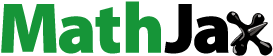
ABSTRACT
This study was carried out to investigate the contribution of two strains of Lactobacilli; Lactobacillus casei 431 and Lactobacillus acidophilus La-5 to the volatile organic compounds (VOCs) in fermented milk. The samples were subjected to the headspace-solid phase micro-extraction (HS-SPME) and gas chromatography mass spectrometry (GC-MS). Prior to the HS-SPME, four different silica fibres were screened for their ability to retain VOCs in the fermented milk. The fibre that retained the highest amount of VOCs was selected and used for the subsequent analyses. A total of 105 compounds made up of a wide range of VOCs such as acids, alcohols, aldehydes, aromatic compounds, esters and ketones were identified. The ketones were the most abundant group of compounds produced by the two strains of lactic acid bacteria (LAB) investigated. In addition, L. casei 431 was able to produce higher concentrations of typical flavour compounds like 2,3-butanedione, 2-heptanone, acetoin and 2-nonanone compared to L acidophilus La-5. As such, the ability of these strains to produce these distinct typical flavour compounds suggests their potential as starter cultures for the production of fermented dairy products with enhanced flavour/or aroma.
RESUMEN
El presente estudio se propuso investigar la contribución que hacen dos cepas de Lactobacilli, Lactobacillus casei 431 y Lactobacillus acidophilus La-5, a los compuestos orgánicos volátiles (VOC) en la leche fermentada. Las muestras fueron sometidas a microextracción en fase sólida de espacio de cabeza (HS-SPME) y a espectrometría de masas por cromatografía de gases (GC-MS). Antes de realizar la HS-SPME se examinaron cuatro diferentes fibras de sílice con la finalidad de determinar su capacidad de retener los VOC en la leche fermentada. Así, se seleccionó la fibra que retuvo mayor cantidad de VOC para utilizarla en análisis posteriores. Se identificaron 105 compuestos integrados por una amplia gama de VOC, a saber: ácidos, alcoholes, aldehídos, compuestos aromáticos, ésteres y cetonas. El grupo más abundante de compuestos producidos por las dos cepas de bacterias del ácido láctico (LAB) investigadas fue el de cetonas. El segundo grupo más copioso fue el de ácidos. Los compuestos derivados de aldehídos, alcoholes y ésteres tuvieron escasa presencia. Además, en comparación con L. acidophilus La-5, L. casei 431 produjo concentraciones más altas de compuestos saborizantes típicos como 2,3-butanodiona, 2-heptanona, acetoína y 2-nonanona. En conclusión, la capacidad de las cepas investigadas para producir distintos compuestos de sabores típicos sugiere su potencial para ser empleadas como cultivos iniciadores destinados a la elaboración de productos lácteos fermentados con sabor/aroma mejorado.
1. Introduction
Health-conscious consumers are constantly demanding for dairy products with high nutritional quality and sensory satisfaction. Whilst consumers are more interested in products that are low in fat and sugar, overall flavour quality remains the core determinant for their food choices (Potts & Peterson, Citation2019). Lactic acid bacteria (LAB) play a major role in the production of characteristic flavours during fermentation and storage that affects the quality and acceptability of fermented dairy products. The overall flavour of fermented milk is a combination of non-volatile and volatile organic compounds (Engels et al., Citation1997). The nature and type of aroma compounds produced by Lactobacillus sp often depends on the species and strains used (Cui et al., Citation2019). The compounds responsible for the aroma are quite diverse and they include; alcohols, aldehydes, esters, fatty acids, ketone and sulphur compounds among others (Beshkova et al., Citation2003; Bozoudi et al., Citation2015; Dan, Wang, Wu et al., Citation2017; Marilley & Casey, Citation2004; G. Smit et al., Citation2005; Valduga et al., Citation2010). In the same way, the precursors are as diverse as the chemical classes of the aroma compounds.
The precursors include the three main components of food matter namely carbohydrates, lipids and proteins. In addition, aroma formation is a complex process involving the generation of precursors and their subsequent conversion into aroma compounds (Smid & Kleerebezem, Citation2014). These aroma compounds which often results from amino acid catabolism by LAB (Yvon & Rijnen, Citation2001) generally impart favourable flavours to the fermented milk (Lin et al., Citation2016). The amino acid converting ability of LAB varies greatly from strain to strain (G. Smit et al., Citation2005). Therefore, an adequate selection of LAB based on metabolic activity is required to produce dairy products with good flavour, adequate acidity and viscosity. The flavour of a dairy product is extremely complex due to the great number of compounds present which have different polarities, volatilities and occurs in a wide range of concentrations (Lasekan et al., Citation2013). Therefore, sample preparation such as extraction and concentration of compounds remains a critical area in volatile analysis. Continuous liquid-liquid extraction has been used in sample preparation for the determination of dairy volatiles (Xanthopoulos et al., Citation1994). Other extraction methods include; ultrasound (Abesinghe et al., Citation2019), supercritical fluid extraction-gas chromatography (Tuomala & Kallio, Citation1996) and headspace solid-phase micro-extraction (HS-SPME) (Dan, Wang, Jin et al., Citation2017).
HS-SPME has become a popular technique widely used to analyse volatile compounds in several matrices, among them, fermented milks (Lasekan et al., Citation2016). SPME coupled with gas chromatography mass spectrometry (SPME-GC-MS) are commonly used for the qualitative and quantitative analysis of volatile organic compounds (VOCs) in various dairy products (Condurso et al., Citation2008; Tunick et al., Citation2013). The volatilomic potentials of Lactobacillus sp. has been reported in many studies in which different species and strain were used (Irlinger et al., Citation2017). For example, L. casei has been shown to produce 2,3-butanedione (diacetyl) and acetoin, that gives the buttery flavor in fermented dairy products (Cui et al., Citation2019). L. acidophilus has the ability to produce acetaldehyde and diacetyl. The amounts of acetaldehyde produced by L. acidophilus have an impact on the flavor of dairy products (Østlie et al., Citation2003). The individual LAB strains’ ability to produce an array of different volatile compounds is a powerful tool for selecting cultures with special flavor attributes (Cuffia et al., Citation2019). VOCs of fermented milk have been widely studied in recent years. However to the best of our knowledge, there are no reported studies on the volatilomic potential of these two strains of LAB (i.e. Lactobacillus acidophilus La-5 and Lactobacillus casei 431).
The purpose of the present study was to characterize the volatile compound profiles of two strains of LAB (Lactobacillus acidophilus La-5 and Lactobacillus casei 431) during milk fermentation, using SPME-GC-MS technique.
2. Materials and methods
2.1. Bacterial isolates and chemicals
Lactobacillus sp. (L. acidophilus La-5 and L. casei 431) was obtained from Chr. Hansen A/S as frozen direct-to-vat starters (DVS) (Gayrettepe, Kuala Lumper, Malaysia). De Man Rogosa Sharpe, (MRS) broth (Merck, Germany) and C8-C40 n-alkanes were obtained from Merck chemicals (Darmstadt, Germany). Each strain of the frozen LAB was propagated in 10 mL MRS broth in three successive transfers at 37°C for 24 h. To separate bacteria, the cultures were centrifuged at 5000 g /15 min. The biomass was washed twice (2×) with distilled water. The LAB were subsequently inoculated into 100 mL of reconstituted skim milk (12% w/v), then incubated at 37°C for 24 h as preculture to obtain approximately ~108 colony forming units (CFU)/mL. Three inoculation treatments were made. Each of the pre-cultures was inoculated (2% v/v) separately into the pasteurized 1 L whole milk and incubated at 37°C for 24 h. The obtained fermented milk products (~ pH 4.6) were stored for volatile analysis at 4°C.
2.2. SPME fibre screening
Different silica fibres [StableFlex polydimethylsiloxane (PDMS), 100 μm; StableFlex polydimethylsiloxane/ divinylbenzene (PDMS/DVB), 65 μm; StableFlex polydimethylsiloxane/ carboxen (PDMS/CAR), 75 μm; StableFlex divinylbenzene/ carboxen/polydimethylsiloxane (DVB/CAR/PDMS), 50/30 μm] obtained from Supelco (Bellefonte, PA, USA) were chosen for the optimization study. Previously, all fibres were conditioned according to the manufacturer’s instructions. Each fibre was exposed to the headspace of the milk fermented by L. casei 431 using similar temperature and time (50°C/20 min). Desorption was carried out for 15 min at 250°C. All analyses were performed in triplicate. The fibre that retained the highest amount of VOCs was selected and used for the subsequent analyses. For that, the chromatographic profiles of the volatile compounds were integrated and the peak area values were averaged and expressed as arbitrary units
2.3. HS-SPME optimization
A factorial central composite rotatable design (CCRD) was used to optimize HS-SPME conditions (Chatterjee et al., Citation2012). For CCRD design, N is the number of experiments which consists of 2 n factorial points and 2 n axial points (α = 1.4142) and nc central points. n is the number of independent variables. The temperature extraction (°C) and extraction time (min) were chosen as variables for the optimization and the levels of each variable are shown in . The responses for the total peak area of VOCs analysed were correlated using a second order polynomial model EquationEquation (1)(1)
(1)
Table 1. Factors, levels and experimental domain of the conditions applied to optimize the extraction by HS-SPME.
Tabla 1. Factores, niveles y dominio experimental de las condiciones aplicadas para optimizar la extracción mediante HS-SPME
Where y is the forecasted response; βo: a constant; βi: the linear coefficient; βii: the quadratic term coefficient; βij: the interaction coefficient and ε: the error associated with the model. The obtained data are presented in and respectively.
Table 2. Experimental conditions and values of response (total area) obtained for the CCRD for the HS-SPME optimization.
Tabla 2. Condiciones experimentales y valores de respuesta (área total) obtenidos para el CCRD de la optimización mediante HS-SPME
2.4. Headspace solid-phase micro-extraction of fermented milk
About 5.0 g of the fermented milk was weighed into a 20 mL vial sealed with a screw-top cap and a PTFE/Silicon septum (Fisher Scientific Inc., Ireland Ltd). The HS-SPME analysis was carried out according to the design established in . A PDMS/CAR fibre selected from the optimization study () was exposed to the headspace of samples. Then the fibre was inserted into the GC injector port provided with 0.75 mm i.d. liner, and the analytes were thermally desorbed at 250°C for 5 min (split-less mode).
Figure 1. The total peak area of compounds obtained by different fibre coatings. Each fibre was exposed to the headspace of the fermented milk by L. casei 431 for 20 min and 50°C.
Figura 1. Área de pico total de compuestos obtenidos mediante diferentes recubrimientos de fibra. Cada fibra fue expuesta al espacio de cabeza de la leche fermentada por L. casei 431 durante 20 minutos a 50°C
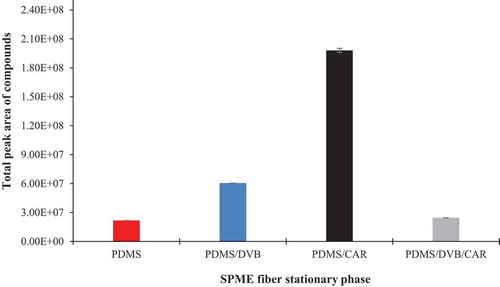
2.5. GC-MS analysis
The method described by Aunsbjerg et al. (Citation2015) was employed for the GC-MS analyses. The tentative identification of VOCs extracted by HS-SPME method from the two types of fermented milk products was carried out using a GC-MS System, Agilent 5890 (Agilent, Stockport, UK) instrument equipped with a GC-17A ver.3 GC with a flame ionization detector (FID) (Shimadzu, Japan), operating in a split-less mode. The fibre was kept in the injector at 250°C for 5 min, with helium gas (purity 99.99%) as carrier gas at a constant flow rate of 1.0 mL/min during desorption. The volatile compounds were separated using a ZEBRON ZB-FFAP column (Agilent, UK), 50 m 0.2 mm internal diameter
0.33 µm film thickness (Crawford Scientific, UK). The oven temperature program was as follow: initial temperature was 40 °C, held for 1 min, increased at 5°C /min to 70°C, and finally 5°C/min to 100°C. The final temperature was then ramped at 10°C/min to 240°C to allow a run time of 30 min. The mass spectrometry was set to record 33–450 atomic mass units, at a sampling rate of 1.11 scans s−1.
2.6. Identification of volatile compounds
Volatiles were tentatively identified by comparing their mass spectra with the mass spectral database (NIST Standard Reference Database 1A V17, Agilent Technologies Inc.) and Kovats retention index (RI). Calculation of the retention indices was carried out by Kovats method (EquationEquation 2(2)
(2) ), using a normal paraffin mixture (C8-C40) as external references (Lasekan & Hussein, Citation2018).
Where RI (x) is the retention index of the unknown compound (x); x: unknown compound that is being analysed; z: the number of carbon atoms of the n-alkane eluted before (x); y: the number of carbon atoms of the retention time n-alkane eluted after (x); Rt (x): the retention time of the unknown compound (x); Rt (z): the retention time of the n-alkane eluted before (x); and Rt (y): the retention time of the n-alkane eluted after (x). Bianchi et al. (Citation2007) and Manral et al. (Citation2008).
2.7. Gas chromatography-olfactometry (GC–O)
The olfactometric analysis of the fermented milk volatiles was carried out with a Trace Ultra 1300 GC (Thermo Fischer, Scientific, Waltham, MA, USA) with a ZB-FFAP column (Agilent, UK), 50 m x 0.2 mm internal diameter x 0.33 μm film thickness (Crawford Scientific, UK) and an olfactory detector port (ODP 3), (Gerstel, Mulheim, Germany). The transfer line to the GC-O sniffing port was held at 250°C. The volume of the extracted milk samples analysed and the oven temperature program was similar to those employed for the GC-MS above. Sniffing was carried out by three trained panellists with over two years of training. These panellists exhibited normalized responses which produced strong agreement.
2.8. Statistical analysis
Analysis of variance (ANOVA) was carried out to determine the most fitted response surface models. Statistical analysis was carried out using the Minitab, Version 18, (Minitab Inc., USA). In addition, Pareto charts were established to analyse the outcomes of the volatiles profiles. The visualization of the differences between the overall VOCs in the fermented milk samples was carried out using the Gene Cluster 3.0/Java Treeview (Windows, Mac OSX, Linux, UNIX) software.
3. Results and discussion
3.1. Selecting for the best SPME fibre
Selecting the best coating fibre from the different fibres is an important step in the optimization process which usually relies on the chemical nature of the targeted analyte. shows the mean of the total peak areas obtained from the four different SPME fibres tested with respect to their capacity to extract the VOCs of the fermented milk. Results revealed significant differences (p < .05) in the fibres’ ability to extract volatiles. In the current study, PDMS/CAR was able to extract the highest amount of volatile compounds (i.e.163 compounds) as reflected in its higher mean peak area (19.80 × 107) (). This was followed by the PDMS/DVB (6.0 × 107) with 60 compounds and PDMS/DVB/CAR as well as PDMS extracted the least amount of volatile compounds (< 3.0 × 107). Therefore, we selected the PDMS/CAR which gave the highest mean peak area value for the assessment of the characteristic volatile profile of the fermented milk products.
3.2. Optimization of the HS-SPME
Studies have shown that the headspace analysis of VOCs by HS-SPME is greatly affected by the vapour pressure of volatile compounds in the vial (Lasekan et al., Citation2016; Pellati et al., Citation2005). In addition, the extraction temperature, equilibrium time and extraction time are the major factors influencing the vapour pressure and the equilibrium of the VOCs in the headspace (Pellati et al., Citation2005). Based on the results of previous studies, the extraction time and extraction temperature were therefore selected and optimized (). Thirteen experiments of the design were carried out at random and the responses are presented in . The results and the predicted regression coefficients of the fitted mathematical models with their corresponding R2 values, and lack of fit test are provided in . The significance of each term was determined using the F-ratio and the P value. In this study, the amount of VOCs extracted from the headspace of the fermented milk was significantly (p < .05) influenced by both the main and interactive effects of the extraction temperature and extraction time. The quadratic effect of the independent variables had no significant influence (p > .05) on the amount of VOCs extracted. This observation was further corroborated by the Pareto chart ()) which showed that increase in either factors (i.e. extraction temperature and extraction time) resulted in significant increases (p < .05) in the amount of VOCs extracted. Similar observations were reported for the extraction of VOCs from Chili (Junior et al., Citation2011) and Brazilian sugar cane spirits (Zacaroni et al., Citation2017). To provide a visual relationship between the independent factors and the amount of VOCs extracted a 3D response surface plot of the peak area of the VOCs against extraction time and extraction temperature is presented in . Results showed that increasing the extraction temperature resulted in an increase in the amount of VOCs. However, when the temperature was increased beyond 50°C, there was significant reduction (p < .05) in the amount of VOCs extracted. This observation is due to the fact the SPME is an exothermic reaction (Pellati et al., Citation2005). The extraction of VOCs decreases with increase in extraction temperature. In the light of these results the best response within the range studied was defined as an extraction time of 60 min and an extraction temperature of 50°C.
Table 3. Coefficients and tests of the significance of the variables obtained for the extraction of the volatile organic compounds from fermented milk.
Tabla 3. Coeficientes y pruebas de significancia de las variables obtenidas para la extracción de compuestos orgánicos volátiles de la leche fermentada
Figure 2. (a): Pareto chart for responses (total peak area versus different conditions tested (b): response surface plot for total peak are versus extraction time, and extraction temperature.
Figura 2. (a): Gráfico de Pareto de las respuestas (área de pico total versus diferentes condiciones probadas); (b): gráfico de superficie de respuesta para el pico total versus tiempo de extracción y temperatura de extracción
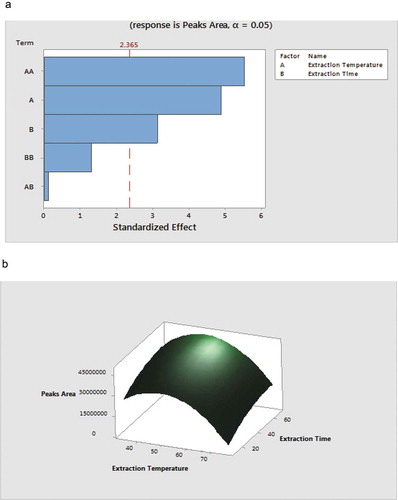
3.3. Volatile organic compounds analysis in the fermented milk
The formation of VOCs in milk and dairy products is complex and it includes glycolysis, lipolysis as well as proteolysis of milk components which is brought about by the enzymatic mechanisms of microflora within the dairy matrix (G. Smit et al., Citation2005). Suffice to say that the production of flavour compounds in fermented dairy products is strain dependent. In this study, both L. casei 431 and L. acidophilus La-5 have the ability to produce VOCs, such as ketones, aldehydes, alcohols, and acids. More than hundred compounds were tentatively identified in the volatile fractions of the fermented milk samples. Of this, eighty-three compounds were identified in the L. casei milk while eighty-four compounds were identified in the La-5 milk. The compounds included seven aldehydes, twenty-one ketones, fifteen acids, twelve esters, nineteen alcohols, thirteen aromatic hydrocarbons, 12 hydrocarbons and six furans (). As shown in , ketones and acids demonstrated to be major VOCs, while the other compounds were minor in the fermented milk samples. Although, the qualitative profile of both L. casei milk and La-5 milk were similar, the relative abundance of the compounds of different chemical families was different. The differences between the L. casei milk and La-5 milk were mainly due to the composition and abundance of their ketones, acids, and alcohols. For instance, in this study, 21 ketones were identified in the fermented milk samples (). Some of these important flavour VOCs were present at relatively high levels. The most prominent of these ketones were the creamy/buttery 2,3-butanedione (12.2–25.5%), fruity 2 heptanone (15.2–17.89%) and the herbal 2-nonanone (1.67–2.67%). These ketones are metabolised from citrate (Pan et al., Citation2014). Ketones have variously been reported as important flavour compounds in fermented milk (Chaves et al., Citation2011; Dan, Wang, Wu et al., Citation2017; Milesi et al., Citation2010). 2,3-Butanedione is a diketone which was the most abundant ketone in the L. casei milk with percentage area twice that of the La-5 milk. In addition, this compound can readily be converted into acetoin by the enzyme diacetyl reductase (Rattray et al., Citation2003). Surprisingly, low levels of acetoin were obtained for both La-5 and L. casei milks. In contrast, 2-heptanone and 2-nonanone contributed significantly to the total amount of ketones in both milk samples. These compounds have been reported as the major contributors to the creamy fresh flavour in fermented milk (Pionnier & Hugelshofer, Citation2006).
Table 4. Volatile compounds identified by HS-SPME-GC–MS/GC-O analysis in fermented milk.
Tabla 4. Compuestos volátiles identificados mediante el análisis HS-SPME-GC–MS/GC-O en leche fermentada
Figure 3. The relative contents of the different classes of volatile compounds (VOCs) detected in the fermented milk samples.
Figura 3. Contenidos relativos de las diferentes clases de compuestos volátiles (VOC) detectados en las muestras de leche fermentada
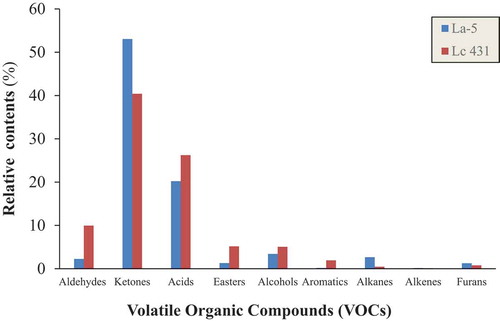
Similar to the ketones, the alcohols are important flavour compounds in dairy products (Pan et al., Citation2014). The fermented milk samples produced an array of alcohols such as; 3-methyl-1-butanol, 3-methyl-2-butenol, 1-hexanol, 2-furanmethanol, 1-octen-3-ol and 2-nonanol all of which occurred in appreciable amount. It is worthy to note that the La-5 milk had a higher total relative alcohol abundance compared to the L. casei milk. Most of these alcohols have been reported in dairy products and some of them are known to impart good flavour to dairy products. For example, 1-octen-3-ol which has a low threshold value (1.5 μg/L) is known to contribute good flavour to dairy products even at low concentrations (Friedrich & Acree, Citation1998).
On the other hand, fifteen acids were detected in the different fermented milk samples. Among these, the majority were acetic acid, butanoic acid, hexanoic acid and octanoic acid. In this study, the acetic acid percentage abundance ranged from 13.1 to 19.5%, with La-5 milk recording higher value of 19.5%. High levels of acetic acid have been reported to contribute to the ‘tart’ flavour of yogurt and ‘vinegary’ taste in fermented milk (Panagiotidis et al., Citation2001). Apart from acetic acid, hexanoic acid and octanoic acid which were in lower concentrations than acetic acid have been reported as contributing fatty/cheesy flavour to fermented milk (Pan et al., Citation2014).
Ethyl acetate was the most significant ester detected in the fermented milk samples. Other esters such as 3/2-methylbutanoate, 2-methylpropanoate, pentyl acetate, 2-ethylhexyl acetate occurred in low concentrations in the fermented milk samples. The contribution of esters to the flavour of dairy products is concentration dependent. At low concentration, esters contribute positively to the overall flavour balance, while at high concentrations, they produces fruity flavour defect (Liu et al., Citation2004).
Fewer numbers of aldehydes were detected in the fermented milk samples. The aldehydes were the malty 3-methylbutanal, hexanal, (z)-2-heptenal, (E)-2-octenal, 3,7-dimethyl-6-octenal, benzaldehyde, and 5-hydroxymethyl furfural. 3-Methylbutanal and hexanal were the predominant aldehydes detected. In numerous food products, aldehydes are key-flavour compounds. Sensorially, they are discerned as malty, almond-like. 3-Methylbutanal is among the most important volatiles in fermented dairy products and it is produced by synthesis action of micro-organisms via both the Ehrlich pathway and via amino-acid biosynthetic pathways of branched-chain amino acids valine, isoleucine and leucine (B. A. Smit et al., Citation2009).
In this study, there were many hydrocarbons and aromatic hydrocarbons in the fermented cow milk samples. These compounds were possibly related to the milk, feed given to the herd, the milk transport containers, materials used during production, and packaging materials (Yüksel & Bakırcı, Citation2015). For example, limonene can be transferred directly from the cows’ feeds (Viallon et al., Citation1999).
Finally, to better visualise the differences between the overall volatile compound profiles generated by the two strains of LAB, a heat map () of their volatilomic potentials was generated. The dark green blocks indicated compounds exhibiting higher peak areas while the red blocks were for compounds showing much lower peak areas. The heat map shows that L. casei 431 exhibited higher abundances for the following compounds; 2-heptanone, 2,3-butanedione, 2-pentanone, 2-butanone, 2-nonanone, butanoic acid, acetoin, 2-furanmethanol, 2-methylbutanoate etc. (Dark green color). On the other hand L. acidophilus La-5 exhibited higher abundances for acetic acid, hexanol, ethyl acetate, isobutanol, oactanoic acid, 3-methyl-1-butanol, 2-hexanone etc. (Dark green color). Overall, L. casei 431 exhibited higher abundances in critical chemical groups (i.e. ketones, esters, alcohols and aromatic hydrocarbons) as compare to L. acidophilus La-5.
Figure 4. Heatmap of the volatile organic compounds (VOCs) identified in fermented milk samples. The most abundant chemical groups are shown in green color while the least abundant group is shown in red color.
Figura 4. Mapa de calor de los compuestos orgánicos volátiles (VOC) identificados en muestras de leche fermentada. Los grupos químicos más abundantes aparecen en color verde, mientras que el grupo menos abundante aparece en color rojo
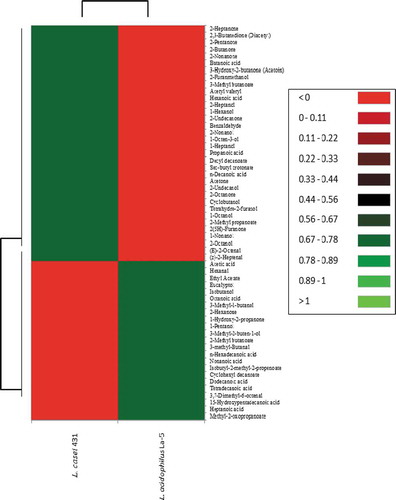
4. Conclusion
According to the results of this study, PDMS/CAR is the best fibre for the extraction of VOCs in fermented milk. In addition, analysis of the VOCs produced during the lactic acid fermentation of Lactobacillus casei 431 and Lactobacillus acidophilus La-5, led to the identification of a wide range of VOCs made up of 7 aldehydes, 21 ketones, 15 acids, 12 esters, 19 alcohols, 12 hydrocarbons, 13 aromatic compounds and 6 furans. Furthermore, the ketones represented a consistently higher percentage in the milk produced by the two strains of the LAB investigated. The ketones were followed by the acids. The aldehydes, alcohols and esters produced relatively lower percentage abundances in the milk samples. The ability of these LAB strains to produce distinct flavour compounds suggests their potential as starter cultures to enhance the development of dairy products. In this study, L. casei 431 was able to produce more typical flavour compounds such as 2,3-butanedione, 2-heptanone, acetoin and 2-nonanone compared to L acidophilus La-5. Overall, L. casei 431 exhibited higher abundances in the production of critical chemical groups (i.e. ketones, esters, alcohols and aromatic hydrocarbons) as compared to L. acidophilus La-5.
Acknowledgments
The authors are grateful for the extensive financial support received from University Putra Malaysia research scheme Grant (9478500). Also, the authors are grateful for LAB strains supplied by Chr. Hansen A/S Malaysia.
Disclosure statement
The authors declares no competing interest
Additional information
Funding
References
- Abesinghe, A. M. N. L., Islam, N., Vidanarachchi, J. K., Prakash, S., Silva, K. F. S. T., & Karim, M. A. (2019). Effects of ultrasound on the fermentation profile of fermented milk products incorporated with lactic acid bacteria. International Dairy Journal, 90(3), 1–14. https://doi.org/10.1016/j.idairyj.2018.10.006
- Aunsbjerg, S. D., Honoré, A. H., Marcussen, J., Ebrahimi, P., Vogensen, F. K., Benfeldt, C., Skov, T., & Knøchel, S. (2015). Contribution of volatiles to the antifungal effect of Lactobacillus paracasei in defined medium and yogurt. International Journal of Food Microbiology, 194(2), 46–53. https://doi.org/10.1016/j.ijfoodmicro.2014.11.007
- Beshkova, D. M., Simova, E. D., Frengova, G. I., Simov, Z. I., & Dimitrov, Z. P. (2003). Production of volatile aroma compounds by kefir starter cultures. International Dairy Journal, 13(7), 529–535. https://doi.org/10.1016/S0958-6946(03)00058-X
- Bianchi, F., Careri, M., Mangia, A., & Musci, M. (2007). Retention indices in the analysis of food aroma volatile compounds in temperature‐programmed gas chromatography: Database creation and evaluation of precision and robustness. Journal of Separation Science, 30(4), 563–572. https://doi.org/10.1002/()1615-9314
- Bozoudi, D., Kotzamanidis, C., Hatzikamari, M., Tzanetakis, N., Menexes, G., & Litopoulou-Tzanetaki, E. (2015). A comparison for acid production, proteolysis, autolysis and inhibitory properties of lactic acid bacteria from fresh and mature Feta PDO Greek cheese, made at three different mountainous areas. International Journal of Food Microbiology, 200(4), 87–96. https://doi.org/10.1016/j.ijfoodmicro.2015.02.008
- Chatterjee, S., Kumar, A., Basu, S., & Dutta, S. (2012). Application of response surface methodology for methylene blue dye removal from aqueous solution using low cost adsorbent. Chemical Engineering Journal, 181-182(1), 289–299. https://doi.org/10.1016/j.cej.2011.11.081
- Chaves, A. C. S. D., Ruas-Madiedo, P., Starrenburg, M., Hugenholtz, J., & Lerayer, A. L. S. (2011). Impart of engineered Streptococcus thermophiles strains overexpressing gly A gene on folic acid and acetaldehyde production in fermented milk. Brazilian Journal of Microbiology, 34(Suppl.1), 114–117. https://doi.org/10.1590/S1517-83822003000500039
- Condurso, C., Verzera, A., Romeo, V., Ziino, M., & Conte, F. (2008). Solid-phase microextraction and gas chromatography mass spectrometry analysis of dairy product volatiles for the determination of shelf-life. International Dairy Journal, 18(8), 819–825. https://doi.org/10.1016/j.idairyj.2007.12.005
- Cuffia, F., Bergamini, C. V., Hynes, É. R., Wolf, I. V., & Perotti, M. C. (2019). Evaluation of autochthonous cultures to improve the cheese flavor: A case study in hard cheese model. Food Science and Technology International, 26(2), 173–184. https://doi.org/10.1177/1082013219881512
- Cui, S., Zhao, N., Lu, W., Zhao, F., Zheng, S., Wang, W., & Chen, W. (2019). Effect of different Lactobacillus species on volatile and nonvolatile flavor compounds in juices fermentation. Food Science & Nutrition, 7(7), 2214–2223. https://doi.org/10.1002/fsn3.2019.7.issue-7
- Dan, T., Wang, D., Jin, R. L., Zhang, H. P., Zhou, T. T., & Sun, T. S. (2017). Characterization of volatile compounds in fermented milk using solid-phase microextraction methods coupled with gas chromatography-mass spectrometry. Journal of Dairy Science, 100(4), 2488–2500. https://doi.org/10.3168/jds.2016-11528
- Dan, T., Wang, D., Wu, S., Jin, R., Ren, W., & Sun, T. (2017). Profiles of volatile flavor compounds in milk fermented with different proportional combinations of Lactobacillus delbrueckii subsp. Bulgaricus and Streptococcus thermophilus. Molecules, 22(10), 1633. https://doi.org/10.3390/molecules22101633
- Engels, W. J. M., Dekker, R., De Jong, C., Neeter, R., & Visser, S. (1997). A comparative study of volatile compounds in the water-soluble fraction of various types of ripened cheese. International Dairy Journal, 7(4), 255–263. https://doi.org/10.1016/S0958-6946(97)00003-4
- Friedrich, J. E., & Acree, T. E. (1998). Gas chromatography olfactometry (GC/O) of dairy products. International Dairy Journal, 8(3), 235–241. https://doi.org/10.1016/S0958-6946(98)80002-2
- Irlinger, F., Helinck, S., & Jany, J. L. (2017). Secondary and Adjunct Cultures. In P. L. H. McSweeney, P. F. Fox, P. D. Cotter, & D. W. Everett (Eds.), Cheese: Chemistry, physics and microbiology (Vol. 1, 4th ed., pp. 273–300). Elsevier.
- Junior, S. B., De Melo, A. D. M. T., Zini, C. A., & Godoy, H. T. (2011). Optimization of the extraction conditions of the volatile compounds from chili peppers by headspace solid phase micro-extraction. Journal of Chromatography A, 1218(21), 3345–3350. https://doi.org/10.1016/j.chroma.2010.12.060
- Lasekan, O., Hosnas, R., Ng, S., Lin, M., Azeez, S., Teoh, L., Gholivand, S., & Shittu, R. (2016). Identification of aromatic compounds and their sensory characteristics in cassava flakes and “garri”(Manihot esculenta Crantz). CyTA-Journal of Food, 14(1), 154–161. https://doi.org/10.1080/19476337.2015.1074944
- Lasekan, O., & Hussein, F. K. (2018). Classification of different pineapple varieties grown in Malaysia based on volatile fingerprinting and sensory analysis. Chemistry Central Journal, 12(1), 140. https://doi.org/10.1186/s13065-018-0505-3
- Lasekan, O., Khatib, A., Juhari, H., Patiram, P., & Lasekan, S. (2013). Headspace solid-phase microextraction gas chromatography–mass spectrometry determination of volatile compounds in different varieties of African star apple fruit (Chrysophillum albidum). Food Chemistry, 141(3), 2089–2097. https://doi.org/10.1016/j.foodchem.2013.05.081
- Lin, J., Hua, B., Xu, Z., Li, S., & Ma, C. (2016). The impact of proteolytic pork hydrolysate on microbial, flavor and free amino acids compounds of yogurt. Korean Journal for Food Science of Animal Resources, 36(4), 558. https://doi.org/10.5851/kosfa.2016.36.4.558
- Liu, S. Q., Holland, R., & Crow, V. L. (2004). Esters and their biosynthesis in fermented dairy products: A review. International Dairy Journal, 14(11), 923–945. https://doi.org/10.1016/j.idairyj.2004.02.010
- Manral, L., Gupta, P. K., Ganesan, K., & Malhotra, R. C. (2008). Gas chromatographic retention indices of fentanyl and analogues. Journal of Chromatographic Science, 46(6), 551–555. https://doi.org/10.1093/chromsci/46.6.551
- Marilley, L., & Casey, M. G. (2004). Flavours of cheese products: Metabolic pathways, analytical tools and identification of producing strains. International Journal of Food Microbiology, 90(2), 139–159. https://doi.org/10.1016/S0168-1605(03)00304-0
- Milesi, M. M., Wolf, I. V., Bergamini, C. V., & Hynes, E. R. (2010). Two strains of nonstarter lactobacilli increased the production of flavor compounds in soft cheeses. Journal of Dairy Science, 93(11), 5020–5031. https://doi.org/10.3168/jds.2009-3043
- Østlie, H. M., Helland, M. H., & Narvhus, J. A. (2003). Growth and metabolism of selected strains of probiotic bacteria in milk. International Journal of Food Microbiology, 87(1–2), 17–27. https://doi.org/10.1016/S0168-1605(03)00044-8
- Pan, D. D., Wu, Z., Peng, T., Zeng, X. Q., & Li, H. (2014). Volatile organic compounds profile during milk fermentation by Lactobacillus pentosus and correlations between volatiles flavor and carbohydrate metabolism. Journal of Dairy Science, 97(2), 624–631. https://doi.org/10.3168/jds.2013-7131
- Panagiotidis, P., Tzia, C., Spanier, A. M., Shahidi, F., Parliament, T. H., & Mussinan, C. (2001). Effect of milk composition and heating on flavour and aroma of yogurt. In A. M. Spanier, F. Shahidi, T. H. Parliment, C. Mussinan, C. -T. Ho, & E. Tratras Contis (Eds.), Food flavours and chemistry: Advances of the new millennium (pp. 160–167). Royal Society Chemistry.
- Pellati, F., Benvenuti, S., Yoshizaki, F., Bertelli, D., & Rossi, M. C. (2005). Headspace solid-phase microextraction-gas chromatography–mass spectrometry analysis of the volatile compounds of Evodia species fruits. Journal of Chromatography A, 1087(1–2), 265–273. https://doi.org/10.1016/j.chroma.2005.01.060
- Pionnier, E., & Hugelshofer, D. (2006). Characterisation of key odorant compounds in creams from different origins with distinct flavours. In Developments in Food Science (Vol. 43, pp. 233–236). Elsevier. https://doi.org/10.1016/S0167-4501(06)80056-7
- Potts, D. M., & Peterson, D. G. (2019). Identification of small molecule flavor compounds that contribute to the somatosensory attributes of bovine milk products. Food Chemistry, 294(1), 27–34. https://doi.org/10.1016/j.foodchem.2019.05.010
- Rattray, F. P., Myling-Petersen, D., Larsen, D., & Nilsson, D. (2003). Plasmid-encoded diacetyl (acetoin) reductase in Leuconostoc pseudomesenteroides. Applied and Environmental Microbiology, 69(1), 304–311. https://doi.org/10.1128/AEM.69.1.304-311.2003
- Smid, E. J., & Kleerebezem, M. (2014). Production of aroma compounds in lactic fermentations. Annual Review of Food Science and Technology, 5(1), 313–326. https://doi.org/10.1146/annurev-food-030713-092339
- Smit, B. A., Engels, W. J., & Smit, G. (2009). Branched chain aldehydes: Production and breakdown pathways and relevance for flavour in foods. Applied Microbiology and Biotechnology, 81(6), 987–999. https://doi.org/10.1007/s00253-008-1758-x
- Smit, G., Smit, B. A., & Engels, W. J. (2005). Flavour formation by lactic acid bacteria and biochemical flavour profiling of cheese products. FEMS Microbiology Reviews, 29(3), 591–610. https://doi.org/10.1016/j.fmrre.2005.04.002
- Tunick, M., Iandola, S., & Van Hekken, D. (2013). Comparison of SPME methods for determining volatile compounds in milk, cheese, and whey powder. Foods, 2(4), 534–543. https://doi.org/10.3390/foods2040534
- Tuomala, T., & Kallio, H. (1996). Identification of free fatty acids and some other volatile flavour compounds from Swiss cheese using on-line supercritical fluid extraction-gas chromatography. Zeitschrift fuer Lebensmittel-Untersuchung und Forschung, 203(3), 236–240. https://doi.org/10.1007/BF01192870
- Valduga, E., Valerio, A., Treichel, H., Nascimento Filho, I., Fúrigo Júnior, A., & Di Luccio, M. (2010). Head Space Solid Phase Micro-Extraction (HS-SPME) of volatile organic compounds produced by Sporidiobolus salmonicolor (CBS 2636). Food Science and Technology, 30(4), 987–992. https://doi.org/10.1590/S0101-20612010000400023
- Viallon, C., Verdier-Metz, I. S. A. B. E. L. L. E., Denoyer, C., Pradel, P., Coulon, J. B., & Berdagué, J. L. (1999). Desorbed terpenes and sesquiterpenes from forages and cheeses. Journal of Dairy Research, 66(2), 319–326. https://doi.org/10.1017/S0022029999003520
- Xanthopoulos, V., Picque, D., Bassit, N., Boquien, C. Y., & Corrieu, G. (1994). Methods for the determination of aroma compounds in dairy products: A comparative study. Journal of Dairy Research, 61(2), 289–297. https://doi.org/10.1017/S0022029900028302
- Yüksel, A. K., & Bakırcı, İ. (2015). An investigation of the volatile compound profiles of probiotic yogurts produced using different inulin and demineralised whey powder combinations. Food Science and Biotechnology, 24(3), 807–816. https://doi.org/10.1007/s10068-015-0105-0
- Yvon, M., & Rijnen, L. (2001). Cheese flavour formation by amino acid catabolism. International Dairy Journal, 11(4–7), 185–201. https://doi.org/10.1016/S0958-6946(01)00049-8
- Zacaroni, L. M., De Sales, P. F., Cardoso, M. D. G., Santiago, W. D., & Nelson, D. L. (2017). Response surface optimization of SPME extraction conditions for the analysis of volatile compounds in Brazilian sugar cane spirits by HS‐SPME‐GC–MS. Journal of the Institute of Brewing, 123(2), 226–231. https://doi.org/10.1002/jib.v123.2