ABSTRACT
The objective of this study was to accelerate the drying process of Chinese dry-cured chicken using high temperatures. Salted chicken samples were treated with different high-temperature–short-time (HTST) combinations (50°C/27 h, 55°C/25 h, 60°C/23 h, 65°C/21 h). The effects of various high temperatures on proteolysis, free amino acids (FAAs), thiobarbituric acid reactive substances (TBARS), and sensory attributes were analyzed. The results revealed that high temperature accelerated lipid oxidation, protein oxidation, and proteolysis without producing undesirable flavors. By using the HTST process, the Warner–Bratzler-shear-force (WBSF) values were significantly increased (P < 0.05), and the scores for color, aroma, and taste were enhanced. The best sensory attributes were obtained with the 55°C/25 h-treated samples. Moreover, samples treated with 55°C/25 h had the highest TBARS values (1.32 mg MDA/kg) and total FAAs contents (4693.2 mg/kg muscle). Therefore, the use of high temperature is an effective way to accelerate the dry-curing process and improve the sensory qualities of Chinese dry-cured chicken.
RESUMEN
El objetivo de este estudio fue acelerar el proceso de secado de pollo curado chino utilizando altas temperaturas. Se trataron muestras saladas de pollo con diferentes combinaciones de periodos cortos a altas temperaturas (HTST) (50°C/27 h, 55°C/25 h, 60°C/23 h, 65°C/21 h). Se analizaron los efectos de diferentes temperaturas altas en la proteólisis, los aminoácidos libres (FAAs), las sustancias reactivas a los ácidos tiobarbitúricos (TBARS) y los atributos sensoriales. Los resultados revelaron que las altas temperaturas habían acelerado la oxidación lipídica, la oxidación proteínica y la proteólisis sin producir sabores indeseables. Mediante la utilización del proceso HTST, los valores de la fuerza cortante de Warner Bratzler (WBSF) (P < 0,05) aumentaron significativamente y se mejoraron los resultados del color, el aroma y el sabor. Los mejores atributos sensoriales se obtuvieron con las muestras tratadas a 55°C/25 h. Además, las muestras tratadas a 55°C/25 h obtuvieron los mayores valores de TBARS (1,32 mg MDA/kg) y de contenido total de FAAs (4693,2 mg/kg de músculo). Por lo tanto, el uso de altas temperaturas es un método efectivo para acelerar el proceso de curado y mejorar las cualidades sensoriales del pollo curado chino.
Introduction
Dry-cured chicken is a traditional dry-cured meat product made in southeast China and is famous for its unique cured flavor. The traditional method for making dry-cured chicken involves natural maturation, which is climate limited and time consuming. Increasing the drying temperature is a potential way of shortening the process of producing dry-cured meat products (Arnau, Serra, Comaposada, Gou, & Garriga, Citation2007) and has been successfully applied to shorten the drying period of Jinhua ham (J. Zhang, Jin, Wang, & Zhang, Citation2011). The Chinese sausage industry has also widely used high-temperature dehydration procedures (50–55°C) to accelerate the process (Feng et al., Citation2014; Sun, Cui, Zhao, Zhao, & Yang, Citation2011; L. Zhang, Lin, Leng, Huang, & Zhou, Citation2013). However, increasing the temperature may significantly influence the chemical and biochemical reactions during the drying period, leading to changes in the sensory attributes or deterioration of the finished products.Thus, understanding the effect of high temperature on the physical and chemical characteristics of the product is significant for manufacturers to improve the profit margin and food qualities of Chinese dry-cured chicken.
During the drying period, muscle proteins and lipids are hydrolyzed mainly by endogenous enzymes, resulting in increased amounts of peptides, free amino acids (FAAs), and free fatty acids (Toldrá, Flores, & Sanz, Citation1997). These products constitute the main characteristics of dry-cured flavor substances and may continue to react with one another or be hydrolyzed to produce volatiles that con tribute to the unique aroma of the final product (Barbieri et al., Citation1992). The effect of temperature during the drying period has been described in previous studies (Gou, Morales, Serra, Guàrdia, & Arnau, Citation2008; Rubio-Celorio, Garcia-Gil, Gou, Arnau, & Fulladosa, Citation2015; Sánchez-Molinero & Arnau, Citation2014). Indeed, temperature is an essential factor because it affects the action of endogenous muscle peptidases, which plays an important role in proteolysis (Mora et al., Citation2015). Martin et al. (Citation1998) observed that the drying temperature determines the levels and the types of compounds released via protein breakdown during the dry curing of Iberian hams. These changes in protein composition contribute to the texture and to the sensory and nutritional quality of meat products (Visessanguan, Benjakul, Riebroy, & Thepkasikul, Citation2004). However, high temperature is an important factor for accelerating lipid oxidation, which also exerts effects on the taste and odor compound formation and is also the main reason for off-flavor, rancidity, or textural modification of dry-cured meat products (Broncano, Petrón, Parra, & Timón, Citation2009; Harkouss et al., Citation2015). The positive effect of high-temperature ripening on lipolysis and lipid oxidation of Jinhua ham has previously been reported (J. Zhang et al., Citation2011). However, to the best of our knowledge, few studies have investigated the use of the HTST process for producing dry-cured chicken or its effects on the product’s proteolysis and sensory qualities.
In this study, the HTST drying process was studied as an alternative method to accelerate the process of Chinese dry-cured chicken. The purpose of this work was to study the influence of HTST on the proteolysis, lipid oxidation, and sensory attributes of dry-cured chicken, compared with the traditional drying method to determine the feasibility of using high temperature to reduce the production time of Chinese dry-cured chicken, and to optimize the process parameters.
Materials and methods
Materials
Chinese native three-yellow-chickens were uniformly slaughtered according to the Animal Experimental Special Committee of Nanjing Agricultural University (NAU), which governs the use of experimental animals. Twenty chicken breasts were collected and subjected to trimming, cleaning, and freezing at −20°C prior to use.
Dry-cured chicken preparation and sampling
After thawing at 0 ~ 4°C for 8 h, the chicken breasts were immersed in precooled curing water at 0 ~ 4°C for 20 h (curing formulation: 300 g of salt in 3 kg of water). The salted breasts were hung in a preheated oven (KBF 115-pgm, Binder, Germany). Control samples were treated with one of the traditional methods used by a local factory: drying for 7 days at 15°C with 70% relative humidity. HTST-treated samples were treated with various temperature–time combinations to achieve the same moisture content with the control: 50°C/27 h, 55°C/25 h, 60°C/23 h, and 65°C/21 h (RH = 70%). Immediately after the drying process, the samples were cooled to room temperature for 1 h and vacuum-packaged (DC-800, Promarks Inc., USA) with plastic vacuum packaging bags. Four samples from each treatment were randomly selected for the evaluation of the moisture content and water activity. Eight samples from each treatment were randomly selected and kept at 4°C, of which four were used for the Warner–Bratzler shear force (WBSF) analysis and four were used for the sensory evaluation. All of the WBSF and sensory evaluations were performed the day after the samples were produced. Four samples from each treatment were randomly selected for the analysis of their chemical properties. The remaining samples were kept at −20°C for further use. The entire production procedure was replicated three times at different time points.
Determination of moisture content and water activity
Moisture content was determined according to the method specified in ISO-1442 (1997). The samples were dehydrated in an oven (DHG-903385-III, Shanghai CIMO Medical Instrument Manufacturing Co., I.TD, Shanghai, China) at 105°C to a constant weight. Water activity (aw) was detected at 25°C using a water activity meter (LabMaster-aw, Novasina AG, Switzerland).
Instrumental texture analysis
Tenderness was evaluated via WBSF analysis according to Jose M. Lorenzo, Bermúdez, et al. (Citation2015) with slight modifications. The samples were placed in vacuum bags (unsealed) and heated to 70°C in a water bath (72°C). After being chilled at 4°C for 8 h, four 25 mm × 10 mm × 10 mm (height × width × length) cores were removed from each sample parallel to the muscle fiber direction. Each core was cut vertically in the direction of the muscle fibers using a Warner–Bratzler shear blade. The WBSF data were obtained using a texture analyzer (TA-XTplus, Stable Microsystems, UK).
Determination of thiobarbituric acid-reactive substances (TBARS)
The thiobarbituric acid-reactive substances (TBARS) concentration was determined according to Salih, Smith, Price, and Dawson (Citation1987) with slight modifications. A 5 g minced sample was homogenized with 25 mL of cold (4°C) extraction solution containing 20% perchloric acid and 20 mL of distilled water and 0.50 mL of butylated hydroxytoluene (BHT) in a Virtis homogenizer at 10,000 rpm for 1 min. The homogenate was centrifuged at 2000 g for 10 min at 4°C. The blended sample was filtered into a 50-mL Erlenmeyer flask. The filtrate was adjusted to 50 mL with distilled water, and 2 mL of the filtrate was added to 2 mL of 0.02 M TBA. Test tubes were heated in a thermostatically controlled water bath for 30 min at 95°C to develop the malonaldehyde–TBA complex and then cooled for 5 min with cold tap water. The absorbance was determined at 532 nm using a multifunctional microplate reader (Model Spectral Max M2e, MD, USA) against a blank containing 2 mL of 10% per-chloric acid and 2 mL of 0.02 M TBA reagent. The TBARS concentration was calculated from a standard curve in triplicate using solutions of 1,1,3,3-tetraethoxypropane (TEP). The results were expressed as mg malonaldehyde (MDA) equivalents per kg of meat sample.
Protein carbonyls
Protein carbonyl content was evaluated according to the method described by L. Zhang et al. (Citation2013). Carbonyl groups were reacted with 2,4-dinitrophenylhydrazine (DNPH) to develop the protein hydrazones, which were detected by measuring the absorbance at 370 nm in a spectrophotometer (UV-2450, SHIMADZU, Japan). Protein concentrations were calculated using a standard BSA assay by measuring the absorbance at 280 nm. The content of carbonyl groups was expressed as nmol carbonyl/mg protein using an extinction coefficient of 21.0 mM−1 × cm−1.
Proteolysis
The protein composition was fractionated according to the method described by Sun et al. (Citation2011) with slight modifications. First, 5 g minced samples were homogenized with 50 mL of phosphate buffer A (15.6 mM Na2HPO4 and 3.5 mM KH2PO4, pH 7.5) at 8000 rpm for 1 min in ice bath. The homogenate was centrifuged at 5000 g for 15 min at 4°C. The extraction was repeated twice. The supernatants, which contained water-soluble proteins, were combined. Then the remaining pellet was homogenized with 50 mL of phosphate buffer B (0.45 M KCl, 15.6 mM Na2HPO4 and 3.5 mM KH2PO4, pH 7.5) at 8000 rpm for 1 min in an ice bath and centrifuged at 5000 g for 15 min at 4°C. The extraction with phosphate buffer B was repeated twice, and the supernatant was combined to obtain salt-soluble proteins.
The concentrations of water-soluble and salt-soluble proteins were determined with a BCA Protein Assay Kit (Pierce, USA). The samples were then mixed with treatment buffer (125 mmol/L Tris, 40 g/L sodium dodecyl sulfate (SDS), and 250 g/L glycerol), heated at 50°C for 20 min and then stored at −80°C for subsequent sodium dodecyl sulfate polyacrylamide gel electrophoresis (SDS-PAGE) according to the method described by Etlinger, Zak, and Fischman (Citation1976). The gels were scanned with a GT-800F scanner (EPSON), and then, the densities of the targeted bands were analyzed by Quantity One software (Bio-Rad).
Free amino acids
Free amino acids (FAAs) were analyzed according to the procedures described by Aro et al. (Citation2010).
Sensory evaluation
Sensory evaluation was performed by an experienced sensory panel, composed of 17 members of the National Centre of Meat Quality and Safety Control. The samples subjected to each treatment were cooked in boiling water for 30 min, and then cooled to room temperature. The chicken breasts were then sliced into pieces with thick nesses of approximately 5 mm, and placed on separate white ceramic plates. Each treatment was identified with a random three-digit code. The panelists were instructed to gargle between evaluations to reduce any effects of other samples. All of the tasting sessions were conducted at the same time of each test day in a quiet room with a mixture of natural and fluorescent light, and with no interactions between panelists. Each panelist was asked to evaluate the sensory attributes of the chicken samples of all five treatments, including the color, aroma, taste, and texture. A 9-point hedonic scale was applied (Lim, Citation2011): 1, dislike extremely; 2, dislike very much; 3, dislike moderately; 4, dislike slightly; 5, neither like nor dislike; 6, like slightly; 7, like moderately; 8, like very much and 9, like extremely.
Statistical analysis
The entire experiment was replicated three times at different times, and a completely randomized design was used. All of the data from the three replicates were analyzed using Excel 2007 (Microsoft, Washington) and SPSS software (SPSS Inc., Chicago, IL, USA). Differences among individual means were compared by Duncan’s multiple range test. Effects were considered significant at P < 0.05.
Results and discussion
Moisture content and water activity (aw) analysis
Increasing the temperature did not significantly affect (P > 0.05) the moisture content or aw of the dry-cured chicken (). Moisture is an important factor in the standardization of the product, and it not only affects the final appearance and juiciness but also has great economic importance to the industry. Generally, higher temperature increases the effective water diffusivity and facilitates the migration of water (Sánchez-Molinero & Arnau, Citation2014). Therefore, the drying-time of each HTST group was adjusted to standardize the moisture of the samples to the control. The value of aw indicates the unbound and free water that is available to support the chemical and biological reactions in a system, especially the growth of microorganisms (Feng et al., Citation2014). No significant differences were found between the aw values, indicating that HTST did not decrease the storage properties of the dry-cured chicken. This finding was consistent with previously reported results for dry-cured hams (Costa-Corredor, Serra, Arnau, & Gou, Citation2009).
Table 1. Moisture content, water activity (aw), and WBSF values of Chinese dry-cured chicken treated with various methods.
Tabla 1. Contenido de humedad, actividad del agua (aw) y valores de WBSF del pollo curado chino tratado con diferentes métodos.
Tenderness determined by WBSF
Elevating the drying-temperature significantly increased (P < 0.05) the WBSF values in the HTST-treated samples (). The WBSF values of the control group were significantly higher than 50HT (P < 0.05) but significantly lower than 65HT (P < 0.05), and there was no significant difference between the control and 55HT ~ 60HT (P > 0.05). WBSF is related to the tenderness, which is one of the most important sensory characteristic of meat product (Cai, Chen, Wan, & Zhao, Citation2011). Huang, Huang, Xu, and Zhou (Citation2011) observed a decrease in tenderness when the temperature was increased from 40°C to 60°C, which was consistent with our study. Increased temperature may strengthen the myofibrillar protein networks or cause protein denaturation, leading to toughness (Bouton, Harris, & Shorthose, Citation1982). However, an appropriate high temperature may also contribute to tenderness by solubilizing the collagen and connective tissue (Bouton et al., Citation1982), which may explain the lower WBSF value in 50HT. Moreover, Christensen, Purslow, and Larsen (Citation2000) observed an increase in tenderness for the same temperature interval and attributed it to collagen solubility, which was not consistent with our results. This was most likely because of the relatively low collagen content in the longissimus dorsi of chicken breast. Excessive tenderness or toughness may have a negative effect on the texture of the final product (Ishiwatari, Fukuoka, & Sakai, Citation2013). The relationship between the WBSF values and the texture of Chinese dry-cured chicken was analyzed in the following sensory evaluation.
Lipid oxidation determined by TBARS
Higher drying temperatures significantly influenced (P < 0.05) the TBARS values of dry-cured chicken (). The results showed that the TBARS values increased between 50HT and 55HT, and then gradually decreased between 55HT and 65HT. Yun, Shahidi, Rubin, and Diosady (Citation1987) reported that the lipid-oxidation depends on the thermal-processing temperature. Therefore, the initial increasing phase may be caused by the higher temperature, which was consistent with other studies (Broncano et al., Citation2009; Wang et al., Citation2013; Wenjiao, Yongkui, Yunchuan, Junxiu, & Yuwen, Citation2014). However, aldehydes are unstable and can be directly degraded into volatile compounds (Ventanas, Estévez, Delgado, & Ruiz, Citation2007), or interact with other groups of proteins (Jin et al., Citation2012), leading to the formation of fluorescent Schiff bases (Harkouss et al., Citation2015). The formation of such products prevents the reaction of aldehydes and TBA, explaining the subsequent decrease from 55HT to 65HT observed in our study. A similar decrease in TBARS was previously reported by Roldan, Antequera, Armenteros, and Ruiz (Citation2014) in lambs. Several reports showed that lipid oxidation played an important role in the development of the typical dry-cured flavor (Barbieri et al., Citation1992; Ruiz, Garcı́a, Muriel, Andrés, & Ventanas, Citation2002). There was no significant difference between the control group and 65HT (P > 0.05), indicating that HTST could achieve the same or even higher levels of lipid oxidation. However, excessive lipid oxidation may result in off-flavor in dry-cured meat products (Böttcher, Steinhäuser, & Drusch, Citation2015). In our study, the TBARS was 0.92–1.32 mg MDA/kg sample, which was higher than the values obtained by Feng et al. (Citation2014) in Chinese sausage and by Cilla, Martínez, Beltrán, and Roncalés (Citation2006) in dry-cured ham, but was lower than the threshold value for off-flavor (2 mg MDA/kg) reported by Wenjiao et al. (Citation2014). The relationship between TBARS and the flavor of dry-cured chicken is further analyzed in the following sensory evaluation.
Table 2. TBARS (mg MDA/kg) and protein carbonyls of Chinese dry-cured chicken treated by different methods.
Tabla 2. TBARS (mg MDA/kg) y carbonilos proteínicos del pollo curado chino tratado con diferentes métodos.
Protein oxidation determined by protein carbonyl
The results of the HTST groups were significantly higher (P < 0.05) than those of the control (), but increasing the temperature from 50HT to 65HT produced no significant effect (P > 0.05). Roldan et al. (Citation2014) reported that protein carbonyls reached similar final values regardless of the heating temperature, which was consistent with our study. Moreover, the higher results in the HTST groups demonstrated the accelerating effect of high temperature on the protein-oxidation rate, which was consistent with a previous study on lamb loins that were heated for 24 h at temperature ranging from 60 to 80°C (Roldan et al., Citation2014). High temperature is known to enhance protein carbonylation because of several effects, such as the release of free catalytic iron and the formation and cleavage of hydroperoxides (Estévez, Citation2011). Protein oxidation during the ripening of meat products was also suggested to be involved in the formation of Strecker aldehydes, which contribute to the dry-cured flavor (Toldra, Citation1998). The protein-oxidation results detected here exceeded those recorded for Chinese-style sausage dried at 55°C for 48 h (L. Zhang et al., Citation2013). This may be due to the different oxidative stabilities of the varying compositions of protein and resistance of muscle fibers to thermal treatment (Ma, Ledward, Zamri, Frazier, & Zhou, Citation2007). Indeed, the formation of protein carbonyls from particular amino acid side chains contributes to the impairment of the myofibrillar protein conformation (Estévez, Citation2011).
Proteolysis
Electrophoretic analysis of water-soluble protein ( and ) and salt-soluble protein ( and ) revealed a significant difference (P < 0.05) between the HTST groups and the control. Water-soluble protein bands with molecular weights of 110 ~ 230 kDa and 35 ~ 50 kDa decreased in the HTST groups, whereas those with a molecular weight of 58 kDa displayed a higher density in 50HT and 55HT but a decreased density in 60HT and 65HT. In addition, those with a smaller molecular weight of 16 ~ 17 kDa increased slightly as the temperature increased. In summary, the HTST process contributes to the degradation of water-soluble proteins with high molecular weights, whereas proteins with smaller molecular weights persisted in 50HT and 55HT.
Figure 1. SDS-PAGE profile of protein fractions of Chinese dry-cured chicken treated with various methods (A–B). (A) Water-soluble protein; (B) Salt-soluble protein. Lanes from left to right: Molecular standards (M), control (C), 50°C/27 h HTST treatment (50HT), 55°C/25 h HTST treatment (55HT), 60°C/23 h HTST treatment (60HT), 65°C/21 h HTST treatment (65HT). Eight micrograms of proteins was loaded per lane for both the water-soluble proteins and salt-soluble proteins.
Figura 1. Perfil SDS-PAGE de fracciones proteínicas de pollo curado chino tratado con varios métodos (A–B). (A) Proteína soluble en agua; (B) Proteína soluble en sal. Líneas de izquierda a derecha: Estándares moleculares (M), control (C), tratamiento HTST a 50°C/27 h (50HT), tratamiento HTST a 55°C/25 h (55HT), tratamiento HTST a 60°C/23 h (60HT), tratamiento HTST a 65°C/21 h (65HT). Se cargaron ocho microorganismos de proteínas por línea para las proteínas solubles en agua y las proteínas solubles en sal.
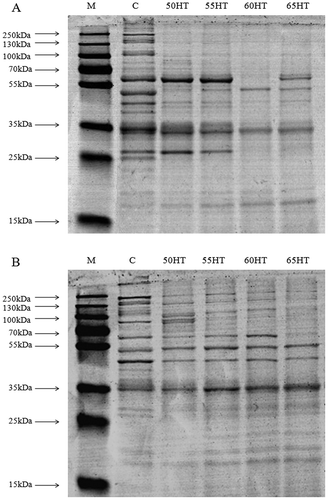
Table 3. Relative values of water-soluble protein bands of Chinese dry-cured chicken treated with various methods.
Tabla 3. Valores relativos de las bandas proteínicas solubles en agua del pollo curado chino tratado con diferentes métodos.
Table 4. Relative values of salt-soluble protein bands of Chinese dry-cured chicken treated with various methods.
Tabla 4. Valores relativos de las bandas proteínicas solubles en sal de pollo curado chino tratado con diferentes métodos.
Salt-soluble proteins had greater thermal stability than water-soluble proteins (). Protein bands with a high molecular weights of 55 kDa ~ 230 kDa gradually decreased from 50HT to 65HT. Bands at 43 ~ 44 kDa and 35 kDa displayed higher densities in 55HT, whereas those at 18 ~ 20 kDa increased slightly as the temperature increased in the HTST groups, which is consistent with the water-soluble protein results.
Proteolysis has an important effect on texture, taste, and, indirectly, the aroma development of dry-cured meat products (Toldra, Citation1998). Harkouss, Safa, Gatellier, Lebert, and Mirade (Citation2014) reported that the rates of proteolysis are increased by 3 or 4 times when the temperature is increased. In this study, high temperature resulted in the degradation of proteins with high molecular weights, which was consistent with previous research on Chinese sausage under similar drying conditions (Feng et al., Citation2014). The results demonstrated the positive effect of high temperature on proteolysis, which is related to the strong activity of muscle proteases in a certain temperature range (50–55°C) (Flores et al., Citation2006). The additional protease activity caused by high temperature may induce two phenomena: 1. both water-soluble and salt-soluble proteins with high molecular weights are degraded, and 2. more native stromal proteins were most likely hydrolyzed into smaller peptides (Molina & Toldra, Citation1992) and FAAs that can directly contribute to the flavor of dry-cured meat products (Cordoba et al., Citation1994).
FAA content
Total FAA content was significantly (P < 0.05) affected by varying the temperature (). Samples treated with 55HT had a significantly (P < 0.05) higher total FAA content (4692.7 mg/kg muscle) and a higher concentration of each individual amino acid, except for tyrosine and taurine, which showed higher content in 50HT. In addition, total FAA content in 65HT was significantly (P < 0.05) lower than in other groups. These differences were most likely attributable to the different activities of the proteolytic enzymes at different temperatures. The results of this study were generally lower than those reported in dry-cured ham (Martı́n, Antequera, Ventanas, Benı́tez-Donoso, & Córdoba, Citation2001; Virgili, Saccani, Gabba, Tanzi, & Soresi Bordini, Citation2007), and lacón (Lorenzo, Fonseca, Gómez, & Domínguez, Citation2015). The conversion of peptides into FAAs would occur during the last step of the proteolytic process involved in ripening, mainly produced by cathepsin, calpains, and amino-peptidases (Toldrá et al., Citation1997). The activities of these enzymes are dependent on the temperature during the drying process (Toldrá et al., Citation1997; Zhao et al., Citation2005). Martı́n et al. (Citation2001) observed that the drying-temperature stimulated proteolytic activity of cathepsin D and exopeptidases of both muscle and microbial origin in Iberian ham, leading to the release of amino acids. Moreover, Zhao et al. (Citation2005) reported that the activities of cathepsin B and cathepsin L during the processing of Jinhua ham increased as the temperature increased. In addition, Toldrá, Rico, and Flores (Citation1992) concluded that high temperature (65 and 69°C for 15 min) inactivated both cathepsin B and arginyl hydrolyzing activities and reduced the cathepsin H, leucyl and tyrosyl hydrolyzing activities (<4%), which could explain the low FAA content in 65HT in our study.
Table 5. Free amino acids (mg/kg muscle) of Chinese dry-cured chicken treated with various methods
Tabla 5. Aminoácidos libres (mg/kg de músculo) de pollo curado chino tratado con diferentes métodos.
At the end of the drying process, the major FAA was alanine, followed by lysine, glutamine, leucine, and arginine. The same FAAs have also been reported as the most abundant in dry-cured meat products, such as Iberian ham (Cordoba et al., Citation1994; Martı́n et al., Citation2001), Parma ham (Sforza et al., Citation2001), dry-cured cecina (Lorenzo, Fonseca, et al., Citation2015), and lacón (Garrido, Domínguez, Lorenzo, Franco, & Carballo, Citation2012). The results also showed that the FAA with the lowest levels at the end of the drying process was taurine, which was consistent with the results of lacón (Garrido et al., Citation2012).
FAAs have been reported as precursors of sour, sweet, and bitter tastes in dry-cured ham (Cordoba et al., Citation1994). Some amino acids were correlated with specific ham tastes, such as glutamic acid with saltiness; alanine with sweetness; arginine, valine, and histidine with bitterness; tyrosine and lysine with aged taste; and leucine with acid taste (Careri et al., Citation1993). The combination of all of the FAAs contributes to the characteristic taste of dry-cured ham (Bermúdez, Franco, Carballo, Sentandreu, & Lorenzo, Citation2014). The higher FAA content in 55HT might make an important contribution to improving the flavor of dry-cured chicken. Besides, the FAAs may also act as flavor precursors in the generation of volatiles (Jurado, García, Timón, & Carrapiso, Citation2007), and the lower FAA content in this study might accordingly indicate a more active degradation of FAAs into volatile compounds (Berdagué, Monteil, Montel, & Talon, Citation1993; Waade & Stahnke, Citation1997) during the HTST process.
Sensory evaluation
No undesirable flavors or tastes were observed by the panelists in the sensory evaluation of the samples (). For the color scores, a significant difference (P < 0.05) was found between the HTST groups and the control. The samples in the HTST groups were significantly more appreciated by the panelists for their light and fresh colors. Samples treated with 50HT, 55HT, and 60HT showed significantly higher scores for aroma (P < 0.05) than 65HT and the control. In addition, the 50HT and 55HT groups showed higher scores for taste. Thus, as the drying-temperature increased, the scores of color, aroma, and taste followed the similar trend. The highest score for texture was observed in 55HT and 60HT, whereas excessive toughness was indicated by lower texture scores in 65HT.
Table 6. Sensory attributes of Chinese dry-cured chicken treated with various methods.
Tabla 6. Atributos sensoriales del pollo curado chino tratado con diferentes métodos.
The sensory quality of the dry-cured meat product was affected by the biochemical reactions during the drying process. The scores revealed a significant effect of high temperature on color, aroma, taste, and texture, which may result from the accelerated lipid oxidation and additional proteolysis. Color is an important trait in food quality and is considered to be an indicator of meat freshness and doneness for consumers (Huang et al., Citation2011). The results showed that samples treated with the HTST process obtained a higher color score because of an impression of brightness. An increase in brightness was also found in the study of dry-cured ham treated with the HTST process (Sánchez-Molinero & Arnau, Citation2014). For the aroma and taste scores, many researchers have reported that a variety of small peptides and FAAs produced by the dry-cured meat products contribute to aroma characteristics (Virgili et al., Citation2007), taste properties, and water-soluble flavor precursors (Koutsidis et al., Citation2008). In addition, Careri et al. (Citation1993) found that hams with the highest acceptability scores had high levels of free tyrosine and lysine. In our study, samples with a higher FAA content obtained a higher score for taste, which confirmed the correlation between FAAs and the taste of dry-cured meat products. Finally, texture is rated by consumers as the most important quality characteristic of meat (Shackelford et al., Citation2001). The scores for texture revealed that high temperature made no significant difference between 55HT and 60HT and the control but did result in remarkable toughness, as indicated by the lower texture scores in 65HT. According to the results of sensory evaluation, the best HTST treatment parameters should be 55HT (55°C/25 h).
Conclusion
By decreasing the drying time to less than 25 h, the HTST process effectively accelerated proteolysis, as reflected by the decrease in the large molecule protein bands of both water-soluble and salt-soluble proteins. Tenderness was significantly (P < 0.05) affected by the temperature, as indicated by the increased WBSF. The oxidation of protein and lipids was accelerated by the HTST drying treatment, while no undesirable flavors or tastes were observed in the sensory panel. Samples treated at 55HT (55°C/25 h) exhibited the highest contents of total FAAs and most of the individual FAAs. The color, aroma, and taste scores in the HTST groups were significantly (P < 0.05) higher than in the control. The best sensory attributes were observed in 55°C/25 h-treated samples. In conclusion, applying high-temperature drying condition is a novel method with great potential to accelerate the manufacture of Chinese dry-cured chicken and improve its sensory properties.
Disclosure statement
No potential conflict of interest was reported by the authors.
Additional information
Funding
References
- Arnau, J., Serra, X., Comaposada, J., Gou, P., & Garriga, M. (2007). Technologies to shorten the drying period of dry-cured meat products. Meat Science, 77(1), 81–89. doi:10.1016/j.meatsci.2007.03.015
- Aro, J., Nyam-Osor, P., Tsuji, K., Shimada, K.-I., Fukushima, M., & Sekikawa, M. (2010). The effect of starter cultures on proteolytic changes and amino acid content in fermented sausages. Food Chemistry, 119(1), 279–285. doi:10.1016/j.foodchem.2009.06.025
- Barbieri, G., Bolzoni, L., Parolari, G., Virgili, R., Buttini, R., Careri, M., & Mangia, A. (1992). Flavor compounds of dry-cured ham. Journal of Agricultural and Food Chemistry, 40(12), 2389–2394. doi:10.1021/jf00024a013
- Berdagué, J.L., Monteil, P., Montel, M.C., & Talon, R. (1993). Effects of starter cultures on the formation of flavour compounds in dry sausage. Meat Science, 35(3), 275–287. doi:10.1016/0309-1740(93)90033-E
- Bermúdez, R., Franco, D., Carballo, J., Sentandreu, M.Á., & Lorenzo, J.M. (2014). Influence of muscle type on the evolution of free amino acids and sarcoplasmic and myofibrillar proteins through the manufacturing process of Celta dry-cured ham. Food Research International, 56, 226–235. doi:10.1016/j.foodres.2013.12.023
- Böttcher, S., Steinhäuser, U., & Drusch, S. (2015). Off-flavour masking of secondary lipid oxidation products by pea dextrin. Food Chemistry, 169, 492–498. doi:10.1016/j.foodchem.2014.05.006
- Bouton, P.E., Harris, P.V., & Shorthose, W.R. (1982). The effect of temperature and ultimate pH on the increase in meat toughness resulting from restraint during cooking. Meat Science, 6(3), 235–241. doi:10.1016/0309-1740(82)90032-8
- Broncano, J.M., Petrón, M.J., Parra, V., & Timón, M.L. (2009). Effect of different cooking methods on lipid oxidation and formation of free cholesterol oxidation products (COPs) in Latissimus dorsi muscle of Iberian pigs. Meat Science, 83(3), 431–437. doi:10.1016/j.meatsci.2009.06.021
- Cai, J., Chen, Q., Wan, X., & Zhao, J. (2011). Determination of total volatile basic nitrogen (TVB-N) content and Warner–Bratzler shear force (WBSF) in pork using Fourier transform near infrared (FT-NIR) spectroscopy. Food Chemistry, 126(3), 1354–1360. doi:10.1016/j.foodchem.2010.11.098
- Careri, M., Mangia, A., Barbieri, G., Bouoni, L., Virgili, R., & Parolari, G. (1993). Sensory property relationships to chemical-data of Italian-type dry-cured ham. Journal of Food Science, 58(5), 968–972. doi:10.1111/j.1365-2621.1993.tb06090.x
- Christensen, M., Purslow, P.P., & Larsen, L.M. (2000). The effect of cooking temperature on mechanical properties of whole meat, single muscle fibres and perimysial connective tissue. Meat Science, 55(3), 301–307. doi:10.1016/S0309-1740(99)00157-6
- Cilla, I., Martínez, L., Beltrán, J.A., & Roncalés, P. (2006). Dry-cured ham quality and acceptability as affected by the preservation system used for retail sale. Meat Science, 73(4), 581–589. doi:10.1016/j.meatsci.2006.02.013
- Cordoba, J.J., Antequera, T.A., Garcia, C.G., Ventanas, J.V., Bote, C.L., & Asensio, M.A. (1994). Evolution of free amino-acids and amines during ripening of Iberian cured ham. Journal of Agricultural and Food Chemistry, 42(10), 2296–2301. doi:10.1021/jf00046a040
- Costa-Corredor, A., Serra, X., Arnau, J., & Gou, P. (2009). Reduction of NaCl content in restructured dry-cured hams: Post-resting temperature and drying level effects on physicochemical and sensory parameters. Meat Science, 83(3), 390–397. doi:10.1016/j.meatsci.2009.06.011
- Estévez, M. (2011). Protein carbonyls in meat systems: A review. Meat Science, 89(3), 259–279. doi:10.1016/j.meatsci.2011.04.025
- Etlinger, J.D., Zak, R., & Fischman, D.A. (1976). Compositional studies of myofibrils from rabbit striated muscle. The Journal of Cell Biology, 68(1), 123–141. doi:10.1083/jcb.68.1.123
- Feng, L., Qiao, Y., Zou, Y.F., Huang, M., Kang, Z.L., & Zhou, G.H. (2014). Effect of flavourzyme on proteolysis, antioxidant capacity and sensory attributes of Chinese sausage. Meat Science, 98(1), 34–40. doi:10.1016/j.meatsci.2014.04.001
- Flores, M., Barat, J.M., Aristoy, M.-C., Peris, M.M., Grau, R., & Toldrá, F. (2006). Accelerated processing of dry-cured ham. Part 2. Influence of brine thawing/salting operation on proteolysis and sensory acceptability. Meat Science, 72(4), 766–772. doi:10.1016/j.meatsci.2005.10.008
- Garrido, R., Domínguez, R., Lorenzo, J.M., Franco, I., & Carballo, J. (2012). Effect of the length of salting time on the proteolytic changes in dry-cured lacón during ripening and on the sensory characteristics of the final product. Food Control, 25(2), 789–796. doi:10.1016/j.foodcont.2011.11.036
- Gou, P., Morales, R., Serra, X., Guàrdia, M.D., & Arnau, J. (2008). Effect of a 10-day ageing at 30°C on the texture of dry-cured hams processed at temperatures up to 18°C in relation to raw meat pH and salting time. Meat Science, 80(4), 1333–1339. doi:10.1016/j.meatsci.2008.06.009
- Harkouss, R., Astruc, T., Lebert, A., Gatellier, P., Loison, O., Safa, H., … Mirade, P.-S. (2015). Quantitative study of the relationships among proteolysis, lipid oxidation, structure and texture throughout the dry-cured ham process. Food Chemistry, 166, 522–530. doi:10.1016/j.foodchem.2014.06.013
- Harkouss, R., Safa, H., Gatellier, P., Lebert, A., & Mirade, P.-S. (2014). Building phenomenological models that relate proteolysis in pork muscles to temperature, water and salt content. Food Chemistry, 151, 7–14. doi:10.1016/j.foodchem.2013.10.164
- Huang, F., Huang, M., Xu, X.L., & Zhou, G.H. (2011). Influence of heat on protein degradation, ultrastructure and eating quality indicators of pork. Journal of the Science of Food and Agriculture, 91(3), 443–448. doi:10.1002/jsfa.v91.3
- Ishiwatari, N., Fukuoka, M., & Sakai, N. (2013). Effect of protein denaturation degree on texture and water state of cooked meat. Journal of Food Engineering, 117(3), 361–369. doi:10.1016/j.jfoodeng.2013.03.013
- Jin, G., He, L., Zhang, J., Yu, X., Wang, J., & Huang, F. (2012). Effects of temperature and NaCl percentage on lipid oxidation in pork muscle and exploration of the controlling method using response surface methodology (RSM). Food Chemistry, 131(3), 817–825. doi:10.1016/j.foodchem.2011.09.050
- Jurado, Á., García, C., Timón, M.L., & Carrapiso, A.I. (2007). Effect of ripening time and rearing system on amino acid-related flavour compounds of Iberian ham. Meat Science, 75(4), 585–594. doi:10.1016/j.meatsci.2006.09.006
- Koutsidis, G., Elmore, J.S., Oruna-Concha, M.J., Campo, M.M., Wood, J.D., & Mottram, D.S. (2008). Water-soluble precursors of beef flavour. Part II: Effect of post-mortem conditioning. Meat Science, 79(2), 270–277. doi:10.1016/j.meatsci.2007.09.010
- Lim, J. (2011). Hedonic scaling: A review of methods and theory. Food Quality and Preference, 22(8), 733–747. doi:10.1016/j.foodqual.2011.05.008
- Lorenzo, J.M., Bermúdez, R., Domínguez, R., Guiotto, A., Franco, D., & Purriños, L. (2015). Physicochemical and microbial changes during the manufacturing process of dry-cured lacón salted with potassium, calcium and magnesium chloride as a partial replacement for sodium chloride. Food Control, 50, 763–769. doi:10.1016/j.foodcont.2014.10.019
- Lorenzo, J.M., Fonseca, S., Gómez, M., & Domínguez, R. (2015). Influence of the salting time on physico-chemical parameters, lipolysis and proteolysis of dry-cured foal “cecina”. LWT – Food Science and Technology, 60(1), 332–338. doi:10.1016/j.lwt.2014.07.023
- Ma, H.J., Ledward, D.A., Zamri, A.I., Frazier, R.A., & Zhou, G.H. (2007). Effects of high pressure/thermal treatment on lipid oxidation in beef and chicken muscle. Food Chemistry, 104(4), 1575–1579. doi:10.1016/j.foodchem.2007.03.006
- Martin, L., Antequera, T., Ruiz, J., Cava, R., Tejeda, J.F., & Cordoba, J.J. (1998). Influence of the processing conditions of Iberian ham on proteolysis during ripening. Food Science and Technology International, 4(1), 17–22. doi:10.1177/108201329800400103
- Martı́n, L., Antequera, T., Ventanas, J., Benı́tez-Donoso, R., & Córdoba, J.J. (2001). Free amino acids and other non-volatile compounds formed during processing of Iberian ham. Meat Science, 59(4), 363–368. doi:10.1016/S0309-1740(01)00088-2
- Molina, I., & Toldra, F. (1992). Detection of proteolytic activity in microorganisms isolated from dry-cured ham. Journal of Food Science, 57(6), 1308–1310. doi:10.1111/jfds.1992.57.issue-6
- Mora, L., Gallego, M., Escudero, E., Reig, M., Aristoy, M.-C., & Toldrá, F. (2015). Small peptides hydrolysis in dry-cured meats. International Journal of Food Microbiology, 212, 9–15. doi:10.1016/j.ijfoodmicro.2015.04.018
- Roldan, M., Antequera, T., Armenteros, M., & Ruiz, J. (2014). Effect of different temperature–time combinations on lipid and protein oxidation of sous-vide cooked lamb loins. Food Chemistry, 149, 129–136. doi:10.1016/j.foodchem.2013.10.079
- Rubio-Celorio, M., Garcia-Gil, N., Gou, P., Arnau, J., & Fulladosa, E. (2015). Effect of temperature, high pressure and freezing/thawing of dry-cured ham slices on dielectric time domain reflectometry response. Meat Science, 100, 91–96. doi:10.1016/j.meatsci.2014.10.005
- Ruiz, J., Garcı́a, C., Muriel, E., Andrés, A.I., & Ventanas, J. (2002). Influence of sensory characteristics on the acceptability of dry-cured ham. Meat Science, 61(4), 347–354. doi:10.1016/S0309-1740(01)00204-2
- Salih, A.M., Smith, D.M., Price, J.F., & Dawson, L.E. (1987). Modified extraction 2-thiobarbituric acid method for measuring lipid oxidation in poultry. [Article]. Poultry Science, 66(9), 1483–1488. doi:10.3382/ps.0661483
- Sánchez-Molinero, F., & Arnau, J. (2014). Effects of the applications of oil drip onto surface and of the use of a temperature of 35°C for 4 days on some physicochemical, microbiological and sensory characteristics of dry-cured ham. Meat Science, 98(2), 81–87. doi:10.1016/j.meatsci.2014.03.021
- Sforza, S., Pigazzani, A., Motti, M., Porta, C., Virgili, R., Galaverna, G., … Marchelli, R. (2001). Oligopeptides and free amino acids in Parma hams of known cathepsin B activity. Food Chemistry, 75(3), 267–273. doi:10.1016/S0308-8146(01)00224-2
- Shackelford, S.D., Wheeler, T.L., Meade, M.K., Reagan, J.O., Byrnes, B.L., & Koohmaraie, M. (2001). Consumer impressions of tender select beef. [Article]. Journal of Animal Science, 79(10), 2605–2614.
- Sun, W., Cui, C., Zhao, M., Zhao, Q., & Yang, B. (2011). Effects of composition and oxidation of proteins on their solubility, aggregation and proteolytic susceptibility during processing of Cantonese sausage. Food Chemistry, 124(1), 336–341. doi:10.1016/j.foodchem.2010.06.042
- Toldra, F. (1998). Proteolysis and lipolysis in flavour development of dry-cured meat products. [Article; Proceedings Paper]. Meat Science, 49, S101–S110. doi:10.1016/s0309-1740(98)90041-9
- Toldrá, F., Flores, M., & Sanz, Y. (1997). Dry-cured ham flavour: Enzymatic generation and process influence. Food Chemistry, 59(4), 523–530. doi:10.1016/S0308-8146(97)00013-7
- Toldrá, F., Rico, E., & Flores, J. (1992). Activities of pork muscle proteases in model cured meat systems. Biochimie, 74(3), 291–296. doi:10.1016/0300-9084(92)90128-2
- Ventanas, S., Estévez, M., Delgado, C.L., & Ruiz, J. (2007). Phospholipid oxidation, non-enzymatic browning development and volatile compounds generation in model systems containing liposomes from porcine Longissimus dorsi and selected amino acids. European Food Research and Technology, 225(5–6), 665–675. doi:10.1007/s00217-006-0462-2
- Virgili, R., Saccani, G., Gabba, L., Tanzi, E., & Soresi Bordini, C. (2007). Changes of free amino acids and biogenic amines during extended ageing of Italian dry-cured ham. LWT – Food Science and Technology, 40(5), 871–878. doi:10.1016/j.lwt.2006.03.024
- Visessanguan, W., Benjakul, S., Riebroy, S., & Thepkasikul, P. (2004). Changes in composition and functional properties of proteins and their contributions to Nham characteristics. Meat Science, 66(3),579– 588. doi:10.1016/s0309-1740(03)00172-4
- Waade, C., & Stahnke, L.H. (1997). Dried sausages fermented with staphylococcus xylosus at different temperatures and with different ingredient levels. Part IV. Amino acid profile. Meat Science, 46(1), 101–114. doi:10.1016/S0309-1740(96)00089-7
- Wang, Q., Zhao, X., Ren, Y., Fan, E., Chang, H., & Wu, H. (2013). Effects of high pressure treatment and temperature on lipid oxidation and fatty acid composition of yak (Poephagus grunniens) body fat. Meat Science, 94(4), 489–494. doi:10.1016/j.meatsci.2013.03.006
- Wenjiao, F., Yongkui, Z., Yunchuan, C., Junxiu, S., & Yuwen, Y. (2014). TBARS predictive models of pork sausages stored at different temperatures. Meat Science, 96(1), 1–4. doi:10.1016/j.meatsci.2013.06.025
- Yun, J., Shahidi, F., Rubin, L.J., & Diosady, L.L. (1987). Oxidative stability and flavour acceptability of nitrite-free meat-curing systems. Canadian Institute of Food Science and Technology Journal–Journal De L Institut Canadien De Science Et Technologie Alimentaires, 20(4), 246–251. doi:10.1016/S0315-5463(87)71195-X
- Zhang, J., Jin, G., Wang, J., & Zhang, W. (2011). Effect of intensifying high-temperature ripening on lipolysis and lipid oxidation of Jinhua ham. LWT – Food Science and Technology, 44(2), 473–479. doi:10.1016/j.lwt.2010.07.007
- Zhang, L., Lin, Y.H., Leng, X.J., Huang, M., & Zhou, G.H. (2013). Effect of sage (Salvia officinalis) on the oxidative stability of Chinese-style sausage during refrigerated storage. Meat Science, 95(2), 145–150. doi:10.1016/j.meatsci.2013.05.005
- Zhao, G.M., Zhou, G.H., Wang, Y.L., Xu, X.L., Huan, Y.J., & Wu, J.Q. (2005). Time-related changes in cathepsin B and L activities during processing of Jinhua ham as a function of pH, salt and temperature. Meat Science, 70(2), 381–388. doi:10.1016/j.meatsci.2005.02.004