Abstract
Caseinate was mixed with trypsin-hydrolyzed bovine gelatin of degree of hydrolysis of 2% in three ratios of 2:1, 4:1 and 6:1 (w/w) and fixed protein concentration of 50 g/L, and treated by transglutaminase of 10 U/g protein at 45°C for 4 h. Three composites generated were protein polymers containing 4-hydroxyproline of 14.50, 10.30 and 6.55 g/kg protein, respectively, and showed better solubility in pH 4.5 but some lower or similar solubility in pH 7–8, higher surface hydrophobicity (1480.1 vs. 1636.1–2143.8) and water holding capacity (WHC) (3.17 vs. 5.96–17.30 kg water/kg protein), but lower in vitro digestibility than caseinate. More hydrolyzed gelatin used in composite preparation conferred the composite enhanced solubility but decreased in vitro digestibility and WHC. The composite generated from caseinate and hydrolyzed gelatin in a ratio of 6:1 (w/w) had the best WHC and might be a potential ingredient for the processed foods such as ham and fish ball.
El procedimiento realizado implicó mezclar gelatina bovina con caseína, hidrolizándola con tripsina en un grado de 2% en tres proporciones de 2:1, 4:1 y 6:1 (w/w), manteniendo una concentración proteica fija de 50 g/L. Posteriormente, La mezcla obtenida fue tratada con 10 U/g de proteína a 45°C durante 4 horas. Tres de los compuestos que se generaron resultaron ser polímeros proteicos que contenían 4-hidroxiprolina de 14,50, 10,30 y 6,55 g/kg de proteína respectivamente. Los mismos mostraron tener mejor solubilidad en un pH de 4,5 y menor o igual solubilidad en un pH de 7–8; una hidrofobicidad superficial más elevada (1480,1 vs. 1636,1–2143,8) y una capacidad de retención hídrica superior (3,17 vs. 5,96–17,30 kg agua/kg de proteína), así como menor digestibilidad in vitro que la caseína. El compuesto de caseína y gelatina hidrolizada en una proporción de 6:1 (w/w) que se generó, resultó tener la mejor capacidad de retención hídrica y podría convertirse en un ingrediente potencial para alimentos procesados como el jamón y la bola de pescado.
Introduction
Transglutaminase (TGase) is able to induce intermolecular and intramolecular cross-linking, acyl-transfer and glutamine deamidation of protein molecules (De Jong & Koppelman, Citation2002). In the past decades, TGase has been widely used to modify functional properties of food proteins, especially when a microbial TGase was isolated from a Streptoverticillium strain (Ando et al., Citation1989). Treatment of food proteins by TGase might lead to desirable or undesirable modification in gelling, emulsifying, rheological properties, in vitro digestibility and other characters. For example, TGase-induced cross-linking could improve solubility of soy protein (Babiker, Khan, Matsudomi, & Kato, Citation1996), decrease emulsifying activity but increase emulsion stability of β-casein (Liu & Damodaran, Citation1999), enhance texture of goat milk yoghurt (Domagała, Wszołek, Tamime, & Kupiec-Teahan, Citation2013), improve thermal stability of whey protein isolate (Damodaran & Agyare, Citation2013). TGase has also been used to generate cross-linked caseinate–gelatin composite (Zhang, Liu, & Zhao, Citation2011), or to prepare edible films from gelatin (Lim, Mine, & Tung, Citation1999), casein–gelatin blend (Chambi & Grosso, Citation2006) and chitosan–whey protein mixture (Di Pierro et al., Citation2006).
Caseinate is one of the protein ingredients widely used in food processing. Gelatin is a low-cost protein ingredient obtained from animal collagen or other tissues by acid or alkaline hydrolysis (Papon, Leblond, & Meijer, Citation2006), and can be used to enhance elasticity, consistency and stability of food products (Sarabia, Gómez-Guillén, & Montero, Citation2000). In the past studies, bovine gelatin of Type A was applied to generate soy protein– and caseinate–gelatin composites (Sheng & Zhao, Citation2013; Zhang & Zhao, Citation2013), resulting in the obtained composites and their dispersions better water holding capacity (WHC) and rheological properties. It is not known yet whether the composites formed by a hydrolyzed gelatin and caseinate also have other properties desirable to the food procedures.
Food proteins can undergo extensive or limited hydrolysis by proteases. Trypsin (EC 3.4.21.4) is one of the serine proteases, and shows higher specificity toward some peptide bonds (Kishimura et al., Citation2007). Application of trypsin in the limited hydrolysis of food proteins was studied extensively (Avramenko, Low, & Nickerson, Citation2013). In the present study, a hydrolyzed bovine gelatin (HBG) was obtained from bovine gelatin of Type A by trypsin-induced limited hydrolysis, and used to prepare casein-hydrolyzed gelatin composite. Commercial caseinate was mixed with the HBG in three blending ratios of 2:1, 4:1 and 6:1 (w/w), respectively, and treated by TGase to prepare three caseinate-hydrolyzed bovine gelatin (CHBG) composites. TGase addition, reaction temperature and time used in composite preparation were briefly investigated. Some functional properties of the composites such as solubility, in vitro digestibility, surface hydrophobicity and WHC were evaluated, with caseinate and a cross-linked caseinate as two controls. The aim was to observe the functional properties of the CHBG composites generated in three blending ratios.
Materials and methods
Materials and chemicals
Caseinate (crude protein content of 83.8% on dry base) was purchased from Shanghai Shan-Pu Chemical Industry Co., Ltd. (Shanghai, China). Bovine gelatin (Type A with an isoelectric point of pH 7–9) was obtained from Hunan Jinlong Gelatin Co., Ltd. (Xiangtan, Hunan, China). Microbial TGase with a measured activity of 110 U/g was obtained from Jiangsu Yi-Ming Fine Chemical Industry Co., Ltd. (Qinxing, Jiangsu, China). Standard protein markers for sodium dodecyl sulfate–polyacrylamide gel electrophoresis (SDS–PAGE) analysis were purchased from Solarbio Science & Technology Co., Ltd. (Beijing, China). Trypsin (120 kU/g) and pepsin (40 kU/g) were from Sigma Chemical Co. (St. Louis, MO, USA). Other chemicals used were of analytical grade. Distilled water was used to prepare all buffers and solutions.
Preparation of HBG
Limited hydrolysis of the bovine gelatin was carried out at 25°C by adding trypsin (addition level in 100 U/g protein) into the gelatin solution (50 g/L, pH 8.0) with continuous stirring. The gelatin was hydrolyzed for 1, 2, 3 and 4 h, respectively, and then heated at a boiling water bath for 15 min to stop trypsin action. Free amino groups of the collected hydrolysates (i.e. HBG) were measured by the o-phthaldialdehyde method (Church, Swaisgood, Porter, & Catignani, Citation1983), and the corresponding degree of hydrolysis (DH) was calculated by the suggested method of Adler-Nissen (Citation1979). A gelatin hydrolysate with DH of 2% was thus bulk prepared with a selected hydrolysis time and used for composite preparation.
Preparation of CHBG composites and cross-linked caseinate
Caseinate and HBG solution with total protein content of 50 g/L were prepared ahead. The two solutions were mixed to give a caseinate–HBG ratio of 4:1 (w/w). TGase of 5–25 U/g protein was rapidly added into the mixed solution, and the reaction was carried out at 50°C for 4 h. After the reaction, the mixture was precipitated at pH 4.6 and washed twice to remove the free HBG as per the method of Zhang et al. (Citation2011). The collected precipitate (CHBG composite) was redispersed in distilled water. The dispersion was adjusted into pH of 6.8, lyophilized and ground into fine particles with diameter less than 150 μm. The CHBG composites were analyzed for 4-hydroxyproline (4-Hyp) content, which was used to select suitable TGase addition.
Similar approach and treatment were used to select suitable reaction temperature and time. For the selection of reaction temperature, the total protein content, caseinate–HBG ratio, reaction time and TGase addition were fixed at 50 g/L, 4:1 (w/w), 4 h and 10 U/g protein, respectively, while the investigated temperature ranged from 25 to 50°C. For the selection of reaction time, the same conditions were used except that the reaction temperature was fixed at 45°C and the investigated reaction time ranged from 1 to 5 h.
Based on the selected conditions and treatment used above, a CHBG composite was prepared and named as CHBG composite (4:1). Similarly, two other composites named as CHBG composite (2:1) and CHBG composite (6:1) were prepared by caseinate–HBG ratios of 2:1 and 6:1 (w/w), respectively. A cross-linked caseinate was prepared with the same conditions but without HBG addition. These samples were redissolved and adjusted into pH of 6.8, lyophilized and grounded into fine particles (<150 μm).
Chemical and electrophoretic analyses
Crude nitrogen content of caseinate, bovine gelatin, the prepared HBG and CHBG composites was assayed by the Kjeldahl method (IDF, Citation2001) in a Kjeltec 2300 Protein Analyzer (Foss Tecator AB, Höganäs, Sweden). Conversion factor used for caseinate and the composites were 6.38 while that used for bovine gelatin and HBG was 5.46.
4-Hyp content was analyzed as per the method of Syk, Ågren, Adawi, and Jeppsson (Citation2001). Protein sample (100 mg), HCl (4 mL, 7.5 mol/L) and phenol (1 mL, 50 g/L) were added into the ampoule bottle successively. The bottle was sealed and heated at 110°C for 24 h. The hydrolysate was neutralized by 10 mol/L NaOH to a pH of 6.0, made to a volume of 25 mL, filtrated through a Whatman No. 4 filter paper, and then diluted by acetate–citrate buffer (pH 6.0). The diluted solution of 2 mL was transferred into a test tube. Chlororamine T solution (0.06 mol/L in 50% n-propanol solution) of 1 mL was added into the tube, and the mixture was incubated at room temperature for 20 min before the addition of p-dimethylaminobenzaldehyde solution (0.05 mol/L, in 3.7 mol/L perchloric acid and 60% n-propanol solution) of 2 mL. The reaction was continued at 60°C for 20 min, cooled down rapidly, and the absorbance was measured at 550 nm by a spectrophotometer (UV-2401PC, Shimadzu, Kyoto, Japan). 4-Hyp standard solutions (1–5 mg/L) were used to generate standard curve. 4-Hyp content of the analyzed sample was calculated and expressed as g/kg protein.
SDS–PAGE analysis was performed, according to the method of Laemmli (Citation1970), by using 150 g/L separating gels and 40 g/L stacking gels. Standard protein markers with molecular mass of 14.4–116.0 kDa were used in the analysis. Sample volume and protein concentration were fixed at 10 μL and 10 g/L, respectively.
Evaluation of functional properties
Solubility of the protein samples was measured at five pH values of 2, 3, 4.5, 7 and 8, respectively, and expressed as nitrogen solubility index (NSI) (Bera & Mukherjee, Citation1989). Each sample of 0.3 g was dispersed in 15 mL phosphate buffer (0.2 mol/L) of the set pH value, and centrifuged at 10,000×g for 15 min at 4°C to collect the supernatant. Nitrogen content of the collected supernatant was assayed by the Kjeldahl method. NSI (in percentage) was then calculated as the nitrogen in the supernatant (g)/total nitrogen in the sample (g) × 100.
In vitro digestibility was assayed, according to the method of Sheng and Zhao (Citation2013), with minor modifications. In the pepsin digestion, the analyzed sample of 0.1 g was dissolved in water of 10 mL, adjusted pH to 2.0, mixed with 2 mg pepsin powder and incubated at 37°C for 2 h. The reaction was stopped by adding 200 g/L trichloroacetic acid solution of 10 mL. After a centrifugation at 10,000×g for 20 min, the collected supernatant was diluted by water of fivefold volume and then measured at 280 nm in the spectrophotometer. In the pepsin–trypsin digestion, the sample was first digested by the added pepsin for 1 h as described above, heated in a boiling water for 5 min, lyophilized, redissolved in 10 mL phosphate buffer (0.2 mol/L, pH 8.0), and further digested by 6 mg trypsin powder at 37°C for another 1 h. The collected supernatant was treated and measured similarly. The measured absorbency values were used to reflect in vitro digestibility of the evaluated samples.
A 1-anilino-8-naphthalene sulfonic acid (ANS) binding method (Hayakawa & Nakai, Citation1985) was used to determine surface hydrophobicity. The dispersion of the analyzed sample of 1.0 g/L (in 0.01 mol/L phosphate buffer, pH 7.0) was centrifuged at 10,000×g for 15 min at room temperature to remove the insoluble particulates. The collected supernatant was measured for protein content and diluted to final concentrations of 0.05–0.5 g/L. ANS solution (8 mmol/L in 0.1 mol/L phosphate buffer, pH 7.0) of 20 μL was added into the diluted solutions of 4 mL and mixed thoroughly. Fluorescence intensities were measured by a fluorescence spectrometer (F4500, Hitachi, Tokyo, Japan) after 15 min reaction. Excitation and emission wavelength were set at 390 and 470 nm, respectively, with a set slits of 5 nm. The slope of the linear regression between the intensities and protein concentrations was used as an index of surface hydrophobicity (H0).
WHC was measured according to the method of Saetae, Kleekayai, Jayasena, and Suntornsuk (Citation2011) with some modifications. Analyzed sample (0.5 g) was mixed thoroughly with some water in pre-weighted centrifuge tubes, kept still at room temperature for 1 h to ensure full hydration, and then centrifuged at 3000×g for 20 min to remove the supernatant. The precipitate was measured for its weight, while the collected supernatant was measured for protein loss by the Kjeldahl method (IDF, Citation2001). The water weight held by the left sample was thus calculated and used to express WHC (kg water /kg protein).
Statistical analyses
All preparation and trials were carried out three times and the data were reported as means ± standard deviations. Differences between the means of multiple groups were analyzed by one-way analysis of variance (ANOVA) with Duncan’s multiple range tests. SPSS 16.0 software (SPSS Inc., Chicago, IL, USA) was used to analyze the data.
Results and discussion
Selected preparation conditions for CHBG composites
When caseinate and HBG were mixed in a ratio of 4:1 (w/w), the impacts of TGase addition, reaction temperature and time on 4-Hyp content of the generated composites were studied. The composites having higher 4-Hyp content indicated that more HBG was conjugated into caseinate. Application of higher levels of three studied conditions was helpful to conjugate more HBG into caseinate (a–c). Based on these conditions used in the reported studies and a consideration of keeping caseinate less self-cross-linked, the three conditions were selected as 10 U/g protein, 45°C and 4 h, respectively. With these conditions, the prepared CHBG composite (4:1) had a 4-Hyp content of (10.30 ± 0.59) g/kg protein. Likewise, the prepared CHBG composite (2:1) and CHBG composite (6:1) had 4-Hyp content of (14.50 ± 0.05) and (6.55 ± 0.63) g/kg protein, respectively. This result indicated that if more HBG was added into reaction system, the generated composite would contain more 4-Hyp, as it was expected.
Figure 1. Impacts of TGase addition (a), reaction temperature (b) and reaction time (c) on 4-Hyp content of the generated caseinate-hydrolyzed gelatin composites. Different lowercase letters on the columns indicate that one-way ANOVA of the mean values is different significantly (p < 0.05).
Figura 1. Impacto de los compuestos gelatinosos hidrolizados con caseína al agregar transglutaminasa en el contenido de 4-hidroxyprolina (a), notándose su temperatura de reacción (b) y el tiempo de reacción (c). Las distintas letras minúsculas en las columnas indican que un anova unidireccional de los valores medios es significativamente diferente (p < 0,05).
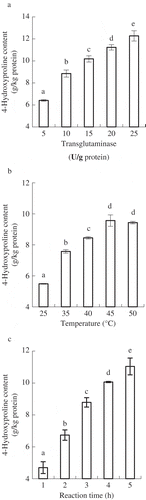
4-Hyp is an abundant and unique imino acid in collagen and gelatin (Hofman, Hall, Cleaver, & Marshall, Citation2011), which enables it a suitable indicator to reflect HBG level in the prepared CHBG composites. 4-Hyp content of the prepared composites was thus used as an index to select suitable conditions. The used TGase addition, reaction temperature and time for the preparation of a caseinate–bovine gelatin composite or a caseinate–glucosamine conjugate were 20 U/g protein, 45°C and 4 h (Zhang et al., Citation2011), or 10 U/g protein, 37°C and 4 h (Jiang & Zhao, Citation2011), respectively. The present study applied the same reaction time and same/similar reaction temperature as these reported studies. To prevent the self-cross-linking of caseinate, a lower TGase addition (10 U/g protein) was used to prepare CHBG composite. Guyot and Kulozik (Citation2011) had pointed out that an increased TGase addition led to an increased cross-linking of caseins. Giosafatto et al. (Citation2012) also gave the same conclusion when ovalbumin was polymerized by TGase.
SDS–PAGE analysis results for the evaluated protein samples are shown in . The band profiles in Lane 4 indicated that bovine gelatin was hydrolyzed, resulting in the formation of the peptides with lower molecular mass. The used TGase-induced caseinate cross-linking (Lane 1 vs. Lane 2), and also conferred the prepared composites (Lanes 5–7) mainly as protein polymers. Most peptide fractions in these composites had molecular mass larger than 116 kDa, and some polymers of much higher molecular mass were observed on the top of stacking gels. Electrophoretic analysis in the past studies also showed that TGase treatment resulted in formation of casein polymers (Ercili Cura et al., Citation2010) or soy protein polymers of higher molecular mass (Tang, Li, & Yang, Citation2006). The present result was consistent to these reported results.
Figure 2. SDS–PAGE profiles of the analyzed protein samples. Lanes 1–7 represent caseinate, cross-linked caseinate, bovine gelatin, HBG and three composites prepared from caseinate and HBG in ratios of 2:1, 4:1 and 6:1 (w/w), respectively. Lane M represents protein markers with molecular mass of 14.4–116.0 kDa.
Figura 2. Perfiles SDS–PAGE de las muestras de proteína analizadas. Las columnas 1 a 7 representan caseína, caseína reticulada, gelatina bovina, gelatina bovina hidrolizada y tres compuestos preparados de caseína y de gelatina bovina hidrolizada en proporciones de 2:1, 4:1 y 6:1 (w/w) respectivamente. La columna M representa marcadores proteicos con masa molecular de 14,4–116,0 kDa.
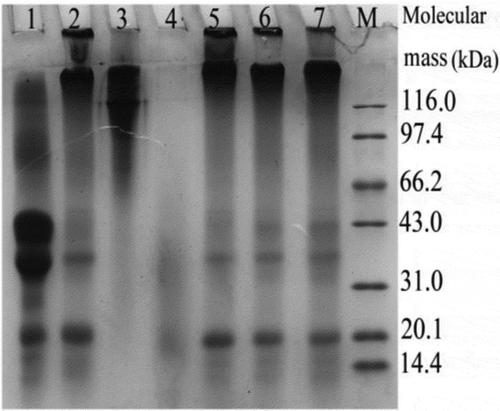
Functional properties of the CHBG composites
The NSI values of the evaluated protein samples at five pH conditions are given in . As it might be expected, caseinate was most insoluble at pH 4.5. The prepared composites were found to be most insoluble at pH 3.0. The solubility of the cross-linked samples was in the order of CHBG composite (2:1), CHBG composite (4:1), CHBG composite (6:1) and cross-linked caseinate in any of the evaluated pH conditions. It meant that conjugation of HBG into caseinate was beneficial to the solubility of the composites. More importantly, HBG amount added in the reaction system showed positive impact on the NSI of the composites, i.e. the prepared composite might have higher NSI when more HBG was used. Bovine gelatin is hydrophilic and soluble in aqueous solutions (Souissi, Bougatef, Triki-Ellouz, & Nasri, Citation2007). Limited enzymatic hydrolysis of bovine gelatin caused a release of smaller peptides and an increased solubility of HBG. When HBG was cross-linked with caseinate by TGase, some hydrophilic groups were induced into the composite, leading to the composite an increased solubility. The widely applied Maillard reaction also can induce the hydrophilic groups of carbohydrates into food proteins. Glycosylation of β-lactoglobulin by aldohexoses (Nacka, Chobert, Burova, Léonil, & Haertlé, Citation1998) or limited glycosylation of whey proteins by dextran (Wang & Ismail, Citation2012) all led to better solubility. These results shared similarity to the present one. Also, formation of isopeptide bonds during cross-linking resulted in the loss of amino groups, shifting maximum insolubility of the composites into lower pH value. Similar findings were observed in other modified proteins. For example, glycosylation of β-lactoglobulin by some monosaccharide or disaccharide shifted its minimum solubility toward more acidic pH (Chevalier, Chobert, Popineau, Nicolas, & Haertlé, Citation2001), and TGase-treated hydrolyzed wheat gluten decreased its isoelectric point from pH 5.5 to pH 4 (Agyare, Xiong, & Addo, Citation2008).
Figure 3. Nitrogen solubility indices of caseinate, cross-linked caseinate and three composites prepared from caseinate and HBG in ratios of 2:1, 4:1 and 6:1 (w/w), respectively, at five evaluated pH conditions. Different lowercase letters on the columns indicate that one-way ANOVA of the mean values is different significantly (p < 0.05).
Figura 3. Índices de solubilidad de nitrógeno de caseína, caseína reticulada y tres compuestos preparados de caseína y de gelatina bovina hidrolizada en proporciones de 2:1, 4:1 y 6:1 (w/w) respectivamente, en cinco condiciones de pH elevado. Las distintas letras minúsculas en las columnas indican que un anova unidireccional de los valores medios es significativamente diferente (p < 0,05).
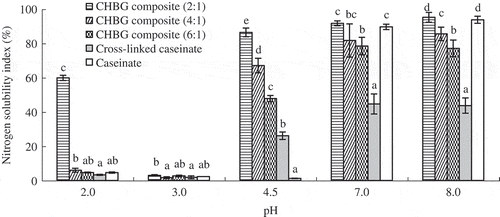
In vitro digestibility of the three composites is shown in . TGase treatment resulted in the composites and cross-linked caseinate impaired in vitro digestibility than caseinate, while incorporation of HBG led to the composites better in vitro digestibility than the cross-linked caseinate. Incorporation of more HBG into the composite would yield a lower in vitro digestibility, as CHBG composite (2:1) exhibited some lower in vitro digestibility than CHBG composite (6:1). It was reported that TGase-induced cross-linking protect the peptide bonds in the proteins (Roos, Lorenzen, Sick, Schrezenmeir, & Schlimme, Citation2003), which gave a negative effect on in vitro digestion. The composites and cross-linked caseinate thus had lower in vitro digestibility than caseinate. Similar findings were found in the case of soy protein isolate (Tang et al., Citation2006), milk proteins (Hiller & Lorenzen, Citation2009) and β-casein (Monogioudi et al., Citation2011) cross-linked by TGase. Trypsin only cleaves the peptide bond on the carboxyl side of arginine and lysine (Kishimura et al., Citation2007). Unlike caseinate, bovine gelatin is poor in the two amino acids (Schrieber & Gareis, Citation2007), thus has less cleaving sites and lower in vitro digestion. It was reported that if DH was used to reflect protein digestibility, gelatin and casein showed DH values of 5.8% and 14.3%, respectively (Rozan et al., Citation1997). This finding suggested that gelatin was less digestible than casein. CHBG composite (6:1) and CHBG composite (2:1) had less and more HBG, thus showed higher and lower in vitro digestibility, respectively.
Figure 4. In vitro digestibility of caseinate, cross-linked caseinate and three composites prepared from caseinate and HBG in ratios of 2:1, 4:1 and 6:1 (w/w), respectively. Different lowercase letters on the columns indicate that one-way ANOVA of the mean values is different significantly (p < 0.05).
Figura 4. Digestibilidad in vitro de caseína, caseína reticulada y de tres compuestos preparados de caseína y de gelatina bovina hidrolizada en proporciones de 2:1, 4:1 y 6:1 (w/w), respectivamente. Las distintas letras minúsculas en las columnas indican que un anova unidireccional de los valores medios es significativamente diferente (p < 0,05).
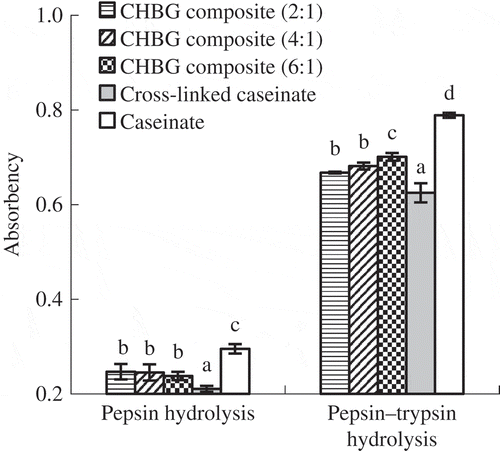
The measured H0 of the composites is listed in . The data showed that the composites had higher H0 values than caseinate, but lower H0 values than cross-linked caseinate (p < 0.05). Among the composites prepared, CHBG composite (6:1) was generated from caseinate and HBG in a ratio of 6:1 (w/w), i.e. more caseinate was used, and thus had the highest H0 value; on the contrary, CHBG composite (2:1) was prepared with less caseinate, and thus had the lowest H0 value. In a study of Hiller and Lorenzen (Citation2009), the H0 values of TGase-treated caseinate, whey protein isolate or total milk protein were enhanced by 2%, 81% and 19%, respectively. This finding supported that the composites and cross-linked caseinate had higher H0 values than caseinate. Due to hydrophilic nature of gelatin (Souissi et al., Citation2007) and its degraded products (e.g. HBG), the H0 values of the composites were thus lower than that of the cross-linked caseinate, especially when more HBG was used in composite preparation. Therefore, CHBG composite (2:1) gave a lower H0 value than CHBG composite (6:1).
Table 1. H0 and WHC of the caseinate, cross-linked caseinate and three composites.
Tabla 1. Hidrofobicidad superficial (H0) y capacidad de retención hídrica (WHC) de la caseína, de la caseína reticulada y de los tres compuestos.
The present study also showed that the cross-linked samples were better in WHC than caseinate (). The hydrated composites and cross-linked caseinate were all found in a gel-like state. Unfortunately, conjugation of HBG into the composites showed some adverse impact on the measured WHC (), especially when the composite contained HBG of higher level. For example, CHBG composite (2:1) had a WHC value of 5.96 kg/kg protein, while CHBG composite (6:1) had a WHC value of 17.30 kg/kg protein. It was reported that cross-linking of skimmed milk proteins (Imm, Lian, & Lee, Citation2000), whey protein concentrate (Soeda, Hokazono, Kasagi, & Sakamoto, Citation2006) and sodium caseinate (Lorenzen, Citation2000) yielded significant increase in WHC. These results supported the cross-linked caseinate and the composites had higher WHC than caseinate. Our previous result (Zhang et al., Citation2011) also showed that a caseinate–gelatin composite had much higher WHC than the cross-linked caseinate. Gelatin conjugation was contributed to the WHC enhancement as gelatin has better swelling and water binding ability (Gómez-Guillén, Giménez, López-Caballero, & Montero, Citation2011). Enzymatic hydrolysis of bovine gelatin broke the peptide chain and thus impaired the swelling and water binding ability of hydrolyzed gelatin. HBG conjugation in the composites thus gave an unfavorable effect on WHC, unlike gelatin conjugation did. CHBG composite (2:1) contained more HBG and thus showed a lower WHC than the cross-linked caseinate. On the contrary, CHBG composite (6:1) contained less HBG and thereof had a WHC similar to the cross-linked caseinate. CHBG composite (6:1) might be a potential ingredient in the production of some processed foods, such as ham, fish ball and others.
Conclusion
CHBG composites could be generated from caseinate and HBG in three blending ratios by TGase. The blending ratios of HBG used had impact on the properties of the composites. The three composites prepared had better solubility in pH 4.5 but also showed lower or similar solubility in pH 7–8 than caseinate. Totally, the composites had lower in vitro digestibility but higher H0 and WHC than caseinate. More importantly, higher level of HBG used in composite preparation was helpful to solubility but adverse to in vitro digestibility and WHC. The composite containing less HBG might be a potential ingredient of better WHC for processed foods.
Acknowledgments
This study was funded by the Innovative Research Team of Higher Education of Heilongjiang Province (No. 2010td11) and the National High Technology Research and Development Program (“863” Program) of China (Project No. 2013AA102205). The authors thank the anonymous reviewers and editors for their valuable advices.
References
- Adler-Nissen, J. (1979). Determination of the degree of hydrolysis of food protein hydrolysates by trinitrobenzenesulfonic acid. Journal of Agricultural and Food Chemistry, 27, 1256–1262. doi:10.1021/jf60226a042
- Agyare, K. K., Xiong, Y. L., & Addo, K. (2008). Influence of salt and pH on the solubility and structural characteristics of transglutaminase-treated wheat gluten hydrolysate. Food Chemistry, 107, 1131–1137. doi:10.1016/j.foodchem.2007.09.039
- Ando, H., Adachi, M., Umeda, K., Matsuura, A., Nonaka, M., Uchio, R. … Motoki, M. (1989). Purification and characteristics of a novel transglutaminase derived from microorganisms. Agricultural and Biological Chemistry, 53, 2613–2617. doi:10.1271/bbb1961.53.2613
- Avramenko, N. A., Low, N. H., & Nickerson, M. T. (2013). The effects of limited enzymatic hydrolysis on the physicochemical and emulsifying properties of a lentil protein isolate. Food Research International, 51, 162–169. doi:10.1016/j.foodres.2012.11.020
- Babiker, E. F. E., Khan, M. A. S., Matsudomi, N., & Kato, A. (1996). Polymerization of soy protein digests by microbial transglutaminase for improvement of the functional properties. Food Research International, 29, 627–634. doi:10.1016/S0963-9969(96)00069-5
- Bera, M. B., & Mukherjee, R. K. (1989). Solubility, emulsifying, and foaming properties of rice bran protein concentrates. Journal of Food Science, 54, 142–145. doi:10.1111/j.1365-2621.1989.tb08587.x
- Chambi, H., & Grosso, C. (2006). Edible films produced with gelatin and casein cross-linked with transglutaminase. Food Research International, 39, 458–466. doi:10.1016/j.foodres.2005.09.009
- Chevalier, F., Chobert, J. M., Popineau, Y., Nicolas, M. G., & Haertlé, T. (2001). Improvement of functional properties of β-lactoglobulin glycated through the Maillard reaction is related to the nature of the sugar. International Dairy Journal, 11, 145–152. doi:10.1016/S0958-6946(01)00040-1
- Church, F. C., Swaisgood, H. E., Porter, D. H., & Catignani, G. L. (1983). Spectrophotometric assay using o-phthaldialdehyde for determination of proteolysis in milk and isolated milk proteins. Journal of Dairy Science, 66, 1219–1227. doi:10.3168/jds.S0022-0302(83)81926-2
- Damodaran, S., & Agyare, K. K. (2013). Effect of microbial transglutaminase treatment on thermal stability and pH-solubility of heat-shocked whey protein isolate. Food Hydrocolloids, 30, 12–18. doi:10.1016/j.foodhyd.2012.04.012
- De Jong, G. A. H., & Koppelman, S. J. (2002). Transglutaminase catalyzed reactions: Impact on food applications. Journal of Food Science, 67, 2798–2806. doi:10.1111/j.1365-2621.2002.tb08819.x
- Di Pierro, P., Chico, B., Villalonga, R., Mariniello, L., Damiao, A. E., Masi, P., & Porta, R. (2006). Chitosan−Whey protein edible films produced in the absence or presence of transglutaminase: Analysis of their mechanical and barrier properties. Biomacromolecules, 7, 744–749. doi:10.1021/bm050661u
- Domagała, J., Wszołek, M., Tamime, A. Y., & Kupiec-Teahan, B. (2013). The effect of transglutaminase concentration on the texture, syneresis and microstructure of set-type goat’s milk yoghurt during the storage period. Small Ruminant Research, 112, 154–161. doi:10.1016/j.smallrumres.2012.12.003
- Ercili Cura, D., Lille, M., Partanen, R., Kruus, K., Buchert, J., & Lantto, R. (2010). Effect of Trichoderma reesei tyrosinase on rheology and microstructure of acidified milk gels. International Dairy Journal, 20, 830–837. doi:10.1016/j.idairyj.2010.06.008
- Giosafatto, C. V. L., Rigby, N. M., Wellner, N., Ridout, M., Husband, F., & Mackie, A. R. (2012). Microbial transglutaminase-mediated modification of ovalbumin. Food Hydrocolloids, 26, 261–267. doi:10.1016/j.foodhyd.2011.06.003
- Gómez-Guillén, M. C., Giménez, B., López-Caballero, M. E., & Montero, M. P. (2011). Functional and bioactive properties of collagen and gelatin from alternative sources: A review. Food Hydrocolloids, 25, 1813–1827. doi:10.1016/j.foodhyd.2011.02.007
- Guyot, C., & Kulozik, U. (2011). Effect of transglutaminase-treated milk powders on the properties of skim milk yoghurt. International Dairy Journal, 21, 628–635. doi:10.1016/j.idairyj.2010.10.010
- Hayakawa, S., & Nakai, S. (1985). Relationships of hydrophobicity and net charge to the solubility of milk and soy proteins. Journal of Food Science, 50, 486–491. doi:10.1111/j.1365-2621.1985.tb13433.x
- Hiller, B., & Lorenzen, P. C. (2009). Functional properties of milk proteins as affected by enzymatic oligomerisation. Food Research International, 42, 899–908. doi:10.1016/j.foodres.2009.04.022
- Hofman, K., Hall, B., Cleaver, H., & Marshall, S. (2011). High-throughput quantification of hydroxyproline for determination of collagen. Analytical Biochemistry, 417, 289–291. doi:10.1016/j.ab.2011.06.019
- IDF. (2001). Milk-determination of nitrogen content-part 1: Kjeldahl method. IDF Standard 20-1. Brussels: International Dairy Federation.
- Imm, J. Y., Lian, P., & Lee, C. M. (2000). Gelation and water binding properties of transglutaminase-treated skim milk powder. Journal of Food Science, 65, 200–205. doi:10.1111/j.1365-2621.2000.tb15979.x
- Jiang, S. J., & Zhao, X. H. (2011). Transglutaminase-induced cross-linking and glucosamine conjugation of casein and some functional properties of the modified product. International Dairy Journal, 21, 198–205. doi:10.1016/j.idairyj.2010.12.004
- Kishimura, H., Tokuda, Y., Yabe, M., Klomklao, S., Benjakul, S., & Ando, S. (2007). Trypsins from the pyloric ceca of jacopever (Sebastes schlegelii) and elkhorn sculpin (Alcichthys alcicornis): Isolation and characterization. Food Chemistry, 100, 1490–1495. doi:10.1016/j.foodchem.2005.11.040
- Laemmli, U. K. (1970). Cleavage of structural proteins during the assembly of the head of bacteriophage T4. Nature, 227, 680–685. doi:10.1038/227680a0
- Lim, L. T., Mine, Y., & Tung, M. A. (1999). Barrier and tensile properties of transglutaminase cross-linked gelatin films as affected by relative humidity, temperature, and glycerol content. Journal of Food Science, 64, 616–622. doi:10.1111/j.1365-2621.1999.tb15096.x
- Liu, M. X., & Damodaran, S. (1999). Effect of transglutaminase-catalyzed polymerization of β-casein on its emulsifying properties. Journal of Agricultural and Food Chemistry, 47, 1514–1519. doi:10.1021/jf981030c
- Lorenzen, P. C. (2000). Techno-functional properties of transglutaminase-treated milk proteins. Milchwissenschaft, 55, 667–670.
- Monogioudi, E., Faccio, G., Lille, M., Poutanen, K., Buchert, J., & Mattinen, M. L. (2011). Effect of enzymatic cross-linking of β-casein on proteolysis by pepsin. Food Hydrocolloids, 25, 71–81. doi:10.1016/j.foodhyd.2010.05.007
- Nacka, F., Chobert, J. M., Burova, T., Léonil, J., & Haertlé, T. (1998). Induction of new physicochemical and functional properties by the glycosylation of whey proteins. Journal of Protein Chemistry, 17, 495–503. doi:10.1023/A:1022530904218
- Papon, P., Leblond, J., & Meijer, P. H. E. (2006). Gelation and transitions in biopolymers. In P. Papon, J. Leblond, & P. H. E. Meijer (Eds.), The physics of phase transitions. Berlin: Springer.
- Roos, N., Lorenzen, P. C., Sick, H., Schrezenmeir, J., & Schlimme, E. (2003). Cross-linking by transglutaminase changes neither the in vitro proteolysis nor the in vivo digestibility of caseinate. Kieler Milchwirtschaftliche Forschungsberichte, 55, 261–276.
- Rozan, P., Lamghari, R., Linder, M., Villaume, C., Fanni, J., Parmentier, M., & Méjean, L. (1997). In vivo and in vitro digestibility of soybean, lupine, and rapeseed meal proteins after various technological processes. Journal of Agricultural and Food Chemistry, 45, 1762–1769. doi:10.1021/jf960723v
- Saetae, D., Kleekayai, T., Jayasena, V., & Suntornsuk, W. (2011). Functional properties of protein isolate obtained from physic nut (Jatropha curcas L.) seed cake. Food Science and Biotechnology, 20, 29–37. doi:10.1007/s10068-011-0005-x
- Sarabia, A. I., Gómez-Guillén, M. C., & Montero, P. (2000). The effect of added salts on the viscoelastic properties of fish skin gelatin. Food Chemistry, 70, 71–76. doi:10.1016/S0308-8146(00)00073-X
- Schrieber, R., & Gareis, H. (2007). Gelatin handbook: Theory and industrial practice. New York, NY: Wiley-VCH Verlag GmbH & Co. KGaA.
- Sheng, W. W., & Zhao, X. H. (2013). Functional properties of limited hydrolysed cross-linked casein–gelatin composites. International Journal of Food Science and Technology, 48, 260–266. doi:10.1111/j.1365-2621.2012.03182.x
- Soeda, T., Hokazono, A., Kasagi, T., & Sakamoto, M. (2006). Improvement of functional properties of WPC by microbial transglutaminase. Journal of the Japanese Society for Food Science and Technology, 53, 74–79. doi:10.3136/nskkk.53.74
- Souissi, N., Bougatef, A., Triki-Ellouz, Y., & Nasri, M. (2007). Biochemical and functional properties of sardinella (Sardinella aurita) by-product hydrolysates. Food Technology and Biotechnology, 45, 187–194.
- Syk, I., Ågren, M. S., Adawi, D., & Jeppsson, B. (2001). Inhibition of matrix metalloproteinases enhances breaking strength of colonic anastomoses in an experimental model. British Journal of Surgery, 88, 228–234. doi:10.1046/j.1365-2168.2001.01649.x
- Tang, C. H., Li, L., & Yang, X. Q. (2006). Influence of transglutaminase-induced cross-linking on in vitro digestibility of soy protein isolate. Journal of Food Biochemistry, 30, 718–731. doi:10.1111/j.1745-4514.2006.00092.x
- Wang, Q., & Ismail, B. (2012). Effect of Maillard-induced glycosylation on the nutritional quality, solubility, thermal stability and molecular configuration of whey protein. International Dairy Journal, 25, 112–122. doi:10.1016/j.idairyj.2012.02.009
- Zhang, Y. N., Liu, N., & Zhao, X. H. (2011). A study on the preparation and some functional properties of a cross-linked casein–gelatin composite by a microbial transglutaminase. International Journal of Food Science and Technology, 46, 2641–2647. doi:10.1111/j.1365-2621.2011.02795.x
- Zhang, Y. N., & Zhao, X. H. (2013). Study on the functional properties of soybean protein isolate cross-linked with gelatin by microbial transglutaminase. International Journal of Food Properties, 16, 1257–1270. doi:10.1080/10942912.2011.583705