204 (■), ITD-00115 (□), ITD-00068 (●), ITD-00205 (○). Data are expressed as the mean ± standard deviation from three independent incubations.
Consumo de fructosa (A) y producción de etanol (B) de las cepas cultivadas en medio SF en presencia de 12% (v/v) de etanol a 30°C. ITD-00056 (▼), ITD-00220 (∆), ITD-00204 (■), ITD-00115 (□), ITD-00068 (●), ITD-00205 (○). Los datos se expresan como media ± desviación estándar de tres incubaciones independientes.
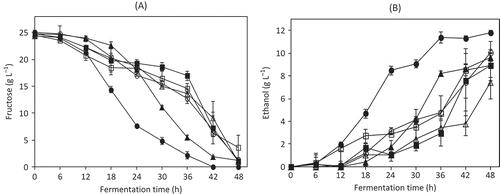
Ethanol production was calculated by subtracting the initial amount of ethanol added to the medium to ethanol present in each sample. Strain ITD-00068 produced the highest quantity of ethanol (11.82 g L−1), while strains ITD-00205, ITD-00056, ITD-00115, ITD-00204, and ITD-00220 produced 10.06, 9.65, 8.93, 8.90, and 7.46 g L−1, respectively ().
Yeast growth, fructose consumption, and ethanol production curves were fitted by numerical solutions to logistic equation (Equations (1), (2), and (3), respectively) in order to obtain the maximum growth rates (µm), maximum fructose consumption rates (kF), and maximum ethanol production rates (kE) ().
Table 2. Kinetic parameters of growth, fructose consumption, and ethanol production of the tested strains in SF medium containing 6% (v/v) ethanol at 30°C.
Parámetros cinéticos de crecimiento, consumo de fructosa y producción de etanol de las cepas cultivadas en medio SF, el cual contenía 6% (v/v) de etanol, a 30°C.
The coefficient of determination (r2) obtained for all cases was >0.94. The highest µm, kF, and, kE values were observed for strain ITD-00068 (0.143, 0.268, and 0.231 h−1, respectively). These values were 15%, 43%, and 51% higher than those observed for strain ITD-00056 that had the second highest values. Statistical analysis demonstrated that these differences were significant (P < 0.05). Based on the results presented above strain ITD-00068 was selected for further molecular and kinetic analyses.
Molecular identification
The amplified ITS1-5.8S-ITS2 products corresponding to strain ITD-00068 were subjected to restriction endonuclease digestion and resulting restriction patterns compared against patterns present in the Yeast-ID database, which allows for the identification of yeast strains based on genomic DNA restriction patterns. This analysis identified strain ITD-00068 as a S. cerevisiae strain with 99% confidence based on the restriction pattern for S. cerevisiae strain CETC1485. In order to confirm this identification, the ITS1-5.8S-ITS2 intergenic region of the strain ITD-00068 was also digested with the enzyme ScrfI, which provides a test that unequivocally differentiated S. cereviciae from S. paradoxus (Fernández-Espinar, Esteve-Zarzoso, Querol, & Barrio, Citation2000). These results (fragments of 320 y 400 pb) demonstrated that the strain ITD-00068 corresponds to S. cerevisiae.
Kinetic characterization
Kinetic analyses of S. cerevisiae ITD-00068 were carried out in fructose-containing medium (25 g L−1). Independent variables were the incubation temperature (20°C and 30°C) and the initial ethanol concentration (0 and 12% v/v). describes the kinetics of fructose consumption and ethanol production obtained under these fermentation conditions. At 20°C, in the presence of 12% (v/v) ethanol, S. cerevisiae ITD-0068 consumed 100% of the fructose added to the culture medium after 120 h of culture (), and S. cerevisiae ITD-00068 consumed 100% of the fructose present in the culture medium in 30 h when grown at 20°C in the absence of added ethanol () while the final ethanol production was very similar under both growth conditions ( and ). Therefore, the presence of ethanol (12% v/v) is a stress factor that quadrupled the time required for total consumption of the initial fructose. However, the yield of ethanol/fructose was not modified (0.43 ± 0.007 and 0.44 ± 0.13, without ethanol and with ethanol, respectively). In addition, during fermentation at 30°C, S. cerevisiae ITD-00068 was unable to completely consume fructose in the presence of 12% (v/v) ethanol. Saccharomyces cerevisiae ITD-00068 consumed approximately 36% (w/v) of the total fructose and produced 3.8 g L−1 ethanol (). When ethanol was absent from the medium, S. cerevisiae ITD-00068 consumed 100% of the fructose after 20 h and produced 10 g L−1 ethanol (). The fructose consumption rate was also calculated as described above. In experiments carried out at 20°C, the rate of fructose consumption was reduced by 72% when ethanol was initially present in the medium, whereas at 30°C reduction was 97% ( ).
Figure 2. Fructose consumption and ethanol production by S. cerevisiae ITD-00068. (A) Fermentation at 20°C in SF medium with 12% (v/v) ethanol added. (B) Fermentation at 20°C in SF medium without ethanol added. (C) Fermentation at 30°C in SF medium with 12% (v/v) ethanol added. (D) Fermentation at 30°C in SF medium without ethanol added. Fructose (○) and ethanol (∆) concentrations. Ethanol production was calculated by subtracting the initial concentration of ethanol, to ethanol determined in every sample when ethanol was added to the medium (A and C). Results represent the mean value of three independent experiments.
Consumo de fructosa y producción de etanol por Saccharomyces cerevisiae ITD-00068. (A) Fermentación a 20°C en medio SF con 12% de etanol adicionado. (B) Fermentación a 20°C en medio SF sin etanol adicionado. (C) Fermentación a 30°C en medio SF con 12% de etanol adicionado. (D) Fermentación a 30°C en medio SF sin etanol adicionado. Concentraciones de fructosa (○) y etanol (∆). La producción de etanol fue calculada restando la concentración inicial de etanol a la concentración determinada en cada muestra, cuando se agregó etanol al medio (A y C). Los resultados representan las medias de 3 experimentos independientes.
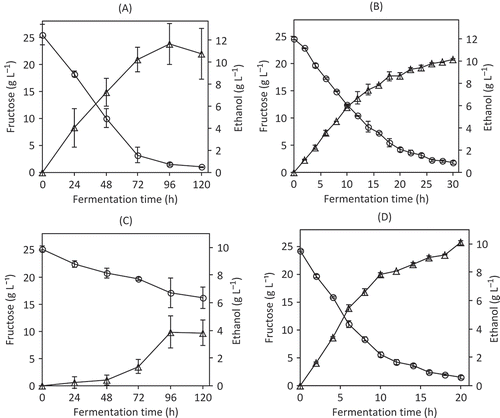
Table 3. Fructose consumption rates by S. cerevisiae ITD-00068 at 20°C or 30°C in the presence or absence of 12% (v/v) ethanol.
Tasas de consumo de fructosa por la cepa S. cerevisiae ITD-00068 a 20 o 30°C en presencia o ausencia de 12% (v/v) de etanol.
Stuck fermentation
When S. cerevisiae ITD-00068 was tested under stuck fermentation conditions, this strain was able to reduce the concentration of reducing sugars from 14 to 3.5 g L−1 in a time of 144 h of fermentation ( ). This figure also shows that the wine strain Fermichamp presented a very similar performance. The residual concentration of sugar must be less than 4 g L−1, to consider the fermentation completed to produce a dry wine (Bisson, Citation1999).
Figure 3. Comparison of sugar consumption kinetics between S. cerevisiae ITD-00068 strain (○) and the wine strain Fermichamp (∆). Fermentation was performed in red wine with 12% (v/v) ethanol and 15 g L−1 of reducing sugars. Data are expressed as the mean ± standard deviation from three independent incubations.
Comparación de las cinéticas de consumo de azúcares de las cepa S. cerevisiae ITD-00068 (○) contra la cepa vínica Fermichamp (∆). Las fermentaciones fueron desarrolladas en vino tinto con 12% de etanol y 15 g azúcares reductores/L. Los datos se expresan como media ± desviación estándar de 3 incubaciones independientes.
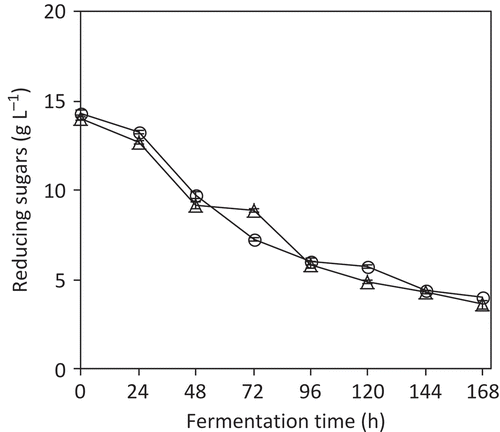
Discussion
Previous reports (Carrasco, Querol, & Del Olmo, Citation2001; Zuzuarregui & Del Olmo, Citation2004) suggest that S. cerevisiae strains have different capacities to survive in media containing ethanol, while Berthels et al. (Citation2004) found that inhibition of fructose consumption by ethanol varied between different S. cerevisiae strains. This could explain the results obtained in this study during the selection of strains where it was observed that the strains tested showed different times to completely consume the fructose present, as well as different rates of growth, glucose consumption, and ethanol production. The logistic equation was employed to obtain kinetic parameters as a means of selecting strain ITD-00068. It should be pointed out that this equation is commonly used to fit growth kinetics (Wachenheim, Patterson, & Ladisch, Citation2003), but it has been also used to fit substrate consumption and product formation data (Asenstorfer, Markides, Iland, & Jones, Citation2003; Van de Lagemaat & Pyle, Citation2005).
Although one of the most important criteria for strain selection in the winemaking industry is the capacity of the strain to survive high ethanol concentrations (Zuzuarregui & Del Olmo, Citation2004), this characteristic is not sufficient for the selection of a strain for the reactivation of a stuck fermentation. A restarter strain for stuck fermentation must be capable not only of surviving high ethanol concentrations but also consuming fructose and producing additional ethanol as shown for strain ITD-00068. Santos et al. (Citation2008) found that non-Saccharomyces strains Z. bailii (a fructophilic strain) and T. delbrueckii had high survival rates in 18% (v/v) ethanol compared to six S. cerevisiae strains with poor survival rates. Nevertheless, two S. cerevisiae strains and one Z. bailii strain consumed all available fructose in a simulated stuck fermentation and the S. cerevisiae strain did so at a more rapid rate.
Bisson (Citation1999) reported that residual sugar values of up to 4 g L−1 were acceptable for dry wines. Saccharomyces cerevisiae ITD-00068 showed an excellent capacity for consuming fructose and producing additional ethanol since this strain reached the 4 g L−1 fructose concentration in 72 h at 20°C in the presence of 12% (v/v) ethanol (). This result exceeded values reported by Santos et al. (Citation2008), who found that a commercial S. cerevisiae strain reached the acceptable sugar concentration (4 g L−1) in 120 h under the same fermentation conditions used in the present study.
Ansanay-Galeote et al. (Citation2001) showed that fermentation rates decreased in S. cerevisiae in the presence of ethanol because of an effect on glucose uptake. In addition, ethanol inhibited the activity of crucial glycolytic enzymes (Chi & Arneborg, Citation1999). In this study, the highest fructose consumption rate (0.140 h−1) was obtained at 30°C, when no ethanol was added to the medium (). When comparing results at two different temperatures, S. cerevisiae ITD-00068 consumed fructose faster (40%) at 30°C than at 20°C, when ethanol was absent in the medium. However, in the presence of ethanol, the fructose consumption rate was 35-fold higher at 20°C than at 30°C. Thus, ethanol and temperature seemed to have a synergic effect on yeast at 30°C when ethanol was initially present in the medium and the yeast could not metabolize all of the fructose in the medium (), which agrees with previous reports (Beltran, Rozès, Mas, & Guillamón, Citation2007; Chaney, Rodriguez, Fugelsang, & Thornton, Citation2006).
The plasma membrane is most sensitive to ethanol stress since ethanol modifies plasma membrane fluidity (Belloch, Orlic, Barrio, & Querol, Citation2008). Ethanol inhibits both yeast growth and hexose transport activity in S. cerevisiae (Leao & Van Uden, Citation1982; Salmon, Vincent, Mauricio, Bely, & Barre, Citation1993), resulting in an inability of the yeast to metabolize hexoses during alcohol fermentation. You, Rosenfield, & Knipple, (Citation2003) found a correlation between ethanol tolerance and increasing levels of unsaturated fatty acids present in S. cerevisiae lipid membranes. Furthermore, it is well known that at low temperatures, yeasts increase the percentage of unsaturated fatty acids present in the cell membrane (Walker & Van Dijck, Citation2006). This could explain the strong inhibition of fructose consumption at 30°C in the presence of 12% (v/v) ethanol. Nevertheless, it would be interesting to investigate the thermodynamic basis of the synergic effect of temperature and ethanol on fructose consumption by S. cerevisiae.
Experiments using red wine showed that strain ITD-00068 adapted to prevailing conditions in a simulated medium of a stuck fermentation. This yeast was also able to successfully reduce the sugar concentration to a level below the maximum acceptable concentration for dry wines. It should be pointed out that S. cerevisiae ITD-00068 presented a fermentation kinetic very similar to that showed by the industrial wine strain Fermichamp, which is known by its capacity to restart stuck fermentations (Guillaume et al., Citation2007). This finding demonstrates that S. cerevisiae ITD-00068 is a potential candidate that can be used as a restarter in a wine stuck fermentation at 20°C.
Acknowledgements
The authors thank the grant DGO-2007-C01-67924 from “Fondo Mixto CONACYT-Gobierno del Estado de Durango.” The first author also acknowledges the scholarship from CONACyT.
References
- Alexandre, H., & Charpentier, C. (1998). Biochemical aspects of stuck and sluggish fermentation in grape must. Journal of Industrial Microbiology and Biotechnology, 20, 20–27.
- Ansanay-Galeote, V., Blondin, B., Dequin, S., & Sablayrolles, J. M. (2001). Stress effect of ethanol on fermentation kinetics by stationary-phase cells of Saccharomyces cerevisiae. Biotechnology Letters, 23, 677–681.
- Asenstorfer, R. E., Markides, A. J., Iland, P. G., & Jones, G. P. (2003). Formation of vitisin A during red wine fermentation and maturation. Australian Journal of Grape and Wine Research, 9, 40–46.
- Belloch, C., Orlic, S., Barrio, E., & Querol, A. (2008). Fermentative stress adaptation of hybrids within the Saccharomyces sensu stricto complex. International Journal of Food Microbiology, 122, 188–195.
- Beltran, G., Rozès, N., Mas, A., & Guillamón, J. M. (2007). Effect of low-temperature fermentation on yeast nitrogen metabolism. World Journal of Microbiology and Biotechnology, 23, 809–815.
- Berthels, N. J., Cordero-Otero, R. R., Bauer, F. F., Pretorius, I. S., & Thevelein, J. M. (2008). Correlation between glucose/fructose discrepancy and hexokinase kinetic properties in different Saccharomyces cerevisiae wine yeast strains. Applied Microbiology and Biotechnology, 77, 1083–1091.
- Berthels, N. J., Cordero-Otero, R. R., Bauer, F. F., Thevelein, J. M., & Pretorius, I. S. (2004). Discrepancy in glucose and fructose utilization during fermentation by Saccharomyces cerevisiae wine yeast strains. FEMS Yeast Research, 4, 683–689.
- Bisson, L. F. (1999). Stuck and sluggish fermentations. American Journal of Enology and Viticulture, 50, 107–119.
- Bisson, L. F., & Butzke, C. E. (2000). Diagnosis and rectification of stuck and sluggish fermentations. American Journal of Enology and Viticulture, 51, 168–177.
- Carrasco, P., Querol, A., & Del Olmo, M. (2001). Analysis of the stress resistance of commercial wine yeast strains. Archives of Microbiology, 175, 450–457.
- Cavazza, A., Poznanski, E., & Trioli, G. (2004). Restart of fermentation of simulated stuck wines by direct inoculation of active dry yeast. American Journal of Enology and Viticulture, 55, 160–167.
- Chaney, D., Rodriguez, S., Fugelsang, K., & Thornton, R. (2006). Managing high-density commercial scale wine fermentations. Journal of Applied Microbiology, 100, 689–698.
- Chi, Z., & Arneborg, N. (1999). Relationship between lipid composition, frequency of ethanol-induced respiratory deficient mutants and ethanol tolerance in Saccharomyces cerevisiae. Journal of Applied Microbiology, 86, 1047–1052.
- Ciani, M., & Maccarelli, F. (1998). Oenological properties of non-Saccharomyces yeasts associated with wine-making. World Journal of Microbiology and Biotechnology, 14, 199–203.
- Dombek, K. M., & Ingram, L. O. (1986). Magnesium limitation and its role in apparent toxicity of ethanol in yeast fermentation. Applied and Environmental Microbiology, 52, 975–981.
- Esteve-Zarzoso, B., Belloch, C., Uruburu, F., & Querol, A. (1999). Identification of yeasts by RFLP analysis of the 5.8S rRNA gene and the two ribosomal internal transcribed spacers. International Journal of Systematic Bacteriology, 49, 329–337.
- Fernández-Espinar, M. T., Esteve-Zarzoso, B., Querol, A., & Barrio, E. (2000). RFLP analysis of the ribosomal internal transcribed spacers and the 5.8S rRNA gene region of the genus Saccharomyces: A fast method for species identification and the differentiation of flor yeasts. Antonie Van Leeuwenhoek International Journal of General and Molecular Microbiology, 78, 87–97.
- Fiore, C., Arrizon, J., Gschaedler, A., Flores, J., & Romano, P. (2005). Comparison between yeasts from grape and agave musts for traits of technological interest. World Journal of Microbiology and Biotechnology, 21, 1141–1147.
- Flood, A. E., Johns, M. R., & White, E. T. (1996). Mutarotation of D-fructose in aqueous-ethanolic solutions and its influence on crystallization. Carbohydrate Research, 288, 45–56.
- Guillamón, J. M., Sabaté, J., Barrio, E., Cano, J., & Querol, A. (1998). Rapid identification of wine yeast species based on RFLP analysis of the ribosomal internal transcribed spacer (ITS) region. Archives of Microbiology, 169, 387–392.
- Guillaume, C., Delobel, P., Sablayrollles, J. M., & Blondin, B. (2007). Molecular basis of fructose utilization by the wine yeast Saccharomyces cerevisiae: A mutated HXT3 allele enhances fructose fermentation. Applied and Environmental Microbiology, 73, 2432–2439.
- Ingram, L. O., & Buttke, T. M. (1984). Effects of alcohols on microorganisms. Advances in Microbial Physiology, 25, 253–300.
- Ivorra, C., Pérez-Ortín, J. E., & Del Olmo, M. (1999). An inverse correlation between stress resistance and stuck fermentations in wine yeasts. A molecular study. Biotechnology and Bioengineering, 64, 698–708.
- Leao, C., & Van Uden, N. (1982). Effects of ethanol and other alkanols on the glucose transport system of Saccharomyces cerevisiae. Biotechnology Letters, 4, 721–724.
- López, M. G., Mancilla-Margalli, N. A., & Mendoza-Díaz, G. (2003). Molecular structures of fructans from Agave tequilana Weber var. Azul. Journal of Agricultural and Food Chemistry, 51, 7835–7840.
- Mancilla-Margalli, N. A., & López, M. G. (2002). Generation of Maillard Compounds from Inulin during the Thermal Processing of Agave tequilana Weber Var. azul. Journal of Agricultural and Food Chemistry, 50, 806–812.
- Martell-Nevárez, M. A., Córdova-Gurrola, E., Soto-Cruz, N. O., Favela-Torres, E., López-Pérez, M. G., & Rutiaga-Quiñones, O. M. (2011). Effect of fermentation temperature on chemical composition of mezcals produced with juice of Agave duranguensis using different genera of native yeast. African Journal of Microbiology Research, 4, 3669–3676.
- Mauricio, J. C., Guijo, S., & Ortega, J. M. (1991). Relationship between phospholipid and sterol content in Saccharomyces cerevisiae and Torulaspora delbrueckii and their fermentation activity in grape musts. American Journal of Enology and Viticulture, 42, 301–308.
- Michel-Cuello, M., Juarez-Flores, B., Aguirre-Rivera, J., & Pinos-Rodriguez, J. (2008). Quantitative characterization of nonstructural carbohydrates of mezcal agave (Agave salmiana otto ex salmdick). Journal of Agricultural and Food Chemistry, 56, 5753–5757.
- Miller, G. L., Blum, R., Glennon, W. E., & Burton, A. L. (1960). Measurement of carboxymethylcellulase activity. Analitical Biochemistry, 2, 127–132.
- Pampulha, M. A., & Loureiro-Dias, M. C. (1990). Activity of glycolytic enzymes of Saccharomyces cerevisiae in the presence of acetic acid. Applied Microbiology and Biotechnology, 34, 375–380.
- Perez, M., Luyten, K., Michel, R., Riou, C., & Blondin, B. (2005). Analysis of Saccharomyces cerevisiae hexose carrier expression during wine fermentation: Both low- and high-affinity HXT transporters are expressed. FEMS Yeast Research, 5, 351–361.
- Pérez-Coello, M. S., Briones-Pérez, A. I., Ubeda-Iranzo, J. F., & Martin-Alvarez, P. J. (1999). Characteristics of wines fermented with different Saccharomyces cerevisiae strains isolated from the La Mancha region. Food Microbiology, 16, 563–573.
- Pina, C., Goncalves, P., Prista, C., & Loureiro-Dias, M. C. (2004). Ffz1, a new transporter specific for fructose from Zygosaccharomyces bailii. Microbiology, 150, 2429–2433.
- Pretorius, I. (2000). Tailoring wine yeast for the new millennium: Novel approaches to the ancient art of winemaking. Yeast, 16, 675–729.
- Querol, A., Barrio, E., & Ramón, D. (1994). Population dynamics of wine yeast strains in natural fermentation. International Journal of Food Microbiology, 21, 315–323.
- Reifenberger, E., Boles, E., & Ciriacy, M. (1997). Kinetic Characterization of individual hexose transporters of Saccharomyces cerevisiae and their relation to the triggering mechanisms of glucose repression. European Journal of Biochemistry, 245, 324–333.
- Reifenberger, E., Freidel, K., & Ciriacy, M. (1995). Identification of novel HXT genes in Saccharomyces cerevisiae reveals the impact of individual hexose transporters on glycolytic flux. Molecular Microbiology, 16, 157–167.
- Salmon, J. M. (1989). Effect of sugar transport inactivation in Saccharomyces cerevisiae on sluggish and stuck enological fermentations. Applied and Environmental Microbiology, 55, 953–958.
- Salmon, J. M., Vincent, O., Mauricio, J. C., Bely, M., & Barre, P. (1993). Sugar transport inhibition and apparent lost of activity in Saccharomyces cerevisiae as a major limiting factor of enological fermentations. American Journal of Enology and Viticulture, 44, 56–64.
- Santos, J., Sousa, M. J., Cardoso, H., Inácio, J., Silva, S., Spencer-Martins, I., & Leao, C. (2008). Ethanol tolerance of sugar transport, and the rectification of stuck wine fermentations. Microbiology, 154, 422–430.
- Sousa, M. J., Miranda, L., Côrte-Real, M., & Leão, C. (1996). Transport of acetic acid in Zygosaccharomyces bailii: Effects of ethanol and their implications on the resistance of the yeast to acidic environments. Applied and Environmental Microbiology, 62, 3152–3315.
- Torresi, S., Frangipane, M. T., & Anelli, G. (2011). Biotechnologies in sparkling wine production. Interesting approaches for quality improvement: A review. Food Chemistry, 129, 1232–1241.
- Tronchoni, J., Gamero, A., Arroyo-López, F. N., Barrio, E., & Querol, A. (2009). Differences in the glucose and fructose consumption profiles in diverse Saccharomyces wine species and their hybrids during grape juice fermentation. International Journal of Food Microbiology, 134, 237–243.
- Van de Lagemaat, J., & Pyle, D. L. (2005). Modelling the uptake and growth kinetics of Penicillium glabrum in a tannic acid-containing solid-state fermentation for tannase production. Process Biochemistry, 40, 1773–1782.
- Varela, C., Pizarro, F., & Agosin, E. (2004). Biomass content governs fermentation rate in nitrogen-deficient wine musts. Applied and Environmental Microbiology, 70, 3392–3400.
- Wachenheim, D. E., Patterson, J. A., & Ladisch, M. R. (2003). Analysis of the logistic function model: Derivation and applications specific to batch cultured microorganisms. Bioresource Technology, 86, 157–164.
- Walker, G. M., & Van Dijck, P. (2006). Physiological and molecular responses of yeasts to the environment. In A. Querol and G. Fleet (Eds.), Yeast in Food and Beverages (pp. 111–152). Berlin: Springer.
- You, K. M., Rosenfield, C. L., & Knipple, D. C. (2003). Ethanol tolerance in the yeast Saccharomyces cerevisiae is dependent on cellular oleic acid content. Applied and Environmental Microbiology, 69, 1499–1503.
- Zuzuarregui, A., & Del Olmo, M. (2004). Analyses of stress resistance under laboratory conditions constitute a suitable criterion for wine yeast selection. Antonie van Leeuwenhoek, 85, 271–280.