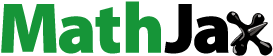
ABSTRACT
The morphological difference between two types of tapioca flour motivated this research, which aims to evaluate the hygroscopic behavior of these products via moisture sorption isotherms (MSI). The MSI of the products were obtained at 25°C, 35°C, 45°C, and 55°C and the experimental data were fitted at seven mathematic models that concern the effect of temperature. MSI had type-II behavior and hysteresis were observed between the moisture adsorption and desorption isotherms, whose magnitudes decreased with higher temperatures. According to adsorption, the two flour are microbiologically stable if they have moisture below 11% and they are less susceptible to becoming moist if they are stored at relative humidity below 70%. The MSI indicated that the tapioca flour more expanded is more hygroscopic and with higher moisture decreases as temperature increases, suggesting distinct storage conditions for the two flour. The modified Oswin model had a good fit to the sorption data.
RESUMEN
La presente investigación se centró en determinar la diferencia morfológica existente entre dos tipos de harina de tapioca. En este sentido, su objetivo fue evaluar el comportamiento higroscópico de estos dos tipos del producto mediante las isotermas de sorción de humedad (MSI). Las MSI de las harinas de tapioca se obtuvieron a 25, 35, 45 y 55°C y los datos experimentales se ajustaron a siete modelos matemáticos referidos al efecto de la temperatura. Las MSI tuvieron un comportamiento tipo II, observándose histéresis entre las isotermas de adsorción y desorción de humedad, cuya magnitud disminuyó a temperaturas más altas. Según la adsorción, las dos harinas son microbiológicamente estables si la humedad es inferior al 11% y son menos susceptibles a humedecerse si se almacenan a una humedad relativa inferior al 70%. Las MSI indicaron que la harina de tapioca más expandida es más higroscópica y, a mayor humedad, la MSI se redujo con el aumento de la temperatura, lo cual da cuenta de la existencia de condiciones de almacenamiento distintas para las dos harinas. El modelo modificado de Oswin se ajustó bien a los datos de sorción.
PALABRAS CLAVE:
1. Introduction
Cassava (Manihot esculenta Crantz) is one of the most widely grown plants worldwide, particularly in the tropics, and stands out as one of the main crops in Brazil (Léotard et al., Citation2009), where it is broadly used as human and animal food (Franck et al., Citation2011; Nwokocha, Aviara, Senan, & Willians, Citation2009). It is a valuable crop, mainly for the economies of countries in development, due to the versatility of in natura and industrialized sub-products and by-products (Zanetti, Cardoso, Dourado, Reina, & Muraishi, Citation2014). Brazil is the fourth-largest producer of cassava in the world, after Nigeria, Thailand, and Indonesia. In the growing season of 2016, 23.71 million tons of cassava roots were harvested. Currently, the Pará state leads the cassava production in Brazil, followed by Paraná and Bahia (Conab Citation2017).
In turn, cassava is more susceptible to spoilage than other tuberous roots such as potato, sweet potato, yam, and corn in regular environmental conditions (An, Yang, & Zhang, Citation2012). This has been attributed to the fact that in the root of cassava, the storage organ, has no dormancy, has no function in propagation and possesses no bud primordia from which regrowth can occur (Cooke, Rickard, & Thompson, Citation1988). Thus, cassava is best used with the aid of appropriate preservation technologies, especially those that reduce water activity (aw) and that limit microorganism action and product spoilage (Dias & Leonel, Citation2006; Falade & Akingbala, Citation2010). The main product obtained from cassava is flour, whose types include tapioca flour (Adebowale, Sanni, & Onitilo, Citation2008).
Tapioca flour is a granulated product obtained from the starch of cassava, which has uneven, polyhedral, or spherical granules (Chisté, Silva, Lopes, & Pena, Citation2012). To obtain the product, the starch with 40% moisture is manually rubbed over an extended cloth into a frame, for the formation of the granules, which are classified into a 3.35 mm mesh (Silva, Cunha, Lopes, & Pena, Citation2013b). According to Silva, Cunha, Lopes, and Pena (Citation2013a), the product may have two forms: tapioca flour expanded type – ET and tapioca flour rounded shape – RS. To obtain tapioca flour ET, the starch granules are submitted to scolding in a metallic plate at ≈ 180°C for 5 min followed by sitting at room temperature for 24 h. Scolding gellifies starch and forms an impermeable layer on the surface of the granules, which prevents water diffusion from within the granules during roasting (≈240°C) and causes their rupture and expansion. In tapioca flour RS, the starch granules are directly submitted to the roasting step (≈240°C), which reduces the possibility of the formation of the impermeable layer on the surface of the granules. Since that favors the diffusion and evaporation of the water contained in the granules, their rounded shape is maintained. Silva et al. (Citation2013a) reported that the apparent densities of the products are 100 kg/m3 (tapioca flour ET) and 610 kg/m3 (tapioca flour RS).
The hygroscopicity study based on moisture adsorption and desorption isotherms of a product provides important information for process development and optimization; helps select packaging which maximize the retention of aroma, color, texture, nutrients, and biological stability; as well as allows estimating product stability over storage (Van Den Berg & Bruin, Citation1981). Additionally, knowing the effect of temperature on moisture sorption is very important as it may significantly affect equilibrium conditions and lead to changes in moisture and aw (Labuza, Tannenbaum, & Karel, Citation1970). Mathematical models that contemplate the effect of temperature on moisture sorption behavior, such as the modified equations of Chung-Pfost, Halsey, Henderson, GAB, and Oswin have been used to represent moisture sorption isotherms of different products (Argyropoulos, Alex, Kohler, & Müller, Citation2012; He et al., Citation2013; Jamali, Kouhila, Mohamed, Idlimam, & Lamharrar, Citation2006).
Chisté et al. (Citation2012) investigated moisture sorption isotherms for tapioca flour; however, the study assessed the sorption behavior of only a single flour type (tapioca flour ET) and at a single temperature (25°C). As a more effective contribution for the study of the hygroscopicity of tapioca flour, the present research aimed to obtain the moisture sorption isotherms of both types of tapioca flour at different temperatures in order to indicate processing and storage conditions for the products.
2. Materials and methods
2.1. Raw material
Samples of both types of tapioca flour (1 kg each) were purchased in the municipalities of Santa Izabel (01°17ʹ55ʹ’ S and 48°09ʹ38ʹ’ W) (tapioca flour ET) and Santarém (02°26ʹ35ʹ’ S and 54°42ʹ30ʹ’ W) (tapioca flour RS), both in Pará, Brazil. Each flour type is largely produced and consumed in these localities. The samples were packaged with low-density polyethylene (LDPE) film under vacuum and room temperature (≈27°C) until the analyses. Granulometry analysis showed the flour had mean particle size of 4.46 mm (tapioca flour ET) and 2.41 mm (tapioca flour RS).
2.2. Physicochemical characterization of tapioca flour
The recommended methods of the Association of Official Analytical Chemists [AOAC] (Citation2010) were adopted to determine the moisture, ashes, total lipids, total proteins (5.75 nitrogen-protein conversion factor), total sugars, and starch contents. The water activity (aw) was determined at 25°C with a digital thermohygrometer (AquaLab 4TE, Decagon, USA). All analyses were performed in triplicate and the results were presented as the replicates mean.
2.3. Obtaining the sorption isotherms
For the hygroscopicity study of the flour, moisture adsorption and desorption isotherms were built at 25°C, 35°C, 45°C, and 55°C in order to simulate bland and extreme conditions of storage for the product. The isotherms were obtained in a vapor sorption analyzer (Aqualab VSA, Decagon, Puma, WA, USA) using the DVS (dynamic vapor sorption) method, which consists of monitoring the moisture and aw values of a sample exposed to environments with different relative humidity (RH) levels. The different RH levels are generated by injection of dry and saturated vapor.
In order to obtain the sorption isotherms, the sample was initially submitted to complementary dehydration in a desiccator with silica gel under vacuum at working temperature for 24 h (Souza, Souza, & Pena, Citation2013). Next, an approximately 1 g sample was weighed in a stainless-steel capsule in the microanalytical balance of the VSA. To obtain equilibrium data, the sample was submitted to different levels of RH induced by changes in the injection of dry and saturated vapor. The data were obtained for an aw range between 0.1 and 0.9 used as convergence criterion for the equilibrium of two consecutive measurements with dm/dt ≥0.05, where dm/dt is the ratio between mass variation and time variation between consecutive measurements.
2.4. Monolayer and storage time of the flour determination
The moisture of the monolayer (M0) for the adsorption and desorption processes was determined from the linear and angular coefficients of the straight obtained through the linear regression of the aw/(1-aw)M versus aw correlation, using the linearized form of the BET equation (Equation (1)) (Brunauer, Emmet, & Teller, Citation1938).
where M = equilibrium moisture (g H2O/100 g dry basis – d.b.); aw = water activity (dimensionless); M0 = monolayer moisture content (g H2O/100 g d.b.); and C = constant related to the heat of sorption of the first layer on primary sites.
Equation (2) proposed by Costa, Carmo, and Pena (Citation2018) was used to estimate the storage time required for the tapioca flour to reach critical moisture. This equation involves both packaging and product properties, which are easily determined.
where t = storage time of the product (days); M = product mass in packaging (g); mmax = maximum moisture to be reached by the product (g H2O/100 g d.b.); mi = initial moisture content of the product (g H2O/100 g); Φ = water vapor permeability of the packaging material (g H2O/m2.day); A = packaging contact area (m2).
2.5. Mathematical modeling of sorption isotherms
The mathematical models presented ahead (Equations (3)–(9)), which concern the effect of temperature, were adjusted to the experimental moisture sorption data of the flour. Goodness of fit was assessed using coefficient of determination (R2), root mean square error (RMSE) (Equation (10)), and mean relative deviation (P) (Equation (11)). The trend of the distribution of residues regarding the fits was also assessed (Equation (12)).
Modified Chung-Pfost (Pfost, Mourer, Chung, & Milliken, Citation1976):
Copace (Corrêa, Martins, & Melo, Citation1995):
Modified Halsey (Iglesias & Chirife, Citation1976b):
Modified Henderson (Thompson, Peart, & Foster, Citation1968):
Modified GAB (Jayas & Mazza, Citation1993):
Modified Oswin (Chen & Morey, Citation1989):
Sigma Copace (Corrêa, Martins, Christ, & Mantovani, Citation1998):
where M = equilibrium moisture (g H2O/100 g d.b.); aw = water activity (dimensionless); T = temperature (K); and a, b, c, M0, C, and k are the models’ parameters.
where = predicted equilibrium moisture (g H2O/100 g d.b.);
= experimental equilibrium moisture (g H2O/100 g d.b.); N = number of experimental determinations; eave = residue (g H2O/100 g d.b.).
2.6. Statistical analysis
The sorption models were fitted by non-linear regression using Statistica 7.0 software and the Levenberg-Marquardt algorithm with a convergence criterion of 10−6. The models whose fits had R2 values close to 1, low RMSE and P values, and random distribution of residues (Arslan & Togrul, Citation2005; Lomauro, Bakshi, & Labuza, Citation1985) were considered effective. Trends in residue distribution indicate the model under- or overestimates the real condition in some regions, which makes it inappropriate to represent the phenomenon investigated (Draper & Smith, Citation1998). According to Peng, Chen, Wu, and Jiang (Citation2007), P values lower than 10% can be adopted as an indicative of a good fit for practical purposes.
3. Results and discussion
3.1. Moisture sorption of tapioca flour
Tapioca flour ET and tapioca flour RS studied had 11.30% and 9.0% moisture, 0.08% and 0.05% ashes, 0.33% and 0.14% lipids, 0.25% and 0.36% proteins, 0.94% and 0.98% total sugars, 82.77% and 80.82% starch and 0.62 and 0.46 aw, respectively. Similar values of composition were observed by Chisté et al. (Citation2012) and Silva et al. (Citation2013a) for this product. The fiber crude content in this flour type varies between 0.20% and 0.22% (Ijioma, Ihediohanma, Okafor, Ofoedu, & Ojimba, Citation2016).
The moisture sorption data for tapioca flour at different temperatures are shown in . The moisture sorption isotherms obtained from these data are presented in , showing the hysteresis phenomenon, and in , highlighting the effect of temperature. The adsorption isotherms indicate ET and RS tapioca flour are microbiologically stable (aw < 0.6) (Jay, Citation2005) when stored between 25°C and 55°C if their moisture levels are up to 14.7% d.b. (12.8% wet basis – w.b.) and 12.4% d.b. (11.0% w.b.), respectively. These results indicate tapioca flour ET required greater care during storage. A value in the same order of magnitude was observed for cassava flour of the dry and water groups (11.3% d.b.) (Chisté, Cardoso, Silva, & Pena, Citation2015).
Table 1. Moisture sorption data of the two tapioca flour at different temperatures.
Tabla 1. Datos de sorción de humedad de las dos harinas de tapioca a diferentes temperaturas.
Figure 1. Moisture adsorption ((○) tapioca flour ET; (●) tapioca flour RS) and desorption ((∆) tapioca flour ET; (▲) tapioca flour RS) isotherms at (a) 25°C; (b) 35°C; (c) 45°C; (d) 55°C.
Figura 1. Adsorción de humedad ((○) harina de tapioca ET; (●) harina de tapioca RS) y desorción ((∆) harina de tapioca ET; (▲) isotermas de harina de tapioca RS) a (a) 25°C; (b) 35°C; (c) 45°C; (d) 55°C.
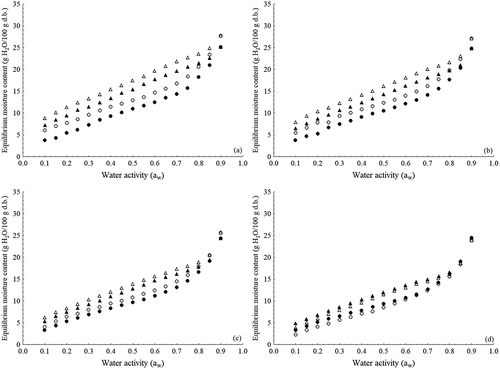
Figure 2. Influence of temperature on moisture adsorption ((a) tapioca flour ET; (b) tapioca flour RS) and desorption ((c) tapioca flour ET; (d) tapioca flour RS) isotherms. Experimental ((○) 25°C; (□) 35°C; (◊) 45°C; (∆) 55°C) and predicted values using the modified Oswin model (line).
Figura 2. Influencia de la temperatura en la adsorción de humedad ((a) harina de tapioca ET; (b) harina de tapioca RS) y desorción ((c) harina de tapioca ET; (d) isotermas de harina de tapioca RS). Valores experimentales ((○) 25°C; (□) 35°C; (◊) 45°C; (∆) 55°C) y pronosticados utilizando el modelo (línea) modificado de Oswin.
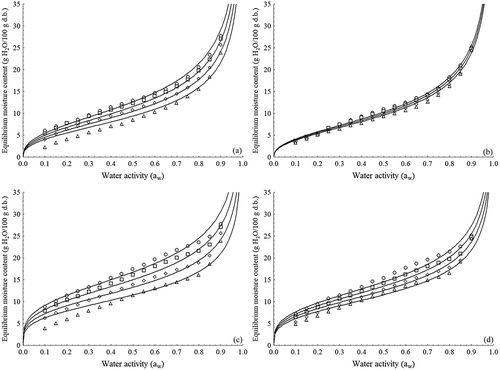
According to the BET classification, the isotherms of the tapioca flour in the temperature range studied had type-II behavior (sigmoidal shape) () (Iglesias & Chirife, Citation1982). It is known that water molecules are strongly bound to hydrophilic biopolymers like proteins and polysaccharides, such as starch. Starchy foods show more Langmuir-like type-II isotherm Blahovec and Yanniotis (Citation2009). Thus, although the product presents other biopolymers in the composition, like fibers and proteins, the sigmoid behavior is primarily attributed to the starch, being the major constituent. Type-II moisture sorption isotherms have been also observed for other cassava-derived products (Ayala-Aponte, Citation2016; Chisté et al., Citation2015, Citation2012; Mishra & Rai, Citation2006; Perdomo et al., Citation2009).
The hysteresis loop observed between the moisture adsorption and desorption isotherms of the flour comprehended the monolayer region until approximately 0.85 aw while its amplitude reduced as temperature increased (). Studies involving starchy products such as rice (Arslan & Togrul, Citation2006; Bingol, Prakash, & Pan, Citation2012), corn flour (Oyelade, Tunde-Akintundeb, Igbekac, Okeb, & Rajid, Citation2008), and millet (Raji & Ojediran, Citation2011) exhibited a hysteresis loop nearly throughout the entire aw range.
For constant aw, the moisture of ET and RS flour decreased as temperature increased, an effect that was more representative of the former than the latter. The behavior observed is explained by the excitation state of water molecules, which increases with temperature, leading to lower sorption and, consequently, lower product moisture (McLaughlin & Magee, Citation1998). Studies on other starchy products such as cassava flour (Farias, Ferreira, Conceição, & Bona, Citation2010) and cassava starch (Ayala-Aponte, Citation2016) reported the same behavior observed in the present research for the effect of temperature on moisture sorption isotherms.
The tapioca flour ET had higher moisture content than the tapioca flour RS for a constant aw and temperature (). The greater affinity for the water molecules of the first one is due to its mean particle size – 4.46 mm (more expanded), since it is submitted to scolding and has smaller density than tapioca flour RS (Silva et al., Citation2013a). Regarding the effect of temperature, the sorption isotherms of this latter were closest (,), in other words, lower moisture decreases (0.7% b.s.) in relation to tapioca flour ET (3.8% b.s.) as function of temperature increases (25–55°C).
The adsorption isotherms had linear behavior up to aw of 0.7, after which product moisture increased exponentially (). This behavior indicated that, regardless of type, tapioca flour requires greater care when stored or handled in an environment with RH above 70%. Under such conditions, the product should be stored in packaging with low water vapor permeability.
The monolayer moisture (M0) of the tapioca flour varied from 5.4 to 7.3 g H2O/100 g d.b for adsorption and 6.7 to 9.6 g H2O/100 g d.b. for desorption (R2 > 0.987). The value of M0 decreased as temperature increased, which was attributed to a reduction in the number of active sites due to physical and chemical changes induced by temperature (Iglesias & Chirife, Citation1976a). According to Labuza (Citation1984), foods with M0 ≤ 10% d.b. are considered stable. Thus, tapioca flour is considered a product with good stability if stored between 25°C and 55°C. Values of M0 in the same order of magnitude were observed for cassava starch (4.4–7.3 g H2O/100 g d.b.) (Ayala-Aponte, Citation2016) and for cassava flour of groups water (7.9 g H2O/100 g d.b.) and dry (9.0 g H2O/100 g d.b.) at 25°C (Chisté et al., Citation2015).
LDPE is the most commonly employed material to package flour and grains (Hernandez, Selke, & Culter, Citation2016; Sarantópoulos & Teixeira, Citation2017). Hence, considering that 500 g tapioca flour are stored in a 22 cm x 17 cm LDPE package, Equation (2) is used to estimate the storage time required for the product to reach critical moisture. The water vapor permeability value of 4.94 g H2O/m2.day estimated by Alves, Ito, Carvalho, Melo, and Godoy (Citation2012) for 80 µm thick LDPE film exposed to 38°C and 90% RH was used.
The storage time was estimated as 24 days for ET flour with 11.3% moisture and 31 days for RS flour with 9.0% moisture. These are the limit times for the flour to reach moisture levels after which the microbiological stability of the products cannot be guaranteed (aw > 0.6) if the product is stored in LDPE packaging. It is worth pointing out that 38°C and 90% RH are extreme conditions for food storage.
3.2. Mathematical modeling of sorption isotherms
According to the values of the statistics used to assess goodness of fit (R2, P and RMSE) and on the random distribution of the residues (), the modified Oswin model best described the moisture adsorption and desorption processes of the tapioca flour in the experimental domain. The isotherms generated by the modified Oswin model are presented in . Overall, the literature indicates the GAB and Halsey models as having the best fits to the moisture sorption data of starchy products such as potato starch (Al-muhtaseb, McMinn, & Magee, Citation2004), cassava flour (Chisté et al., Citation2015; Farias et al., Citation2010), rice (Bingol et al., Citation2012), tapioca flour (Chisté et al., Citation2012), and cassava (Koua, Koffi, Ghaba, & Toure, Citation2014).
Table 2. Statistical parameters of mathematical modeling of moisture sorption data of tapioca flour.
Tabla 2. Parámetros estadísticos del modelado matemático de los datos de sorción de la humedad de la harina de tapioca.
4. Conclusion
The hygroscopic assessment of tapioca flour expanded type (ET) and tapioca flour rounded shape (RS) showed the moisture sorption isotherms of the products are type II (sigmoid) and that the microbiological stability between 25°C and 55°C is assured if the moisture of the products is below 12.8% for the former and 11.0% for the latter. In order to meet such conditions, the storage time of the products at 38°C and 90% RH packaged in low-density polyethylene film was estimated as 24 and 31 days, respectively. The tapioca flour ET has grater affinity with water molecules than tapioca flour RS, as function of the larger surface area of the former. Moreover, the tapioca flour ET presents higher moisture decreases as temperature increases, so this flour requires greater control during handling and storage. Finally, the modified Oswin model can be used with good precision to describe the sorption isotherms of both flour.
Acknowledgments
The authors acknowledge Coordenação de Aperfeiçoamento de Pessoal de Nível Superior (CAPES, Brazil) for the scholarship of J. R. Carmo.
Disclosure statement
The authors have no conflict of interest.
Additional information
Funding
References
- Adebowale, A. A., Sanni, L. O., & Onitilo, M. O. (2008). Chemical composition and pasting properties of tapioca grits from different cassava varieties and roasting methods. African Journal of Food Science, 2, 77–82.
- Al-muhtaseb, A. H., McMinn, W. A. M., & Magee, T. R. A. (2004). Water sorption isotherms of starch powders. Part II: Thermodynamic characteristics. Journal of Food Engineering, 62(2), 135–142. doi:10.1016/S0260-8774(03)00202-4
- Alves, R. M. V., Ito, D., Carvalho, J. L. V., Melo, W. F., & Godoy, R. L. O. (2012). Stability of biofortified sweet potato flour. Brazilian Journal of Food Technology, 15(1), 59–71. doi:10.1590/S1981-67232012000100007
- An, D., Yang, J., & Zhang, P. (2012). Transcriptome profiling of low temperature treated cassava apical shoots showed dynamic responses of tropical plant to cold stress. BMC Genomics, 13–64. doi:10.1186/1471-2164-13-64
- Argyropoulos, D., Alex, R., Kohler, R., & Müller, J. (2012). Moisture sorption isotherms and isosteric heat of sorption of leaves and stems of lemon balm (Melissa officinalis L.) established by dynamic vapor sorption. LWT – Food Science and Technology, 47(2), 324–331. doi:10.1016/j.lwt.2012.01.026
- Arslan, N., & Togrul, H. (2005). Modelling of water sorption isotherms of macaroni stored in a chamber under controlled humidity and thermodynamic approach. Journal of Food Engineering, 69(2), 133–145. doi:10.1016/j.jfoodeng.2004.08.004
- Arslan, N., & Togrul, H. (2006). Moisture sorption behavior and thermodynamic characteristics of rice stored in a chamber under controlled humidity. Biosystems Engineering, 95(2), 181–195. doi:10.1016/j.biosystemseng.2006.06.011
- Association of Official Analytical Chemists (AOAC). (2010). Official methods of analysis of Association of Official Analytical Chemists International (18th ed.). Arlington, VA: Author.
- Ayala-Aponte, A. A. (2016). Thermodynamic properties of moisture sorption in cassava flour. DYNA, 83(197), 139–145. doi:10.15446/dyna.v83n197.51543
- Bingol, G., Prakash, B., & Pan, Z. (2012). Dynamic vapor sorption isotherms of medium grain rice varieties. LWT – Food Science and Technology, 48(2), 156–163. doi:10.1016/j.lwt.2012.02.026
- Blahovec, J., & Yanniotis, S. (2009). Modified classification of sorption isotherms. Journal of Food Engineering, 91(1), 72–77. doi:10.1016/j.jfoodeng.2008.08.007
- Brunauer, S., Emmet, T. H., & Teller, F. (1938). Adsorption of gases in multimolecular layers. Journal of the American Chemical Society, 60(2), 309–319. doi:10.1021/ja01269a023
- Chen, C., & Morey, R. V. (1989). Comparison of four EMC/ERH equations. Transactions of the ASAE, 32(3), 983–990. doi:10.13031/2013.31103
- Chisté, R. C., Cardoso, J. M., Silva, D. A., & Pena, R. S. (2015). Hygroscopic behaviour of cassava flour from dry and water groups. Ciência Rural, 45(8), 1515–1521. doi:10.1590/0103-8478cr20140338
- Chisté, R. C., Silva, P. A., Lopes, A. S., & Pena, R. S. (2012). Sorption isotherms of tapioca flour. International Journal of Food Science and Technology, 47(4), 870–874. doi:10.1111/j.1365-2621.2011.02900.x
- Conab – Companhia Nacional de Abastecimento. (2017). Mandioca: Levantamento safra 2015-2016. Retrieved from http://www.conab.gov.br
- Cooke, R., Rickard, J., & Thompson, A. (1988). The storage of tropical root and tuber crops cassava, yam and edible aroids. Experimental Agriculture, 24(4), 457–470. doi:10.1017/S0014479700100201
- Corrêa, P. C., Martins, D. S. R., & Melo, E. C. (1995). Umigrãos: Programa para o cálculo do teor de umidade de equilíbrio para os principais produtos agrícolas. Viçosa, Brasil: Centro Nacional de Treinamento em Armazenagem.
- Corrêa, P. C., Martins, J. H., Christ, D., & Mantovani, B. H. M. (1998). Curvas de dessorção e calor latente de vaporização para as sementes de milho pipoca (Zea mays). Revista Brasileira De Engenharia Agrícola E Ambiental, 2(1), 75–79. doi:10.1590/1807-1929/agriambi.v2n1p75-79
- Costa, T. S., Carmo, J. R., & Pena, R. S. (2018). Powdered tucupi condiment: Sensory and hygroscopic. Food Science and Technology, 38(1), 33–40. doi:10.1590/1678-457X.36816
- Dias, L. T., & Leonel, M. (2006). Phisico-chemical characteristics of cassava flours from different regions of Brazil. Ciência E Agrotecnologia, 30(4), 692–700. doi:10.1590/S1413-70542006000400015
- Draper, N., & Smith, H. (1998). Applied regression analysis (3rd ed.). New York, NY: Wiley.
- Falade, K., & Akingbala, J. (2010). Utilization of cassava for food. Food Reviews International, 27(1), 51–83. doi:10.1080/87559129.2010.518296
- Farias, J. F., Ferreira, M. F., Conceição, W. A. S., & Bona, E. (2010). Determination of the water sorption isotherms for cassava flour. Brazilian Journal of Food Research, 1(2), 83–87. doi:10.14685/rebrapa.v1i2.21
- Franck, H., Christian, M., Nöel, A., Brigitte, P., Joseph, H. D., Cornet, D., & Mathurin, N. C. (2011). Effects of cultivar and harvesting conditions (age, season) on the texture and taste of boiled cassava roots. Food Chemistry, 126(1), 127–133. doi:10.1016/j.foodchem.2010.10.088
- He, X., Lau, A. K., Sokhansanj, S., Lim, C. J., Bi, X. T., Melin, S., & Keddy, T. (2013). Moisture sorption isotherms and drying characteristics of aspen (Populus tremuloides). Biomass & Bioenergy, 57, 161–167. doi:10.1016/j.biombioe.2013.07.007
- Hernandez, R. J., Selke, S. E., & Culter, J., . D. (2016). Plastics packaging – Properties, processing, applications and regulations (3rd ed.). Cincinnati, OH: Munich Hanser.
- Iglesias, H. A., & Chirife, C. (1982). Handbook of isotherms. New York, NY: Academic Press.
- Iglesias, H. A., & Chirife, J. (1976a). Local isotherm concept and modes of moisture binding in food products. Journal of Agricultural and Food Chemistry, 24(1), 77–79. doi:10.1021/jf60203a048
- Iglesias, H. A., & Chirife, J. (1976b). Prediction of the effect of temperature on water sorption isotherms of food material. Journal of Food Technology, 11, 109–116. doi:10.1111/j.1365-2621.1976.tb00707.x
- Ijioma, B. C., Ihediohanma, N. C., Okafor, D. C., Ofoedu, C. E., & Ojimba, C. N. (2016). Physical, chemical and sensory attributes of tapioca grits from different cassava varieties. Asian Journal of Agriculture and Food Sciences, 4, 46–53. doi:10.13140/RG.2.2.14481.33129
- Jamali, A., Kouhila, K., Mohamed, L. A., Idlimam, A., & Lamharrar, A. (2006). Moisture adsorption-desorption isotherms of Citrus reticulata leaves at three temperatures. Journal of Food Engineering, 77(1), 71–78. doi:10.1016/j.jfoodeng.2005.06.045
- Jay, M. J. (2005). Microbiologia de alimentos (6th ed.). Porto Alegre, Brasil: Artmed.
- Jayas, D. S., & Mazza, G. (1993). Comparison of five three-parameter equations for the description of adsorption data of oats. Transactions of the ASAE, 36, 119–125. doi:10.13031/2013.28322
- Koua, B. K., Koffi, P. M. E., Ghaba, P., & Toure, S. (2014). Thermodynamic analysis of sorption isotherms of cassava (Manihot esculenta). Journal of Food Science and Technology, 51(9), 1711–1723. doi:10.1007/s13197-012-0687-y
- Labuza, T. P. (1984). Application of chemical kinetics to deterioration of foods. Journal of Chemical Education, 61(4), 348–358. doi:10.1021/ed061p348
- Labuza, T. P., Tannenbaum, S. R., & Karel, M. (1970). Water content and stability of low moisture and intermediate moisture foods. Food Technology, 24(5), 35–42.
- Léotard, G., Duputié, A., Kjellberg, F., Douzery, E. J. P., Debain, C., Granville, J. J., & McKey, D. (2009). Phylogeography and the origin of cassava: New insights from the northern rim of the Amazonian basin. Molecular Phylogenetics and Evolution, 53(1), 329–334. doi:10.1016/j.ympev.2009.05.003
- Lomauro, C. J., Bakshi, A. S., & Labuza, T. P. (1985). Evaluation of food moisture sorption isotherm equations. Part I. Fruit, vegetable and meat products. LWT – Food Science and Technology, 18(2), 111–117.
- McLaughlin, C. P., & Magee, T. R. A. (1998). The determination of sorption isotherm and the isosteric heats of sorption for potatoes. Journal of Food Engineering, 35(3), 267–280. doi:10.1016/S0260-8774(98)00025-9
- Mishra, S., & Rai, T. (2006). Morphology and functional properties of corn, potato and tapioca starches. Food Hydrocolloids, 20(5), 557–566. doi:10.1016/j.foodhyd.2005.01.001
- Nwokocha, L. M., Aviara, N. A., Senan, C., & Willians, P. A. (2009). A comparative study of some properties of cassava (Manihot esculenta Crantz) and cocoyam (Colocasia esculenta, Linn) starches. Carbohydrate Polymers, 76(3), 362–367. doi:10.1016/j.carbpol.2008.10.034
- Oyelade, O. J., Tunde-Akintundeb, T. Y., Igbekac, J. C., Okeb, M. O., & Rajid, O. Y. (2008). Modelling moisture sorption isotherms for maize flour. Journal of Stored Products Research, 44(2), 179–185. doi:10.1016/j.jspr.2007.10.005
- Peng, G., Chen, X., Wu, W., & Jiang, X. (2007). Modeling of water sorption isotherm for corn starch. Journal of Food Engineering, 80(2), 562–567. doi:10.1016/j.jfoodeng.2006.04.063
- Perdomo, J., Cova, A., Sandoval, A. J., García, L., Laredo, E., & Müller, A. J. (2009). Glass transition temperatures and water sorption isotherms of cassava starch. Carbohydrate Polymers, 76(2), 305–313. doi:10.1016/j.carbpol.2008.10.023
- Pfost, H. B., Mourer, S. G., Chung, D. S., & Milliken, G. A. (1976). Summarizing and reporting equilibrium moisture data for grains. Saint Joseph, MI: ASAE.
- Raji, A. O., & Ojediran, J. O. (2011). Moisture sorption isotherms of two varieties of millet. Food and Bioproducts Processing, 89(3), 178–184. doi:10.1016/j.fbp.2010.06.001
- Sarantópoulos, C. I. G. L., & Teixeira, F. G. (2017). Embalagens Plásticas Flexíveis: Principais polímeros e avaliação de propriedades (2nd ed.). Campinas, Brasil: CETEA/ITAL.
- Silva, P. A., Cunha, R. L., Lopes, A. S., & Pena, R. S. (2013a). Characterization of tapioca flour obtained in Pará state, Brazil. Ciência Rural, 43(1), 185–191. doi:10.1590/S0103-84782012005000130
- Silva, P. A., Cunha, R. L., Lopes, A. S., & Pena, R. S. (2013b). Obtainment of tapioca flour: Part 1 - Process evaluation. Boletim Centro De Pesquisa De Processamento De Alimentos, 31(1), 13–24.
- Souza, T. C. L., Souza, H. A. L., & Pena, R. S. (2013). A rapid method to obtaining moisture sorption isotherms of a starchy product. Starch/Stärke, 65(5–6), 433–436. doi:10.1002/star.201200184
- Thompson, T. L., Peart, R. M., & Foster, G. H. (1968). Mathematical simulation of corn drying – A new model. Transactions of the ASAE, 24, 582–586. doi:10.13031/2013.39473
- Van Den Berg, C., & Bruin, S. (1981). Water activity and its estimation in food systems: Theoretical aspects. In L. B. Rockland & G. F. Stewart (Eds.), Water activity: Influences on food quality (pp. 2–61). New York, NY: Academic Press.
- Zanetti, E. G. B., Cardoso, E. M. G., Dourado, D. P., Reina, E., & Muraishi, C. T. (2014). Performance of two cassava varieties submitted to different spacings, grown in the Cerrado region. Brazilian Journal of Applied Technology for Agricultural Science, 7, 39–46. doi:10.5935/PAeT.V7.N1.04