ABSTRACT
This study determined the activities of Bidens pilosa and Moringa oleifera leaf extracts on microbial count of ground beef during 6-day cold storage. Fresh ground beef sample was treated with butylated hydroxytoluene (BHT) (0.2 g/kg), M. oleifera (ML, 1 g/kg) and B. pilosa (BP, 1 g/kg) leaf extracts and compared with the control. The result of the phytochemical contents revealed that ML extract had higher phenolic and flavonoid contents than BP extract (p > 0.05). The antibacterial assay of the extracts revealed an appreciable broad-spectrum activity against tested bacteria with minimum inhibitory concentrations between 0.6 and 10.0 mg/mL. Addition of ML leaf extract to ground beef sample lowered total viable and lactic acid bacteria (p < 0.05) counts than control and BHT treatments at day 3 of storage. These results suggest that ML leaf extract could be used as potential sources of natural antimicrobial agent in meat products.
RESUMEN
Este estudio determinó las actividades de los extractos de hoja de B. pilosa y M. oleifera en los recuentos microbianos de ternera triturada durante 6 días de almacenamiento en frío. Se trató con BHT una muestra fresca de ternera triturada (0,2 g/kg) y extractos de hoja M. oleifera (ML, 1 g/kg) y B. pilosa (BP, 1 g/kg), entonces estos fueron contrastados con la muestra control. Los resultados de los contenidos fitoquímicos revelaron que el extracto de ML tenía un mayor contenido fenólico y de flavonoides en comparación con el extracto de BP (p > 0,05). El ensayo antibacteriano de los extractos reveló una apreciable actividad de amplio espectro frente a las bacterias examinadas con concentraciones inhibitorias mínimas utilizando rangos entre 0,6 y 10,0 mg/mL. La adición de extracto de hoja de ML a la muestra de ternera triturada disminuyó el recuento total viable de bacteria ácido-láctica (p < 0,05) más que en la muestra control y los tratamientos de BHT en tercer día de almacenamiento. Estos resultados sugieren que el extracto de hoja de ML podría ser utilizado como fuente potencial de agente antimicrobiano natural en los productos cárnicos.
Introduction
Meat preservatives play important roles in the delivery of high-quality and safe meat and meat products to consumers by acting as antimicrobials and antioxidants. Meat preservatives prevent oxidation, enzymatic reaction and growth of foodborne and pathogenic microorganisms that cause deterioration, nutritional and economic losses to meat industries (Falowo, Fayemi, & Muchenje, Citation2014; Sánchez-Ortega et al., Citation2014). Presently, most consumers are looking for processed food without the addition of chemical preservatives, such as butylated hydroxytoluene (BHT), tertiary-butylhydroquinone and butylated hydroxyanisole, because of their toxicity and health-associated risk (Lobo, Patil, Phatak, & Chandra, Citation2010; NTP, Citation2011). Conversely, the use of synthetic preservatives have also been found to be either ineffective to completely delay microbial spoilage or eliminate important pathogens such as Listeria monocytogenes in meat products (Gutierrez, Barry-Ryan, & Bourke, Citation2009; Tajkarimi, Ibrahim, & Cliver, Citation2010). Nowadays, numerous edible medicinal plants have been identified as alternative to chemical preservatives; these medicinal plants are known to exhibit secondary metabolites including antioxidant and antimicrobial compounds that promote good health (Jaberian, Piri, & Nazari, Citation2013). Recent studies on the use of medicinal plants as preservatives in meat and meat products have shown that they can extend meat shelf-life by reducing the growth of pathogenic and spoilage microorganisms (Kobus-Cisowska, Flaczyk, Rudzińska, & Kmiecik, Citation2014; Kozłowska, Ścibisz, Zaręba, & Ziarno, Citation2015).
Biden pilosa and Moringa oleifera are plants with a lot of potentials in this regard. Both plants are considered as potential functional ingredients and promising sources of natural antioxidants (Bartolome, Villaseñor, & Yang, Citation2013; Sreelatha & Padma, Citation2009). Conventionally, almost all parts of these plants (the whole plant, the aerial parts and/or the roots) have been used on an extensive basis, either as food or medicinal components (Yang, Citation2014). The leaves are particularly rich in protein, essential amino acids and other micronutrients (Adedapo, Jimoh, & Afolayan, Citation2011; Moyo, Masika, Hugo, & Muchenje, Citation2011; Yassa & Tohamy, Citation2014). The extracts of both plants have been identified to possess several functional properties, such as antioxidant, antihypertensive, antimicrobial, antifungal, antiulcer, antitumor and anticancer activities (Bartolome et al., Citation2013). The in vitro activity of plant extracts have also been reported against several foodborne and human pathogenic microorganisms including Klebsiella, Bacillus, Pseudomonas, Staphylococcus, Salmonella and Escherichia coli (Khan, Kihara, & Omoloso, Citation2001; Rahman et al., Citation2010). However, the potential of M. oleifera and B. pilosa as alternative natural preservatives in meat products has not been fully studied. The preservative effect of medicinal plants is apparently related to the presence of polyphenols in the plants (Kim et al., Citation2013). Therefore, the objective of this study was to evaluate the antimicrobial activities of M. oleifera and B. pilosa leaf extracts in ground beef.
Materials and methods
Plant sample and extract preparation
B. pilosa and M. oleifera leaf stalk were obtained from the University of Fort Hare farm (South Africa) and Moringa South Africa Ltd., respectively. The dry plant samples (200 g) were exhaustively macerated with 800 mL of ethanol–water solution (7:3) at room temperature for 2 days with agitation. Each extract was separated from the residue by filtration using Whatman no. 1 filter paper and then concentrated under reduced pressure at 55°C using a rotary evaporator. The extract solvent was removed by freeze-drying and the dried extracts were used for the determination of the antioxidant activity at concentration of 1 mg/mL. Determination of the nutritive values of the plants was carried out on the dry samples. All analyses were done in triplicate. The dried powder of plant extracts were then stored at 20°C for further analysis.
Determination of total phenolic and flavonoid content in plant extracts
Total phenol contents of the extracts were determined as described by Wolfe, Wu, and Liu (Citation2003). The extract was mixed with 5 mL of Folin–Ciocalteu reagent (previously diluted with water 1:10 v/v) and 4 mL (75 g/L) of sodium carbonate. The tubes were vortexed for 15 s and allowed to stand for 30 min at 40°C for color development. Absorbance was then measured at 765 nm using the Hewlett-Packard UV–VIS spectrophotometer. Total phenolic contents were expressed as milligram per gram gallic acid equivalent (GAE) using the following equation based on the calibration curve: y = 0.181x, r2 = 0.993, where x was the absorbance and y was the GAE (mg/g).
Total flavonoids were determined using the method described by Ordonez, Gomez, Vattuone, and Isla (Citation2006). An aliquot of 0.5 mL of 2% AlCl3 ethanol solution was added to 0.5 mL of sample solution. The samples were incubated for 1 h at room temperature, followed by measuring the absorbance at 420 nm. A yellow color indicated the presence of flavonoids. Total flavonoid contents were calculated as rutin (Ru) (mg/g) using the following equation based on the calibration curve: y = 0.2645x, r2 = 0.992, where x was the absorbance and y was the Ru equivalent (mg/g).
In vitro antimicrobial activity of plant extracts
Bacterial strains and growth conditions
Bacterial isolates used in this study were reference strains obtained from GI Microbiology and Biotechnology laboratory, Agricultural Research Council-Animal Production Institute, Irene, South Africa. These strains were chosen for their histories in pathological effects on humans and deterioration of food products. The bacteria strains include four gram-positive and four gram-negative strains (). The test organisms were inoculated on 10 mL previously sterilized nutrient agar media, mixed thoroughly and transferred immediately to the sterile petri dish in an aseptic condition using a sterile loop. The bacterial strains were incubated at 37°C overnight. After incubation, the test organisms were maintained on nutrient broth, and then standardized at 560 nm to achieve 105 colony-forming units per mL (CFU/mL).
Table 1. Antioxidant content of M. oleifera and B. pilosa leaf extracts.
Tabla 1. Contenido antioxidante de los extractos de hoja de Moring oleifera y Biden pilosa.
Table 2. Antimicrobial activity of B. pilosa and M. oleifera plant extracts (20 mg/mL).
Tabla 2. Actividad antimicrobiana de los extractos de plantas B. pilosa y M. oleifera (20 mg/ml).
Agar well diffusion test
The inhibitory effects of the plant extracts on the test bacteria were determined by the agar well diffusion method. Twenty milliliters of Müeller-Hinton agar solution in McCartney bottles were autoclaved and cooled in a water bath at 50°C. One hundred microliters of each standardized bacteria strain were added to the suspension and poured into a sterile petri dish (90 mm diameter), and then allowed to stand at room temperature to reach solidification. Two wells per petri dish were bored into the agar plate under aseptic condition and 20 mg/mL of each plant extract were dispensed into each agar well. Plates were left for 30 min at room temperature to allow the extracts to diffuse into the agar before incubation at 37°C for 24 h. After incubation, clear zone of inhibitions were measured and expressed in millimeter.
Determination of minimum inhibitory concentration of the plant extracts
The minimum inhibitory concentration (MIC) of the extracts was determined by a broth microdilution method as described by Jorgensen and Turnidge (Citation2007). Ninety-six well culture plates were prepared, and serial twofold dilutions of the extracts were dispensed into the plate wells. The volume of dispensed extract was 100 µL per well in the concentration range of 20 to 0.625 mg/mL. The same volume (100 µL) of bacterial culture at a density of 105 CFU/mL was added to the wells, and the culture plates were incubated at 37°C for 24 h. The lowest concentration of the plant extract required to inhibit visible growth of the tested microorganism was designated as the MIC. The minimum bactericidal concentration (MBC) was determined by streaking the suspension in the well with concentrations greater than the MIC. After that, the subcultured agar plates were incubated overnight at 37°C. The MBC was defined as the lowest concentration of extract at which no viable microorganism was detected by subculture.
Preparation of meat sample
Fresh beef samples (Muscularis longissimus thoracis et lumborum, LTL) were obtained from 40 nondescript cattle at high throughput commercial abattoir and processed after 48 h postmortem. Beef samples were cut into small cubes after removal of visible fat and connective tissues, and minced in a sterile meat grinder (CombinMax600, China). A portion (1200 g) of the ground beef was randomly assigned to one of the following treatments: (1) NC (negative control, meat without additives); (2) BP (meat with 1 g/kg B. pilosa extract); (3) ML (meat with 1 g/kg M. oleifera extract), (4) BPML (meat with 1 g/kg B. pilosa and M. oleifera extract) and (5) PC (positive control, meat with 0.2 g/kg BHT). Immediately after adding the extracts and BHT, the ground beef samples were aerobically packed in polyethylene bags (O2 permeability = 6000–8000 cm3/(24 h × m2 × atm), water vapor transmission = 83 g/(24 h × m2) and 50% relative humidity) and stored at 4 ± 1°C for 6 days and then analyzed for microbial counts.
Microbiological quality of the ground beef
Samples for microbial analysis were taken immediately after addition of extracts and again after 3 and 6 days of refrigerated storage for determination of total viable counts (TVCs) and lactic acid bacteria (LAB). Ten grams of meat sample was homogenized with 90 mL of 0.1% sterile buffered peptone water (Oxoid CM0509) in a Stomacher bag (Seward 400 Circulator) for 2 min at room temperature. For each sample, appropriate serial 10-fold dilutions were prepared by diluting 1 mL of homogenate in 9 mL of 0.1% peptone water. The pour-plate method was used for the determination of microbial counts. TVCs were determined using a standard plate count agar (Oxoid CM0463) after incubation for 48 h at 37°C. Determination of LAB was carried out on deMan, Rogosa and Sharpe (MRS; Oxoid CM0359) medium after 72 h incubation at 30°C. Microbiological counts were expressed as the log10 of CFU per gram of ground beef (log CFU/g). All data presented are the mean values of three replicates.
Statistical analysis
Data obtained on antioxidant and antimicrobial contents of the plant extracts were analyzed using Student’s t-test and PROC ANOVA procedures of the Statistical Analysis System (SAS, version 1.9.3 of 2007). Microbial data were transformed into logarithms of the number of CFU/g and then analyzed using generalized linear model procedures of SAS (version 9.1.3 of 2007) with plant extracts as source of variations. Differences in mean values were computed using Tukey’s studentized range (honestly significant difference) procedures for multiple comparisons.
Results and discussion
Antioxidant capacity of the plant extract
The result of phenol and flavonoid contents of the plant extracts are presented in . The total phenol (77.5 ± 0.94 mg GAE/g dry weight (DW)) and flavonoid (17.4 ± 0.15 mg Ru/g DW) content of M. oleifera extracts were numerically higher than the extract of B. pilosa (75.9 ± 0.08 mg GAE/g DW) and (14.9 ± 0.03 mg Ru/g DW) for phenol (p > 0.05) and flavonoids (p < 0.05), respectively. Intrinsically, phenolic and flavonoid compounds from plant extracts have been considered to possess antioxidant and antimicrobial properties (Cowan, Citation1999; Koolen, da Silva, Gozzo, de Souza, & de Souza, Citation2013; Oyedemi, Oyedemi, Arowosegbe, & Afolayan1, Citation2012). They have also been shown to possess redox properties which play an important role in absorbing and neutralizing free radicals, quenching singlet and triplet oxygen, or decomposing peroxides (Adedapo et al., Citation2011; Moyo, Oyedemi, Masika, & Muchenje, Citation2012). However, these results were slightly higher than those reported by Cortes-Rojas, Chagas-Paula, Da Costa, Souza, and Oliveira (Citation2013), Sreelatha and Padma (Citation2009), Sultana, Anwar, and Ashraf (Citation2009) and Ogbunugafor et al. (Citation2012). The difference could be attributed to variation in the environment where the plant was collected, the season, the physiological stage of the plant when they were harvested and extraction method (Taylor & Van Staden, Citation2001).
Antimicrobial activity of M. oleifera and B. pilosa leaf extracts
The result of the in vitro antimicrobial activity of the extracts obtained from M. oleifera and B. pilosa against the tested organisms are shown in . The extracts showed greater inhibitory effect against gram-negative bacteria than gram-positive bacteria strains. The inhibitory activity of M. oleifera and B. pilosa extracts against the test organism ranged from 9 to 19 mm and 12–17 mm, respectively. The best inhibitory effect of the extracts was observed against E. coli (ML 19 mm, BP 16 mm) and Shigella flexinerii (ML 18 mm, BP 17 mm). However, the extract of M. oleifera was found inactive against Bacillus cereus and Serratia marcescens while that of B. pilosa was inactive against Pseudomonas aeruginosa and S. marcescens. The resistant capability of these bacteria (B. cereus, P. aeruginosa and S. marcescens) against the extracts could be attributed to their cell wall or outward membrane structure, which has been reported to restrict the penetration of the plant extract (Biswas, Rogers, McLaughlin, Daniels, & Yadav, Citation2013; Hayek & Ibrahim, Citation2012; Nisa et al., Citation2013). Other work has also reported the resistance of S. marcescens and P. aeruginosa bacteria to plant extracts (Escalona-Arranz et al., Citation2010; Koolen et al., Citation2013). The MIC of extracts against test organisms ranged from 0.6 to 10 mg/mL with B. pilosa showing higher activity against Staphylococcus epidermidis at 0.6 mg/mL (). The highest MBC was observed at 5 mg/mL for both extracts (). The inhibitory effect, MIC and MBC of the extract against pathogenic bacteria strains indicated that these plants are potential candidate of antimicrobial sources.
Effect of M. oleifera and B. pilosa leaf extracts on microbial quality of raw ground beef during storage at 4°C
and show the effect of plant extracts and storage time on microbial quality of raw ground beef. The initial TVC across the treatment ranges from 6.27 ± 0.37 to 6.78 ± 0.32 log CFU/g from day 0 to 3 (p > 0.05). However, at day 3, the control group (5.80 ± 1.05 log CFU/g) and BP treatment (5.86 ± 0.19 log CFU/g) had the highest (p < 0.05) total bacterial count, followed by the BHT treatment (5.30 ± 5.58 log CFU/g) and the least in beef containing MLBP (5.06 ± 0.75 log CFU/g) and ML (5.28 ± 0.53 log CFU/g) extracts. A similar trend was also observed for the LAB count across the treatments. At day 0, the LAB count ranged from 3.07 ± 0.24 to 4.62 ± 0.53 log CFU/g. While at day 3, beef samples containing ML (3.17 ± 0.43 log CFU/g) and MLBP (1.66 ± 0.24 log CFU/g) extracts demonstrated the lowest LAB count compared to the control (3.45 ± 0.30 log CFU/g) (p < 0.05). This is in accordance with the findings of Kim et al. (Citation2013) who reported a great reduction in microbial count of raw beef patties treated with natural antioxidants. The antimicrobial activities of plant extracts have been linked to the presence of the bioactive compounds, such as phenol and flavonoid, which have been reported to form to complexes with extracellular and soluble proteins and bacterial cell walls, although their mode of mechanism has not been fully understood (Kim et al., Citation2013; Krishnan et al., Citation2014). However, the application of BP extracts at concentration of 1 g/kg did not decrease the TVC and LAB counts of the beef samples during the storage days compared to control. At day 6, LAB counts were not statistically affected by the extract compared to the negative control and the BHT treatment. This is indicating that the use of extracts at concentration of 1 g/kg could not inhibit the growth of microbes beyond day 3. Similar results have been reported by Jin, Ha, and Choi (Citation2015) who found that the addition of Caesalpinia sappan L. extract at 0.05% and 0.1% to pork sausage did not reduce the microbial counts compared to the control during a 4-week cold storage.
Figure 3. Effect of Moringa oleifera (ML) and Biden pilosa (BP) leaf extracts on total viable count of ground beef during storage at 4°C (p > 0.05).
Figura 3. Efectos de los extractos de hoja de Moringa oleifera (ML) y Biden pilosa (BP) en el recuento total viable de ternera triturada durante almacenamiento a 4°C (p > 0,05).
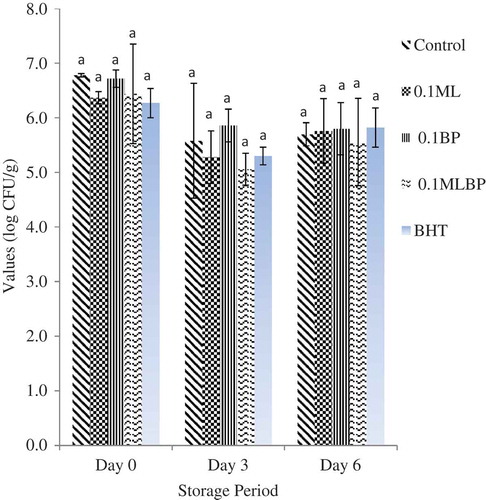
Figure 4. Effect of Moringa oleifera (ML) and Biden pilosa (BP) leaf extracts on lactic acid bacteria count of ground beef during storage at 4°C (p < 0.05).
Figura 4. Efecto de los extractos de hoja de Moringa oleifera (ML) y Biden pilosa (BP) en el recuento de bacteria láctica de ternera triturada durante almacenamiento a 4°C (p < 0,05).
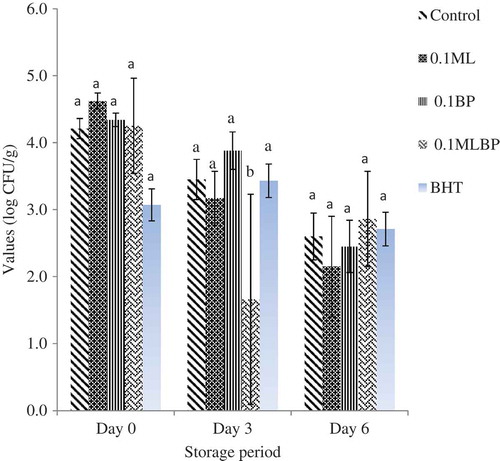
However, the gradual decrease in the bacterial counts with the increase in storage day in this study could be due to some intrinsic factors such as high proteins and fat content coupled with other extrinsic factors, such as temperature and oxygen, which have been reported to affect the behavior of bacteria in food systems. Reports have shown that food protein and fat contents can bind and/or solubilize phenolic compounds to reduce their availability for antimicrobial activity during storage (El Abed et al., Citation2014). Similar result has also been reported by Bhaduri and Cottrell (Citation2004) who found a sharp decrease in TVCs of ground chicken refrigerated at 4°C for 7 days.
Conclusion
The in vitro antimicrobial assay showed that the B. pilosa and M. oleifera possess potential antibacterial activity against the tested organisms, particularly against gram-negative bacteria. The presence of these phenolic and flavonoid compounds at concentration more than 1 g/kg make M. oleifera a potential source of bioactive compounds for meat preservation during cold storage. In addition, the application of M. oleifera extracts in ground beef revealed that this plant is a promising candidate as natural antimicrobial agent in meat industry.
Disclosure statement
No potential conflict of interest was reported by the authors.
Additional information
Funding
References
- Adedapo, A., Jimoh, F., & Afolayan, A. (2011). Comparison of the nutritive value and biological activities of the acetone, methanol and water extracts of the leaves of Bidens pilosa and Chenopodium album. Acta Poloniae Pharmaceutica Drug Research, 68(1), 83–92.
- Bartolome, A.P., Villaseñor, I.M., & Yang, W. (2013). Bidens pilosa L. (Asteraceae): Botanical properties, traditional uses, phytochemistry, and pharmacology. Evidence-Based Complementary and Alternative Medicine, 2013, 1–51. doi:10.1155/2013/340215
- Bhaduri, S., & Cottrell, B. (2004). Survival of cold-stressed Campylobacter jejuni on ground chicken and chicken skin during frozen storage. Applied and Environmental Microbiology, 70(12), 7103–7109. doi:10.1128/AEM.70.12.7103-7109.2004
- Biswas, B., Rogers, K., McLaughlin, F., Daniels, D., & Yadav, A. (2013). Antimicrobial activities of leaf extracts of guava (Psidium guajava L.) on two gram-negative and gram-positive bacteria. International Journal of Microbiology, 2013, 1–7. doi:10.1155/2013/746165
- Cortes-Rojas, D.F., Chagas-Paula, D.A., Da Costa, F.B., Souza, C.R.F., & Oliveira, W.P. (2013). Bioactive compounds in Bidens pilosa L. populations: A key step in the standardization of phytopharmaceutical preparations. Brazilian Journal of Pharmacognosy, 23(1), 28–35.
- Cowan, M. (1999). Plant products as antimicrobial agents. Clinical Microbiology Reviews, 12, 564.
- El Abed, N., Kaabi, B., Smaali, M.I., Chabbouh, M., Habibi, K., Mejri, M., … Ahmed, S.B.H. (2014). Chemical composition, antioxidant and antimicrobial activities of Thymus capitata essential oil with its preservative effect against Listeria monocytogenes inoculated in minced beef meat. Evidence-Based Complementary and Alternative Medicine, 2014, 1–11. doi:10.1155/2014/152487
- Escalona-Arranz, J.C., Péres-Roses, R., Urdaneta-Laffita, I., Camacho-Pozo, M.I., Rodríguez-Amado, J., & Licea-Jiménez, I. (2010). Antimicrobial activity of extracts from Tamarindus indica L. leaves. Pharmacognosy Magazine, 6(23), 242–247. doi:10.4103/0973-1296.66944
- Falowo, A.B., Fayemi, P.O., & Muchenje, V. (2014). Natural antioxidants against lipid–protein oxidative deterioration in meat and meat products: A review. Food Research International, 64, 171–181. doi:10.1016/j.foodres.2014.06.022
- Gutierrez, J., Barry-Ryan, C., & Bourke, P. (2009). Antimicrobial activity of plant. Essential oils using food model media: Efficacy, synergistic potential and interactions with food components. Food Microbiology, 26, 142–150. doi:10.1016/j.fm.2008.10.008
- Hayek, S.A., & Ibrahim, S.A. (2012). Antimicrobial activity of Xoconostle pears (Opuntiamatudae) against Escherichia coli O157: H7in laboratory medium. International Journal of Microbiology, 2012, 1–6. doi:10.1155/2012/368472
- Jaberian, H., Piri, K., & Nazari, J. (2013). Phytochemical composition and in vitro antimicrobial and antioxidant activities of some medicinal plants. Food Chemistry, 136, 237–244. doi:10.1016/j.foodchem.2012.07.084
- Jin, S., Ha, S., & Choi, J. (2015). Effect of Caesalpinia sappan L. extract on physico-chemical properties of emulsion-type pork sausage during cold storage. Meat Science, 110, 245–252. doi:10.1016/j.meatsci.2015.08.003
- Jorgensen, J.H., & Turnidge, J.D. (2007). Susceptibility test methods: Dilution and disk diffusion methods. In P.R. Murray, E.J. Baron, J.H. Jorgensen, M.L. Landry, & M.A. Pfaller (Eds.), Manual of clinical microbiology (9th ed., pp. 1152–1172). Washington, DC: ASM Press.
- Khan, M.R., Kihara, M., & Omoloso, A.D. (2001). Antimicrobial activity of Bidens pilosa, Bischofia javanica, Elmerillia papuana and Sigesbekia orientalis. Fitoterapia, 72(6), 662–665. doi:10.1016/S0367-326X(01)00261-1
- Kim, S.-J., Min, S.C., Shin, H.-J., Lee, Y.-J., Cho, A.R., Kim, S.Y., & Han, J. (2013). Evaluation of the antioxidant activities and nutritional properties of ten edible plant extracts and their application to fresh ground beef. Meat Science, 93, 715–722. doi:10.1016/j.meatsci.2012.11.029
- Kobus-Cisowska, J., Flaczyk, E., Rudzińska, M., & Kmiecik, D. (2014). Antioxidant properties of extracts from Ginkgo biloba leaves in meatballs. Meat Science, 97, 174–180. doi:10.1016/j.meatsci.2014.01.011
- Koolen, H.H.F., da Silva, F.M.A., Gozzo, F.C., de Souza, A.Q.L., & de Souza, A.D.L. (2013). Antioxidant, antimicrobial activities and characterization of phenolic compounds from buriti (Mauritia flexuosa L. f.) by UPLC–ESI-MS/MS. Food Research International, 51, 467–473. doi:10.1016/j.foodres.2013.01.039
- Kozłowska, M., Ścibisz, I., Zaręba, D., & Ziarno, M. (2015). Antioxidant properties and effect on lactic acid bacterial growth of spice extracts. CyTA – Journal of Food, 13(4), 573–577.
- Krishnan, K.R., Babuskina, S., AzhaguSaravanaBabu, P., Sasikala, M., Sabina, K., Archana, G., … Sukumar, M. (2014). Antimicrobial and antioxidant effects of spice extracts on the shelf life extension of raw chicken meat. International Journal of Food Microbiology, 171, 32–40. doi:10.1016/j.ijfoodmicro.2013.11.011
- Lobo, V., Patil, A., Phatak, A., & Chandra, N. (2010). Free radicals, antioxidants and functional foods: Impact on human health. Pharmacognosy Reviews, 4(8), 118–126. doi:10.4103/0973-7847.70902
- Moyo, B., Masika, P.J., Hugo, A., & Muchenje, V. (2011). Nutritional characterization of Moringa (Moringa oleifera Lam.) leaves. African Journal of Biotechnology, 10(60), 12925–12933.
- Moyo, B., Oyedemi, S., Masika, P.J., & Muchenje, V. (2012). Polyphenolic content and antioxidant properties of Moringa oleifera leaf extracts and enzymatic activity of liver from goats supplemented with Moringa oleifera leaves/sunflower seed cake. Meat Science, 91, 441–447. doi:10.1016/j.meatsci.2012.02.029
- National Toxicology Program, Department of Health and Human Services (NTP). (2011). Butylated hydroxyanisole, report on carcinogens, twelfth edition. Retrieved October 12, 2014, from http://www.ntp.niehs.nih.gov/go/roc
- Nisa, H., Kamili, A.N., Bandh, S.A., Amin, S., Lone, B.A., & Parray, J.A. (2013). Phytochemical screening, antimicrobial and antioxidant efficacy of different extracts of Rumex dentatus L. – A locally used medicinal herb of Kashmir Himalaya. Asian Pacific Journal of Tropical Disease, 3(6), 434–440. doi:10.1016/S2222-1808(13)60097-3
- Ogbunugafor, H., Igwo-Ezikpe, M., Igwilo, I., Ozumba, N., Adenekan, S., Ugochukwu, C., … Ekechi, A. (2012). In vitro and in vivo evaluation of antioxidant properties of Moringa oleifera ethanolic leaves extract and effect on serum lipid indices in rat. Macedonian Journal of Medical Sciences, 5(4), 397–403.
- Ordonez, A.A.L., Gomez, J.G., Vattuone, M.A., & Isla, M.I. (2006). Antioxidant activities of Sechium edule (Jacq.) Swart extracts. Food Chemistry, 97, 452–458.
- Oyedemi, S.O., Oyedemi, B.O., Arowosegbe, S., & Afolayan1, A.J. (2012). Phytochemicals analysis and medicinal potentials of hydroalcoholic extract from Curtisia dentata (Burm.f) C.A. Sm stem bark. International Journal of Molecular Sciences, 13(12), 6189–6203. doi:10.3390/ijms13056189
- Rahman, M.M., Rahman, M.M., Akhter, S., Jamal, M.A., Pandeya, D.R., Haque, M.A., … Rahman, A. (2010). Control of coliform bacteria detected from diarrhea associated patients by extracts of Moringa oleifera. Nepal Medical College Journal, 12(1), 12–19.
- Sánchez-Ortega, I., García-Almendárez, B.E., Santos-López, E.M., Amaro-Reyes, A., Barboza-Corona, J.E., & Regalado, C. (2014). Antimicrobial edible films and coatings for meat and meat products preservation. The Scientific World Journal, 2014, 1–18. doi:10.1155/2014/248935
- Sreelatha, S., & Padma, P.R. (2009). Antioxidant activity and total phenolic content of Moringa oleifera leaves in two stages of maturity. Plant Foods for Human Nutrition, 64, 303–311. doi:10.1007/s11130-009-0141-0
- Sultana, B., Anwar, F., & Ashraf, M. (2009). Effect of extraction solvent/technique on the antioxidant activity of selected medicinal plant extracts. Molecules, 14, 2167–2180. doi:10.3390/molecules14062167
- Tajkarimi, M.M., Ibrahim, S.A., & Cliver, D.O. (2010). Antimicrobial herb and spice compounds in food. Food Control, 21, 1199–1218. doi:10.1016/j.foodcont.2010.02.003
- Taylor, J.L.S., & Van Staden, J. (2001). The effect of age, season and growth conditions on anti-inflammatory activity in Eucomis autumnalis (Mill.) Chitt. Plant extracts. Plant Growth Regulation, 34(1), 39–47. doi:10.1023/A:1013366926113
- Wolfe, K., Wu, X., & Liu, R.H. (2003). Antioxidant activity of apple peels. Journal of Agricultural and Food Chemistry, 51, 609–614. doi:10.1021/jf020782a
- Yang, W. (2014). Botanical, pharmacological, phytochemical, and toxicological aspects of the antidiabetic plant Bidens pilosa L. Evidence-Based Complementary and Alternative Medicine, 2014, 1–14.
- Yassa, H.D., & Tohamy, A.F. (2014). Extract of Moringa oleifera leaves ameliorates streptozotocin-induced diabetes mellitus in adult rats. Acta Histochemica, 116, 844–854. doi:10.1016/j.acthis.2014.02.002