Abstract
The objective of the presented work was to determine the effect of infrared heating on the physicochemical characteristics of Mexican common bean flour. Variety of Pinto Villa beans were used in the present experiment. Infrared heating was fitted at 0, 10, 20 and 40 Hz. Power absorption, relative crystallinity by X-ray diffraction, proximate analysis, water absorption capacity, water absorption index, viscosity, and total, resistant and available starch methods were used to characterize the common bean flour. X-ray diffraction data showed changes in the diffraction patterns attributable to the possible formation of amylose–lipid complex. Water absorption capacity (WAC) and water absorption index (WAI), as well viscosity profiles, were affected by changes in the starch and protein content. Sample with highest power absorbed (20 Hz) show highest values of WAC and WAI and lowest value of viscosity. The content of resistant starch (RS) was highest at medium absorbed power (10 Hz).
El objetivo del presente estudio consistió en determinar el efecto que el calentamiento infrarrojo tiene en las características fisicoquímicas de la harina del frijol mexicano común. En el presente experimento, se utilizaron frijoles de la variedad Pinto Villa. Se calibró el calentamiento infrarrojo a 0, 10, 20 y 40 Hz. Para caracterizar la harina de frijol común, se utilizaron métodos de absorción de potencia, de cristalinidad relativa a partir de difracción por rayos x, de análisis químico, de capacidad de absorción de agua, de índice de absorción de agua, de viscosidad y de almidón total, resistente y disponible. Los datos obtenidos de difracción por rayos x revelaron cambios en los patrones de difracción atribuibles a la posible formación de complejos de amilosa-lípidos. La capacidad de absorción de agua (caa) y el índice de absorción de agua (iaa), así como los perfiles de viscosidad fueron afectados por los cambios en el contenido de almidón y de proteína. La muestra con la más alta absorción de poder (20 Hz) resultó tener los valores de caa y de iaa más elevados y el valor de viscosidad más bajo. El contenido de almidón resistente (ar) fue más alto en la muestra con un poder absorbido medio (10 Hz).
Introduction
Common beans are staple food for undeveloped countries. They are well known as a rich source of carbohydrates, proteins, vitamins and minerals. Of the carbohydrates, starch and nonstarch polysaccharides (dietary fibre) are the major constituents (Vargas-Torres, Osorio-Díaz, Tovar, Paredes-López, Ruales & Bello-Pérez, Citation2004). Based on the hydrolysis rate of starch, it is classified as rapidly digestible starch (RDS), slowly digestible starch (SDS) and resistant starch (RS) (Englyst, Kingman, & Cummings, Citation1992). RS is defined as the sum of starch and products of starch degradation not absorbed in the small intestine of healthy individuals (Asp, Citation1992). The digestibility of the starch (or starchy foods) strongly depends on starch structure and on its processing (Lehmann & Robin, Citation2007).
Cereal starches with A-type pattern are rapidly digested (RDS), whereas tubers and high amylase starches with B-type pattern are somewhat resistant to amylolytic hydrolysis (RS) (Biliaderis, Citation1991). The legume starches with C-type pattern, an intermediate between A-type and B-type patterns, are slow digestible starches (SDS) (Englyst, Kingman, & Cummings, Citation1992). The reduced bioavailability of legume starches has been attributed to the presence of intact tissue/cell structures enclosing starch granules, high levels of amylose (30–65%), high content of viscous soluble dietary fibre components, presence of a large number of antinutrients, ‘B’ type crystallites and strong interactions between amylose chains (Deshpande & Cheryan, Citation1984; Hoover & Sosulski, Citation1985; Siddhuraju & Becker, Citation2001; Tovar, de Francisco, Bjorck, & Asp, Citation1991; Wursch, Dal Vedovo, & Koellreuter, Citation1986). Unfortunately, the difference in digestion properties between A-type cereal starch and B-type potato starch disappeared after cooking (Zhang, Venkatachalam, & Hamaker, Citation2006). They claim that the cooking process completely destroys the semicrystalline structure of native starch granules, with a loss of SDS and an increase of RDS. Besides these crystalline features, the physically inaccessible starch fractions also contribute to the slow digestion.
RS is classified into four types: RS type I is defined as physically inaccessible starch; type II is defined as RS granules; type III is defined as retrograded starch (Champ, Citation2004); and type IV is defined as the RS where novel chemical bonds other than α-(1–4) or α-(1–6) are formed (Sajilata, Singhal, & Kulkarni, Citation2006). In legumes, parts of starches are physically entrapped within the food matrix (RS I) and part as starch granules (RS II), therefore, resisting or delaying the action of digestive enzymes on the starch. These starches are, however, made available when the legume is milled or processed (Wursch et al., Citation1986).
Also, common bean cotyledon, embryonic axis and seed coat are rich sources of protein with 27, 48 and 5 g/100 g content (Morales de Leon, Vazquez-Mata, Torres, Gil-Zenteno, & Bressani, Citation2007). The major obstacle in encouraging the use of common beans is their long cooking time needed to tenderize them (Wang & Daun, Citation2005). Therefore, common beans are processed and consumed in a variety of forms all over the world, depending on the cultural and taste preferences. Several domestic methods for the processing of legumes include dehulling, soaking, boiling, pressure cooking, sprouting and fermentation.
Additionally, these processes improve the beans nutritional value by reducing some of the antinutritional factors (Rocha-Guzman et al., 2008). Few technologies are experimented as emerging technologies such as infrared heating. This is a short time high temperature process that uses electromagnetic radiation in the infrared region (wavelength of 1.8–3.4 nm) to rapidly heat materials (Zheng, Fasina, Sosulski, & Tyler, Citation1998). The process has shown a considerable reduction in the cooking time of legumes, such as cowpeas, lentils and split peas (Arntfield et al., Citation2001; Mwangwela, Waniska, & Minnaar, Citation2006). An opposite effect was reported by several authors (Abdul-Kadir, Bargman, & Rupnow, Citation1990), having increased cooking time after infrared heating, possibly because of the low amounts of water (<18g/100g). Abdul-kadir et al. (Citation1990) showed that infrared heating at low amounts of water increased the cooking time, but also increased water imbibition. The main objective of this study was to determine the effect of infrared heating on the physicochemical characteristics of Mexican common bean flour.
Materials and methods
Common bean (Phaseolus vulgaris L.) (cv Pinto Villa) donated by INIFAP Sinaloa was used (year of harvest: 2010). The seeds were manually cleaned.
Infrared treatment of common beans
The whole seeds without tempering treatment were exposed to infrared heating in a prototype apparatus (Cinvestav – Qro). Briefly, it has two linear arrays of four infrared emitters facing each other, each emitter having a length of 60 cm and a diameter of 0.95 cm (3/8”) and a separation distance of 2.54 cm (1”). The emitters for each array were rounded and made from an alloy of Ni–Cr and isolated with MgO ceramic. The emitters were supported by a frame made of stainless steel and were provided with stainless steel optical reflectors.
The electrical power delivered to each emitter was 900 W (surface temperature of each element was about 900 K) with a wavelength peak emission at 2.9 µm (Gonzalez-Hernandez et al., Citation1996).
Seeds were set into the stainless steel trays on the conveyor at three frequencies (10, 20 and 40 Hz and a control, 0 Hz, with exposure times of 212, 103 and 51 s, respectively), i.e., at higher frequencies, we have higher conveyor speed and lower exposure times to radiation.
Milling
Irradiated seeds were milled in a knife mill (Pulvex 200) and sieved to obtain an initial particle size of 3 mm. After that, particles were milled in a coffee mill and sieved into a particle size lower than 0.25 mm (sieve #60). Flour samples were stored in hermetic seal bags and refrigerated (5°C) until analysis.
Power absorption
The power absorption was determined following the method of Khraisheh, McMinn, and Magee (Citation2004). The expression used was the following equation:
Relative crystallinity percentage
Diffraction and crystallinity percentage for untreated and treated common bean samples were determined using an X-ray Diffractometer (RIGAKU, model Dmax2100, Tokyo, Japan). The samples were placed in the sample cups and then scanned from 20° to 60° (2θ), using cobalt (Co), wavelength (λ) of 1.7889 Å, with a range of 20 KV/2 mA–40 KV/50 mA. Crystallinity percentage was determined by calculating the total area under the diffraction curve and subtracting the corresponding area of no coherent diffraction using Fitk 0.9.7 (curve fitting and peak fitting software) (Arámbula, Barrón, González, Moreno, & Luna, Citation2001).
Proximate analysis
Treated and untreated common bean flours were used for proximate analysis using AOAC (2001) methods for moisture (925.10), ash (923.03), fat (920.39) and protein (46.10), using 6.25 as factor N to protein.
Physical–chemical test
Water absorption capacity (WAC) and water absorption index (WAI)
The WAC was determined following the method of Anderson, Conway, Pfeifer, and Griffin (Citation1969). The WAI was estimated by the method of Anderson et al. (Citation1969). Briefly, flour sample (treated and untreated) (1 g) was added to distilled water (12 mL), and agitated for 30 min at 20°C. After that the sample was set into centrifuge tubes of 25 mL, blended and centrifuged at 4500 rpm for 25 min. The supernatant liquid layer was recollected into the test tube and the recovered volume was measured. The wet pellet was weighted and WAI was calculated as g of pellet per g of dry weight. The WAC was calculated as in the next equation:
Viscosity
The viscosity of the sample was monitored during a thermal treatment, of which the results are called pasting profiles (Newport Scientific, Citation1995) by the use of a Rapid Visco Analyzer (RVA, Newport Scientific, Warriewood, Australia). Moisture content was determined and adjusted to 14 g/100 g (wb). For the profile, 4 g of sample (dry weight basis) was mixed with distilled water to reach a constant weight of 28 g per sample. The suspension was mixed for 2 min using rotary blades to ensure a uniform dispersion and to stabilize the temperature at 50°C (1 min). Then, the temperature was increased to 90°C (5 min) at a constant rate of 5.6°C per min and finally, the mixture was cooled at 50°C (1 min) at the cooling rate of 5.6°C for 7.5 min (Gaytan, Martínez-Bustos, & Morales, Citation2006).
The primary starch pasting viscosity parameters derived from the RVA curve were minimum viscosity, maximum viscosity and total setback (final minus minimum viscosity) (Zeng, Morris, Batey, & Wrigley, Citation1997).
Starch
Total starch
Total starch determination was done by the use of MEGAZYME kit based on the enzymatic method of α-amilase/amiloglucosidase (AA/AMG). The readings were done at 510 nm in a spectrophotomer UV/Vis Cary (Varian).
Total starch was calculated using the next equation:
Resistant starch
Resistant starch (RS) was determined by the use of the AACC International Approved Method 32–40 (AACC, Citation2000). Each sample was read in a spectrophotometer at 510 nm. RS was obtained using the next equation:
where, ΔE = absorbance of the sample versus blank 510 nm, F = absorbance of GOPOD reaction for 100 µg of D-glucose, Vf = final volume of the sample, Vf/0.1 = volume correction, 1/1000 = µg to mg conversion, W = dry weight of the sample, 100/W = conversion factor for determination of % of RS, 162/180 = conversion factor of unbounded D-glucose to anhydrous D-glucose.
Available starch(AS)
Available starch (AS) was determined using the supernatant from the three washing steps of the RS procedure, following the international approved method of the AACC 76–13 (AACC, Citation2000). Upper layer was poured into 100 mL sodium acetate buffer 100 mM (pH 6.0) in a volumetric flask. An aliquot (0.1 mL) of each sample was taken and set in a tube of 25 mL and added 10 µL of diluted AMG (300 U/mL) in sodium acetate buffer 100 mM (pH 6.0) and incubated for 20 min at 50°C. After that, it was added to 3 mL of GOPOD and again was incubated at 50°C for 20 min. Readings were obtained in spectrophotometer at 510 nm. AS percentage was calculated using the next equation (note that RS plus AS equals the total starch):
Results and discussion
The results about power absorption are shown in . Results were obtained by the use of Equation (1) (Khraisheh et al., Citation2004). Samples showed a non-lineal behaviour of power absorption with respect to time. When a biological material as food is exposed to radiation, part of the emitted waves are absorbed by the sample and retained in the superficial layers, other waves travel deeper and another are reflected. Thus, radiation increases the excitement of molecules (vibration and rotation), increasing their temperature, and forming a molecular cluster called overlapping (Decarreau, 1985).
Table 1. Power absorption (Pabs) and Peak distance in X-ray diffraction shown by experimental samples.
Tabla 1. Potencia absorbida y distancia de picos en difracción de rayos X mostrada por las muestras experimentales.
Proximate analysis results are shown on . The lowest protein value was obtained for the sample PVB 20. This sample shows high power absorption (i.e. more radiated sample). Coelho, de Medeiros, and Flores (Citation1978) found lower protein content in macacar beans radiated at 1 kGy. High power absorption is related with the exposure time and energy. These variables could be influenced by several reactions in proteins as scission, crosslinking, interactions with another molecules and precipitation, which could be the result of the total protein content (Delincee, Villavicencio, & Mancini-Filho, Citation1998). Samples with lower power absorption have not shown differences with respect to untreated samples. These results in crude proteins are not in agreement with several authors who reported no differences between radiated and nonradiated legumes (Hania and El-Niely, Citation2007). However, they agree in terms of fat, ash and ELN, which have not shown differences related to the infrared treatment.
Table 2. Proximate analysis of treated and untreated Pinto Villa common bean.
Tabla 2. Análisis proximal de frijol común Pinto Villa tratado y sin tratar.
Results obtained from diffraction spectra for the treated and untreated common beans samples are shown in . The diffraction spectra for untreated bean showed three peaks (). The first peak was observed at 17.52°–17.7° [5.88–5.91 Å], the second peak was found at 20.88°–21.3° [4.87–5.02 Å], and the third peak was located at 26.85°–26.94° [3.96–3.95 Å]. Donald (Citation2004) described that the starch from common beans show three peaks, the first at about 16°–19° (~17°), the second at about 20°–22° (~21°), and the third one at about 25°–27° (~26°). This crystalline structure is identified as C-type. Huang et al. (Citation2007) evaluated three legumes and in all cases, they showed crystalline structure as C-type.
Figure 1. X-ray diffractogram for experimental samples (PVB is untreated common bean, PV10 is common bean at 10 Hz, PV20 is common bean at 20 Hz and PV40 is common bean at 40 Hz).
Figura 1. Difractograma de rayos X de las muestras experimentales (PVB es frijol no tratado, PV10 es frijol comun a 10 Hz, PV20 es frijol comun a 20 Hz, PV40 es frijol comun a 40 Hz).
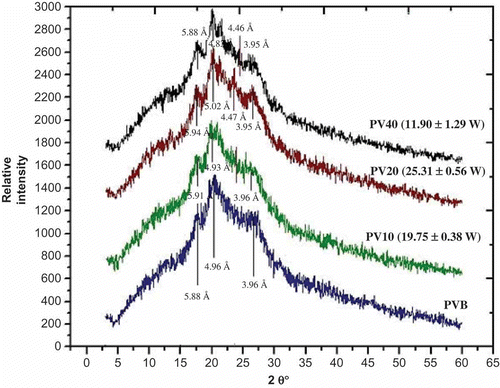
The diffraction spectra for infrared-heated common beans showed a weak shift in peaks in the samples PV20 and PV10; these conditions showed high power absorption (). Samples PV20 and PV40 showed a low pronounced peak at 23.61°–23.64° (4.46 and 4.47 Å); this behaviour is typical of a type V structure. At high water level, swelling of the amorphous region in the starch granule promoted the transformation of crystalline regions. As water becomes limiting, only part of crystallites melt with this mechanism (Maaruf, Che Man, Asbi, Junainah, & Kennedy, Citation2001). On the other side, the free lipids form a complex with amylose molecules by helicoidal inclusion (Hoover & Hadziyev, Citation1981; Zobel, Citation1988).
The relative per cent of crystallinity was calculated. The result is shown in . Sample PV20 (sample with high power absorption) shows higher crystalline phase (treated samples). Also, this sample shows more changes in the diffraction spectra, related with structural changes and the presence of amylose–lipid complex, which drives to an increasing crystalline phase. Bao and Corke (Citation2002) irradiated white rice with gamma rays. They found an increase in the crystallinity at high irradiation levels. Similar results were reported by Rombo and Taylor (Citation2004). Similar findings were reported by Gani, Bashir, Wani, and Masoodi (Citation2012) for bean starch; in both cases, the radiation used was gamma rays; in the present experiment it was infrared radiation. Infrared radiation covers small part of the electromagnetic spectrum with wavelength longer than the visible light. Infrared (IR) is also known to bring about the photodegradation of starch, resulting in the cleavage of glycosidic bonds accompanied by the shortening of the amylose chain and a debranching of the amylopectin chain due to the formation of free radicals (Merlin and Fouassier, Citation1981).
Table 3. Physicochemical characteristics of sample.
Tabla 3. Caracteristicas fisicoquímicas de la muestra.
The results about WAC are shown in . Sample PVB20 showed the highest WAC. IR heating is a dry thermal process that involves molecular changes in the structure of the starch and proteins (Bellido, Arntfield, Cenkowski, & Scalon, Citation2006). The WAC shows a behaviour linearly related with a medium crystalline phase and this is related with the power absorption. Thus, the behaviour could be related with the presence of high content of native starch. Several authors (Desphande, Sathe, & Salunkhe, Citation1984) have indicated that WAC is related to the starch, type and amount of protein present in the cultivar. Mwasaru, Muhammad, Bakar, and Che Man (Citation1999) found that WAC is a function of the water–protein and water–water molecular interactions. Therefore, they concluded that higher absorption capacity is caused by physical retention of water into the new structure built by the processed food. The infrared heating is a complex process, but like many heating processes, the first step is associated with the heat transfer into the seed. Mwangwela et al. (Citation2006) reported that the increase in WAC in infrared-heated cowpea seeds was associated with a fracture of the seed coat and cotyledon. In general, the results found in the present work agree with these authors and support an increase in the water absorption capacity following infrared heating of common beans.
Results obtained for WAI from treated and untreated common beans are shown in . Untreated common beans, in general, showed higher values of WAI in comparison to infrared irradiated beans, at higher frequencies (p < 0.05). Gujska, Czarnecki, and Khan (Citation1996) indicated that denaturation of proteins, starch gelatinization and fibre swelling due to hydrothermal treatments in common beans are responsible for the increase in WAI. Guan and Hanna (Citation2004) reported that higher values of WAI are associated with a higher degree of starch damage (depolymerized starch). There are no reports on changes in WAI as a result of infrared heating of common beans but Rocha-Guzmán et al. (Citation2008) reported a rise in WAI for extruded flours of Pinto Villa, Flor de Mayo and Bayo Victoria cultivars as a result of an extrusion process.
The maximum viscosity in the pasting profile indicates the maximum swelling of starch granules. After gelatinization and during cooling, starch pastes show an increase in the viscosity, depending on amylose content (Zeng et al., Citation1997).
Data about maximum viscosity (Vmax), minimum viscosity (Vmin), total setback (VRt) from treated and untreated common beans are shown in . Sample PV40 (sample with lower power absorption) shows an increase in the viscosity in comparison with the untreated sample (PVB) that could be related with the crosslinking of starch mediated by free radicals present in the sample (Bhat & Karim, Citation2009). On the other side, sample PV20 (higher power absorption) shows a reduction in the viscosity, which may be related with starch breaking (Hayashi and Aoki, Citation1985) related with the power absorption from this sample (high power absorption 25.31 W). This could be sustained by more degradation of the starch granule, data supported by X-ray experiments (% relative crystallinity), and water absorption capacity (highest WAC (3.1 ± 0.0 mL of water/g flour)).
Table 4. Viscosity data from experimental samples.
Tabla 4. Viscosidad de las muestras experimentales.
Several works with radiation and common beans have been done by several authors. Similar behaviour as observed in the present work was reported by McArthur and D´Appolonia (1984) for gamma-radiated common beans. It is important to indicate that the energy of gamma radiation is about 1.24 meV, while the IR radiation shows an energy level between 1.24 and 124 eV. An alternative explanation was provided by Bellido et al. (Citation2006), when they found that the use of IR on common beans increases the presence of air bubbles within the structure of irradiated beans. They hypothesized that air bubbles were created due to internal venting of superheated steam at weak points of the pulse structure (seed was first infrared-heated and then milled). Since soaking controls the hydration of beans by the diffusion process, such a mechanism could be valid to explain higher water absorption capacity. Also, at higher available water level, the gelatinization process is more efficient and its behaviour reflected in the RX diffractogram and in the assay of relative crystallinity.
The total setback () shows lowest values in untreated common bean flour. The IR radiation could affect in a relative grade the granule structure of the irradiated common bean starch, increasing their instability (Thomas and Atwell, Citation1999). The post-gelatinization process shows a molecular rearrangement of the starch, which affects the texture and viscosity of starchy foods. This phenomenon is known as retrogradation.
Total, resistant and available starch
Results about total starch are shown in . No effects from infrared heating were observed in comparison with untreated common bean flour. This result is in agreement with the report by Kashani and Valadon (Citation1984). They worked with irradiated pistachios and did not find effects of radiation doses in the total starch amount. Fasina, Tyler, Pickard, Zheng, and Wang (Citation2001) reported that infrared heating did not change the content of total starch in the legumes.
Table 5. Total, resistant and available starch in treated and untreated samples.
Tabla 5. Almidón total, resistente y disponible en las muestras tratadas y sin tratar.
Results about RS are shown in . The highest content of RS was observed in sample PV10. This increase could be related to more retrogradation effect. This fact is associated with structural rearrangements to develope new crystalline zones. Rombo, Taylor, and Minnaar (Citation2001) reported that samples of irradiated corn and kidney beans showed a maximum digestibility at low radiation doses, but increased starch digestibility at high radiation doses. Rombo et al. (Citation2004), for the same systems, claimed that the previously described behaviour is related with the increase of the ß bond in the starch, and thus the increasing pancreatic α-amilase activity too.
Emami, Meda, and Tyler (Citation2011) found that high intensity infrared heating (micronization) diminished the content of RS in comparison with unprocessed samples. Similar results for microwaved-common beans were observed by Marconi, Ruggeri, Capelloni, Leonardi, and Carnovale (Citation2000). Hódsági, Jámbor, Juhász, Gergely, Gelencsér & Salgó, (Citation2012) Hodsagi et al. (2012) did not find any tendency about RS content when the microwave power was increased in legumes.
Conclusions
Infrared heating at medium power absorption (10 Hz) increased the content of RS of common bean flour. WAC increased linearly as a function of power absorption. WAI in general was higher in untreated common beans than in treated beans. The viscosity was lower when more power absorption was applied to the samples. Sample PV20 showed more relative crystallinity, but this was not related with the amount of enzymatically digestible starch.
Acknowledgements
EEAA and ANMM acknowledge the graduate scholarships provided from CONACYT. The present research was partially funded by a grant from FOMIX-CONACYT-DGO
References
- AACC (2000). Approved methods of the AACC. (10th ed.). St Paul, MN, USA: American Asociation of Cereal Chemists.
- Abdul-Kadir, R., Bargman, T. J., & Rupnow, J. H. (1990). Effect of infrared heat processing on rehydration rate and cooking of Phaseolus vulgaris (Var. Pinto). Journal of Food Science, 55(5), 1472–1473.
- Anderson, R. A., Conway, H. F., Pfeifer, V. F., & Griffin Jr, E. L. (1969). Roll and extrusion-cooking of grain sorghum grits. Cereal Science, 14, 372–375, 381.
- AOAC, Official Methods of Analysis (2001) 17th Ed., AOAC INTERNATIONAL, Gaithersburg, MD, Method 925.10; 923.03; 920.39;46.10
- Arámbula, V. G., Barrón, A. L., González, H. J., Moreno, M. E., & Luna, B. G. (2001). Efecto del tiempo de cocimiento y reposo del grano de maíz (Zea mays L.) nixtamalizado, sobre las características fisicoquímicas, reológicas, estructurales y texturales del grano, masa y tortilla de maíz. Archivos Latinoamericanos De Nutrición, 51, 187–194.
- Arntfield, S. D., Scanlon, M. G., Malcolmson, L. J., Watts, B. M., Cenkowski, S., Ryalnd, D. & Savoie, V. (2001). Reduction in lentil cooking time using micronization: Comparison of 2 micronization temperatures. Journal of Food Science, 66, 500–505.
- Asp, N. G. (1992). Resistant starch. Proceedings from the second plenary meeting of EURESTA. European Journal of Clinical Nutrition, 46, S1.
- Bao, J. S., & Corke, H. (2002). Pasting properties of g-irradiated rice starches as affected by pH. Journal of Agriculture and Food Chemistry, 50, 336–341.
- Bellido, G., Arntfield, S. D., Cenkowski, S., & Scalon, M. (2006). Effects of micronization pretreatments on the physicochemical properties of navy and black beans (Phaseolus vulgaris L). LWT – Food Science and Technology, 39, 779–787.
- Bhat, R., & Karim, A. A. (2009). Impact of radiation processing on starch. Reviews in Food Science and Food Safety, 8, 44–58.
- Biliaderis, C. G. (1991). The structure and interactions of starch with food constituents. Canadian Journal of Physiological and Pharmacological, 69, 60–78.
- Champ, M. M. J. (2004). Physiological aspects of resistant starch and in vivo measurements. Journal of AOAC International, 87, 749–755.
- Coelho, L. C. B. B., de Medeiros, R. B., & Flores, H. (1978). Effect of storage on the amino acid composition and biological quality of irradiated macacar beans Vigna unguiculata (L). Walp. Journal of Food Science, 43, 215–217, 221.
- Decareau, R. V., (1985). Microwaves in the Food Processing Industry. Orlando: Academic Press, pp. 2–37.
- Delincee, H., Villavicencio, A. L. C. H., & Mancini-Filho, J. (1998). Protein quality of irradiated Brazilian beans. Radiation Physics and Chemistry, 52, 43 47.
- Deshpande, S. S., & Cheryan, M. (1984). Effects of phytic acid, divalent cations, and their interactions on α-amylase activity. Journal of Food Science, 49, 516–519.
- Desphande, S. S., Sathe, S. K., & Salunkhe, D. K. (1984). Dry beans of Phaseolus: A review. Part 3. CRC Critical Reviews in Food Science and Nutrition, 21, 137–140.
- Donald, A. M. (2004). Understanding starch structure and functionality. In: A. C. Eliassion (Ed.), Starch in food: Structure, function and applications (156–184). Cambridge, NY: CRS Press. LLC.
- Emami, S., Meda, V., & Tyler, R. T. (2011). Effect of micronisation and electromagnetic radiation on physical and mechanical properties of Canadian barley. International Journal of Food Science & Technology, 46, 421–428.
- Englyst, H. N., Kingman, S. M., & Cummings, J. H. (1992). Classification and measurement of nutritionally important starch fractions. European Journal of Clinical Nutrition, 46, 33–50.
- Fasina, O., Tyler, B., Pickard, M., Zheng, G. -H., & Wang, N. (2001). Effect of infrared heating on the properties of legume seeds. International Journal of Food Science & Technology, 36, 79–90.
- Gani, A., Bashir, M., Wani, S. M., & Masoodi, F. A. (2012). Modification of bean starch by γ-irradiation: Effect on functional and morphological properties. LWT – Food Science and Technology, 49, 162–169.
- Gaytan, M. M., Martínez-Bustos, F., &Morales, S. E. (2006). Aplicación de un proceso de cocimiento dieléctrico en la elaboración de harinas instantáneas de maíz amarillo para preparación de frituras de masa y tortillas. Archivos Latinoamericanos De Nutrición, 50, 366–373.
- Gonzalez-Hernandez, J., De la Luz Martínez, J., Sanchez-Sinencio, F., Martinez-Bustos, F., Figueroa-Cardenas, J. D., & Ruiz-Torres, M. (1996). Method of cooking corn dough tortillas using infrared radiation. US Patent, 5, 469.
- Guan, J., & Hanna, M. A. (2004). Extruding foams from corn starch acetate and native corn starch. Biomacromolecules, 5, 2329–2339.
- Gujska, E., Czarnecki, Z., & Khan, K. (1996). High temperature extrusion of pinto bean (Phaseolus vulgaris) and field pea (Pisum sativum) flours. Polish Journal of Food and Nutrition Science, 46, 51–60.
- Hannia, F. G., & El-Niely, E. (2007). Effect of radiation processing on antinutrients, in-vitro protein digestibility and protein efficiency ratio bioassay of legume seeds. Radiation Physics and Chemistry, 76, 1050–1057.
- Hayashi, T., & Aoki, S. (1985). Effect of irradiation on the carbohydrate metabolism responsible for sucrose accumulation in potatoes. Journal of Agricultural and Food Chemistry, 33, 14–17.
- Hoover, R., & Hadziyev, D. (1981). Characterization of potato starch and its monoglyceride complexes. Starch/Starke, 33, 290–300.
- Hoover, R., & Sosulski, F. (1985). Studies on the functional characteristics and digestibility of starches from Phaseolus vulgaris biotypes. Starch/Stärke, 37, 181–191.
- Huang, D., Schols, H. A., van Soest, J. G., Jin, Z., Sulmann, E., & Voragen, A. G. J. (2007). Physicochemical properties and amylopectin chain profiles of cowpea, chickpea and yellow pea starches. Food Chemistry, 101, 1338–1345.
- Hódsági, M., Jámbor, A., Juhász, E., Gergely, S., Gelencsér, T., & Salgó, A. (2012). Effects of microwave heating on native and resistant starches. Acta Alimentaria, 41, 233–247.
- Kashani, G. G., & Valadon, L. R. G. (1984). Effects of gamma irradiation on the lipids, carbohydrates and proteins of Iranian pistachio kernels. Journal of Food Technology, 19, 631–638.
- Khraisheh, M. A. M., McMinn, W. A. M., & Magee, T. R. A. (2004). Quality and structural changes in starchy foods during microwave and convective drying. Food Research International, 37, 497–503.
- Lehmann, U., & Robin, F. (2007). Slowly digestible starch – its structure and health implications: A review. Trends in Food Science & Technology, 18, 346–355.
- Maaruf, A. G., Che Man, Y. B., Asbi, B. A., Junainah, A. H., & Kennedy, J. F. (2001). Effect of water content on the gelatinization temperature of sago starch. Carbohydrate Polymers, 46, 331–337.
- MacArthur, L. A., & D’Appolonia, B. L. (1984). Gamma radiation of wheat. II. Effects of low dosage radiations on starch properties. Cereal Chemistry, 61, 321–326.
- Marconi, E., Ruggeri, S., Cappelloni, M., Leonardi, D., & Carnovale, E. (2000). Physicochemical, nutritional, and microstructural characteristics of chickpeas (Cicer arietinum L.) and common beans (Phaseolus vulgaris L.) following microwave cooking. Journal of Agriculture and Food Chemistry, 48, 5986–5994.
- Merlin, A., & Fouassier, J. P. (1981). Processus de photo-amorcage de la polymerisation de monomeres vinyliques: mise au point d-un modele reactionnel en solution. Journal de Chimie Physique et de Physico-Chimie Biologique, 78, 267–278.
- Morales de Leon, J. C., Vazquez-Mata, N., Torres, N., Gil-Zenteno, L., & Bressani, R. (2007). Preparation and characterization of protein isolate from fresh and hardened beans (Phaseolus vulgaris L. Journal of Food Science, 72, C96–C102.
- Mwangwela, A. M., Waniska, R. D., & Minnaar, A. (2006). Hydrothermal treatments of two cowpea (Vigna unguiculata L. Walp) varieties: Effect of micronisation on physicochemical and structural characteristics. Journal of the Science of Food and Agriculture, 86, 35–45.
- Mwasaru, M. A., Muhammad, K., Bakar, J., & Che Man, Y. (1999). Effects of isolation technique and conditions on the extractability, physicochemical and functional properties of pigeon-pea (Cajanus cajan) and cowpea (Vigna unguiculata) protein isolates. I. Physicochemical properties. Food Chemistry, 67, 435–443.
- Newport Scientific (1995). Operation Manual for the series 3 rapid Visco Analyser. Newport Sci. Pty. Ltd. Sydney, Australia.
- Rocha-Guzmán, N. E., Gallegos-Infante, J. A., González-Laredo, R. F., Bello-Pérez, A., Delgado-Licon, E., Ochoa-Martínez, A. & Prado-Ortiz, M.J. (2008). Physical properties of extruded products made with three Mexican common beans (Phaseolus vulgaris L). Plant Foods for Human Nutrition, 63, 99–104.
- Rombo, G. O., Taylor, J. R. N., & Minnaar, A. (2001). Effect of irradiation, with and without cooking of maize and kidney bean flours, on porridge viscosity and in vitro starch digestibility. Journal of Science and Food Agriculture, 81, 497–502.
- Rombo, G. O., Taylor, J. R. N., & Minnaar, A. (2004). Irradiation of maize and bean flours: Effects on starch physicochemical properties. Journal of Science and Food Agriculture, 84, 350–356.
- Sajilata, M. G., Singhal, R. S., & Kulkarni, P. R. (2006). Resistant Starch – A Review. Comprehensive Reviews in Food Science and Food Safety, 5, 1–17.
- Siddhuraju, P., &Becker, K. (2001). Effect of various domestic processing methods on antinutrients and in vitro protein and starch digestibility of two indigenous varieties of indian tribal pulse, Mucuna pruriens Var. utilis. Journal of Agricultural and Food Chemistry, 49(6), 3058–3067.
- Thomas, D. J., & Atwell, W. A. (Eds.) (1999). Starches. St. Paul, Minnesota, USA: Eagan Press.
- Tovar, J., de Francisco, A., Bjorck, I., & Asp, N. G. (1991). Relationship between microstructure and in vitro digestibility of starch in precooked leguminous seed flours. Food Structure, 10, 19–26.
- Vargas-Torres, A., Osorio-Díaz, P., Tovar, J., Paredes-López, O., Ruales, J., & Bello-Pérez, L. A. (2004). Chemical composition, starch bioavailability and indigestible fraction of common beans (Phaseolus vulgaris L). Starch/Stärke, 56, 74–78.
- Wang, N., & Daun, J. K. (2005). Determination of cooking times of pulses using an automated Mattson cooker apparatus. Journal of the Science of Food and Agriculture, 85, 1631–1635.
- Wursch, P., Dal Vedovo, S., & Koellreuter, B. (1986). Cell structure and starch nature as key determinants of the digestion rate of starch in legume. American Journal of Clinical Nutrition, 43, 25–29.
- Zeng, M., Morris, C. F., Batey, I. L., & Wrigley, C. W. (1997). Sources of variation for starch gelatinization, pasting, and gelation properties in wheat. Cereal Chemistry, 74, 63–71.
- Zhang, G., Venkatachalam, M., & Hamaker, B. R. (2006). Structural basis for the slow digestion property of native cereal starches. Biomacromolecules, 7, 3259–3266.
- Zheng, G. H., Fasina, O., Sosulski, F. W., & Tyler, R. T. (1998). Nitrogen solubility of cereals and legumes subjected to micronization. Journal of Agricultural and Food Chemistry, 46, 4150–4157.
- Zobel, H. F. (1988). Molecules to granules: A comprehensive starch review. Starch/Starke, 40, 44–50.