ABSTRACT
The effects of different concentration of flaxseed gum (FG) (0.1–0.5%, w/w) on the stability of soybean oil emulsion were studied by particle size, rheological properties, creaming stability and nuclear magnetic resonance (NMR). Results showed that emulsion particle size decreased significantly with the increase in FG concentration. Rheological measurements showed FG exhibited thickening and gelling properties. Viscosity, storage modulus, and loss modulus increased accordingly with the increase in FG concentrations, and emulsions with 0.5% FG looked like a viscoelastic solid. Emulsions with a higher FG concentration exhibited better creaming stability and structure. With the increase of FG concentration, the 1H and 13C NMR spectra line widths in high field also increased, which confirms that the interaction between FG and oil molecules is enhanced. These results show that FG can substitute for other emulsifiers or stabilizers in emulsions, and is beneficial to the stability of emulsions.
RESUMEN
Se estudiaron los efectos de diferentes concentraciones de goma de linaza (FG) (0.1% - 0.5%, p/p) sobre la estabilidad de la emulsión de aceite de soya, atendiendo el tamaño de partícula, sus propiedades reológicas, la estabilidad de la crema y mediante la aplicación de resonancia magnética nuclear (RMN). Los resultados mostraron que el tamaño de partícula de la emulsión disminuyó significativamente con el aumento de la concentración de FG. Las mediciones reológicas dieron cuenta de que la FG exhibió propiedades espesantes y gelificantes. La viscosidad, el módulo de almacenamiento y el módulo de pérdida aumentaron conforme se incrementaron las concentraciones de FG; las emulsiones con 0.5% de FG mostraron la apariencia de un sólido viscoelástico. Las emulsiones con mayor concentración de FG exhibieron mejor estabilidad y estructura en la formación de crema. Con el aumento de la concentración de FG, también se incrementaron los anchos de línea de los espectros de RMN, 1H y 13C en campo alto, lo que confirma el aumento de la interacción entre la FG y las moléculas de aceite. Estos resultados revelan que la FG puede sustituir a otros emulsionantes o estabilizadores en las emulsiones, siendo beneficiosa para la estabilidad de estas.
1. Introduction
Emulsion is a mixture of two immiscible solutions (usually oil and water), with one liquid dispersed into another insoluble liquid in the form of droplets. In the food industry, many products are based on emulsions, such as milk, cream, sauce and beverages. From a physicochemical point of view, emulsions are thermodynamically unstable systems that could break down over time via Gravity separation, coalescence, flocculation, and phase inversion (Sriprablom, Luangpituksa, Wongkongkatep, Pongtharangkul, & Suphantharika, Citation2019). In practical industrial applications, emulsion stability is of great significance to its formation, production process and storage (Zhao et al., Citation2018). Therefore, how to produce high-quality emulsions that can maintain stability for a certain period of time is of great significance.
Animal fat can give meat good texture and unique flavor, but excessive intake of animal fat will increase the risk of cardiovascular disease, hyperlipidemia and other diseases, so reducing the amount of animal fat in meat products is imperative (Arihara, Citation2006; Vandendriessche, Citation2008). However, the processing characteristics and sensory quality of meat products will be affected by simply reducing the content of animal fat. Fat substitutes have emerged in order to ensure as many characteristics as possible similar to full-fat products. Vegetable oil rich in unsaturated fatty acids is one of the widely studied fat substitutes for meat products. Adding vegetable oil directly is easy to produce oil precipitation, which affects the processing characteristics and quality of meat products. Therefore, the pre-emulsified vegetable oil used to improve the processing stability of vegetable oil has attracted extensive attention of researchers (Ansorena & Astiasarán, Citation2004; Ayo et al., Citation2007; Cáceres, García, & Selgas, Citation2008; Jiménez-Colmenero, Herrero, Pintado, Solas, & Ruiz-Capillas, Citation2010).
Polysaccharides and proteins are the most commonly used emulsifiers and could control the shelf life of emulsions, many studies have confirmed this idea (Derkach, Zhabyko, Voron’Ko, Maklakova, & Dyakina, Citation2015). The emulsifier prepared by single protein or polysaccharide has poor stability and high cost due to improper process conditions. Flaxseed gum is a natural hydrophilic colloid composed of neutral polysaccharide, acid polysaccharide and protein extracted from flaxseed. Like other hydrophilic colloids, FG has good water retention, thickening, rheological and gelation properties. It is considered that 4%~20% of FG proteins have unique emulsifying properties (Cui & Mazza, Citation1996; Hussain et al., Citation2015;Thakur, Mitra, Pal, & Rousseau, Citation2009). In recent years, FG is often used as a new emulsifier in food processing to improve the emulsifying stability of emulsion. Soybean oil contains linoleic acid and unsaturated fatty acid, which can reduce blood lipid and cholesterol and prevent cardiovascular and cerebrovascular diseases. Leguminous phospholipids are beneficial to the development and growth of the brain, nerves and blood vessels. Soybean oil contains palmitic acid, linoleic acid, linoleic acid, vitamin E, vitamin D, vitamin A, carotene, calcium, iron, phosphorus, lecithin and other nutrients, which has high nutritional value and therapeutic and health effects.
The emulsifying effect of FG has been preliminarily studied. Although FG has many good functions, its application in pre-emulsified vegetable oil emulsion has rarely been reported, especially on the emulsifying stability of soybean oil. Nuclear magnetic resonance (NMR), a fast and accurate technique to study emulsions, has been proven valuable, and it can be used to determine the fat and moisture content, the stability and the droplet size distribution of emulsions (Lingwood, Chandrasekera, Kolz, Fridjonsson, & Johns, Citation2012; Opedal, Sørland, & Sjöblom, Citation2010). An important advantage of NMR over other techniques is that it can be used without dilution and pretreatment of emulsions. Moreover, high-field NMR could provide information about functional groups in the form of signal peaks at different chemical shift values (Xie et al., Citation2016). High-field 1H NMR could measure different acyl groups in oil, high-field 13C NMR could provide the positional distribution information of acyl groups in oil (Zamora, Navarro, & Hidalgo, Citation1994). Therefore, NMR is a valuable tool in the field of emulsions.
In this study, we added different concentrations of FG to prepare emulsions and used optical images and traditional parameters, such as particle size, rheological properties, creaming index, to measure the stability of emulsions. To obtain more information, we applied NMR spectroscopy, which may allow us to gain a better understanding of the interaction between FG and oil molecules.
2. Materials and methods
2.1. Materials
FG (powder, purity 99.8%) comes from Lvqi Biotechnology Co., Ltd. (Xinjiang Province, China). Soybean oil was purchased from a local supermarket. Deuterium oxide was produced by Adamas Industrial Corporation (Shanghai, China).
2.2. Sample preparation
FG was dissolved in deionized water and mixed using a magnetic agitator to assure complete hydration. The final FG solution concentration was 0.1%, 0.2%, 0.3%, 0.4%, and 0.5% (w/w). Then, 10% (w/w) soybean oil was added to the prepared FG solution and was homogenized using a high-speed homogenizer (IKA T25-Digital Ultra Turrax, Staufen, Germany) for 2 min at 12,500 rpm. The samples were then passed through a high-pressure homogenizer at 500 MPa and 300 Mpa.
2.3. Measurement of particle size
The particle size of the emulsions was performed using a Malvern Mastersizer 3000 instrument (Malvern Instruments Ltd, Worcestershire, UK). Mean volume diameter D4,3 was used to represent the size of particles.
2.4. Rheological properties
Rheological measurements were performed on a Physica MCR 301 rheometer (Anton Paar, Graz, Austria). The frequency scanning test was carried out at 25°C to record the storage modulus (G’) and loss modulus (G”), the angular frequency range was set to 1–100 rad/s and the strain amplitude was selected as 2%. Apparent viscosity was measured by continuous shear test which was performed at 25°C over the shear rate range of 0.01–100 s−1.
2.5. Emulsion stability
According to Meng Wang’s methods (Wang et al., Citation2017).The creaming stability was calculated by creaming index (%) = 100 × (HS/HE). Where HS is the height of the serum layer, HE is the total height of emulsions.
2.6. Optical microscopy
We placed a drop of emulsion on the glass sides, covered with a coverslip and then quickly put it under a 400 × optical microscope, which was equipped with a CCD camera to capture images.
2.7. High-field NMR (HF-NMR) measurements
Emulsions were prepared with D2O in order to minimize the resonance of water which would interfere the information of spectrum. High-field 1H and 13C NMR spectra were conducted on a 400 MHz Bruker NMR spectrometer (Bruker Group, Fallanden, Switzerland) at 25°C. 1H NMR spectra were acquired by 16 repetitions using spectral width (SW) of 8,012 Hz and acquisition time (AQ) of 4.090 s. 13C NMR spectra were acquired by 1,024 repetitions using SW of 24,038 Hz and AQ of 1.363 s. Chemical shifts are expressed in δ units (ppm).
2.8. Statistical analysis
The data were analyzed using SAS 9.2 for windows by an analysis of variance and Duncan’s multiple range test. The results were presented as the mean value ± standard deviation. P < .05 was as the significance level. All the experiments were repeated three times.
3. Results and discussion
3.1. Measurement of particle size
The value of D4,3 decreased as FG concentration increased from 0.1% to 0.5% (). The decline was significant (p < .05) in emulsions with 0.1–0.4% FG. However, no difference was found between the emulsions at concentrations of 0.4% and 0.5% of FG. The D4,3 values of emulsions with 0.1% FG were 62.45 μm. However, the D4,3 values were only 21.08 μm for emulsions with 0.5% FG, which decreased nearly 66%. These findings indicate that a high FG concentration could contribute to the formation of smaller particle sizes and enhance the stability of emulsions, and these findings are consistent with the results of previous studies (Bai, Huan, Li, & Mcclements, Citation2017; Farshchi, Ettelaie, & Holmes, Citation2013; Wang et al., Citation2017).
Table 1. The average particle size (D4,3, μm) of emulsions stabilized by FG.
Tabla 1. El tamaño medio de partícula (D4,3, μm) de emulsiones estabilizadas por FG.
FG prevented flocculation and coalescence of emulsions by adsorbing on the surface of droplets (Dickinson, Citation2009). With the increase of FG concentration, there are two main reasons for the decrease of average droplet diameter. (i) a low FG concentration was not enough to wrap the surface of the oil droplets, and thus, droplets gathered to decrease the surface area. However, a greater droplet surface area can be covered with enough emulsifier concentration, leading to smaller droplet sizes (Tcholakova, Denkov, & Danner, Citation2004); (ii) emulsions with high emulsifier concentration could cover droplet surfaces more rapidly and reduce the re-coalescence of droplets (Jafari, Assadpoor, He, & Bhandari, Citation2008). Dickinson (Citation2009) also reported that slow migration rate and low colloid concentration are not conducive to the formation of stable emulsions.
3.2. Rheological properties
shows the apparent viscosity of emulsions as a function of shear rate (0.1–100 1/s) with different concentrations of FG. As expected, with the increase of shear rate, the apparent viscosity of the samples decreases, and all the samples exhibit typical shear-thinning flow behavior, which indicates that the samples exhibit pseudoplastic flow behavior. Moreover, when the shear rate increases to a certain extent, the viscosity remains unchanged and is not affected by the shear rate. This finding was consistent with previous studies. (Wang et al., Citation2017; Zhao et al., Citation2015). In fact, the shear thinning behavior of emulsion is related to the properties of continuous phase and dispersed phase (Lorenzo, Zaritzky, & Califano, Citation2008). At lower shear rates, the stretching FG molecules interweave with each other to form aggregates, thus, the flow resistance of continuous phase was relatively strong, and emulsions showed higher apparent viscosity. With the increase of shear rate, the aggregation and arrangement of molecules along the flow direction are gradually destroyed (Oh, So, & Yang, Citation1999). The flow resistance of continuous phase decreased with the increase of shear rate, which reveals the shear thinning behavior. We can also observe from that samples with higher FG concentration had a higher viscosity. Previous studies have shown that the rheological properties of the emulsion are closely related to the stability of the emulsion. (Felix, Romero, & Guerrero, Citation2017; Hu et al., Citation2016). So increased apparent viscosity with increasing FG concentration indicates that FG contributed to the viscosity of the system and to the formation of a more stable emulsion.
Figure 1. Effects of FG concentrations on the apparent viscosity of emulsions.
Figura 1. Efectos de las concentraciones de FG en la viscosidad aparente de las emulsiones.
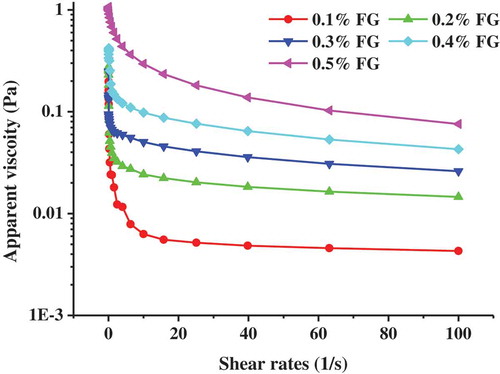
In order to further study the structure of emulsions, we have carried out dynamic frequency scanning on the storage modulus (G ‘) and loss modulus (G’) of emulsion. In general, G’ is related to the elasticity of the emulsion, which reflects the properties of the solid, and G” is related to the viscosity of the emulsion, which represents the properties of the liquid. As can be observed (), an increase in FG concentrations always involves an increase in both G’ and G”. The poorest viscoelastic response was obtained when the FG concentration was the lowest (0.1%). In other words, with the addition of FG, the viscoelasticity increased, which indicated that the rigidity of the samples increased and the structure strengthened (Yuan, Ritzoulis, & Chen, Citation2018). Similar results were extensively observed by previous researchers (Castel, Rubiolo, & Carrara, Citation2017; Felix et al., Citation2017). We can also observe from that in the emulsions with 0.5% FG, G’ was higher than G” throughout the frequency range, indicating that solutions with 0.5% FG showed a gel-like behavior. As reported by Almdal, Dyre, Hvidt, and Kramer (Citation1993), the ideal elastic gel should have the characteristics of G’ > G”, and G’ is independent of frequency. Moreover, G’ and G” in emulsions with 0.5% FG were less dependent on frequency, thereby also suggesting a stronger structure of the samples. The results further showed that the structure of emulsion could be enhanced by adding FG.
3.3. Creaming stability measurement
The creaming stability of the emulsions stabilized by FG was determined after 1 and 24 h of storage (). After 1 h of storage, emulsions with FG concentration greater than 0.3% became resistant to creaming. By contrast, other emulsions were immediately separated into two phases. The creaming stability of emulsions significantly (P < 0.05) improved with the increase in FG concentration after 1 or 24 h after storage given that the creaming index decreased significantly (P < 0.05) with the addition of FG. This phenomenon indicated that FG had an obvious influence on the creaming stability of emulsions and can promote the stability of emulsions, which was in good agreement with the findings of previous researchers (Liang, Wong, Pham, & Tan, Citation2016; Zhao et al., Citation2015).
Figure 3. Effects of FG concentrations on the creaming stability of emulsions. a-d;A-D Different letters indicate significant differences (P < 0.05).
Figura 3. Efectos de las concentraciones de FG en la estabilidad de la crema de las emulsiones.a-d; A-D Una letra diferente indica diferencias significativas (P < 0.05).
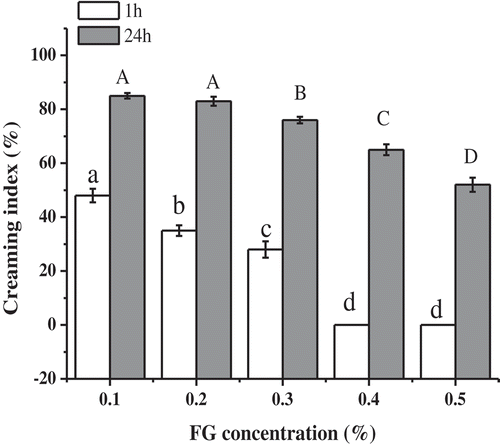
Due to the instability of the emulsion itself, it will be separated into two phases during storage, thus reducing the free energy of the system. And during the storage, many factors, such as flocculation, aggregation, Ostwald ripening may cause emulsion stratification (Dickinson, Citation2009; Zhu, Liu, Chen, Cheng, & Zou, Citation2018). However, because of its comprehensive properties, such as thickener, water retaining agent or gel agent, FG can be widely used in emulsion to improve its stability. Once oil droplets were formed, FG would quickly adsorb on the surface of oil droplets, thus preventing them from colliding with each other to form larger droplets (Dickinson, Citation2009). On the other hand, FG can slowdown or even prevent oil-water separation by increasing the viscoelastic properties of continuous phases. This result was consistent with the findings on higher viscosity in the addition of FG ().
3.4. Optical microscopy
The microscopic images in show the effect of FG concentration on the structure of samples. It can be seen that emulsions with different FG concentration showed great differences in the structure. Larger particle sizes were found in lower FG concentration samples, corroborating our findings regarding particle size (). The extent of flocculation decreased as a function of FG concentration, which was previously reported by other researchers (Sun, Sun, Wei, Liu, & Zhang, Citation2007; Wang et al., Citation2017). At a lower FG concentration, there is not enough FG to cover the surface of oil droplets. Some oil droplet molecules gather together to form large particles under the action of FG, while others are separated into layers on the surface. Thus, the samples were unstable. This phenomenon showed that the concentration of FG has a significant effect on the emulsion. This condition could be explained by the depletion flocculation caused by non-adsorbing FG, which resulted in enhanced serum separation of emulsions (Dickinson, Citation2009). By contrast, the emulsions with a higher FG concentration had a smaller particle size, because sufficient FG was available to wrap the surface of oil droplets, thereby causing the samples to disperse uniformly and have higher stability.
3.5. HF 1H and 13C NMR measurements
In the past decades, high-field 1H and 13C NMR spectroscopy had been widely used to study the structural, spatial, and electronic structures of individual organic compounds (Kononova et al., Citation2017; Rakhmatullin et al., Citation2015). In fact, NMR spectroscopy can also provide a broad opportunity to study the structure of oil dispersions and determine their physicochemical properties. It can measure different acyl groups in oils and fats, and provide useful information for the analysis of emulsion structure (Guillén & Ruiz, Citation2010). It is also a powerful tool for studying the mobility of oil droplets. In our emulsions, considering the higher mobilities of oil droplet molecules in the aqueous phase and at the interface compared with FG, the oil signal was thought to be the dominant signal in the NMR spectra (Day, Xu, Hoobin, Burgar, & Augustin, Citation2007).
shows the high-field 1H NMR spectra distribution of samples with 0.1–0.5% FG. As we can see, samples with different FG concentrations exhibited the same resonance patterns but varying line widths, suggesting that the interaction between oil and FG at the oil–water interface was weaker than that of oil molecules, which means interaction between oil molecules played a major role in the signals. According to previous studies, the increase in line width with increased FG concentration showed a stronger interaction between oil and FG molecules (Day et al., Citation2007). In addition, the increase in line width reduced the mobility of oil molecules, which may be attributed to the increased interaction between oil molecules and FG (Day et al., Citation2007; Wang et al., Citation2017). Previous researches have extensively confirmed the chemical shifts of triglycerides in high-field 1H NMR (Fernández, Hilty, Wider, & Wüthrich, Citation2002; Liu et al., Citation2017). The chemical shifts obtained in HF 1H NMR for the groups on the oil molecules are shown in . As seen from the table, -(CH2)n-group had more freedom of mobility, which suggested it was further away from the interface and had more space to stretch.
Table 2. Chemical shift obtained in HF 1H NMR for the groups on the oil molecules.
Tabla 2. Desplazamiento químico obtenido en HF 1H RMN para los grupos de las moléculas de aceite.
Figure 5. The high-field (HF) 1H NMR spectra of the emulsions in D2O containing 10% soybean oil with different FG concentration of: (a) 0.1%, (b) 0.2%, (c) 0.3%, (d) 0.4%, (e) 0.5%.
Figura 5. Los espectros de RMN 1H de campo alto (HF) de las emulsiones en D2O que contienen 10% de aceite de soya con diferentes concentraciones de FG de: (A) 0.1%, (B) 0.2%, (C) 0.3%, (D) 0.4%, (E) 0.5%.
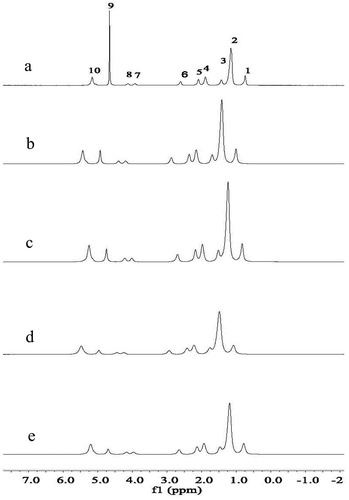
High-field 13C NMR can be used to confirm the acyl positional distribution of oils, which was superior to the high-field 1H NMR (R. Zamora et al., Citation1994). shows the 13C NMR spectra of samples with different FG concentrations. As we can see, emulsions with 0.1% FG displayed weak 13C spectra, and many signal peaks were not scanned. This phenomenon may be due to the poor stability of emulsions. In contrast, samples with 0.2–0.5% FG displayed strong 13C spectra and all of them appeared similar, with the differences being seen only in the line width and height of peak. For a certain peak, the linewidth was positively correlated with FG concentration. For example, when the chemical shift is 22.53 ppm, the linewidth of samples containing 0.2%-0.5% FG is 5.42, 7.24, 8.53 and 9.72, respectively. This finding was consistent with the results observed in the high-field 1H NMR spectra, indicating the increased interaction between FG and oil molecules with increased FG concentration. Moreover, the emulsions with higher FG concentration displayed a smoother baseline in the spectra, thereby potentially serving as a reference to evaluate the stability of emulsions.
Figure 6. The high-field (HF) 13C NMR spectra of the emulsions in D2O containing 10% soybean oil with different FG concentration of: (a) 0.1%, (b) 0.2%, (c) 0.3%, (d) 0.4%, (e) 0.5%.
Figura 6. El espectro de RMN 13C de campo alto (HF) de las emulsiones en D2O que contiene 10% de aceite de soya con diferente concentración de FG de: (a) 0.1%, (b) 0.2%, (c) 0.3%, (d) 0.4%, (e) 0.5%.
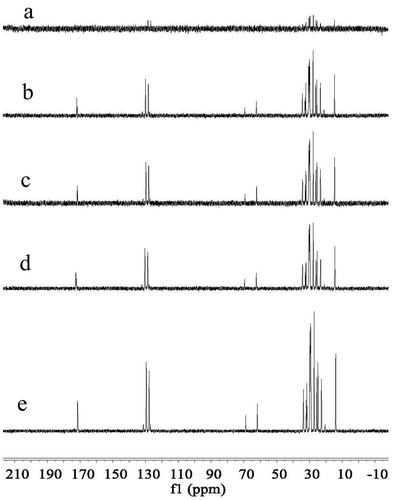
4. Conclusions
In our research, FG has been proved to function as an effective stabilizer for oil-in-water emulsions. D4,3 decreased significantly with the increase in FG concentration, indicating that a higher FG concentration could improve the stability of emulsions. As for apparent viscosity, all samples exhibited a typical shear thinning flow behavior. Similar to apparent viscosity, G’ and G” showed an uptrend with the increase in FG, and samples with 0.5% FG behaved like a viscoelastic solid. The creaming stability of emulsions improved with the increase in FG concentration. Microscopic images also confirmed that higher FG could form a more stable emulsion. High-field NMR spectra provided information on the mobility of oil molecules and the interaction between oil and FG at the interface. With the increase in FG concentration, the line widths in the high-field 1H and 13C NMR spectra increased, confirming the increased interaction between FG and oil molecules. In addition, -(CH2)n- was confirmed as the most mobile group and furthest away from the oil/FG interface. These findings indicate that FG can stabilize emulsions and that a 0.05% concentration corresponds to better stability of emulsions.
Disclosure statement
No potential conflict of interest was reported by the authors.
Additional information
Funding
References
- Almdal, K., Dyre, J., Hvidt, S., & Kramer, O. (1993). Towards a phenomenological definition of the term ‘gel’. Polymer Gels & Networks, 1, 5–17. doi:10.1016/0966-7822(93)90020-I
- Ansorena, D., & Astiasarán, I. (2004). The use of linseed oil improves nutritional quality of the lipid fraction of dry-fermented sausages. Food Chemistry, 87, 69–74. doi:10.1016/j.foodchem.2003.10.019
- Arihara, K. (2006). Strategies for designing novel functional meat products. Meat Science, 74, 219–229. doi:10.1016/j.meatsci.2006.04.028
- Ayo, J., Carballo, J., Serrano, J., Olmedilla-Alonso, B., Ruiz-Capillas, C., & Jiménez-Colmenero, F. (2007). Effect of total replacement of pork backfat with walnut on the nutritional profile of frankfurters. Meat Science, 77, 173–181. doi:10.1016/j.meatsci.2007.02.026
- Bai, L., Huan, S., Li, Z., & Mcclements, D. J. (2017). Comparison of emulsifying properties of food-grade polysaccharides in oil-in-water emulsions: Gum arabic, beet pectin, and corn fiber gum. Food Hydrocolloids, 66, 144–153. doi:10.1016/j.foodhyd.2016.12.019
- Cáceres, E., García, M. L., & Selgas, M. D. (2008). Effect of pre-emulsified fish oil as source of PUFA n-3 on microstructure and sensory properties of mortadella, a Spanish bologna type sausage. Meat Science, 80, 183–193. doi:10.1016/j.meatsci.2007.11.018
- Castel, V., Rubiolo, A. C., & Carrara, C. R. (2017). Droplet size distribution, rheological behavior and stability of corn oil emulsions stabilized by a novel hydrocolloid (Brea gum) compared with gum arabic. Food Hydrocolloids, 63, 170–177. doi:10.1016/j.foodhyd.2016.08.039
- Cui, W., & Mazza, G. (1996). Physicochemical characteristics of flaxseed gum. Food Research International, 29, 397–402. doi:10.1016/0963-9969(96)00005-1
- Day, L., Xu, M., Hoobin, P., Burgar, I., & Augustin, M. A. (2007). Characterisation of fish oil emulsions stabilised by sodium caseinate. Food Chemistry, 105, 469–479. doi:10.1016/j.foodchem.2007.04.013
- Derkach, S., Zhabyko, I., Voron’Ko, N., Maklakova, A., & Dyakina, T. (2015). Stability and the rheological properties of concentrated emulsions containing gelatin–κ-carrageenan polyelectrolyte complexes. Colloids & Surfaces A Physicochemical & Engineering Aspects, 483, 216–223. doi:10.1016/j.colsurfa.2015.04.007
- Dickinson, E. (2009). Hydrocolloids as emulsifiers and emulsion stabilizers. Food Hydrocolloids, 23, 1473–14829. doi:10.1016/j.foodhyd.2008.08.005
- Farshchi, A., Ettelaie, R., & Holmes, M. (2013). Influence of pH value and locust bean gum concentration on the stability of sodium caseinate-stabilized emulsions. Food Hydrocolloids, 32, 402–411. doi:10.1016/j.foodhyd.2013.01.010
- Felix, M., Romero, A., & Guerrero, A. (2017). Influence of pH and Xanthan Gum on long-term stability of crayfish-based emulsions. Food Hydrocolloids, 72, 372–380. doi:10.1016/j.foodhyd.2017.06.018
- Fernández, C., Hilty, C., Wider, G., & Wüthrich, K. (2002). Lipid-protein interactions in DHPC micelles containing the integral membrane protein OmpX investigated by NMR spectroscopy. Proceedings of the National Academy of Sciences of the United States of America, 99, 13533–13537. doi:10.1073/pnas.212515099
- Guillén, M. D., & Ruiz, A. (2010). 1H nuclear magnetic resonance as a fast tool for determining the composition of acyl chains in acylglycerol mixtures. European Journal of Lipid Science & Technology, 105, 502–507. doi:10.1002/ejlt.200300799
- Hu, H. Y., Xing, L. J., Hu, Y. Y., Qiao, C. L., Wu, T., Zhou, G. H., & Zhang, W. G. (2016). Effects of regenerated cellulose on oil-in-water emulsions stabilized by sodium caseinate. Food Hydrocolloids, 52, 38–46. doi:10.1016/j.foodhyd.2015.06.019
- Hussain, M., Bakalis, S., Gouseti, O., Zahoor, T., Anjum, F. M., & Shahid, M. (2015). Dynamic and shear stress rheological properties of guar galactomannans and its hydrolyzed derivatives. International Journal of Biological Macromolecules, 72, 687–691. doi:10.1016/j.ijbiomac.2014.09.019
- Jafari, S. M., Assadpoor, E., He, Y., & Bhandari, B. (2008). Re-coalescence of emulsion droplets during high-energy emulsification. Food Hydrocolloids, 22(7), 1191-1202. doi:10.1016/j.foodhyd.2007.09.006
- Jiménez-Colmenero, F., Herrero, A., Pintado, T., Solas, M. T., & Ruiz-Capillas, C. (2010). Influence of emulsified olive oil stabilizing system used for pork backfat replacement in frankfurters. Food Research International, 43, 2068–2076. doi:10.1016/j.foodres.2010.06.010
- Kononova, O., Litvinov, R. I., Blokhin, D. S., Klochkov, V. V., Weisel, J. W., Bennett, J. S., & Barsegov, V. (2017). Mechanistic basis for the binding of RGD- and AGDV-peptides to the platelet integrin αIIbβ3. Biochemistry, 56(13), 1932–1942. doi:10.1021/acs.biochem.6b01113
- Liang, Y., Wong, S. S., Pham, S. Q., & Tan, J. J. (2016). Effects of globular protein type and concentration on the physical properties and flow behaviors of oil-in-water emulsions stabilized by micellar casein–Globular protein mixtures. Food Hydrocolloids, 54(Part A), 89–98. doi:10.1016/j.foodhyd.2015.09.024
- Lingwood, I. A., Chandrasekera, T. C., Kolz, J., Fridjonsson, E. O., & Johns, M. L. (2012). Emulsion droplet sizing using low-field NMR with chemical shift resolution and the block gradient pulse method. Journal of Magnetic Resonance, 214, 281–288. doi:10.1016/j.jmr.2011.11.020
- Liu, Q., Wu, J. E., Zhi, Y. L., Aggarwal, A., Yang, H., & Wang, S. (2017). Evaluation of the metabolic response of Escherichia coli to electrolysed water by 1 H NMR spectroscopy. LWT - Food Science and Technology, 79, 428–436. doi:10.1016/j.lwt.2017.01.066
- Lorenzo, G., Zaritzky, N., & Califano, A. (2008). Modeling rheological properties of low-in-fat o/w emulsions stabilized with xanthan/guar mixtures. Food Research International, 41, 487–494. doi:10.1016/j.foodres.2008.02.005
- Oh, M. H., So, J. H., & Yang, S. M. (1999). Rheological evidence for the silica-mediated gelation of Xanthan Gum. Journal of Colloid & Interface Science, 216, 320–328. doi:10.1006/jcis.1999.6325
- Opedal, N. V. D. T., Sørland, G., & Sjöblom, J. (2010). Emulsion stability studied by Nuclear Magnetic Resonance (NMR). Energy & Fuels : an American Chemical Society Journal, 24, 3628–3633. doi:10.1021/ef100268x
- Rakhmatullin, I. Z., Galiullina, L. F., Garipov, M. R., Strel’Nik, A. D., Shtyrlin, Y. G., & Klochkov, V. V. (2015). Dynamic NMR study of dinitrophenyl derivatives of seven-membered cyclic ketals of pyridoxine. Magnetic Resonance in Chemistry, 53, 805–812. doi:10.1002/mrc.4251
- Sriprablom, J., Luangpituksa, P., Wongkongkatep, J., Pongtharangkul, T., & Suphantharika, M. (2019). Influence of pH and ionic strength on the physical and rheological properties and stability of whey protein stabilized o/w emulsions containing xanthan gum. Journal of Food Engineering, 242, 141–152. doi:10.1016/j.jfoodeng.2018.08.031
- Sun, W., Sun, D., Wei, Y., Liu, S., & Zhang, S. (2007). Oil-in-water emulsions stabilized by hydrophobically modified hydroxyethyl cellulose: Adsorption and thickening effect. Journal of Colloid & Interface Science, 311(1), 228–236. doi:10.1016/j.jcis.2007.02.082
- Tcholakova, S., Denkov, N. D., & Danner, T. (2004). Role of surfactant type and concentration for the meandrop size during emulsification in turbulent flow. Langmuir the Acs Journal of Surfaces & Colloids, 20, 7444–7458. doi:10.1021/la049335a
- Thakur, G., Mitra, A., Pal, K., & Rousseau, D. R. (2009). Effect of flaxseed gum on reduction of blood glucose and cholesterol in type 2 diabetic patients. International Journal of Food Sciences & Nutrition, 60(s6), 126–136. doi:10.1080/09637480903022735
- Vandendriessche, F. (2008). Meat products in the past, today and in the future. Meat Science, 78, 104–113. doi:10.1016/j.meatsci.2007.10.003
- Wang, M., Feng, M. Q., Jia, K., Sun, J., Xu, X. L., & Zhou, G. H. (2017). Effects of flaxseed gum concentrations and pH values on the stability of oil-in-water emulsions. Food Hydrocolloids, 6(7), 54–62. doi:10.1016/j.foodhyd.2017.01.004
- Xie, Y., Chen, J., Zhang, S., Fan, K., Chen, G., Zhuang, Z., … Yang, L. (2016). The research about microscopic structure of emulsion membrane in O/W emulsion by NMR and its influence to emulsion stability. International Journal of Pharmaceutics, 500(s1–2), 110–119. doi:10.1016/j.ijpharm.2016.01.032
- Yuan, B., Ritzoulis, C., & Chen, J. (2018). Extensional and shear rheology of a food hydrocolloid. Food Hydrocolloids, 74, 296–306. doi:10.1016/j.foodhyd.2017.08.019
- Zamora, R., Navarro, J. L., & Hidalgo, F. J. (1994). Identification and classification of olive oils by high-resolution13C nuclear magnetic resonance. Journal of the American Oil Chemists’ Society, 71, 361–364. doi:10.1007/BF02540514
- Zhao, Q., Long, Z., Kong, J., Liu, T., Sun-Waterhouse, D., & Zhao, M. (2015). Sodium caseinate/flaxseed gum interactions at oil–Water interface: Effect on protein adsorption and functions in oil-in-water emulsion. Food Hydrocolloids, 43, 137–145. doi:10.1016/j.foodhyd.2014.05.009
- Zhao, S., Tian, G., Zhao, C., Li, C., Bao, Y., DiMarco-Crook, C., … Zheng, J. (2018). The stability of three different citrus oil-in-water emulsions fabricated by spontaneous emulsification. Food Chemistry, 269, 577–587. doi:10.1016/j.foodchem.2018.07.062
- Zhu, Y. Q., Liu, W., Chen, X., Cheng, C., & Zou, L. Q. (2018). Advances in stabilization mechanism and stability control of food grade Pickering emulsion. Science & Technology of Food Industry, 39, 315–322.