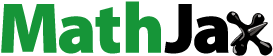
ABSTRACT
Unlike conventional extraction (CE) methods, high hydrostatic pressure extraction (HHPE) uses less solvent volume and reduced extraction times. We show here that HHPE increases extractability, antioxidant capacity and bio-accessibility of the bioactive compounds from discarded blueberries when compared to CE. Bioactive compounds were extracted by CE with agitation and by HHPE at 500 MPa for 5, 10 and 15 min. The highest content of anthocyanins (117.08mg 100g−1 FW), polyphenols (9.56mg 100g−1 FW), flavonoids (0.62mg 100g−1 FW) and antioxidant capacities (1853.69 and 2059.55 μMg−1 FW for DPPH and FRAP, respectively) with HHPE for 15 min. Likewise, HHPE for 15 min resulted in the highest bio-accessibility of polyphenols (62.2%) and flavonoids (62.2%), whereas 85.1% and 60.9% of antioxidant capacity measured as DPPH and FRAP, respectively. Only anthocyanins showed decreased bio-accessibility after HHPE. Antioxidant potential of discarded blueberries can be enhanced with HHPE by increasing the extractability of bioactive compounds and their bio-accessibility.
RESUMEN
A diferencia del método de extracción convencional (CE), la extracción con alta presión hidrostática (HHPE) permite utilizar un menor volumen de solvente y reducir los tiempos de extracción. Aquí mostramos que el HHPE aumenta el rendimiento de extracción, la capacidad antioxidante y la bio-accesibilidad potencial de los compuestos bioactivos de arándanos descartados en comparación con CE. Los compuestos bioactivos se extrajeron mediante CE con agitación y con HHPE a 500 MPa durante 5, 10 y 15 min. El mayor contenido de antocianinas (117.08mg 100g−1 FW), polifenoles (9.56mg 100g−1 FW), flavonoides (0.62mg 100g−1 FW) y capacidad antioxidante (1853.69 y 2059.55 μMg−1 FW mediante DPPH y FRAP, respectivamente) fue con HHPE durante 15 min. Del mismo modo, el HHPE durante 15 minutos dio como resultado la mayor bio-accesibilidad potencial de polifenoles (62.2%) y flavonoides (62.2%), mientras que 85.1% y 60.9% de la capacidad antioxidante cuantificada como DPPH y FRAP, respectivamente. Solo las antocianinas mostraron una disminución de la bio-accesibilidad potencial después de HHPE. El potencial antioxidante de los arándanos descartados puede mejorar con HHPE al incrementar el rendimiento de extracción de los compuestos bioactivos y su bio-accesibilidad potencial.
PALABRAS CLAVE:
1. Introduction
Consumption of wholesome fruit and vegetables aids in the prevention of age-related diseases (Nicklett & Kadell, Citation2013). Berries are a rich source of bioactive compounds that perform diverse valuable functions in human health. Among berries, blueberry is a popular North-American fruit (Yemmireddy, Chinnan, Kerr, & Hung, Citation2013) and an important crop for Chile (Retamales et al., Citation2014). In Chile, the blueberry market has expanded in the last years, total exports of the blueberries of the 2016–2017 season (week 11) around 103,000 tons, 13% more than the total exported in 2015/16 and 9% more than was forecasted for this crop season. As a summary, 63% of the volumes of this campaign were destined to the U.S, 22% to Europe and 12% to the Far East, becoming the main producer in South America. (Chilean Blueberry Committee, Citationn.d.; Retamales et al., Citation2014). Blueberries normally soften during the postharvest chain compromising their final quality. As a consequence, significant volumes of blueberries are rejected in the marketplace due to their firmness levels below retail standards (Paniagua, East, Hindmarsh, & Heyes, Citation2013). Blueberries (belonging to the Ericaceae family; genus Vaccinium) can be classified as highbush (V. corymbosum), lowbush (V. angustifolium), and rabbiteye (V. virgatum). They have been considered among the fruits with the highest antioxidant potentials (Skrovankova, Sumczynski, Mlcek, Jurikova, & Sochor, Citation2015) having a high content of phenolic compounds including anthocyanins and chlorogenic acid. Anthocyanins are natural pigments responsible for the orange, red, blue, and violet colors of some fruits and flowers (Hidalgo & Almajano, Citation2017). These compounds have received the most attention since they are major contributors of the color in berries. Anthocyanins are the main phenolic components of berries and have shown to be twice as effective than the available commercial antioxidants such as butylated hydroxyanisole (BHA) and α-tocopherol (Martín, Kuskoski, Navas, & Asuero, Citation2017).
The anthocyanins stand for a unique subset of phenolic secondary metabolites found in plant tissues. They are one of the many compounds being included in the flavonoid group, featuring a bi-phenolic structure (Barnes, Nguyen, Shen, & Schug, Citation2009). Fruits are rich in polyphenols with a high health beneficial activity (Mrduljaš, Krešić, & Bilušić, Citation2018). However, to perform their biological functions, bioactive compounds must be available to some extent in the target tissue. Consequently, the biological properties of bioactive compounds may depend on their bio-availability (Saura-Calixto, Serrano, & Goñi, Citation2007). Bio-availability is described as the fraction of ingested nutrient that is absorbed and utilized (Rein et al., Citation2013). Before becoming bio-available, bioactive compounds must first be made bio-accessible. Bio-accessibility is the amount of an ingested nutrient that is potentially available for absorption and is dependent only on digestion and release from the food matrix (Etcheverry, Grusak, & Fleige, Citation2012). Thus, by quantifying potential bio-accessibility we can infer the proportion of bioactive compounds that are ready for absorption. (Rodríguez-Roque, Rojas-Graü, Elez-Martínez, & Martín-Belloso, Citation2013).
Bioactive compounds extraction processes mainly depend on compound structure, material source and the extracting solvents. Conventional extraction is time-consuming, requires a large amount of solvent and has a low efficiency (Zhang, Lin, & Ye, Citation2018) . Moreover, if thermic, a prolonged extraction can cause bioactive compounds degradation and a decrease in the antioxidant activity (Uribe, Delgadillo, Giovagnoli-Vicuña, Quispe-Fuentes, & Zura-Bravo, Citation2015). High hydrostatic pressure extraction (HHPE), also known as cold isostatic pressure extraction, has been widely used in the food industry to extend shelf-life by reducing the number of bacteria and increasing the activity and/or stability of enzymes (Altuner & Tokuşoǧlu, Citation2013). It may also be used on raw materials and ingredients to change their molecular structure. An important application of HHPE is the extraction of polyphenol compounds at room temperature. In this process, a raw material mixture and the solvent are processed by HHPE for a time span similar to that of food processes. After quick release of the pressure, the mixture is separated and purified and the raw material components are extracted. HHPE has been successfully employed in the extraction of ginsenosides from ginseng (Lee et al., Citation2011), polyphenols from green tea leaves (Xi et al., Citation2009), anthocyanins from grape skin pomace (Putnik et al., Citation2018) and grape waste (Corrales, Toepfl, Butz, Knorr, & Tauscher, Citation2008) and flavonoids from propolis (Shouqin, Jun, & Changzheng, Citation2005).
The aim of this study was to investigate to what extend the antioxidant content and in vitro potential bio-accessibility of bioactive compounds (anthocyanin, polyphenols and flavonoid contents) of discarded blueberries extracted by HHPE differ from conventional extraction
2. Materials and methods
2.1. Chemicals
Trolox® (6-hydroxy-2,5,7,8-tetramethylchroman-2-carboxylic acid), methanol (Tedia, USA), DPPH (2,2-diphenyl-1-picryhydrazyl) and Folin–Ciocalteau’s phenol reagent were purchased from Merck (Darmstadt, Germany). Gallic acid and quercetin standards were purchased from Sigma-Aldrich (St. Louis, MO, USA). Sodium acetate was purchased from Merck; acetic acid, TPTZ (2, 4, 6-tripyridyl-s-triazine), and ferric chloride hexahydrate (FeCl3 x 6 H2O) were purchased from Sigma-Aldrich (St. Louis, MO, USA). Milli-Q water was obtained through a Millipore filter system (Millipore Co., USA). FRAP reagent was prepared with 2.5 mL of a 10 mm L−1 TPTZ solution in 40 mmol L−1 HCl with 2.5 mL of 20 mm L−1 FeCl3 and 25 mL of 0.3 mol L−1 acetate buffer at a pH of 3.6) All enzymes were from Sigma Aldrich (Sigma P7000, P1750). Enzymes and bile extracts (Sigma, B8631) used for in vitro digestion were of porcine origin.
2.2. Raw material and sample preparation
The discarded blueberries (O’Neal variety) (damaged, smaller than 10 mm, dehydrated) were obtained from a local producer (La Serena, Chile). Discarded blueberries were washed, dried with absorbent paper and thereafter homogenized in a blender (Philips, HR1720, Amsterdam). The pulp was stored at 4ºC until use.
2.3. Proximate analysis
The methodologies described by Association of Official Analytical Chemists (AOAC) were used. Moisture content, crude protein, lipid content, crude fiber and crude ash content were determined by the AOAC methods number 934.06, 960.52, 960.39, 962.09 and 923.03, respectively (AOAC, Citation1990). All measurements were carried out in triplicate.
2.4. Determination of anthocyanins
2.4.1. Conventional extraction (CE)
Conventional extraction was performed at 0.1 MPa by the method described by (Khanal, Howard, & Prior, Citation2010) and (Barnes et al., Citation2009), with modifications. Briefly, 2 g of discarded blueberries were mixed with 30 mL of an acetone/water/acetic acid extraction solvent (ratio of 70:29.5:0.5). The mixture was agitated for 16 h at room temperature (RT, 20ºC) in an orbital shaker (Boeco, OS20, Germany), centrifuged at 2,000 × g for 10 min at 15ºC, and the supernatant collected. The supernatant was filtered through Whatman paper (grade 1) and re-extracted three times with 10 mL of solvent for 30 min under the same conditions. The filtrate was mixed slowly with chloroform at a 1/1 (v/v) ratio and centrifuged at 6,000 × g for 10 min at 5ºC to separate the phases. The aqueous phase (upper layer) was centrifuged at 6,000 × g for 10 min at 5ºC to remove all the non-aqueous phase. The clean aqueous phase was transferred to a 250 mL boiling flask and evaporated in a rotary evaporator (Büchi, RE12, Switzerland) at 40°C under vacuum. The discarded blueberry extract was reconstituted with double-distilled water and acidified to pH 2.5 with a 50 mL of 0.05% HCl.
2.4.2. High hydrostatic pressure extraction (HHPE)
Two grams of discarded blueberries were packed individually with extraction solvent (acetone/water/acetic acid ratio of 70/29.5/0.5) and hermetically sealed in high density polyethylene bags. The packaged samples were placed in a 2 L cylindrical loading container at RT (20ºC) and pressurized at 500 MPa for 5, 10 and 15 min with pulses of 1 min each in a high hydrostatic pressure equipment (Avure Technologies Inc., USA). The samples were then treated as described above in the CE method.
2.4.3. Determination of total anthocyanin content (TAC)
The total anthocyanin content of the aqueous blueberry extract was determined by the spectrophotometric pH-differential method of (Giusti & Wrolstad, Citation2005) and (Lee, Durst, & Wrolstad, Citation2005) and was expressed as mg of cyanidin-3-glucoside equivalents per 100 g of fresh weight (FW) on the basis of anthocyanins molar extinction coefficient (26,900 L/(mol*cm)).
2.5. Determination of antioxidant capacities and bioactive compounds
2.5.1. Conventional extraction (CE)
Conventional extraction was carried out for 5 g of discarded blueberries. The berries were mixed at a 1/10 ratio with extraction solvent (80% methanol). The mixture was agitated (orbital shaker) for 24 h at RT and then centrifuged at 6,000 × g for 10 min at 15ºC. The supernatant was filtered through Whatman paper (grade 1). The solid filter cake was re-extracted under the same conditions. Both filtrates were transferred into a round-bottom flask (250 mL) and evaporated by using a rotary evaporator under reduced pressure at 40°C. The discarded blueberry extract was dissolved in 80% methanol and diluted to a final volume of 25 mL.
2.5.2. High hydrostatic pressure extraction (HHPE)
Five grams of discarded blueberries were packed individually with extraction solvent (80% methanol) and hermetically sealed in high density polyethylene bags. The samples were extracted as mentioned in section of HHPE for anthocyanins.
2.5.3. Determination of DPPH radical scavenging capacity
The antioxidant capacity was measured using the 2,2´-diphenyl-1-picryhydrazyl free radical scavenging (DPPH) method (Brand-Williams, Cuvelier & Berset, Citation1995; Kim, Lee, Lee, & Lee, Citation2002). A solution of 50 μM DPPH in aqueous methanol (80%) was mixed for 20 min. Then, 0.1 mL of 50 μM DPPH or discarded blueberry extract was mixed with 3.9 mL of DPPH solution and incubated for 30 min in darkness. DPPH was measured spectrophotometrically at 517 nm. Total DPPH was calculated from a calibration curve (y = −0.5259x + 0.5978; R2 = 0.9993) of the synthetic antioxidant Trolox at concentrations between 0.08 to 1.28 mM. The antioxidant capacity determined by DPPH assay was expressed as micromolar Trolox equivalent per gram of fresh weight (µM TE g-1 FW). All measurements were carried out in triplicate.
2.5.4. Determination of ferric reducing ability of plasma (FRAP) assay
FRAP assay was used to determine the reducing power of discarded blueberry extracts according to Benzie and Strain (Citation1996), with some modifications. The freshly made FRAP reagent was warmed to 37°C. Sixty-microliter aliquots of discarded blueberry extract were mixed with 180 µL of distilled water and 1800 µL of FRAP reagent and incubated at 37°C for 2 h. The reaction mixture absorbance was measured in a spectrophotometer at 593 nm. Antioxidant capacity by FRAP assay was calculated from a Trolox calibration curve (y = 0.001x + 0.0621; R2 = 0.9977) at concentrations between 100 and 1500 μM. The values were expressed as micromolar Trolox equivalent per gram of fresh weight (µM TE g−1 FW).
2.5.5. Determination of total polyphenol content (TPC)
Total polyphenols content was measured spectrophotometrically using the Folin-Ciocalteau reagent (FC) (Que, Mao, Fang, & Wu, Citation2008). A 0.5 mL aliquot of discarded blueberry extract was mixed with 0.5 mL of FC reagent and 2 mL of 20% Na2CO3. The sample was shaken and incubated for 15 min at RT. Ten milliliters of ultra-pure water were added, and the sample was cleared from the precipitate by centrifugation for 15 min at 6,000 x g. Finally, the absorbance was measured in a spectrophotometer (Spectronic® 20 GenesysTM131, USA) at a wavelength of 725 nm. Total polyphenol content was calculated from a gallic acid (GA) calibration curve (y = 0.003x + 0.037; R2 = 0.9940) at concentrations between 50 and 600 µg mL−1. The TPC values were expressed as mg GA equivalents g−1 FW. All measurements were performed in triplicate.
2.5.6. Determination of total flavonoid content (TFC)
Total flavonoid content was measured spectrophotometrically according to Dini, Tenore, and Dini (Citation2010) with modifications. A 500 μL aliquot of discarded blueberry extract was mixed with 2 mL of double-distilled water (ddH2O) and 150 μL NaNO2 aqueous solution (5 g 100 mL−1). One hundred fifty microliters of AlCl3 aqueous solution (10 g 100 mL−1) was added after 5 min. Finally, 1 mL of NaOH (1 M) and 1.2 mL of ddH2O were added to the mixture after 6 min. The absorbance was measured at a wavelength of 415 nm in a spectrophotometer. The TFC was calculated from a quercetin calibration curve (y = 0.0019x – 0.0142; R2 = 0.9913) at concentrations between 20 and 100 µg mL−1. The TFC was expressed in mg quercetin equivalent (QE) per gram FW. All measurements were performed in triplicate.
2.6. Phenolic profile: fractionation, identification and quantitation of phenolic compounds by HPLC
The phenolic compounds extracted either by CE or HHPE were fractionated according to (León-González et al., Citation2013). The obtained concentrated discarded blueberry extract was filtered (0.45 μm membrane filter) and phenolic measured by HPLC.
The chromatographic conditions used to identify and quantify phenolic compounds are described in (Vega-Gálvez et al., Citation2014). Identification and quantification of some of the main phenolic compounds was performed by comparing the samples with the retention times and spectra of the 27 standards (Gallic Acid, Pyrogallol, 3-Hydroxytyrosol, Protocatechuic, Chlorogenic Acid, Catechin, Tyrosol, Epicatechin, 4-hydroxybenzoic Acid, Eriocitrin, Caffeic Acid, Syringic Acid, Vanillic Acid, Rutin, Ellagic Acid, Hesperidin, p-coumaric Acid, Trans-sinapic Acid, Trans-Ferulic Acid, Myricetin, 3-Methylcatechol, Benzoic Acid, Methoxybenzoic Acid, Quercetin, Salicylic Acid, Trans-Cinnamic Acid, Kaempferol), considering the peak area of maximum absorption wavelength. The results from the main phenolic compounds were expressed as mg per 100 g FW.
2.7. In vitro digestion and potential relative bio-accessibility
The in vitro digestion model was performed according to Minekus et al. (Citation2014). This protocol simulates the different phases of digestion: oral, gastric and intestinal (small intestine). This protocol simulates the different phases of digestion: oral, gastric and intestinal (small intestine). The maximal amount of total bioactive compounds and antioxidant capacity that is released from matrix in the gastrointestinal tract is called bio-accessibility. To quantify potential bio-accessibility the recovery of total bioactive compounds and antioxidant capacity was estimated in each discarded blueberry extract before and after the in vitro digestion (the sum of all digestion phases). Potential relative bio-accessibility percentages were calculated as following:
Where CD is the concentration of bioactive compounds or antioxidant capacity in the bio-accessible fraction and CI is the initial concentration of bioactive compounds or total antioxidant capacity before digestion. All measurements were performed in triplicate.
2.8. Percent yield
The percentage of yield increase or decrease of quantified bioactive compounds in this study was calculated with the following formula:
Percent yield > 0 indicates a percentage increase; Percent yield< 0 a decrease.
2.9. Statistical analysis
Statgraphics Plus® 5.1 software was used to determine significant differences among samples by using ANOVA (One-way analysis of variance). Fisher’s least significant difference test was used as significance testing; differences were taken as statistically significant when p ≤ 0.05. Also, the Multiple Range Test (MRT) was used to find homogeneous groups within each of the analyzed parameters. For all samples, three different batches (n = 3) were considered to perform the statistical analysis.
3. Results and discussion
3.1. Proximate analysis of discarded blueberry
Proximate analysis of discarded fresh blueberries (per 100 g FW) presented a moisture content of 79.6 ± 0.2 g; crude protein of 0.8 ± 0.0 g; total lipids of 0.3 ± 0.1 g; crude fiber of 2.3 ± 0.0 g; crude ash of 0.2 ± 0.0 g; carbohydrates (by difference) of 16.8 ± 0.3 g. Similar values were found by Michalska and Łysiak (Citation2015) in blueberries in a fresh form with 84.0 g 100 g−1 for moisture content,, 0.6 g 100 g−1 for proteins, 0.4 g 100 g−1 for total lipids and 9.7 g 100 g−1 for carbohydrates.
3.2. Anthocyanin content and potential bio-accessibility
Anthocyanin extractability increased with HHPE at all extraction times applied compared to CE (). The highest anthocyanin content (117.1 mg anthocyanin per 100 grams of discarded blueberry extract) was obtained extracting at 500 MPa during 15 min. A previous study reported a range between 12.7 and 331 mg per 100 gram of total anthocyanin content in berry extracts (Lapornik, Prošek, & Wondra, Citation2005). HHPE results before in vitro digestion showed in that the anthocyanin content increased from 15.1, 17.2 and 39.4% with the increase of extraction time from 5, 10 and 15 min respectively when compared to CE.
Table 1. Anthocyanin content and potential relative bio-accessibility from discarded blueberry extracts obtained by CE (Conventional extraction) and HHPE (High hydrostatic pressure extraction). Different lowercase letters in columns indicate significant differences between mean values (p ≤ 0.05).
Tabla 1. Contenido de antocianinas y bio-accesibilidad relativa potencial de extractos de arándanos descartados obtenidos por CE (extracción convencional) y HHPE (extracción por alta presión hidrostática). Las diferentes letras minúsculas en las columnas indican diferencias significativas entre los valores medios (p ≤ 0.05).
The slight increase of anthocyanins could result from plant cell disruption caused by HHPE, leading to a higher extractability of these compounds. This was also shown in grape, cashew apple juice, purple sweet potato nectar and grape skin pomace (M. Corrales et al., Citation2008; Putnik et al., Citation2018; Queiroz et al., Citation2010; Wang et al., Citation2012). A similar trend was observed in anthocyanin content for pressure treated strawberry purées at 600 MPa, in which the content increased in 9.8% compared to the unprocessed purée (Patras, Brunton, Da Pieve, & Butler, Citation2009).
Our results showed that the anthocyanin content positively correlated with extraction time (). This can be explained since under high-pressure conditions, the diffusion speed of the solvent is either very high or the pressure equilibration between the inside and outside of the cells occurs in a very short time (Briones-Labarca, Giovagnoli-Vicuña, & Cañas-Sarazúa, Citation2019). Bioactive compounds can be efficiently extracted with HHPE by increasing solvent power, solvent density and solubility of polar compounds (Oey, Lille, Van Loey, & Hendrickx, Citation2008).
Bioactive compounds released from the food matrix during digestion are potentially bio-accessible. There are many reports on the bioactive compounds content extractable from food, but few reports on the effects of extraction methods on their yield and potential bio-accessibility. Patras et al. (Citation2009) reported that in several fruits, anthocyanin degradation may occur after applying high hydrostatic pressure. This is a result of indirect oxidation by the phenolic quinones generated by polyphenol oxidase and peroxidase. Several reports show that HHPE increases the extractability of colored pigments from food, along with the increase in polyphenols (Briones-Labarca, Giovagnoli-Vicuña, Figueroa-Alvarez, Quispe-Fuentes, & Pérez-Won, Citation2013; M. Corrales et al., Citation2008; Margarita Corrales, García, Butz, & Tauscher, Citation2009; Z. Shouqin, Citation2005).
Since high pressure may affect the conformation and/or structure of macromolecules and metabolites, these may or may not exert their biological action (Carbonell-Capella, Buniowska, Barba, Esteve, & Frígola, Citation2014), therefore, the bio-accessibility evaluation from bioactive compounds extracted by HHPE should include the analysis not only of native compounds, but also of their metabolic products. Our results emphasize that the high-pressure effect on anthocyanin content cannot be generalized, although the composition of the matrix, the activity of the oxidative enzymes, the pressure and holding time could compromise the efficiency of the extraction.
shows the effect after in vitro digestion on anthocyanin content. The highest anthocyanin content was obtained after in vitro digestion at 500 MPa for 15 min (23.2 ± 0.9 mg 100g−1 FW) probably because of the permeability effect of HHPE as discussed above and of the solvent which increases anthocyanins solubility. However, HHPE at 500 MPa for 15 min after in vitro digestion resulted in no significant difference in anthocyanin content when compared with CE.
The percentage of potential relative bio-accessibility of anthocyanins was calculated as the ratio between the mean levels of anthocyanins from discarded blueberry extracts before and after the in vitro digestion process. The percentage of bio-accessible anthocyanins in HHPE samples (500 MPa for 5 and 10 min) was significantly lower (p ≤ 0.05) than that in the CE samples. However, the percentage of HHPE at 500 MPa for 15 min after in vitro digestion was the highest compared to CE. In summary, during gastric digestion the stability of anthocyanins in discarded blueberry extracts showed a significant decrease for both extraction methods evaluated. Correa-Betanzo et al. (Citation2014) reported that total anthocyanin content by spectrophotometry and the sum of individual anthocyanin by LC-MS after in vitro digestion were 17.0 and 18.3% when compared to crude extract of wild blueberry, respectively. Bobrich et al. (Citation2014) evaluated the anthocyanin bio-accessibility in plum cultivars, obtaining values of 56% for Black Diamond and 59% for Queen Garnet.
The bio-accessibility of polyphenols, such as anthocyanins, could be limited because these bioactive compounds are degraded during digestion (Carrillo, Buvé, Panozzo, Grauwet, & Hendrickx, Citation2017). Because, during gastric conditions at acidic pH, the anthocyanins are stable, however, the anthocyanins could be degraded by the alkaline pH after the intestinal digestion phase (Bobrich et al., Citation2014; Sengul, Surek, & Nilufer-erdil, Citation2014).
3.3. Antioxidant capacity
The evaluation of food antioxidant capacity is considered to be an important step prior to the definition of the food’s antioxidant phytochemical nature, since a high antioxidant potency warrants the classification of these food products as “super foods” (Li et al., Citation2017).
Antioxidant capacity was estimated by DPPH and FRAP assays. The FRAP ranges extended from 1721 to 2060 μM TE g−1 FW, and those of DPPH from 1778 to 1854 μM TE g−1 FW. The antioxidant capacity of discarded blueberry extracts measured as DPPH and FRAP increased significantly (p ≤ 0.05) with HHPE extraction time, from 1.8, 3.2 and 4.3% for DPPH and from 16.3, 16.9 and 19.7% for FRAP at 500 MPa at 5, 10 and 15 min, respectively, when compared to CE (). These results show a higher antioxidant capacity of discarded blueberries when extracted with HHPE compared to CE.
Table 2. Antioxidant capacity from discarded blueberry extracts measured by DPPH and FRAP after CE (Conventional extraction) and HHPE (High hydrostatic pressure extraction). Different letters in columns indicate significant differences between the mean values (p ≤ 0.05).
Tabla 2. Capacidad antioxidante de extractos de arándanos descartados cuantificada mediante DPPH y FRAP después de CE (extracción convencional) y HHPE (extracción con alta presión hidrostática). Las diferentes letras en las columnas indican diferencias significativas entre los valores medios (p ≤ 0.05).
High hydrostatic pressure extraction allows the extraction of bioactive constituents of plants or herbs at room temperature. When cell membranes are destroyed by high-pressure, a high number of bioactive compounds are extracted from the cells (Joo, Kim, Kim, & Kim, Citation2013). Furthermore, high-pressure may inactivate degrading enzymes, accounting for both higher extraction yields and antioxidant capacity compared to the CE method (Prasad et al., Citation2009b).
The strong antioxidant capacity from discarded blueberry extracts places blueberries as rich in bioactive compounds. Higher antioxidant capacity from samples extracted with high-pressure was reported by (Patras et al., Citation2009), who showed that for pressurized strawberry purée at 500 and 600 MPa, the mean anti-radical power values were higher than those of thermally processed samples. However, for blackberry purées, no significant differences in antioxidant activity values were observed among samples (unprocessed, thermally processed and HHP at 400 and 500 MPa), except at 600 MPa that produced a significant increase (67%) compared to the unprocessed sample (Patras et al., Citation2009).
According to the Pascal theory (Bi, Zhang, Liu, & Wang, Citation2009), pressure transfers to the whole material both uniformly and instantly during the HHPE process. Thus, the equilibration of pressure between the inside and outside of the cells could occur in a very short time. Under these circumstances, the diffusion speed of the solvent is high, allowing bioactive compounds to be rapidly released therefore increasing the antioxidant capacity (Morata et al., Citation2014). Similar results were reported for flavonoids extracted from propolis and litchi (Prasad et al., Citation2009b; Zhang Shouqin et al., Citation2005).
3.4. Total polyphenols and flavonoid content
The total polyphenols content (TPC) of discarded blueberry extracts was used to estimate the flavonoid and non-flavonoid polyphenol compounds.
The TPC content of the discarded blueberry extracts measured as Gallic acid equivalent, increased significantly (p ≤ 0.05) by 8.8, 25.5 and 29.0% after 5, 10 and 15 min of HHPE, respectively, when compared to CE (). Likewise, the TFC measured as quercetin equivalent increased by 11.5, 20.4 and 23.2% at 5, 10 and 15 min of HHPE, respectively, when compared to CE (). The extraction of polyphenols and flavonoids was positively influenced by the pressure time. This increase could result from plant cell disruption caused by high hydrostatic pressure, leading to a higher extractability of these compounds (Queiroz et al., Citation2010; Wang et al., Citation2012). This behavior has also been observed after HHPE in green tea, longan fruit pericarp, Osage-Orange fruit and Korean red ginseng (E.M. Altuner, Işlek, Çeter, & Alpas, Citation2012; Lee et al., Citation2011; Prasad et al., Citation2009b; Xi et al., Citation2009).
Figure 1. (a) Total polyphenols content [mg AGE g −1] and B) flavonoid content [mg QE g − 1] from discarded blueberry extract obtained by CE (Conventional extraction) and HHPE (High hydrostatic pressure extraction). Different letters above the bars indicate significant differences between mean values (p ≤ 0.05).
Figura 1. (a) Contenido total de polifenoles [mg AGE g −1] y B) contenido de flavonoides [mg QE g−1] del extracto de arándano descartado obtenido por CE (extracción convencional) y HHPE (extracción por alta presión hidrostática). Las diferentes letras sobre las barras indican diferencias significativas entre los valores medios (p ≤ 0.05).
![Figure 1. (a) Total polyphenols content [mg AGE g −1] and B) flavonoid content [mg QE g − 1] from discarded blueberry extract obtained by CE (Conventional extraction) and HHPE (High hydrostatic pressure extraction). Different letters above the bars indicate significant differences between mean values (p ≤ 0.05).Figura 1. (a) Contenido total de polifenoles [mg AGE g −1] y B) contenido de flavonoides [mg QE g−1] del extracto de arándano descartado obtenido por CE (extracción convencional) y HHPE (extracción por alta presión hidrostática). Las diferentes letras sobre las barras indican diferencias significativas entre los valores medios (p ≤ 0.05).](/cms/asset/c16bece2-93d5-4c3e-a3ce-5eb99d16250d/tcyt_a_1624622_f0001_b.gif)
The phenolic compounds of discarded blueberry extracts was identified by HPLC. We detected gallic, chlorogenic, caffeic, syringic and rutin acid in the discarded blueberry extracts (). HHPE (5, 10 and 15 min) resulted in an increased amount of caffeic acid whereas the amount of gallic acid, chlorogenic acid and rutin decreased upon HHPE (). Syringic acid was not affected by HHPE. Hydroxycinnamic acids such as ferulic, p-coumaric, and caffeic are present in the cell wall and cytoplasm of plant cells (Faulds & Williamson, Citation1999). We suggest that HHPE induced cell wall hydrolysis, which may have increased caffeic acid release from the discarded blueberries into the extract.
Figure 2. HPLC chromatograms of phenolic compounds from discarded blueberry extracts. A) standard phenolic compounds, B) Bound phenolic compounds, C) Free phenolic compounds. Retention times are in minutes.
Figura 2. Cromatogramas de HPLC de compuestos fenólicos a partir de extractos de arándanos descartados. A) Estándares de Compuestos fenólicos, B) Compuestos fenólicos ligados, C) Compuestos fenólicos libres. Los tiempos de retención se encuentran en minutos.
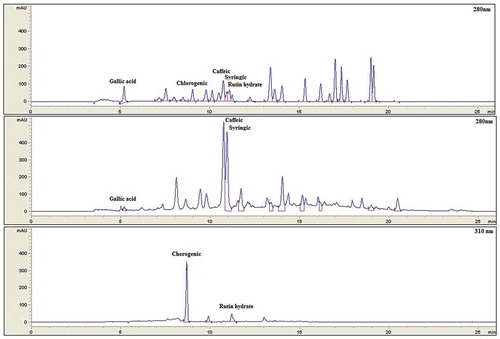
Figure 3. Phenolic compounds in discarded blueberry extracts from CE (Conventional extraction) and HHPE (High hydrostatic pressure extraction). Different letters above the bars indicate significant differences between mean values (p ≤ 0.05),




Figura 3. Compuestos fenólicos en extractos de arándanos descartados obtenidos por CE (extracción convencional) y HHPE (extracción con alta presión hidrostática). Las letras diferentes sobre las barras indican diferencias significativas entre los valores medios (p ≤ 0.05),




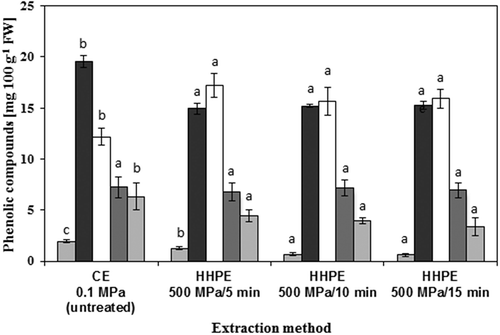
3.5. Antioxidant capacity and in vitro potential bio-accessibility of bioactive compounds from discarded blueberry extracts
Food processing can affect antioxidant components and their capacity in different ways depending on their initial concentration, chemical structure, oxidation state, localization in the cell, possible interactions with other food components and type of processing applied. Moreover, the food matrix, which surrounds nutrients and bioactive compounds like a lattice network is affected by processing, thereby affecting their content and their potential bio-accessibility (Oghbaei & Prakash, Citation2013).
The assessment of the total antioxidant capacity after the application of in vitro gastrointestinal digestion could be a good approximation for determining potential bio-accessibility and bio-availability by preserving the possible synergic, cooperative and competitive activities of the molecules (Jilani, Cilla, Barberá, & Hamdi, Citation2015). shows the potential relative bio-accessibility percentage of antioxidant capacity (DPPH and FRAP assays) and bioactive compounds (polyphenols and flavonoids) obtained by CE and HHPE from discarded blueberry extracts. The potential relative bio-accessibility of antioxidant capacity and bioactive compounds were influenced by in vitro digestion. The potential relative bio-accessibility percentage exhibited in general a significant (p ≤ 0.05) lower antioxidant capacity than the corresponding before in vitro digestion discarded blueberry extracts. The DPPH measured antioxidant capacity of bio-accessible bioactive compounds, there were significant differences between CE and HHPE of discarded blueberry extracts. Antioxidant capacity of discarded blueberry extracts obtained by CE showed the lower potential relative bio-accessibility than HHPE when measured by FRAP. In , the highest relative bio-accessibility values for DPPH and FRAP were observed at 500 MPa for 5 min (85.1% and 60.9%, respectively). As regards the TPC and TFC (), again after in vitro digestion decreased (p < .05) the TPC and TFC values for all extraction methods with respect to values before in vitro digestion. Bio-accessibility of polyphenols and flavonoids was found to be between 55.5%–62.2% and 52.9%–62.2%, respectively.
Table 3. Potential relative bio-accessibility of discarded blueberry extracts obtained by CE (Conventional extraction) and HHPE (High hydrostatic pressure extraction). Different letters in columns indicate significant differences between the mean values (p ≤ 0.05).
Tabla 3. Bio-accesibilidad relativa potencial de extractos de arándanos descartados obtenidos por CE (extracción convencional) y HHPE (extracción por alta presión hidrostática). Las diferentes letras en las columnas indican diferencias significativas entre los valores medios (p ≤ 0.05).
Antioxidant solubility and other physicochemical conditions (pH, oxygen, light, and the matrix composition) during the digestion are some factors to be considered (Rein et al., Citation2013). For example, after in vitro digestion, the polyphenols could have been degraded by the alkaline pH and subsequent decrease in the TPC, TFC and the antioxidant capacity (Bobrich et al., Citation2014). The degradation of bioactive compounds may also come from enzymatic reactions in the sample, resulting in the degradation of bioactive compounds (Amoateng, Assumeng-Koffuor, Sarpong, & Oteng-Agyapong, Citation2011). Likewise, the processing effect on potential bio-accessibility of antioxidant capacity and bioactive compounds can exert a positive or negative effect, depending on the food matrix in the processing conditions (Lafarga, Villaró, Bobo, Simó, & Aguiló-Aguayo, Citation2019).
4. Conclusions
The results show that HHPE is an efficient extraction technique that impacted not only the extractability of bioactive compounds such as anthocyanins and polyphenols but also the antioxidant capacity that resulted from the increased concentration of these molecules in the extracts. The highest yields of bioactive compounds and the strongest antioxidant capacity from discarded blueberry extracts were obtained by HHPE over CE. With a few exceptions, the potential bio-accessibility of bioactive compounds was similar in HHPE and CE samples. Therefore, the extracting conditions examined in this study did not alter the potential bio-accessibility of bioactive compounds and their antioxidant capacity. Given that HHPE provides a higher extraction yield, requires less extraction time and facilitates the production of natural products, the application of HHPE could provide an alternative method to CE for extracting bioactive compounds from blueberries.
Conflicts of interest
The authors declare no have conflict of interest.
Acknowledgments
The authors acknowledge the financial support for this research, which was partly funded by Fondecyt Regular nº1120069, the Research Office of Universidad de La Serena and CONICYT-PCHA/National Doctorate/2016-21160335.
Additional information
Funding
References
- Altuner, E. M., Işlek, C., Çeter, T., & Alpas, H. (2012). High hydrostatic pressure extraction of phenolic compounds from Maclura pomifera fruits. African Journal of Biotechnology, 11(4), 930–937. doi:10.5897/AJB11.2506
- Altuner, E. M., & Tokuşoǧlu, Ö. (2013). The effect of high hydrostatic pressure processing on the extraction, retention and stability of anthocyanins and flavonols contents of berry fruits and berry juices. International Journal of Food Science and Technology, 48(10), 1991–1997. doi:10.1111/ijfs.12185
- Amoateng, P., Assumeng-Koffuor, G., Sarpong, K., & Oteng-Agyapong, K. (2011). Free Radical Scavenging and Anti-lipid Peroxidative Effects of a Hydro-ethanolic Extract of the Whole Plant of Synedrella nodiflora (L.) Gaertn (Asteraceae). Free Radicals and Antioxidants, 1(3), 70–78. doi:10.5530/ax.2011.3.10
- Association of official analytical chemists - AOAC. (1990). In Williams S. (Ed.), Official Method of Analysis (15th ed., pp. 152–164). Washington, DC: Association of Official Analytical Chemists.
- Barnes, J. S., Nguyen, H. P., Shen, S., & Schug, K. A. (2009). General method for extraction of blueberry anthocyanins and identification using high performance liquid chromatography-electrospray ionization-ion trap-time of flight-mass spectrometry. Journal of Chromatography A, 1216(23), 4728–4735.
- Benzie, I. F. F., & Strain, J. J. (1996). The Ferric Reducing Ability of Plasma (FRAP) as a Measure of ‘“ Antioxidant Power ”’: The FRAP Assay. Analytical Biochemistry, 76, 70–76. doi:10.1006/abio.1996.0292
- Bi, H. M., Zhang, S. Q., Liu, C. J., & Wang, C. Z. (2009). High hydrostatic pressure extraction of salidroside from rhodiola sachalinensis. Journal of Food Process Engineering, 32(1), 53–63. doi:10.1111/j.1745-4530.2007.00202.x
- Bobrich, A., Fanning, K. J., Rychlik, M., Russell, D., Topp, B., & Netzel, M. (2014). Phytochemicals in Japanese plums: Impact of maturity and bioaccessibility. Food Research International, 65(PA), 20–26. doi:10.1016/j.foodres.2014.06.030
- Briones-Labarca, V., Giovagnoli-Vicuña, C., & Cañas-Sarazúa, R. (2019). Optimization of extraction yield, flavonoids and lycopene from tomato pulp by high hydrostatic pressure-assisted extraction. Food Chemistry, 278, 751–759. doi:10.1016/j.foodchem.2018.11.106
- Briones-Labarca, V., Giovagnoli-Vicuña, C., Figueroa-Alvarez, P., Quispe-Fuentes, I., & Pérez-Won, M. (2013). Extraction of β-carotene, vitamin C and antioxidant compounds from Physalis peruviana (Cape Gooseberry) assisted by high hydrostatic pressure. Food and Nutrition Science, 2013(August), 109–118. doi:10.4236/fns.2013.48A014
- Carbonell-Capella, J. M., Buniowska, M., Barba, F. J., Esteve, M. J., & Frígola, A. (2014). Analytical methods for determining bioavailability and bioaccessibility of bioactive compounds from fruits and vegetables: A review. Comprehensive Reviews in Food Science and Food Safety, 13(2), 155–171. doi:10.1111/1541-4337.12049
- Carrillo, C., Buvé, C., Panozzo, A., Grauwet, T., & Hendrickx, M. (2017). Role of structural barriers in the in vitro bioaccessibility of anthocyanins in comparison with carotenoids. Food Chemistry, 227, 271–279. doi:10.1016/j.foodchem.2017.01.062
- Chilean Blueberry Committee. (n.d.). Overview global blueberry market | CBBC. Retrieved from http://www.comitedearandanos.cl/overview-global-blueberry-market/
- Corrales, M., García, A. F., Butz, P., & Tauscher, B. (2009). Extraction of anthocyanins from grape skins assisted by high hydrostatic pressure. Journal of Food Engineering, 90(4), 415–421. doi:10.1016/j.jfoodeng.2008.07.003
- Corrales, M., Toepfl, S., Butz, P., Knorr, D., & Tauscher, B. (2008). Extraction of anthocyanins from grape by-products assisted by ultrasonics, high hydrostatic pressure or pulsed electric fields: A comparison. Innovative Food Science and Emerging Technologies, 9(1), 85–91.
- Correa-Betanzo, J., Allen-Vercoe, E., McDonald, J., Schroeter, K., Corredig, M., & Paliyath, G. (2014). Stability and Biological Activity of Wild Blueberry (Vaccinium Angustifolium) Polyphenols During Simulated In Vitro Gastrointestinal Digestion. Food Chemistry, 165, 522–531. doi:10.1016/j.foodchem.2014.05.135
- Cuvelier, B.-W., M.E., & Berset, C. (1995). Use of a Free Radical Method to Evaluate Antioxidant Activity. LWT- Food Science and Technology, 28, 25–30. doi:10.1016/S0023-6438(95)80008-5
- Dini, I., Tenore, G. C., & Dini, A. (2010). Antioxidant compound contents and antioxidant activity before and after cooking in sweet and bitter Chenopodium quinoa seeds. LWT - Food Science and Technology, 43(3), 447–451. doi:10.1016/j.lwt.2009.09.010
- Etcheverry, P., Grusak, M. A., & Fleige, L. E. (2012). Application of in vitro bioaccessibility and bioavailability methods for calcium, carotenoids, folate, iron, magnesium, polyphenols, zinc, and vitamins B 6, B 12, D, and E. Frontiers in Physiology, 3(August), 1–22.
- Faulds, C. B., & Williamson, G. (1999). The role of hydroxycinnamates in the plant cell wall. Journal of the Science of Food and Agriculture, 79(3), 393–395. doi:10.1002/(SICI)1097-0010(19990301)79:3<393::AID-JSFA261>3.0.CO;2-H
- Giusti, M., & Wrolstad, R. E. (2005). Characterization and measurement of anthocyanins by UV- Visible spectroscopy. Current Protocols in Food Analytical Chemistry, 00(1), F1.2.1–F1.2.13. doi:10.1002/0471142913.faf0102s00
- Hidalgo, G.-I., & Almajano, M. (2017). Red Fruits: Extraction of Antioxidants, Phenolic Content, and Radical Scavenging Determination: A Review. Antioxidants, 6(1), 7. doi:10.3390/antiox6010007
- Jilani, H., Cilla, A., Barberá, R., & Hamdi, M. (2015). Biosorption of green and black tea polyphenols into Saccharomyces cerevisiae improves their bioaccessibility. Journal of Functional Foods, 17, 11–21.
- Joo, H., Kim, C.-T., Kim, I.-H., & Kim, Y. (2013). Anti-obesity effects of hot water extract and high hydrostatic pressure extract of garlic in rats fed a high-fat diet. Food and Chemical Toxicology : an International Journal Published for the British Industrial Biological Research Association, 55, 100–105. doi:10.1016/j.fct.2012.12.044
- Khanal, R. C., Howard, L. R., & Prior, R. L. (2010). Effect of heating on the stability of grape and blueberry pomace procyanidins and total anthocyanins. Food Research International, 43(5), 1464–1469. doi:10.1016/j.foodres.2010.04.018
- Kim, D. O., Lee, K. W., Lee, H. J., & Lee, C. Y. (2002). Vitamin C equivalent antioxidant capacity (VCEAC) of phenolic phytochemicals. Journal of Agricultural and Food Chemistry, 50(13), 3713–3717.
- Lafarga, T., Villaró, S., Bobo, G., Simó, J., & Aguiló-Aguayo, I. (2019). Bioaccessibility and antioxidant activity of phenolic compounds in cooked pulses. International Journal of Food Science and Technology, 1–8. doi:10.1111/ijfs.14082
- Lapornik, B., Prošek, M., & Wondra, A. G. (2005). Comparison of extracts prepared from plant by-products using different solvents and extraction time. Journal of Food Engineering, 71(2), 214–222. doi:10.1016/j.jfoodeng.2004.10.036
- Lee, H. S., Lee, H. J., Yu, H. J., Ju, D. W., Kim, Y., Kim, C. T., … Suh, H. J. (2011). A comparison between high hydrostatic pressure extraction and heat extraction of ginsenosides from ginseng (Panax ginseng CA Meyer). Journal of the Science of Food and Agriculture, 91(8), 1466–1473.
- Lee, J., Durst, R. W., & Wrolstad, R. E. (2005). Determination of total monomeric anthocyanin pigment content of fruit juices, beverages, natural colorants, and wines by the pH differential method: Collaborative study. Journal of AOAC International, 88(5), 1269–1278. doi:10.5555/jaoi.2005.88.5.1269
- León-González, A. J., Truchado, P., Tomáss-Barberán, F. A., López-Lázaro, M., Barradas, M. C. D., & Martín-Cordero, C. (2013). Phenolic acids, flavonols and anthocyanins in Corema album (L.) D. Don berries. Journal of Food Composition and Analysis, 29(1), 58–63. doi:10.1016/j.jfca.2012.10.003
- Li, K., Ma, C., Jian, T., Sun, H., Wang, L., Xu, H., … Su, H. (2017). Making good use of the byproducts of cultivation: Green synthesis and antibacterial effects of silver nanoparticles using the leaf extract of blueberry. Journal of Food Science and Technology, 54(11), 3569–3576. doi:10.1007/s13197-017-2815-1
- Martín, J., Kuskoski, E. M., Navas, M. J., & Asuero, A. G. (2017). Antioxidant Capacity of Anthocyanin Pigments. In G. C. Justino (Ed.), Flavonoids. Rijeka: IntechOpen. doi:10.5772/67718.
- Michalska, A., & Łysiak, G. (2015). Bioactive Compounds of Blueberries: Post-Harvest Factors Influencing the Nutritional Value of Products. International Journal of Molecular Sciences, 16(8), 18642–18663. doi:10.3390/ijms160818642
- Minekus, M., Alminger, M., Alvito, P., Ballance, S., Bohn, T., Bourlieu, C., … Brodkorb, A. (2014). A standardised static in vitro digestion method suitable for food – An international consensus. Food & Function, 5(5), 1113–1124. doi:10.1039/c3fo60702j
- Morata, A., Loira, I., Vejarano, R., Bañuelos, M. A., Sanz, P. D., Otero, L., & Suárez-Lepe, J. A. (2014). Grape Processing by High Hydrostatic Pressure: Effect on Microbial Populations, Phenol Extraction and Wine Quality. Food and Bioprocess Technology, 8(2), 277–286. doi:10.1007/s11947-014-1405-8
- Mrduljaš, N., Krešić, G., & Bilušić, T. (2018). Polyphenols: Food Sources and Health Benefits. In M. C. Hueda (Ed.), Functional Food. Rijeka: IntechOpen.
- Nicklett, E., & Kadell, A. (2013). Fruit and Vegetable Intake Among Older Adults: A Scoping Review. Maturitas, 75(4), 305–312. doi:10.1016/j.maturitas.2013.05.005
- Oey, I., Lille, M., Van Loey, A., & Hendrickx, M. (2008). Effect of high-pressure processing on colour, texture and flavour of fruit- and vegetable-based food products: A review. Trends in Food Science and Technology, 19(6), 320–328.
- Oghbaei, M., & Prakash, J. (2013). Effects of processing and digestive enzymes on retention, bioaccessibility and antioxidant activity of bioactive components in food mixes based on legumes and green leaves. Food Bioscience, 4, 21–30. doi:10.1016/j.fbio.2013.08.001
- Paniagua, A. C., East, A. R., Hindmarsh, J. P., & Heyes, J. A. (2013). Moisture loss is the major cause of firmness change during postharvest storage of blueberry. Postharvest Biology and Technology, 79, 13–19. doi:10.1016/j.postharvbio.2012.12.016
- Patras, A., Brunton, N. P., Da Pieve, S., & Butler, F. (2009). Impact of high pressure processing on total antioxidant activity, phenolic, ascorbic acid, anthocyanin content and colour of strawberry and blackberry purées. Innovative Food Science and Emerging Technologies, 10(3), 308–313.
- Prasad, N. K., Yang, B., Zhao, M., Wang, B. S., Chen, F., & Jiang, Y. (2009a). Effects of high-pressure treatment on the extraction yield, phenolic content and antioxidant activity of litchi (Litchi chinensis Sonn.) fruit pericarp. International Journal of Food Science and Technology, 44(5), 960–966. doi:10.1111/j.1365-2621.2008.01768.x
- Prasad, N. K., Yang, E., Yi, C., Zhao, M., & Jiang, Y. (2009b). Effects of high pressure extraction on the extraction yield, total phenolic content and antioxidant activity of longan fruit pericarp. Innovative Food Science & Emerging Technologie, 10, 155–159. doi:10.1016/j.ifset.2008.11.007
- Putnik, P., Bursać Kovačević, D., Ježek, D., Šustić, I., Zorić, Z., & Dragović-Uzelac, V. (2018). High-pressure recovery of anthocyanins from grape skin pomace (Vitis vinifera cv. Teran) at moderate temperature. Journal of Food Processing and Preservation, 42(1), e13342. doi:10.1111/jfpp.2018.42.issue-1
- Que, F., Mao, L., Fang, X., & Wu, T. (2008). Comparison of hot air-drying and freeze-drying on the physicochemical properties and antioxidant activities of pumpkin (Cucurbita moschata Duch.) flours. International Journal of Food Science and Technology, 43(7), 1195–1201.
- Queiroz, C., Moreira, C. F. F., Lavinas, F. C., Lopes, M. L. M., Fialho, E., & Valente-Mesquita, V. L. (2010). Effect of high hydrostatic pressure on phenolic compounds, ascorbic acid and antioxidant activity in cashew apple juice. High Pressure Research, 30(4), 507–513. doi:10.1080/08957959.2010.530598
- Rein, M. J., Renouf, M., Cruz-Hernandez, C., Actis-Goretta, L., Thakkar, S. K., & Da Silva Pinto, M. (2013). Bioavailability of bioactive food compounds: A challenging journey to bioefficacy. British Journal of Clinical Pharmacology, 75(3), 588–602.
- Retamales, J. B., Palma, M. J., Morales, Y. A., Lobos, G. A., Moggia, C. E., & Mena, C. A. (2014). Blueberry Production In Chile : Current Status and Future Developments. Revista Brasileira de Fruticultura, 36(1), 58–67. doi:10.1590/0100-2945-446/13
- Rodríguez-Roque, M. J., Rojas-Graü, M. A., Elez-Martínez, P., & Martín-Belloso, O. (2013). Changes in vitamin C, phenolic, and carotenoid profiles throughout in vitro gastrointestinal digestion of a blended fruit juice. Journal of Agricultural and Food Chemistry, 61(8), 1859–1867. doi:10.1021/jf3044204
- Saura-Calixto, F., Serrano, J., & Goñi, I. (2007). Intake and bioaccessibility of total polyphenols in a whole diet. Food Chemistry, 101(2), 492–501. doi:10.1016/j.foodchem.2006.02.006
- Sengul, H., Surek, E., & Nilufer-erdil, D. (2014). Investigating the effects of food matrix and food components on bioaccessibility of pomegranate (Punica granatum) phenolics and anthocyanins using an in-vitro gastrointestinal digestion model. FRIN. doi:10.1016/j.foodres.2014.05.055
- Shouqin, Z. (2005). Note: effect of high hydrostatic pressure on extraction of flavonoids in propolis. Food Science and Technology International, 11(3), 213–216.
- Shouqin, Z., Jun, X., & Changzheng, W. (2005). High hydrostatic pressure extraction of flavonoids from propolis. Journal of Chemical Technology and Biotechnology, 80(1), 50–54. doi:10.1002/jctb.1153
- Skrovankova, S., Sumczynski, D., Mlcek, J., Jurikova, T., & Sochor, J. (2015). Bioactive compounds and antioxidant activity in different types of berries. International Journal of Molecular Sciences, 16(10), 24673–24706. doi:10.3390/ijms161024673
- Uribe, E., Delgadillo, A., Giovagnoli-Vicuña, C., Quispe-Fuentes, I., & Zura-Bravo, L. (2015). Extraction techniques for bioactive compounds and antioxidant capacity determination of chilean papaya (Vasconcellea pubescens) fruit. Journal of Chemistry, 2015, 1–8. doi:10.1155/2015/347532
- Vega-Gálvez, A., López, J., Torres-Ossandón, M. J., Galotto, M. J., Puente-Díaz, L., Quispe-Fuentes, I., & Di Scala, K. (2014). High hydrostatic pressure effect on chemical composition, color, phenolic acids and antioxidant capacity of Cape gooseberry pulp (Physalis peruviana L.). LWT - Food Science and Technology, 58(2), 519–526. doi:10.1016/j.lwt.2014.04.010
- Wang, Y., Liu, F., Cao, X., Chen, F., Hu, X., & Liao, X. (2012). Comparison of high hydrostatic pressure and high temperature short time processing on quality of purple sweet potato nectar. Innovative Food Science and Emerging Technologies, 16, 326–334.
- Xi, J., Shen, D., Zhao, S., Lu, B., Li, Y., & Zhang, R. (2009). Characterization of polyphenols from green tea leaves using a high hydrostatic pressure extraction. International Journal of Pharmaceutics, 382(1–2), 139–143. doi:10.1016/j.ijpharm.2009.08.023
- Yemmireddy, V. K., Chinnan, M. S., Kerr, W. L., & Hung, Y. C. (2013). Effect of drying method on drying time and physico-chemical properties of dried rabbiteye blueberries. LWT - Food Science and Technology, 50(2), 739–745.
- Zhang, Q. W., Lin, L. G., & Ye, W. C. (2018). Techniques for extraction and isolation of natural products : A comprehensive review. Chinese Medicine, 1–26. doi:10.1186/s13020-018-0177-x