Abstract
This research was conducted to develop and optimize a methodology to quantify the content of secoisolariciresinol diglucoside (SDG), the main vegetable lignan present in flaxseed. However, at the time of this work, commercial SDG was not available for standard use. Therefore, an effective method to obtain purified SDG from flaxseed was developed using chromatographic techniques. The SDG obtained was of approximately 97% purity. The methanol extraction time, alkali concentrations, and reaction temperature were optimized through a response surface methodology (RSM), and the optimal conditions established for the extractions were 47°C, 58 mmol L−1 sodium methoxide, and 24 h. This methodology was then applied to study the variation of SDG content in flaxseed from different cultivars. The SDG content in different varieties of flaxseed ranged from 10.8 to 17.9 mg g−1 in defatted flaxseed flour and from 6.0 to 10.9 mg g−1 in whole flaxseed.
El objetivo de esta investigación fue desarrollar y optimizar una metodología para cuantificar el contenido de secoisolariciresinol diglucósido (SDG), el cual es el principal lignano vegetal presente en linaza. Sin embargo, al realizar este trabajo no existía SDG comercial para ser utilizado como estándar externo. Por lo tanto, se desarrolló un método efectivo para obtener SDG purificado usando técnicas cromatográficas. El SDG obtenido fue de 97% de pureza. Se optimizó el tiempo de extracción metanólica, concentración de alcali y temperatura de reacción a través de un diseño de superficie respuesta. Las condiciones óptimas establecidas fueron: 47°C, 58 mmol L−1 de metóxido de sodio y 24 h. Esta metodología luego fue aplicada para estudiar la variación del contenido de SDG en linaza de diferentes cultivares. El contenido de SDG en diferentes variedades de linaza se encontró en el rango de 10,8 a 17,9 mg g−1 en harina desgrasada de linaza y de 6,0 a 10,9 mg g−1 en linaza entera.
Palabras claves:
Introduction
Flaxseed (Linum usitatissimum L.) is an important source of both insoluble and soluble fiber and is highly rich in polyunsaturated fatty acids, especially α-linolenic acid (Muir & Wescott, Citation2003). In addition, flaxseed is also known for its high content of lignans, a plant secondary metabolite, particularly secoisolariciresinol diglucoside (SDG), compared to those found in other grains, legumes, fruits, and vegetables (Meagher & Beecher, Citation2000; Thompson, Boucher, Liu, Cotterchio, & Kreiger, Citation2006). Carob seed flour has high contents of isolariciresinol and pinoresinol (Durazzo et al., Citation2014); isolariciresinol is the predominant lignan in pomegranate (Fischer, Jaksch, Carle, & Kammerer, Citation2012) and mataisolariciresinol is the main lignan in cereals like wheat, rye (Hosseinian & Mazza, Citation2009), and legumes (Konar, Citation2013). Also, the vegetables green bean, carrot, cauliflower, white cabbage, iceberg lettuce, and artichoke have important contents of secoisolariciresinol (Konar, Poyrazoğlu, Demir,&Artik, Citation2012).
SDG is an esterified ester polymer that is composed of 3-hydroxy-3-methyl glutaric acid (HMGA) and other phenolic compounds such as glycosides of p-coumaric acid and ferulic acid (Johnsson et al., Citation2002; Kamal-Eldin et al., Citation2001; Struijs, Vincken, Doeswijk, Voragen, & Gruppen, Citation2009). Their formation occurs in the outer layer of the seed; therefore, the greatest concentration of SDG is found in the hulls of flaxseeds (Hano et al., Citation2006). In addition to being esterified into an oligomer, SDG is complexed with insoluble fiber, gums, polysaccharides, and mucilage associated with the hull (Thompson, Robb, Serraino, & Cheung, Citation1991). SDG is the main precursor of the mammalian lignans enterodiol (ED) and enterolactone (EL), which are formed by intestinal mammalian bacteria (Clavel, Borrmann, Braune, Dore, & Blaut, Citation2006; Eeckhaut et al., Citation2008; Wang, Meselhy, Li, Qin, & Hattori, Citation2000). The enterolignans ED and EL have phytoestrogenic effects due to their similarity to 17β-estradiol, which regulates sex-specific functions, and may be involved in preventing some cancers (Muir & Wescott, Citation2003). SDG and its metabolites are known for their potential benefits to human health because they act as antioxidants (Hu, Yuan, & Kitts, Citation2007; Kitts, Yuan, Wijewickreme, & Thompson, Citation1999), reducing serum cholesterol levels and inhibiting the development of type I and II diabetes (Prasad, Citation2000; Zhang et al., Citation2008), and it has been proven that lignans can reduce the incidence of breast cancer and prostate cancer through modulation of the synthesis, bioavailability, and activity of steroid hormones by binding to low levels of estrogen receptors (Muir & Wescott, Citation2003; Touillaud et al., Citation2007).
In a first instance, for the extraction of lignans from flaxseed, an alcoholic extraction and alkaline hydrolysis are usually used to release SDG from its oligomer. shows the extraction methods used by several authors showing that mainly are differentiated according to the solvent, temperature, and time of extraction conditions. Hydrolysis of ester and glycosidic bonds is performed to simplify the chromatographic analysis of the SDG–oligomer-containing extracts. On the one hand, strong acid hydrolysis breaks both bonds, resulting in addition in a destruction of some lignans and cause transformation in other lignans; on the other hand, alkaline hydrolysis breaks only the ester bonds (Smeds et al., Citation2007). On this basis, Chen, Liu, Shi, and Ma (Citation2007) developed a high performance liquid chromatography (HPLC) method with ultraviolet (UV) detection for the analysis of SDG in flaxseed, which involves extraction from defatted flaxseed flour (DFF) with methanol and basic hydrolysis with aqueous sodium hydroxide. To simplify this extraction methodology, Eliasson, Kamal-Eldin, Andersson, and Åman (Citation2003) eliminated the alcohol extraction step by using aqueous alkaline hydrolysis directly, resulting in a higher yield than that obtained by the hydrolysis of alcoholic extracts (Johnsson, Kamal-Eldin, Lundgren, & Åman, Citation2000). Other technique includes the use of supercritical CO2 for lignan extraction from flaxseed which results in lower SDG extraction compared to the traditional solvent extractions (Comin, Temelli, & Saldaña, Citation2011). However, supercritical water is suitable for SDG extraction from flaxseed (Kanmaz & Ova, Citation2013).
Table 1. Flaxseed lignan extraction methods.
Tabla 1. Métodos de extracción de lignanos de linaza.
To determine the conditions that optimize an extraction of SDG from flaxseed, response surface methodology (RSM) is a useful tool. Zhang et al. (Citation2007) optimized with RSM the ethanol–water extraction of lignans from flaxseed using ethanol concentration, temperature, and extraction time as factors. RSM allows determining the levels of each factor and their interactions that result in maximum yield (Myers, Montgomery, & Anderson-Cook, Citation2009).
To quantify SDG, many authors have purified SDG by using an external standard because this compound was not available at the time of analysis. Johnsson et al. (Citation2000) have purified SDG from flaxseed using chromatographic methods. Starting with an extraction with 1,4-dioxane/ethanol and alkaline hydrolysis, they were able to purify SDG by reverse-phase chromatography using silica gel, and they were finally able to confirm the identity of the compound by nuclear magnetic resonance (NMR). This same technique was used by other authors, who conducted similar studies for the quantification of SDG (Eliasson et al., Citation2003). Afterward, Li et al. (Citation2008) and Yuan, Li, Xu, Wang, and Liu (Citation2008) purified SDG with chromatographic techniques using solid-phase extraction (SPE), silica gel columns, and thin-layer chromatography prior to the extraction with methanol. An effective method has also been developed for the purification of SDG from flaxseed, which includes a series of extractions, involving microwave and ion-exchange chromatography and reverse-phase SDG, and produces over 95% purity (Stasevich, Mikhalenok, & Kurchenko, Citation2009). By contrast, Bravi, Perretti, Marconi, Patrizi, and Fantozzi (Citation2011) used the aglycone secoisolariciresinol (SECO) as a SDG standard, considering it as an equivalent compound for the calibration curve and subsequent quantification in a study of lignan determination in flaxseed oil.
Currently, there are patented purification techniques, among them Westcott and Muir (Citation1998) created a process of lignan SDG extraction from defatted flaxseed, which has also been performed by other researchers (Beejmohun et al., Citation2007; Zhang et al., Citation2007). This method uses methanol extraction, alkaline hydrolysis, anion exchange chromatography, and finally separation by reverse-phase HPLC and yields SDG in more than 90% purity. Thus, a process for isolating and purifying SDG extractions, mainly using supercritical CO2 and chromatographic separation, was also patented (Pihlava, Hyvarinen, Ryhanen, & Hietantemi, Citation2004). The methods most commonly used for lignan determination in flaxseed are based on HPLC coupled with different detector systems such as UV (Herchi et al., Citation2014).
The aim of this work was to develop and optimize a lignan extraction from flaxseed for the quantification of SDG in different flaxseed cultivars by HPLC/UV. To develop the methodology, the methanol extraction, alkali concentrations, reaction temperature, and chromatographic conditions were optimized by RSM. At the time of this work, no commercial standard was available for SDG. Therefore, it was necessary to purify SDG from flaxseed to be used for quantification of SDG by HPLC.
Materials and methods
Materials
Flaxseeds for extraction, optimization, and purification of SDG were of the Celestina variety, year 2007, and obtained from Seeds Baer SA (Araucanía Region, Chile). The flax varieties used to study the contents of SDG consisted of two Chilean varieties (Celestina and Carmine) and two cultivars from Canada (Bethune and Sorrel), all obtained in 2008. Subsequently, the same varieties were harvested in 2009 from two locations: Cajón and Gorbea, both located in La Araucania, Chile. Samples were kept refrigerated at 4°C before being analyzed by HPLC.
All reagents were of analytical and HPLC grade and were purchased from Merck (Darmstadt, Germany). SECO standard and the enzyme β-glucosidase from almonds (5.2 units mg−1 solid) were purchased from Sigma-Aldrich (Saint Louis, MO, USA).
Purification of SDG
SDG extraction
Flaxseeds were ground in a coffee grinder to obtain fine flour. DFF was prepared by the Soxhlet method for 2 h at 70°C with n-hexane, as described by Johnsson et al. (Citation2000) with some modifications. DFF (5 g) was mixed with 100 mL of 0.1 mmol L−1 sodium methoxide and sonicated for 30 min. The mixture was incubated at 60°C for 24 h with agitation (100 rpm). The solution was filtered through Whatman paper Nº1 to remove the solid waste and then neutralized with 12 mmol L−1 HCl. Twenty milliliters of 0.1 mmol L−1 sodium acetate at pH 5.0 was added, and the mixture was centrifuged for 15 min at 4200 g, after which the supernatant was filtered. The solvent was evaporated at 40°C, and 60 mL of 0.1 mmol L−1 sodium acetate at pH 5.0 was added to the resulting aqueous extract. The solution was centrifuged again for 15 min at 4200 g and filtered. The lignans were cleaned by SPE using 6 mL Sep-Pack® Vac C18 cartridges (500 mg capacity) (Waters, Milford, MA, USA). The columns were activated by successive washing with methanol and deionized water. Then, 5 mL of sample was loaded and eluted with 2 mL of methanol. The procedure was performed in duplicate, after which 4 mL of lignan extract (containing SDG) was obtained and subsequently purified by HPLC.
Purification by HPLC
A pump model constaMetric 3200, a UV-Visible detector model SM4000 (LDC Analytical, Riviera Beach, FL, USA) and a manual injector model 7161 (Rheodyne, Oak Harbor, WA, USA) were used. Separation was achieved on a Luna C8 column (250 × 4.6 mm id, 5 µm particle size; Phenomenex, Torrance, CA, USA) operated at 20°C. The detection was made at a wavelength of 280 nm. The chromatograms were analyzed using Clarity software®, and the injection volume was 100 µL. The mobile phase consisted of 1% aqueous acetic acid/acetonitrile (85:15 v/v) with a flow rate of 1 mL min−1 (Chen et al., Citation2007; Chimichi, Bambagiotti-Alberti, Coran, Giannellini, & Biddau, Citation1999). A fraction collector model 2212 Helirac (LKB Bromma, Sweden) was used, which fractionates every 30 s. The process was repeated 20 times to obtain approximately 9 mL in each fraction. The fractions, corresponding to the peaks observed at 280 nm, were measured in a T70 UV/Vis spectrophotometer (Pharma Test, Hainburg, Germany) and were concentrated by Sep-Pack® SPE C18 cartridges, Vac 6 cc (500 mg) (Waters, IADET, Santiago, Chile), following the same procedure described above. Samples were eluted with 2 mL of methanol. The solvent was evaporated to dryness at 40°C in a forced-air oven model ZRD-A-5030 (Zhicheng®) to gravimetrically obtain the amount of solids in the sample.
Enzymatic confirmation
One milliliter of 0.1 mmol L−1 sodium acetate at pH 5.0 and 0.25 mg of β-glucosidase enzyme was added to the dried fractions corresponding to each of the peaks detected. Enzymatic hydrolysis was performed at 40°C for 12 h, according to Renouard et al. (Citation2010), with modifications. β-glucosidase enzyme is efficient to remove the glycosyls groups from the SDG and release the aglycone (SECO). All fractions were centrifuged at 4200 g for 5 min, and the supernatants were then separated by Sep-Pack® SPE C18 cartridges, Vac 6 cc (500 mg) (Waters), following the same methodology described above. The hydrolyzed fractions were analyzed by HPLC and compared with the standard of SECO, and the chromatographic conditions included a Luna C8 column, 250 × 4.6 mm id, and 5 µm particle size (Phenomenex). The mobile phase consisted of 1% aqueous acetic acid/acetonitrile (65:35 v/v) with a flow rate of 1 mL min−1 and detected at 280 nm. Therefore, the SECO-hydrolyzed fraction identified corresponds to the fraction of SDG.
Optimization and quantification of SDG from flaxseed
To optimize the SDG extraction from flaxseed, a response surface methodology through the Box–Behnken design was developed. The extraction method to assess the concentration of sodium methoxide (50 and 150 mmol L−1), the reaction times (12 and 24 h), and the temperature (40 and 60°C) were used, which involved 15 experimental runs including three central points. The optimal condition of extraction for quantification of SDG in flaxseed cultivars was used. The procedure was performed in triplicate, and samples were kept refrigerated at 4°C before being analyzed by HPLC. For HPLC measurements, the same equipment described above was used. The injection volume was 20 µL, and a Luna C8 column with 250 × 4.6 mm id and a 5 µm particle size (Phenomenex) was used at 20°C. The mobile phase consisted of 1% aqueous acetic acid/acetonitrile (85:15 v/v) with a flow rate of 1 mL min−1.
Validation of the analytical methodology
Optimal conditions of lignan extraction from flaxseed were obtained using the second-order polynomial model of RSM. The experimental and predicted SDG contents were compared in order to determine the validity of the model.
To ensure the reliability of the results, the limit of detection (LOD) and limit of quantification (LOQ) for SDG were determined by performing five calibration curves with five concentration levels. The accuracy and precision were evaluated by spiking the standard and by calculating the coefficient of variation (CV), respectively.
Statistical analysis
Multifactorial analysis of variance (ANOVA) to determine statistically significant differences (p < 0.05) between cultivars (Bethune, Carmine, Celestina, and Sorrel), between the crop years (2008 and 2009), and between the location of cultivation (Cajón and Gorbea) was performed. The statistical analyses and RSM were performed using Statgraphics Centurion XV (StatPoint, Rockville, MD, USA).
Results and discussion
Purification of SDG
The release of the SDG molecule from the oligomer by an alkaline hydrolysis using methanol as a solvent was performed. Using this methanolic base hydrolysis method to hydrolyze, the ester bond between SDG and HMGA was achievable. With this technique, the union in a single step of the alcoholic extraction and aqueous base hydrolysis was possible, thus shortening the entire process of lignan extraction. After the methanolic base hydrolysis, the extract was neutralized using 12 mmol L−1 HCl to prevent the ionization of carboxylic and phenolic groups. The methanol extract was centrifuged in order to precipitate high-molecular-weight molecules, such as cellulose and gums present in flaxseed, permitting working with a supernatant composed by lignan fractions. The solvent was evaporated and then reconstituted in an aqueous solution at pH 5.0, and the aqueous extract was subsequently centrifuged and filtered again.
SPE to separate the lignans from other water-soluble compounds, including some proteins that may interfere with HPLC analysis, was performed. In this HPLC methodology, a C8 column instead of a C18 column was used, but almost the same retention time was obtained using the same mobile phase as used by Chen et al. (Citation2007). shows a chromatogram of the lignan extract, and one of the peaks observed corresponds to SDG. To identify and purify SDG, each peak was collected. The fractions were subjected to enzymatic hydrolysis with β-glucosidase to produce SECO from SDG () and then compared with the external SECO standard. shows a comparison of the peak retention time of 9.6 min from the enzymatic hydrolysis with the respective SECO standard, thus verifying that the 9.6 min peak from the corresponding lignan extract corresponds to SDG. The SDG obtained was approximately of 97% purity (). Once the purified compound was obtained, SPE to change the solvent in which it was dissolved (mobile phase) and to leave only the metabolite dissolved in methanol was performed. Then, it was possible to evaporate the solvent at 40°C and gravimetrically determine the amount of SDG standard. Approximately, 10 mg of the compound was obtained, which was then dissolved in methanol at a concentration of 1 mg mL−1.
Figure 1. HPLC chromatogram of lignan SDG and other compounds extracted from flaxseed recorded at 280 nm. The injection volume was 100 µL and the mobile phase consisted of 1% aqueous acetic acid/acetonitrile (85:15 v/v) with a flow rate of 1 mL min−1.
Figura 1. Cromatograma HPLC del lignano SDG y otros compuestos extraídos desde linaza registrado a 280 nm. El volumen de inyección fue 100 µL y la fase móvil consistió de 1% de ácido acético acuoso/acetonitrilo (85:15 v/v) con flujo de 1 mL min−1.
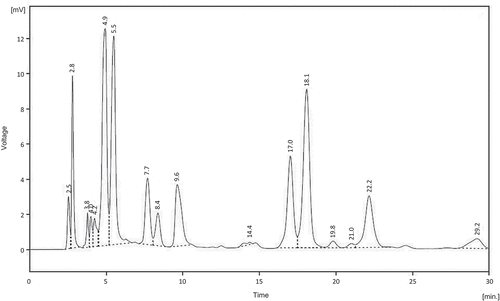
Figure 2. Enzymatic hydrolysis with β-glucosidase to produce SECO from SDG.
Figura 2. Hidrolisis enzimatica con β-glucosidasa para producir SECO desde SDG.
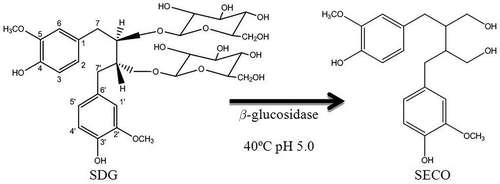
Figure 3. HPLC chromatogram for the enzymatic test recorded at 280 nm. The injection volume was 20 µL and the mobile phase consisted of 1% aqueous acetic acid/acetonitrile (65:35 v/v) with a flow rate of 1 mL min−1.
Figura 3. Cromatograma HPLC para la confirmación enzimática registrado a 280 nm. El volumen de inyección fue 20 µL y la fase móvil consistió de 1% de ácido acético acuoso/acetonitrilo (65:35 v/v) con flujo de 1 mL min−1.
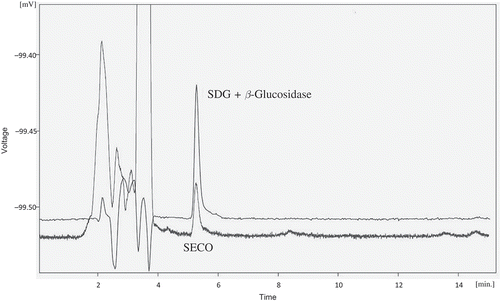
Figure 4. HPLC chromatogram of lignan SDG purified by collecting fractions, recorded at 280 nm. The injection volume was 20 µL and the mobile phase consisted of 1% aqueous acetic acid/acetonitrile (85:15 v/v) with a flow rate of 1 mL min−1.
Figura 4. Cromatograma HPLC del lignano SDG purificado a través de recolector de fracciones registrado a 280 nm. El volumen de inyección fue 20 µL y la fase móvil consistió de 1% de ácido acético acuoso/acetonitrilo (85:15 v/v) con flujo de 1 mL min−1.
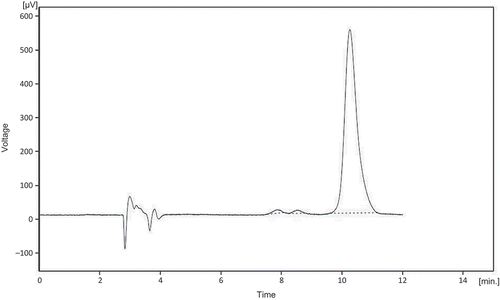
Optimization of SDG extraction from flaxseed by RSM
A Box–Behnken design was performed to determine the optimal conditions of temperature (X1), sodium methoxide concentration (X2), and time (X3) for the SDG extraction from flaxseed. The values of the three independent variables and SDG yield (Y) are shown in . A second-order polynomial equation for SDG was obtained as follows:
Table 2. Box–Behnken design and its results.
Tabla 2. Diseño Box–Behnken y sus resultados.
The results of the ANOVA for the fitted model are summarized in . The adjusted model has a coefficient of correlation (R2 = 0.862). Since the p-value of the Durbin–Watson test was not significant (p > 0.05), there is no indication of autocorrelation in the residuals at the 5% significant level. The non-significant lack of fit indicates that the model is adequate for the observed data at 95% confidence level. The ANOVA indicated that the concentration of sodium methoxide has a statistically significant effect (p < 0.05) on the SDG yield, in addition with the quadratic term of temperature (p < 0.05). The predicted model, through the estimated response surface (), was used to obtain the maximum SDG, which features the following conditions as optimal for the extraction process: 47°ºC, 58 mmol L−1 sodium methoxide, and 24 h. At this point, 9.4 mg of SDG per gram of whole flaxseed was obtained as predicted value.
Table 3. Analysis of variance for the fitted model.
Tabla 3. Análisis de varianza para el modelo ajustado.
Validation of the methodology
The suitability of the predicted model equation for the optimum SDG yield was tested. The experimental conditions were evaluated in the same variety in which the optimization was made (Celestina), i.e. a comparison of SDG experimental values from the predicted ones of Celestina harvested in 2008 and 2009 (Cajón and Gorbea) was performed. The SDG contents were 7.64 ± 0.51, 10.91 ± 0.72, and 8.83 ± 0.60 mg g−1 whole flaxseed, respectively. The experimental values were found to be close to the predicted one (9.4 mg g−1 whole flaxseed).
The accuracy of the method by calculating the CV in three replicates of each variety of flaxseed studied was verified. The CV was between 0.7% and 6.7%. The accuracy of the method was measured by adding a known amount of SDG standard to the samples, and the average recovery rate was 104.5% ± 8.8%. The LOD of the method was 1.8 µg g−1 and the LOQ was 5.4 µg g−1.
SDG content in flaxseed cultivars
The method for the determination of SDG to four varieties of flax (Celestina, Carmine, Bethune, and Sorrel) was applied. The first two correspond to cultivars of Chilean origin, and the last two were of Canadian origin. The compound was identified by comparing retention times with the respective standards, and the amount of SDG was in the range of 11.3–13 µg g−1 in DFF and 6.9 and 7.6 µg g−1 in whole flaxseeds (). These values were similar to those obtained by other authors. Kanmaz and Ova (Citation2013) reported 12.9 mg g−1 of SDG dry weight (d.w.). Chen et al. (Citation2007) observed between 5.7 and 13 mg g−1 of SDG in flaxseed from the Gansu province, China. Yuan et al. (Citation2008) found SDG in DFF in the range of 6–29 mg g−1 and Li et al. (Citation2008) reported a SDG content of 15.4 mg g−1 in flaxseed. In addition, Johnsson et al. (Citation2000) obtained SDG in the range of 11.7–24.1 mg g−1 in DFF and 6.1–13.3 mg g−1 in whole flaxseed from cultivars of Denmark and Sweden. Hao and Beta (Citation2012) reported that the SDG content in flaxseed hulls varied from 16.4 to 33.9 mg g−1. The SDG content found in this research was higher than that found in other food sources, i.e. total lignans in carob seed flour ranged from 1.6 to 17 µg g−1 d.w. (Durazzo et al., Citation2014), 45.8 µg g−1 d.w. in pomegranate twigs (Fischer et al., Citation2012), 67 µg g−1 in rye (Smeds, Jauhiainen, Tuomola, & Peltonen-Sainio, Citation2009), and 7.36 and 3.10 mg g−1 d.w. in white and black sesame seeds, respectively (Kim et al., Citation2014).
Figure 6. SDG content of flaxseed in four varieties according to their location of cultivation (Cajón and Gorbea, harvested in 2009) and year of harvest (2008). Bar graphs with the same letter are not significantly different (p < 0.05).
Figura 6. Contenido de SDG de linaza en cuatro variedades de acuerdo a su localidad de cultivo (Cajón y Gorbea, cosechada en 2009) y año de cosecha (2008). Gráficos de barras con la misma letra no muestra diferencias significativas (p < 0.05).
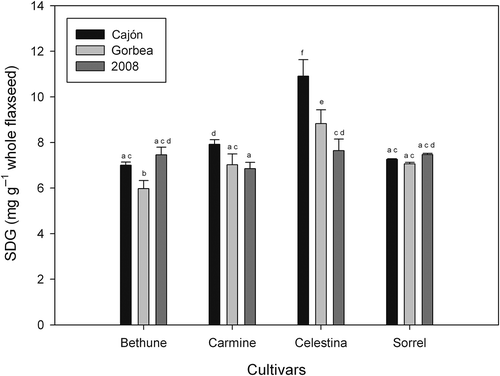
In this study, the highest content of SDG was found in the Celestina variety, followed by Sorrel, while lower levels were found in the Bethune and Carmine varieties. No significant differences were found between the Canadian strains; however, the differences between the Chilean varieties (Celestina and Carmine) were statistically significant (p < 0.05), as they correspond to varieties with higher and lower SDG contents, respectively. All the varieties described above were obtained in 2008.
The content of SDG was also measured in the 2009 harvest of the same varieties from two different areas, Cajón and Gorbea, both in the region of La Araucanía. The highest content of SDG was observed in the cultivars from Celestine, where the contents of lignans in Cajón and Gorbea were 10.9 and 8.8 mg g−1 of whole flaxseed, respectively. These results showed a similar pattern to that from crop year 2008, where the Chilean variety (Celestine) presented a significantly higher SDG content than other cultivars. The Sorrel and Bethune Canadian cultivars showed the lowest levels of SDG for both areas; in Cajón, the SDG content reached 7.1 and 6.0 mg g−1 of whole flaxseed, respectively. shows the SDG content of the different varieties of flax studied in various locations of cultivation and harvest years. In regard to the location of cultivation, the SDG content varied significantly between the cultivars from Gorbea and Cajón, and the highest content was found in the latter location. However, no significant differences were found for the cultivar from Sorrel when grown in both locations. Significantly different amounts of flax were obtained from Gorbea and Cajón because of the drastic difference in their soil types. Gorbea is composed of trumao soils (volcanic soils) and Cajón is mainly composed of transition soils (between trumao and clay soil). Another difference between these two locations was that, at the time of cultivation, there were three irrigation systems in Gorbea, while no irrigation systems existed in Cajón. These conditions can explain the difference in performance and content of SDG. The varieties of flax from the 2008 harvest were different from the Gorbea and Cajón crops in 2009 (p < 0.05), specifically in the Chilean varieties (Celestina and Carmine); however, harvesting these varieties in Cajón significantly increased their performance. In contrast, there was no significant increase in yield at harvest in Cajón for the Canadian variety Bethune. However, there was a reduction in the harvest yield in Gorbea. These results indicate that agronomic variations significantly influence the amount of lignans found in flaxseed.
Conclusions
A method for the purification of SDG from flaxseed using chromatographic techniques as well as HPLC/UV was developed. This method involves the extraction from DFF with sodium methoxide, SPE, and purification of the compound by HPLC. The process of lignan extraction from flaxseed by combining the alcohol extraction and the alkaline hydrolysis in a single step, through a methanolysis with 58 mmol L−1 sodium methoxide at 47°C for 24 h, was optimized. This method was validated and applied for the quantification of SDG and was found that different cultivars of Canadian and Chilean varieties of flaxseed have significantly different SDG contents. In addition, differences in SDG content were observed between harvests of 2008 and 2009, as well as between the location of crops (Cajón and Gorbea). The content of SDG in flaxseed ranged between 6.0 and 10.9 mg g−1 in whole flaxseeds. This work could be used in an easy way for characterizing SDG among flaxseed.
Acknowledgments
The authors wish to thank Mr Fernando Asenjo for his technical assistance. This research was supported by the project FONDECYT 1080277, for which the authors are deeply indebted. C. Fuentealba obtained the CONICYT doctoral fellowship in 2009.
References
- Beejmohun, V., Fliniaux, O., Grand, É., Lamblin, F., Bensaddek, L., Christen, P. … Mesnard, F. (2007). Microwave-assisted extraction of the main phenolic compounds in flaxseed. Phytochemical Analysis, 18(4), 275–282. doi:10.1002/pca.973
- Bravi, E., Perretti, G., Marconi, O., Patrizi, E., & Fantozzi, P. (2011). Secoisolariciresinol diglucoside determination in flaxseed (Linum usitatissimum L.) oil and application to a shelf life study. Food Chemistry, 126(4), 1553–1558. doi:10.1016/j.foodchem.2010.11.169
- Chen, J., Liu, X., Shi, Y. P., & Ma, C. Y. (2007). Determination of the lignan secoisolariciresinol diglucoside from flaxseed (Linum usitatissimum L.) by HPLC. Journal of Liquid Chromatography & Related Technologies, 30(4), 533–544. doi:10.1080/10826070601093853
- Chimichi, S., Bambagiotti-Alberti, M., Coran, S. A., Giannellini, V., & Biddau, B. (1999). Complete assignment of the 1H and 13C NMR spectra of secoisolariciresinol diglucoside, a mammalian lignan precursor isolated from Linum usitatissimum. Magnetic Resonance in Chemistry, 37(11), 860–863. doi:10.1002/(SICI)1097-458X(199911)37:11<860::AID-MRC559>3.0.CO;2-A
- Clavel, T., Borrmann, D., Braune, A., Dore, J., & Blaut, M. (2006). Occurrence and activity of human intestinal bacteria involved in the conversion of dietary lignans. Anaerobe, 12(3), 140–147. doi:10.1016/j.anaerobe.2005.11.002
- Comin, L. M., Temelli, F., & Saldaña, M. A. (2011). Supercritical CO2 extraction of flax lignans. Journal of the American Oil Chemists Society, 88(5), 707–715. doi:10.1007/s11746-010-1704-9
- Coran, S. A., Giannellini, V., & Bambagiotti-Alberti, M. (2004). High-performance thin-layer chromatographic-densitometric determination of secoisolariciresinol diglucoside in flaxseed. Journal of Chromatography A, 1045(1–2), 217–222. doi:10.1016/j.chroma.2004.06.042
- Degenhardt, A., Habben, S., & Winterhalter, P. (2002). Isolation of the lignan secoisolariciresinol diglucoside from flaxseed (Linum usitatissimum L.) by high-speed counter-current chromatography. Journal of Chromatography A, 943(2), 299–302. doi:10.1016/S0021-9673(01)01467-4
- Durazzo, A., Turfani, V., Narducci, V., Azzini, E., Maiani, G., & Carcea, M. (2014). Nutritional characterisation and bioactive components of commercial carobs flours. Food Chemistry, 153, 109–113. doi:10.1016/j.foodchem.2013.12.045
- Eeckhaut, E., Struijs, K., Possemiers, S., Vincken, J. P., De Keukeleire, D., & Verstraete, W. (2008). Metabolism of the lignan macromolecule into enterolignans in the gastrointestinal lumen as determined in the simulator of the human intestinal microbial ecosystem. Journal of Agricultural and Food Chemistry, 56(12), 4806–4812. doi:10.1021/Jf800101s
- Eliasson, C., Kamal-Eldin, A., Andersson, R., & Åman, P. (2003). High-performance liquid chromatographic analysis of secoisolariciresinol diglucoside and hydroxycinnamic acid glucosides in flaxseed by alkaline extraction. Journal of Chromatography A, 1012(2), 151–159. doi:10.1016/s0021-9673(03)01136-1
- Fischer, U. A., Jaksch, A. V., Carle, R., & Kammerer, D. R. (2012). Determination of lignans in edible and nonedible parts of pomegranate (Punica granatum L.) and products derived therefrom, particularly focusing on the quantitation of isolariciresinol using HPLC-DAD-ESI/MSn. Journal of Agricultural and Food Chemistry, 60(1), 283–292. doi:10.1021/jf203598m
- Hano, C., Martin, I., Fliniaux, O., Legrand, B., Gutierrez, L., Arroo, R. R. J. … Lainé, E. (2006). Pinoresinol–lariciresinol reductase gene expression and secoisolariciresinol diglucoside accumulation in developing flax (Linum usitatissimum) seeds. Planta, 224(6), 1291–1301. doi:10.1007/s00425-006-0308-y
- Hao, M. L., & Beta, T. (2012). Qualitative and quantitative analysis of the major phenolic compounds as antioxidants in barley and flaxseed hulls using HPLC/MS/MS. Journal of the Science of Food and Agriculture, 92(10), 2062–2068. doi:10.1002/jsfa.5582
- Herchi, W., Arráez-Román, D., Trabelsi, H., Bouali, I., Boukhchina, S., Kallel, H. … Fernández-Gutierrez, A. (2014). Phenolic compounds in flaxseed: A review of their properties and analytical methods. An overview of the last decade. Journal of Oleo Science, 63(1), 7–14. doi:10.5650/jos.ess13135
- Hosseinian, F. S., & Mazza, G. (2009). Triticale bran and straw: Potential new sources of phenolic acids, proanthocyanidins, and lignans. Journal of Functional Foods, 1(1), 57–64. doi:10.1016/j.jff.2008.09.009
- Hu, C., Yuan, Y. V., & Kitts, D. D. (2007). Antioxidant activities of the flaxseed lignan secoisolariciresinol diglucoside, its aglycone secoisolariciresinol and the mammalian lignans enterodiol and enterolactone in vitro. Food and Chemical Toxicology, 45(11), 2219–2227. doi:10.1016/j.fct.2007.05.017
- Johnsson, P., Kamal-Eldin, A., Lundgren, L. N., & Åman, P. (2000). HPLC method for analysis of secoisolariciresinol diglucoside in flaxseeds. Journal of Agricultural and Food Chemistry, 48(11), 5216–5219. doi:10.1021/jf0005871
- Johnsson, P., Peerlkamp, N., Kamal-Eldin, A., Andersson, R. E., Andersson, R., Lundgren, L. N., & Åman, P. (2002). Polymeric fractions containing phenol glucosides in flaxseed. Food Chemistry, 76(2), 207–212. doi:10.1016/S0308-8146(01)00269-2
- Kamal-Eldin, A., Peerlkamp, N., Johnsson, P., Andersson, R., Andersson, R. E., Lundgren, L. N., & Åman, P. (2001). An oligomer from flaxseed composed of secoisolariciresinoldiglucoside and 3-hydroxy-3-methyl glutaric acid residues. Phytochemistry, 58(4), 587–590. doi:10.1016/S0031-9422(01)00279-5
- Kanmaz, E. O., & Ova, G. (2013). The effective parameters for subcritical water extraction of SDG lignan from flaxseed (Linum usitatissimum L.) using accelerated solvent extractor. European Food Research and Technology, 237(2), 159–166. doi:10.1007/s00217-013-1974-1
- Kim, J. H., Seo, W. D., Lee, S. K., Lee, Y. B., Park, C. H., Ryu, H. W., & Lee, J. H. (2014). Comparative assessment of compositional components, antioxidant effects, and lignan extractions from Korean white and black sesame (Sesamum indicum L.) seeds for different crop years. Journal of Functional Foods, 7, 495–505. doi:10.1016/j.jff.2014.01.006
- Kitts, D. D., Yuan, Y. V., Wijewickreme, A. N., & Thompson, L. U. (1999). Antioxidant activity of the flaxseed lignan secoisolariciresinol diglycoside and its mammalian lignan metabolites enterodiol and enterolactone. Molecular and Cellular Biochemistry, 202, 91–100. doi:10.1023/A:1007022329660
- Konar, N. (2013). Non-isoflavone phytoestrogenic compound contents of various legumes. European Food Research and Technology, 236(3), 523–530. doi:10.1007/s00217-013-1914-0
- Konar, N., Poyrazoğlu, E. S., Demir, K., & Artik, N. (2012). Effect of different sample preparation methods on isoflavone, lignan, coumestan and flavonoid contents of various vegetables determined by triple quadrupole LC-MS/MS. Journal of Food Composition and Analysis, 26(1–2), 26–35. doi:10.1016/j.jfca.2012.01.002
- Li, X., Yuan, J.-P., Xu, S.-P., Wang, J.-H., & Liu, X. (2008). Separation and determination of secoisolariciresinol diglucoside oligomers and their hydrolysates in the flaxseed extract by high-performance liquid chromatography. Journal of Chromatography A, 1185(2), 223–232. doi:10.1016/j.chroma.2008.01.066
- Meagher, L. P., & Beecher, G. R. (2000). Assessment of data on the lignan content of foods. Journal of Food Composition and Analysis, 13(6), 935–947. doi:10.1006/jfca.2000.0932
- Muir, A. D., & Wescott, N. D. (Eds.). (2003). Flax: The genus linum. Saskatchewan, CA: Taylor & Francis.
- Myers, R. H., Montgomery, D. C., & Anderson-Cook, C. M. (2009). Response surface methodology: Process and product optimization using designed experiments. New York, NY: Wiley.
- Pihlava, J. M., Hyvarinen, H., Ryhanen, E. L., & Hietantemi, V. (2004). Process for isolating and purifying secoisolariciresinol diglucoside (SDG) from flaxseed. U.S. Patent No. 2004/0030108. United States Patent Application.
- Prasad, K. (2000). Antioxidant activity of secoisolariciresinol diglucoside-derived metabolites, secoisolariciresinol, enterodiol, and enterolactone. International Journal of Angiology, 9(4), 220–225. doi:10.1007/bf01623898
- Renouard, S., Hano, C., Corbin, C., Fliniaux, O., Lopez, T., Montguillon, J. … Lainé, E. (2010). Cellulase-assisted release of secoisolariciresinol from extracts of flax (Linum usitatissimum) hulls and whole seeds. Food Chemistry, 122(3), 679–687. doi:10.1016/j.foodchem.2010.03.036
- Smeds, A. I., Eklund, P. C., Sjöholm, R. E., Willför, S. M., Nishibe, S., Deyama, T., & Holmbom, B. R. (2007). Quantification of a broad spectrum of lignans in cereals, oilseeds, and nuts. Journal of Agricultural and Food Chemistry, 55(4), 1337–1346. doi:10.1021/jf0629134
- Smeds, A. I., Jauhiainen, L., Tuomola, E., & Peltonen-Sainio, P. (2009). Characterization of variation in the lignan content and composition of winter rye, spring wheat, and spring oat. Journal of Agricultural and Food Chemistry, 57(13), 5837–5842. doi:10.1021/jf9004274
- Stasevich, O. V., Mikhalenok, S. G., & Kurchenko, V. P. (2009). Isolation of secoisolariciresinol diglucoside from lignan-containing extract of Linum usitatissimum seeds. Chemistry of Natural Compounds, 45(1), 21–23. doi:10.1007/s10600-009-9217-1
- Struijs, K., Vincken, J. P., Doeswijk, T. G., Voragen, A. G. J., & Gruppen, H. (2009). The chain length of lignan macromolecule from flaxseed hulls is determined by the incorporation of coumaric acid glucosides and ferulic acid glucosides. Phytochemistry, 70(2), 262–269. doi:10.1016/j.phytochem.2008.12.015
- Thompson, L. U., Boucher, B. A., Liu, Z., Cotterchio, M., & Kreiger, N. (2006). Phytoestrogen content of foods consumed in Canada, including isoflavones, lignans, and coumestan. Nutrition and Cancer-an International Journal, 54(2), 184–201. doi:10.1207/s15327914nc5402_5
- Thompson, L. U., Robb, P., Serraino, M., & Cheung, F. (1991). Mammalian lignan production from various foods. Nutrition and Cancer-an International Journal, 16(1), 43–52. doi:10.1080/01635589109514139
- Touillaud, M. S., Thiebaut, A. C. M., Fournier, A., Niravong, M., Boutron-Ruault, M.-C., & Clavel-Chapelon, F. (2007). Dietary lignan intake and postmenopausal breast cancer risk by estrogen and progesterone receptor status. JNCI Journal of the National Cancer Institute, 99(6), 475–486. doi:10.1093/jnci/djk096
- Wang, L. Q., Meselhy, M. R., Li, Y., Qin, G. W., & Hattori, M. (2000). Human intestinal bacteria capable of transforming secoisolariciresinol diglucoside to mammalian lignans, enterodiol and enterolactone. Chemical & Pharmaceutical Bulletin, 48(11), 1606–1610. doi:10.1248/cpb.48.1606
- Westcott, N. D., & Muir, A. D. (1998). Process for extracting lignans from flaxseed. U.S. Patent No. 5, 705,618.
- Yuan, J. P., Li, X., Xu, S. P., Wang, J. H., & Liu, X. (2008). Hydrolysis kinetics of secoisolariciresinol diglucoside oligomers from flaxseed. Journal of Agricultural and Food Chemistry, 56(21), 10041–10047. doi:10.1021/jf8020656
- Zhang, W., Wang, X., Liu, Y., Tian, H., Flickinger, B., Empie, M. W., & Sung, S. Z. (2008). Dietary flaxseed lignan extract lowers plasma cholesterol and glucose concentrations in hypercholesterolaemic subjects. British Journal of Nutrition, 99(6), 1301–1309. doi:10.1017/S0007114507871649
- Zhang, W. B., & Xu, S. Y. (2007). Microwave-assisted extraction of secoisolariciresinol diglucoside from flaxseed hull. Journal of the Science of Food and Agriculture, 87(8), 1455–1462. doi:10.1002/jsfa.2793
- Zhang, Z. S., Li, D., Wang, L. J., Ozkan, N., Chen, X. D., Mao, Z. H., & Yang, H. Z. (2007). Optimization of ethanol-water extraction of lignans from flaxseed. Separation and Purification Technology, 57(1), 17–24. doi:10.1016/j.seppur.2007.03.006