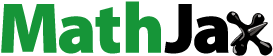
ABSTRACT
The aim of this work was to evaluate the effects of oily tomato extract (OTE) at concentrations of 0, 1, 3 and 5% on the physicochemical and antioxidant properties of gelatin-based films. Characterization of the extract showed lycopene and β-carotene concentrations of 159.15 and 20.38 μg/mL, respectively. The results showed that the addition of OTE was associated with a decrease in moisture content, a lower solubility, a lower water vapor permeability, and a lower Young’s modulus, while the elongation percentage showed a significant increase. The addition of OTE improved the barrier properties of the films against UV and visible light. The higher the OTE concentration was, the greater the amounts of lycopene and β-carotene within the films, and therefore, the higher the antioxidant activity was (2.49 μM Trolox equivalents per g of film). The results suggest that the addition of OTE to gelatin-based films can improve their mechanical properties and increase their antioxidant capacity.
RESUMEN
El objetivo de este trabajo fue evaluar el efecto del extracto oleoso de tomate (OTE) a 0, 1, 3 y 5% sobre las propiedades fisicoquímicas y antioxidantes de películas a base de gelatina. En la caracterización del extracto se encontró una concentración de licopeno y β-caroteno de 159.15 y 20.38 µg/mL respectivamente. Los resultados mostraron que la adición del OTE provocó una reducción en el contenido de humedad, menor solubilidad, baja permeabilidad al vapor de agua y menor módulo de Young, mientras que el porcentaje de elongación presentó un aumento significativo. La incorporación de OTE mejoraron las propiedades de barrera a la luz UV-visible. A mayor concentración de OTE, se observó mayor presencia de licopeno y β-caroteno en las películas y por lo tanto una mayor actividad antioxidante (2.49 µM ET/g de película). Los resultados sugieren que la adición del OTE en las películas de gelatina pueden mejorar las propiedades mecánicas e incrementar la capacidad antioxidante.
1. Introduction
In recent years, several innovative technological strategies have been developed to prolong the useful life of foods while maintaining their quality and ensuring microbial safety. These technologies include the use of edible films and coatings based on biopolymers such as proteins, carbohydrates, and lipids as an effective alternative of food packaging in terms of environmental sustainability and consumer acceptability (Etxabide, Uranga, Guerrero, & de la Caba, Citation2017; Norajit, Kim, & Ryu, Citation2010; Tongnuanchan, Benjakul, & Prodpran, Citation2012). Among the various biopolymers, proteins have great potential due to their abundance, low cost and good film-forming capacity. However, protein-based films are very rigid and inflexible, with poor mechanical, transport and solubility properties. Therefore, plasticizers and short-chain hydrophobic substances have been incorporated to improve said properties (Cerqueira, Souza, Teixeira, & Vicente, Citation2012; Vieira, Da Silva, Dos Santos, & Beppu, Citation2011). The most common plasticizers used in protein-based films are polyethylene glycol, glycerol and sorbitol; these compounds, separately or in combination, act by decreasing the intermolecular forces of the film, resulting in greater mobility and flexibility of the film, improving its mechanical properties (Vieira et al., Citation2011). On the other hand, the addition of short chain lipids to films improves their barrier properties, decreasing the water vapor permeability rate (Atarés, Bonilla, & Chiralt, Citation2010; Tongnuanchan et al., Citation2012).
Gelatine is a natural biopolymer derived from various sources (swine, bovine and fish) that has been successfully used to develop containers that can carry functional ingredients (Da Silva E Silva, Pino Hernández, Da Silva Araújo, Peixoto Joele, & Lourenço, Citation2018; Etxabide et al., Citation2017). Currently, the active compounds that have been incorporated into the formulations of edible films include antimicrobial and antioxidant agents (Gómez-Estaca, Calvo, Sánchez-Faure, Montero, & Gómez-Guillén, Citation2015; Ma et al., Citation2012). Incorporating natural antioxidants into packaging materials improves the stability of food products by reducing the levels of reactive oxygen species, which act as initiators of the oxidation processes (Gómez-Estaca, López-de-Dicastillo, Hernández-Muñoz, Catalá, & Gavara, Citation2014).
Tomatoes (Solanum lycopersicum L.) are an important source of antioxidant compounds. Tomatoes have a high content of phytonutrients such as ascorbic acid, phenolic compounds and carotenoids (Borguini & Da Silva Torres, Citation2009; Toor & Savage, Citation2005). Lycopene, in particular, is the most characteristic compound of the tomato as it represents 80% of the total carotenoids and is responsible for the red color of the tomato (Erge & Karadeniz, Citation2011; Rosales et al., Citation2011). On the other hand, β-carotene provides the protection against UV/visible light (Rosales et al., Citation2011). Both carotenoids are primarily responsible for the antioxidant properties of tomatoes due to their ability to inhibit the production of singlet oxygen (1O2) and to trap peroxyl radicals (ROO•) (Oroian & Escriche, Citation2015; Zanfini, Corbini, La Rosa, & Dreassi, Citation2010). In recent years, the antioxidant properties of these carotenoids have been extracted for the design and fortification of food (Ranveer, Patil, & Sahoo, Citation2013), which also impacts consumer selection due to striking colors. Gómez-Estaca et al. (Citation2015) reported the use of these extracted antioxidants in the preparation of coatings and edible films with application in food packaging. Some studies mention that the incorporation of carotenoids into edible films improves their color and their mechanical and barrier properties against UV/visible light (Gómez-Estaca et al., Citation2015; Januario, Sobral, Trindade, & Carvalho, Citation2009). However, little is known about the effects of the addition of these antioxidants to sheep gelatin, and its effects on their functional and barrier properties. Thus, the objective of the present study was to obtain an oily tomato extract rich in lycopene and β-carotene and evaluate its effects on the physicochemical and antioxidant properties of gelatin-based edible films.
2. Materials and methods
2.1. Vegetable material and chemicals
Cherry tomatoes were obtained from greenhouses located in Tulancingo, Hidalgo, Mexico. Bovine gelatin was purchased from Fermont (Productos Químicos Monterrey SA de CV, Monterrey, Nuevo León, Mexico). Glycerol, sorbitol, linseed oil, glacial acetic, sodium acetate (anhydrous), potassium persulfate, hexane, ethanol and acetone were purchased from JT Baker SA de CV (Avantor Performance Materials, Ecatepec, Mexico, Mexico) lycopene, β-carotene, Tween 80, 2,2ʹ-diphenyl-1-picrylhydrazyl (DPPH), trolox (6-Hydroxy-2,5,7,8-tetramethylchromane-2-carboxylic acid), ABTS [2,2′-azinobis-(3-ethylbenzothiazoline-6-sulfonic acid)], TPTZ (2,4,6-tri[2-pyridyl]-s-triazine), and ferric chloride were purchased from Sigma-Aldrich (Sigma-Aldrich Química SA de CV, Toluca, Mexico, Mexico).
2.2. Preparation of the oily extract
The oily tomato extract was prepared as described by López-Palestina et al. (Citation2018). The tomatoes were ground (Osterizer blender, model BLSTBC4129-013, Toluca, Mexico State, Mexico) together with crude linseed oil at a ratio of 2:1. The obtained mixture was subjected to an ultrasonic bath (Ultrasonic Cleaner, Mod. 32V118A, Freeport, IL, USA) at a frequency of 40 kHz for 30 min at 30°C, using 10 min intervals and 5 min of rest between each interval. Subsequently, the mixture was centrifuged at 15,000 × g for 10 min (Thermo Scientific centrifuge, Mod. ST 16R, Waltham, MA, USA) to separate the oil from the aqueous fraction. Finally, the supernatant was recovered as oily tomato extract (OTE). The content of lycopene and β-carotene in tomato fruits, as well as their antioxidant activity, were measured to compare the extraction efficiency of linseed oil with that of the solvents used. For this, 0.1 g of lyophilized tomato (Freeze dryer Model 79480 LABCONCO, Kansas City, MO, USA) was mixed with 20 mL of acetone:ethanol:hexane (1:1:2, v/v) or with 10 mL of linseed oil. The obtained mixtures were subjected to an ultrasonic bath and centrifugation under the operating conditions described above. Afterwards, a 0.5 mL sample of the oily extract was dissolved in 10 mL of hexane. These procedures were carried out under dim light conditions.
2.3. Quantification of lycopene and β-carotene
The concentrations of lycopene and β-carotene were spectrophotometrically determined according to the methods described by Fish, Perkins-Veazie, and Collins (Citation2002), with some modifications. The absorbance of the supernatants was measured at 503 and 478 nm (Spectrophotometer model 6715 UV/Vis, Jenway, Techne Inc. Staffordshire, UK) for lycopene and β-carotene, respectively. The concentrations of lycopene and β-carotene were determined from standard curves generated for each of the carotenoids.
2.4. Antioxidant activity
2.4.1. DPPH free radical scavenging assay
To carry out the 2,2ꞌ-diphenyl-1-picrylhydrazyl (DPPH) method described by Brand-Williams, Cuvelier, and Berset (Citation1995), 0.3 mL of the supernatants were mixed with 2.7 mL of a 6 × 10−5 M DPPH solution in cold ethanol; the mixture was placed in the dark for 60 min at 4°C, and then, the absorbance was then measured at 517 nm. The results were expressed in μM Trolox equivalents (TE) per mL of OTE.
2.4.2. Trolox equivalent antioxidant capacity method (TEAC)
To carry out the TEAC method (Re et al., Citation1999), the ABTS•+ radical was obtained from the reaction between ABTS (7 mM) and potassium persulfate (2.45 mM), incubated in the dark at room temperature (25 ± 2°C) for 16 h. Once the radical ABTS•+ was formed, it was diluted in ethanol to obtain an absorbance value of 0.700 (± 0.1) at 734 nm. The samples were reacted with the diluted ABTS•+ for 6 min and the absorbance was immediately measured at 734 nm. The results were expressed in μM Trolox equivalents per mL of OTE.
2.4.3. Analysis of the ferric antioxidant reducing power (FRAP)
For the analysis of the ferric antioxidant reducing power (Benzie & Strain, Citation2000), the FRAP reagent was prepared by mixing acetate buffer (0.3 M pH 3.6) with a solution of 10 mM of TPTZ (2, 4, 6 tripyridyl-s-triazine) in 40 mM HCl, and a solution of 20 mM of FeCl3 6H2O, at a ratio of 10:1:1 and maintained at 37°C. The assay solutions were prepared by mixing 2.25 mL of the FRAP reagent with 0.3 mL of the supernatants. The solutions were then incubated in the dark for 30 min at room temperature; the absorbance was subsequently measured at 593 nm. The results were expressed in μM Trolox equivalents per mL of OTE.
2.5. Preparation of the films
The films were prepared as described by Alparslan, Baygar, Baygar, Hasanhocaoglu, and Metin (Citation2014), with slight modifications. The film-forming solutions (FFS) were prepared with a gelatin concentration of 8 g per 100 mL of distilled water. Glycerol (15 g/100 g of gelatin) and sorbitol (10 g/100 g of gelatin) were added to the gelatin solution to function as plasticizers. The solution was mixed for 30 min at 45°C. Oily tomato extract was added to the film solution at a concentration of 0, 1, 3 or 5% (v/v) to obtain four different treatments. To stabilize the treatments containing OTE, a volume of Tween 80 corresponding to 25% of the OTE content was added to the solutions. The FFS were homogenized in an Ultra Turrax (IKA T25, DS1, Wilmington, NC, USA), progressively increasing the homogenization rate under the following conditions: 8000 × g for 2 min, 14000 × g for 2 min and 17500 × g for 1 min. The films were cast by placing 10 mL of the FFS in 9 cm diameter Petri dishes. Drying was done at 25°C for 48 h with a relative humidity (RH) of 57 ± 5%. Following drying, the films were removed from the Petri dishes and stored at 25 ± 2°C and a relative humidity (RH) of 75%, which was provided by a saturated solution of NaCl.
2.6. Characterization of the films
2.6.1. Moisture content
The moisture content (H) of the films was determined according to the method described by Antoniou, Liu, Majeed, Qazi, and Zhong (Citation2014) by drying 2 cm × 2 cm sections of film at 105°C for 24 h. The samples were kept at 50% RH for 3 days. They were then cooled to room temperature and 0% RH in a desiccator containing silica gel. The moisture content was determined using the following equation:
where W0 and W1 are the weight of the initial and dry samples, respectively.
2.6.2. Solubility of the films in water
The percentage of solubility of the films in water was determined according to the method described by Antoniou et al. (Citation2014). It was expressed as a percentage of the dry matter of the solubilized films after 24 h of immersion in distilled water. Sections of dried films (2 cm × 2 cm) were weighed (W0) and then immersed in 30 mL of distilled water at 25 ± 1°C under constant stirring for 24 h, followed by centrifugation at 1500 × g for 10 min. The undissolved matter was dried at 105°C for 24 h until reaching a constant weight (W1). The solubility in water (S) was calculated using the following equation:
2.6.3. Permeability to water vapor
Water vapor permeability (WVP) was evaluated according to the American Society for Testing and Materials (ASTM) standard method E96, with the modifications proposed by Aila-Suárez et al. (Citation2013). Each film was placed and sealed in a circular aluminum cell with a circular opening of 0.000282 m2, and then stored at 25°C in a desiccator to maintain a relative humidity gradient of 75% across the film. Silica gel (0% RH) was used to seal each aluminum cell and a saturated NaCl solution (75% RH) was used within the desiccator. The transport of water vapor was determined from the weight gained within the aluminum cell. After reaching steady-state conditions (approximately 2 h), eight weight measurements were taken during the following 8 h. The changes in cell weight were plotted against time. The slope of each line was calculated by linear regression (r2 > 0.98) and the water vapor transmission rate (WVP) was calculated dividing the slope of the line (g/s) by the area of cells (m2). After the permeation tests, the thickness of the films was measured (manual micrometer, Mitutoyo Co., Kobe, Japan) to calculate the WVP (g/Pa∙s∙m). Water vapor permeability was calculated using the following equation:
where w was weight gain (kg), x film thickness (m), t elapsed time for the weight gain (s), and ∆P the partial vapor pressure difference between the dry atmosphere and pure water (2642 Pa at 22°C).
2.6.4. Mechanical properties
Mechanical properties of the films, such as percentage elongation at break, tensile strength and Young’s modulus were evaluated according to standard methods ASTM D882-95a (ASTM, Citation1995) using a texturometer with cell load of 50 kg (Brookfield CT3 texture analyzer, Warrington, UK). The films were cut into rectangles (10 cm long × 2 cm wide) that were kept for 5 days in desiccators with a saturated solution of NaCl (75% RH). The separation between the meters was 5 cm, and the deformation speed was 0.10 mm/s. The thickness of the films was measured at 5 random positions using a hand micrometer (Mitutoyo Co., Kobe, Japan). The average value was used to calculate the cross-sectional area of the films. The percentage elongation at break, tensile strength and Young’s modulus were calculated using the following equations:
Where Fmax is the load at failure (force at which the films break), t is the initial film thickness, and w is the initial film width.
Where lf is the final length of the film at failure and l0 is the initial length (5 cm) of the film between grips.
Where F is equal to the force applied to the structure, A is the cross-sectional area of the film, ∆l is the change in length of the film when the force is applied to it and l0 is the initial length.
2.6.5. Optical properties
The color parameters of the films (values of L*, a* and b*) were measured using a HunterLab colorimeter (Minolta, CM508d, Minolta Camera. Co., Ltd, Osaka, Japan). The values of a* (red-green) and b* (yellow-blue) were used to calculate the values of chroma (C*) and angle hue (°h).
Three samples of each treatment were measured, with ten measurements being taken of each sample.
The light transmittance and transparency of the films were determined according to the methods of Li, Miao, Wu, Chen, and Zhang (Citation2014). The films were cut into rectangular pieces and placed in a UV-Vis spectrophotometer (model 6715 UV/Vis, Jenway, Techne Inc. Staffordshire, UK) using air as a reference. Subsequently, the ultraviolet and visible light barrier properties of the films were measured at wavelengths between 200 nm and 800 nm. Three repetitions of each sample were used, and the results obtained were expressed as a percentage of transmittance. The opacity (O) was calculated as the quotient of absorbance at 600 nm and thickness of the film in mm.
2.6.6. Concentration of lycopene and β-carotene in films
To determine the concentration of carotenoids in the films, 1 g of sample was weighed after mixing it with 10 mL of hexane following the same procedure described in Section 2.3.
2.6.7. Antioxidant properties
The DPPH and ABTS assays, and the ferric reducing antioxidant power (FRAP) assay, were used to measure the antioxidant activity of the films, for which film samples of 1 g were dissolved in 10 mL of ethanol. The measurements were made as described above for the oily tomato extract.
2.7. Statistical analysis
The data obtained from all of the analyses were expressed as the means ± standard deviations. The data were analyzed using analysis of variance (ANOVA) and Tukey’s multiple comparisons test (p ≤ 0.05). All analyzes were carried out using SAS System for Windows v9.4.
3. Results and discussion
3.1. Concentration of carotenoids and antioxidant activity in the oily extract
shows the content and antioxidant activities of the lycopene and β-carotene extracted from tomato fruits (wet basis) using linseed oil and the acetone-ethanol-hexane (1:1:2) mixture. The concentration of carotenoids (lycopene and β-carotene) extracted with linseed oil was 8.49% lower when compared to the concentration obtained with solvents. The greater antioxidant activity of the extracts obtained with the solvents, compared to OTE, could be explained by the presence of other antioxidant compounds such as ascorbic acid and phenolic compounds. These compounds were not extracted when using linseed oil due to its lipophilic nature. Despite this, the concentration of carotenoids extracted using linseed oil is similar to that reported in the literature. Borguini and Da Silva Torres (Citation2009) reported concentrations of 2.57 and 0.44 mg of lycopene and β-carotene, respectively, for each 100 g of fresh tomato. In three different varieties of tomato, Zanfini et al. (Citation2010) reported lycopene concentrations ranging from 0.85 to 11.27 mg/100 g fw and β-carotene concentrations ranging from 0.420 to 710 mg/100 g fw. The antioxidant activity found in the present study is also very similar to that reported by other authors such as Rosales et al. (Citation2011) (105 to 223 μM ET/100 g fw), Erge and Karadeniz (Citation2011) (42 to 57 μM ET/100 g fw) and Nour, Trandafir, and Ionica (Citation2013) (81 to 174 μM ET/100 g fw). The use of linseed oil to obtain oily tomato extracts rich in lycopene and β-carotene can be a cost-efficient, viable and solvent-free alternative suitable for human consumption. shows the concentration of carotenoids and the antioxidant activity of the oily extract.
Table 1. Lycopene and β-carotene content and antioxidant capacity in 100 g of fresh tomato extracted with crude linseed oil and organic solvents.
Tabla 1. Concentración de licopeno y β-caroteno y capacidad antioxidante en 100 g de tomate fresco extraidos con aceite de linaza crudo y solventes orgánicos.
Table 2. Lycopene and β-carotene content and antioxidant capacity of the tomato oily extract.
Tabla 2. Concentración de licopeno y β-caroteno y capacidad antioxidante del extracto oleoso de tomate.
3.2. Moisture content and solubility of the films
shows the percentage of moisture present in the films. The moisture content in the films without OTE is high, which may be explained by the presence of glycerol in the film formulation. Antoniou et al. (Citation2014) and Cerqueira et al. (Citation2012) mention that a higher content of plasticizers leads to a higher moisture content. This higher moisture content is due to the hydrophilic nature of glycerol, which modifies the polymer network, creating mobile regions with greater distances between chains that allows a greater amount of water to accumulate within the film. In contrast, the moisture content of the films decreases significantly with the inclusion of OTE, which may be due to the hydrophobic nature of OTE. These results are similar to those reported by Ma et al. (Citation2012) for gelatin-based films containing olive oil. The authors mention that partial interactions between proteins and water are replaced by interactions between proteins and oil, which may explain the decrease in the moisture content of the films.
Figure 1. Moisture content and solubility on gelatin-based films with oily tomato extract (OTE). Bars represent means values ± standard deviations. The same letters are not significantly different (p > 0.05).
Figura 1. Contenido de humedad y solubilidad de películas a base de gelatina con extracto oleoso de tomate (OTE). Las barras representan las medias ± desviaciones estándar. Letras iguales no son significativamente diferentes (p > 0.05).
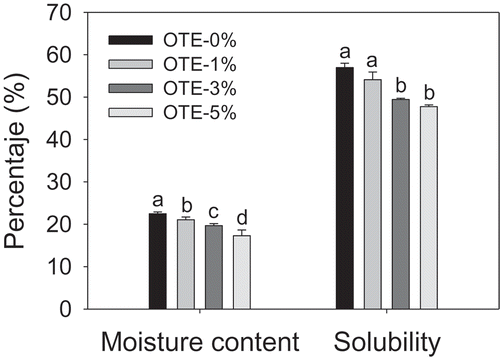
The solubility percentage of the films indicates their insolubility in water, or their hydrophobicity, and is a parameter that has commercial importance, as it influences different applications, such as the packaging of water-rich foods (Cerqueira et al., Citation2012; Ma et al., Citation2012). The results of the present study show that the behavior of this parameter is similar to that of the moisture content (), that is, the presence of glycerol in the films provides them with a greater affinity to water. Nevertheless, films with 3 and 5% OTE were significantly less soluble. These results suggest that the incorporation of OTE makes gelatin less soluble in water. The results obtained in this study are similar to those obtained for other gelatin-based films that have incorporated lipid substances into their structure (Cerqueira et al., Citation2012; Gómez-Estaca et al., Citation2015; Ma et al., Citation2012).
3.3. Permeability to water vapor (WVP)
shows that the WVP of the films was significantly affected by the addition of OTE. The PWV decreased 34.60% in the film samples with OTE-1% and decreased up to 64.49% in films with OTE-5%, when compared to the control films (OTE-0%). OTE, due to its lipophilic characteristics, directly affects the water vapor permeation rate (Atarés et al., Citation2010). This behavior and the range of WVP values found in the present work are similar to those of gelatin-based films containing olive or corn oil (Cerqueira et al., Citation2012; Ma et al., Citation2012). The WVP values found in this work are adequate for using the films as packaging material for food, which requires a controlled transport of water vapor to extend the useful life of the products.
Table 3. Water vapor permeability (WVP) and mechanical properties of gelatin-based films with oily tomato extract (OTE).
Tabla 3. Permeabilidad al vapor de agua (WVP) y propiedades mecánicas de películas a base de gelatina con extracto oleoso de tomate (OTE).
3.4. Mechanical properties
shows the mechanical properties of gelatin-based films containing OTE: tensile strength, elongation and Young’s modulus. As seen, the control films, OTE-1% and OTE-3%, did not present significant differences in elongation percentage and resistance to tension. The addition of OTE-5% to the films caused a significant increase in elongation and significant decreases in both tensile strength and Young’s modulus. This effect was probably due to the higher concentration of OTE, which might have resulted in the substitution of polymers for lipids in the film matrix, leading to the formation of weak bonds. In other words, the addition of OTE might have reduced the interaction between gelatin molecules, which in turn reduced the continuity and cohesion of the protein network (Atarés et al., Citation2010; Ma et al., Citation2012; Tongnuanchan et al., Citation2012). This caused a decrease in the stiffness of the films and an increase in their extensibility/elasticity. These observations are consistent with what has been previously reported with fish skin gelatin-based films with essential citrus oils and gelatin-based films with olive oil (Ma et al., Citation2012; Tongnuanchan et al., Citation2012).
In general, the films with OTE showed high extensibility/elasticity, but poor stiffness, this is more evident in the OTE-3% film, with respect to the Control (). This could have been caused not only by the addition of OTE but also by the high concentrations of glycerol and sorbitol present in the films. Antoniou et al. (Citation2014) reported that the tensile strength of edible films correlates inversely with the concentration of plasticizers (glycerol and sorbitol), whereas elongation correlates positively with it. The results obtained in this study show that the films obtained could be used as protective films in low moisture foods due to their elongation properties.
3.5. Optical properties
Optical properties are essential for determining the suitability of a film to be applied on the surface of food products, since they can affect the appearance of the coated product (Jridi et al., Citation2014). The appearance of the gelatin film is shown in and lists the color properties of the films. In general, gelatin-based films without OTE showed a slightly bright, luminous appearance, with a yellowish °hue (°h = 119.77) and very low chromaticity (colorless to the naked eye). However, the brightness decreased significantly as the concentration of OTE increased in the film formulation, while the redness (a*) and yellowness (b*) increased. The OTE-5% films showed a redder hue and a more intense color (higher chromaticity). These results indicate that the lycopene contained in the OTE contributed to the reddish-orange color of the films. This is observed in fresh tomatoes, where lycopene is the main carotenoid responsible for the red color (Zanfini et al., Citation2010). The color values found in this study are similar to those reported in the literature when carotenoids are incorporated into shrimp protein films and pork gelatin films (Gómez-Estaca et al., Citation2015; Januario et al., Citation2009).
Table 4. Color parameters on gelatin-based films with oily tomato extract (OTE).
Tabla 4. Parámetros de color en películas a base de gelatina con extracto oleoso de tomate (OTE).
Figure 2. Appearance of gelatin-based films with 0 (a), 1 (b), 3 (c) and 5 (d) % of tomato oily extract.
Figura 2. Apariencia de películas a base de gelatina con 0 (a), 1 (b), 3 (c) y 5 (d) % de extracto oleoso de tomate.
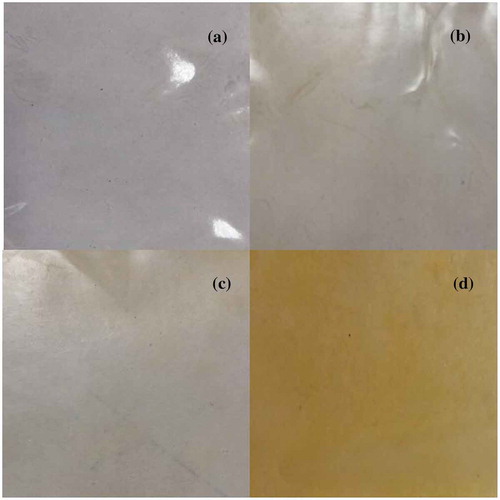
Edible films used for coating food should protect the coated product from the effects of light, especially from UV radiation (Li et al., Citation2014). shows the results of spectroscopic scanning at wavelengths between 200 and 800 nm. In general, all the films showed good UV barrier properties, especially at 280 nm, when light transmission was less than 5%. This can be attributed to the presence of gelatin in the films. According to the literature, protein-based films have high UV barrier properties due to the presence of aromatic amino acids that absorb UV light (Jridi et al., Citation2014). Increasing the concentration of OTE in the films improved their UV barrier properties. In the OTE-5% films, light transmittance was close to zero at 280 nm. Furthermore, the OTE incorporated into the films also showed excellent visible light barrier properties, mainly between 350 and 500 nm. The decrease in light transmittance could be explained by the presence of lycopene and β-carotene in the OTE, as they are lipophilic pigments with the ability to absorb light over a wide spectrum of wavelengths (Rodriguez-Amaya, Citation2002). The results of the present study suggest that the UV light barrier properties exhibited by gelatin-based films with OTE can potentially retard the UV light-induced oxidation of compounds in food systems, as has been mentioned in other studies in which antioxidants have been incorporated into edible films (Gómez-Estaca et al., Citation2015; Norajit et al., Citation2010). The incorporation of OTE also caused an increase in the opacity of the films (). The OTE-5% films showed significantly higher opacity due to the higher concentration of OTE in the formulation of the films, which caused a light scattering effect (Gómez-Estaca et al., Citation2015).
Table 5. Light transmittance (%T) and opacity on gelatin-based films with oily tomato extract (OTE).
Tabla 5. Transmisión de luz (%T) y opacidad de películas a base de gelatina con extracto de oleoso de tomate (OTE).
3.6. Content of lycopene and β-carotene and antioxidant activity
shows the lycopene and β-carotene contents in gelatin-based films with OTE. In the films with OTE, the carotenoid content increased significantly when the concentration of OTE increased. The lycopene content varied from 9.65 to 46.29 μg per gram of film, while the β-carotene content ranged from 1.21 to 5.79 μg. The values found in the present work are inferior to those reported by Gómez-Estaca et al. (Citation2015) for gelatin-based films with extracts containing carotenoids. This may be due to the low concentration of carotenoids in the oily tomato extract.
Figure 3. Lycopene and β-carotene content (µg per g of film) in gelatin-based films with oily tomato extract (OTE). Bars represent mean values ± standard deviations. The same letters are not significantly different (p > 0.05).
Figura 3. Contenido de licopeno y β-caroteno (µg por g de película) en películas a base de gelatina con extracto de oleoso de tomate (OTE). Las barras representan las medias ± desviaciones estándar. Letras iguales no son significativamente diferentes (p > 0.05).
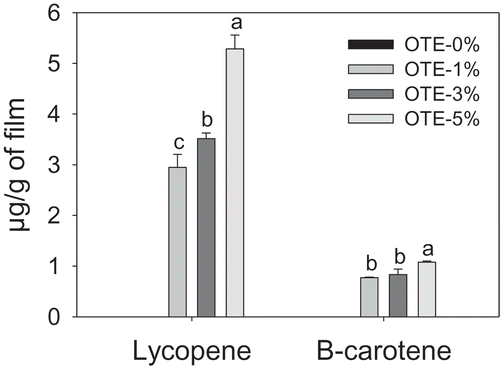
The antioxidant properties of the films were determined using the DPPH, ABTS and FRAP methods. A variety of methods were used in order to obtain a better understanding of the antioxidant properties of the films. The films with OTE-5% showed the highest antioxidant capacity (). The elimination of the DPPH radicals and the ferric reducing antioxidant power (FRAP) indicated that the maximum antioxidant activity was 1.47 and 1.52 μM Trolox per g of film, respectively.
Figure 4. Antioxidant capacity of gelatin-based films with oily tomato extract (OTE) determined by DPPH, ABTS and FRAP assays. Bars represent mean values ± standard deviations. Bars with the same letters are not significantly different (p > 0.05).
Figura 4. Capacidad antioxidante de películas a base de gelatina con extracto oleoso de tomate (OTE) determinada mediante los ensayos de DPPH, ABTS y FRAP. Las barras representan las medias ± desviaciones estándar. Letras iguales no son significativamente diferentes (p > 0.05).
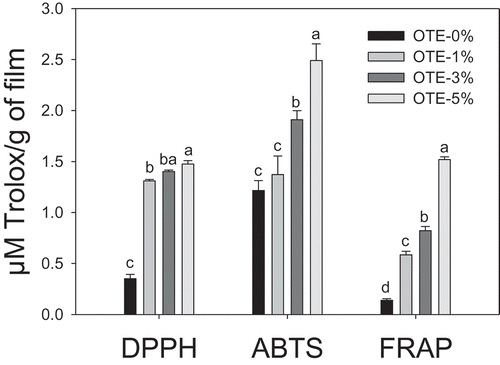
A maximum of 2.49 μM Trolox per g of film was found with the discoloration assay of the ABTS●+ radical. The higher antioxidant activity yielded by the ABTS method may be explained by the greater sensitivity of this method to the antioxidant activity of lipophilic compounds when compared with the DPPH and FRAP methods (Alam, Bristi, & Rafiquzzaman, Citation2013). Tongnuanchan et al. (Citation2012) found that the ABTS method yielded a higher antioxidant activity for gelatin-based films containing essential citrus oils than the FRAP and DPPH methods. The results found in this study are similar to those reported in other studies that also confirm that the incorporation of natural antioxidants provides antioxidant properties to films (Ruiz-Navajas, Viuda-Martos, Sendra, Perez-Alvarez, & Fernández-López, Citation2013; Tongnuanchan et al., Citation2012). The results obtained in this study show that antioxidant activity is directly related to the concentrations of lycopene and β-carotene in the films ( and ). This is because both carotenoids are considered excellent antioxidants, mainly due to their ability to inhibit the action of singlet oxygen (1O2) and lipid oxidation, and to trap peroxyl radicals (ROO•) (Oroian & Escriche, Citation2015).
4. Conclusions
Using linseed oil to extract lycopene and β-carotene from fresh tomato and obtain oily tomato extract proved to be an effective solvent-free method with low operating requirements. The incorporation of the oily tomato extract into gelatin-based films caused reductions in moisture content, solubility and permeability to water vapor. Moreover, the films with OTE-5% showed significant reductions in tensile strength and Young’s modulus, but an increase in percentage of elongation. The incorporation of 3 and 5% of oily extracts rich in lycopene and β-carotene improved the UV barrier properties of the films and gave them a reddish coloration. The results showed that gelatin-based films with oily tomato extracts rich in lycopene and β-carotene can be used as active films due to their in vitro antioxidant properties.
Acknowledgments
The main author thanks the National Council for Science and Technology (CONACyT, Mexico) for scholarship No. 402763 for graduate studies in Agri-Food Production Science at the TecNM-Instituto Tecnologico de Roque.
Disclosure statement
No potential conflict of interest was reported by the authors.
Additional information
Funding
References
- Aila-Suárez, S., Palma-Rodríguez, H. M., Rodríguez-Hernández, A. I., Hernández-Uribe, J. P., Bello-Pérez, L. A., & Vargas-Torres, A. (2013). Characterization of films made with chayote tuber and potato starches blending with cellulose nanoparticles. Carbohydrate Polymers, 98(1), 102–107.
- Alam, M. N., Bristi, N. J., & Rafiquzzaman, M. (2013). Review on in vivo and in vitro methods evaluation of antioxidant activity. Saudi Pharmaceutical Journal, 21(2), 143–152.
- Alparslan, Y., Baygar, T., Baygar, T., Hasanhocaoglu, H., & Metin, C. (2014). Effects of gelatin-based edible films enriched with laurel essential oil on the quality of rainbow trout (Oncorhynchus mykiss) fillets during refrigerated storage. Food Technology and Biotechnology, 52(3), 325–333.
- American Society for Testing and Materials (ASTM). (1995). Standard method for water vapor transmission of materials. Philadelphia Annual Book of ASTM Standards. D96-95.
- Antoniou, J., Liu, F., Majeed, H., Qazi, H. J., & Zhong, F. (2014). Physicochemical and thermomechanical characterization of tara gum edible films: Effect of polyols as plasticizers. Carbohydrate Polymers, 111, 359–365.
- Atarés, L., Bonilla, J., & Chiralt, A. (2010). Characterization of sodium caseinate-based edible films incorporated with cinnamon or ginger essential oils. Journal of Food Engineering, 100(4), 678–687.
- Benzie, I., & Strain, J. (2000). The ferric reducing ability of plasma as a measure of antioxodant. Analytical Biochemistry, 239(1), 70–76.
- Borguini, R. G., & Da Silva Torres, E. A. F. (2009). Tomatoes and tomato products as dietary sources of antioxidants. Food Reviews International, 25(4), 313–325.
- Brand-Williams, W., Cuvelier, M. E., & Berset, C. (1995). Use of a free radical method to evaluate antioxidant activity. LWT - Food Science and Technology, 28(1), 25–30.
- Cerqueira, M. A., Souza, B. W. S., Teixeira, J. A., & Vicente, A. A. (2012). Effect of glycerol and corn oil on physicochemical properties of polysaccharide films - A comparative study. Food Hydrocolloids, 27(1), 175–184.
- Da Silva E Silva, N., Pino Hernández, E. J. G., Da Silva Araújo, C., Peixoto Joele, M. R. S., & Lourenço, L. D. F. H. (2018). Development and optimization of biodegradable fish gelatin composite film added with buriti oil. CYTA - Journal of Food, 16(1), 340–349.
- Erge, H. S., & Karadeniz, F. (2011). Bioactive compounds and antioxidant activity of tomato cultivars. International Journal of Food Properties, 14(5), 968–977.
- Etxabide, A., Uranga, J., Guerrero, P., & de la Caba, K. (2017). Development of active gelatin films by means of valorisation of food processing waste: A review. Food Hydrocolloids, 68, 192–198.
- Fish, W. W., Perkins-Veazie, P., & Collins, J. K. (2002). A quantitative assay for lycopene that utilizes reduced volumes of organic solvents. Journal of Food Composition and Analysis, 15(3), 309–317.
- Gómez-Estaca, J., Calvo, M. M., Sánchez-Faure, A., Montero, P., & Gómez-Guillén, M. C. (2015). Development, properties, and stability of antioxidant shrimp muscle protein films incorporating carotenoid-containing extracts from food by-products. LWT - Food Science and Technology, 64(1), 189–196.
- Gómez-Estaca, J., López-de-Dicastillo, C., Hernández-Muñoz, P., Catalá, R., & Gavara, R. (2014). Advances in antioxidant active food packaging. Trends in Food Science and Technology, 35(1), 42–51.
- Januario, M. M. S., Sobral, P. J. A., Trindade, C. S. F., & Carvalho, R. A. (2009). Gelatin films with lycopene: Mechanical, water vapor barrier, color, and light barrier properties. Italian Journal of Food Science, 21, 126–129.
- Jridi, M., Hajji, S., Ayed, H. B., Lassoued, I., Mbarek, A., Kammoun, M., & Nasri, M. (2014). Physical, structural, antioxidant and antimicrobial properties of gelatin-chitosan composite edible films. International Journal of Biological Macromolecules, 67, 373–379.
- Li, J. H., Miao, J., Wu, J. L., Chen, S. F., & Zhang, Q. Q. (2014). Preparation and characterization of active gelatin-based films incorporated with natural antioxidants. Food Hydrocolloids, 37, 166–173.
- López-Palestina, C. U., Aguirre-Mancilla, C. L., Raya-Pérez, J. C., Ramírez-Pimentel, J. G., Gutiérrez-Tlahque, J., & Hernández-Fuentes, A. D. (2018). The effect of an edible coating with tomato oily extract on the physicochemical and antioxidant properties of garambullo (Myrtillocactus geometrizans) fruits. Agronomy, 8, 248.
- Ma, W., Tang, C. H., Yin, S. W., Yang, X. Q., Qi, J. R., & Xia, N. (2012). Effect of homogenization conditions on properties of gelatin-olive oil composite films. Journal of Food Engineering, 113(1), 136–142.
- Norajit, K., Kim, K. M., & Ryu, G. H. (2010). Comparative studies on the characterization and antioxidant properties of biodegradable alginate films containing ginseng extract. Journal of Food Engineering, 98(3), 377–384.
- Nour, V., Trandafir, I., & Ionica, M. E. (2013). Antioxidant compounds, mineral content and antioxidant activity of several tomato cultivars grown in Southwestern Romania. Notulae Botanicae Horti Agrobotanici Cluj-Napoca, 41(1), 136–142.
- Oroian, M., & Escriche, I. (2015). Antioxidants: Characterization, natural sources, extraction and analysis. Food Research International, 74, 10–36.
- Ranveer, R. C., Patil, S. N., & Sahoo, A. K. (2013). Effect of different parameters on enzyme-assisted extraction of lycopene from tomato processing waste. Food and Bioproducts Processing, 91(4), 370–375.
- Re, R., Pellegrini, N., Proteggente, A., Pannala, A., Yang, M., & Rice-Evans, C. (1999). Antioxidant activity applying an improved ABTs radical cation decolorization assay. Free Radical Biology and Medicine, 26(98), 1231–1237.
- Rodriguez-Amaya, D. B. (2002). Food carotenoids: Analysis, composition and alterations during storage and processing of foods. Forum of Nutrition, 56, 35–37.
- Rosales, M. A., Cervilla, L. M., Sánchez-Rodríguez, E., Rubio-Wilhelmi, M. D. M., Blasco, B., Ríos, J. J., & Ruiz, J. M. (2011). The effect of environmental conditions on nutritional quality of cherry tomato fruits: Evaluation of two experimental Mediterranean greenhouses. Journal of the Science of Food and Agriculture, 91(1), 152–162.
- Ruiz-Navajas, Y., Viuda-Martos, M., Sendra, E., Perez-Alvarez, J. A., & Fernández-López, J. (2013). In vitro antibacterial and antioxidant properties of chitosan edible films incorporated with Thymus moroderi or Thymus piperella essential oils. Food Control, 30(2), 386–392.
- Tongnuanchan, P., Benjakul, S., & Prodpran, T. (2012). Properties and antioxidant activity of fish skin gelatin film incorporated with citrus essential oils. Food Chemistry, 134(3), 1571–1579.
- Toor, R. K., & Savage, G. P. (2005). Antioxidant activity in different fractions of tomatoes. Food Research International, 38(5), 487–494.
- Vieira, M. G. A., Da Silva, M. A., Dos Santos, L. O., & Beppu, M. M. (2011). Natural-based plasticizers and biopolymer films: A review. European Polymer Journal, 47(3), 254–263.
- Zanfini, A., Corbini, G., La Rosa, C., & Dreassi, E. (2010). Antioxidant activity of tomato lipophilic extracts and interactions between carotenoids and ∝-tocopherol in synthetic mixtures. LWT - Food Science and Technology, 43(1), 67–72.