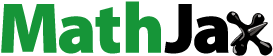
ABSTRACT
Soluble dietary fiber was extracted from spent Morchella importuna by optimizing the extraction conditions using the response surface method. Response surface analysis results indicated that the extraction yield of MI-SDF reached up to 15.38 ± 0.16% under the optimized conditions with optimal lye concentration of 2.9%, extraction temperature of 71°C and extraction time of 2 h. The results of scanning electron microscope (SEM), Fourier transform infrared spectroscopy (FT-IR), and X-ray diffraction analyses suggested that M. importuna-derived soluble dietary fiber (MI-SDF) exhibited the typical porous fiber structures, polysaccharide functional groups, and cellulose crystal structure. And MI-SDF was applicable to food processing at <250°C. During in vitro fecal fermentation, MI-SDF significantly increased the abundance and diversity of beneficial bacteria and decreased those of harmful bacteria. The levels of total and individual short-chain fatty acids were significantly increased. This study will provide valuable information for further investigation of MI-SDF.
GRAPHICAL ABSTRACT
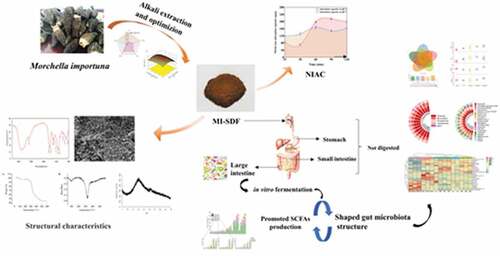
RESUMEN
En este estudio se extrajo fibra dietética soluble de Morchella importuna empleando el método de superficie de respuesta y optimizando las condiciones de extracción. Los resultados del análisis de superficie de respuesta indicaron que, en las condiciones optimizadas – concentración óptima de lejía de 2.9%, temperatura de extracción de 71°C y tiempo de extracción de 2 horas – el rendimiento de extracción de MI-SDF fue de 15.38 ± 0.16%. Además, los resultados de los análisis con microscopio electrónico de barrido (SEM), espectroscopia infrarroja por transformada de Fourier (FT-IR) y difracción de rayos X mostraron que la fibra dietética soluble derivada de M. importuna (MI-SDF) presenta las típicas estructuras de fibra porosa, grupos funcionales de polisacáridos y estructura cristalina de celulosa. Asimismo, la MI-SDF puede ser utilizada en el procesamiento de alimentos a <250°C Durante la fermentación fecal in vitro, la MI-SDF aumentó significativamente la abundancia y la diversidad de las bacterias beneficiosas, al tiempo que disminuyó las de las bacterias nocivas. Por otra parte, se constató un aumento significativo de los niveles de ácidos grasos de cadena corta totales e individuales. Este estudio proporciona información valiosa para una mayor investigación de la MI-SDF.
1. Introduction
Dietary fiber (DF) is a polysaccharide that cannot be decomposed by digestive tract enzymes. It is known as the 7th vital nutrient in organism (He et al., Citation2022) and has a wide range of pharmacological effects such as weight loss, intestine and stomach function regulation, and cancer prevention and control (He et al., Citation2022; McRae, Citation2018; Neyrinck et al., Citation2020). Recently, its influence on the host’s health during digestion and fermentation has attracted increasing attention (C. Chen et al., Citation2016; He et al., Citation2022; H. Y. Wang et al., Citation2018). Short-chain fatty acids (particularly, acetic, propionic, and butyric acids) produced from fermentation of dietary fiber in the large intestine have been reported to have positive effects on microbial composition and could reduce the risk of colon-related diseases and metabolic syndromes such as obesity, diabetes, cancer, intestinal diseases, and inflammation (Makki et al., Citation2018; Neyrinck et al., Citation2020; Wang et al., Citation2019). Therefore, it is urgent to develop natural and healthy dietary fiber sources regulating the function of the intestines and stomach without side effects. Some dietary fiber sources such as oat bran (Kedia et al., Citation2009), cereal flour (Comino et al., Citation2018), Indian edible seaweeds (Ajanth Praveen et al., Citation2019), Panax ginseng (Hua et al., Citation2019), Saccharina japonica (Deng et al., Citation2021), potato residue (Ma et al., Citation2021), fruit peel (Tejada-Ortigoza et al., Citation2022) and soy hulls (L. Li et al., Citation2022) have been reported to regulate intestinal microorganism. The edible fungi have advantages over the above-mentioned dietary fiber sources due to their high nutritional value and unique characteristics, and they are more suitable for consumers at all ages and special groups such as children and diabetes patients (M. Y. Li et al., Citation2021; Zhao et al., Citation2018). The dietary fibers extracted from edible fungi are healthy, green, and safe with high biochemistry and pharmacology values (Caz et al., Citation2015; Liang et al., Citation2021; Ng et al., Citation2017; L. W. Wang et al., Citation2021). It has been reported that dietary fiber extracted from edible fungi such as Cookeina speciosa (Cantu-Jungles et al., Citation2018), Lentinus edodes (Xue et al., Citation2020), Pleurotus ostreatus (Laforteza et al., Citation2020) and Flammulina velutipes (W. L. Wang et al., Citation2021) can regulate intestinal microorganisms. The intake of dietary fiber derived from F. velutipes mycorrhizae by obese mice could attenuate lipid metabolism disorders through the gut microbiota (W. L. Wang et al., Citation2021). Soluble dietary fiber extracted from L. edodes by-products during in vitro fermentation could change the composition of the intestinal microbial community to maintain intestinal health (Xue et al., Citation2020).
True morels (Morchella spp., Pezizales, and Ascomycota) are a world-recognized rare edible, and medicinal macrofungi, and they are abundant in polysaccharides, dietary fibers, proteins, amino acids, and so on (Tietel & Masaphy, Citation2018). In recent years, the research on Morchella polysaccharides is mainly focused on antioxidation, immunostimulatory, anti-inflammatory, hepatoprotective, anti-fatigue, and anti-tumor (Badshah et al., Citation2021; S. H. Li et al., Citation2017; Wen et al., Citation2021; Yang et al., Citation2019; N. N. Zhang et al., Citation2022; Z. Zhang et al., Citation2022). It is reported that a new heteropolysaccharide (MIPW50–1) isolated from Morchella importuna could stimulate macrophage function, rise phagocytosis of RAW264.7 cells (Wen et al., Citation2019). Two novel glucans (MIPB50-W and MIPB50-S-1) isolated from M. importuna have been shown to have immunostimulatory activity (Wen et al., Citation2021). However, there are few studies on Morchella polysaccharides effects on gut microbiota.
M.importuna, as one of the few species of Morchella, has been successfully domesticated by mushroom farmers in China in recent years, and its cultivation area accounts for over 95% of the artificial cultivation area (Du et al., Citation2012; Wen et al., Citation2021). Morchella dried products are divided into four grades: Grade 1, Grade 2, Export grade and non-graded dried products (Du et al., Citation2014) [Supplemental content 1]. The fruiting bodies of M. importuna contain rich dietary fiber (Xu et al., Citation2016). However, in the processing of M. importuna, the non-graded dried products were mass-produced and generally removed, resulting in great waste. The additional value of M. importuna needs to be further explored and effectively applied. In addition, the in vitro fermentation characteristics of dietary fiber extracted from M. importuna and its potential roles in intestinal microbiota regulation remain to be further investigated. L-cysteine hydrochloride, bile salt and hemin were purchased from Solarbio (Beijing, China).
In this study, the non-graded dried M. importuna products were used as raw materials. The structural characteristics of M. importuna-derived soluble dietary fiber (MI-SDF) was investigated. Its extraction process was optimized, and its in vitro fecal fermentation-induced the changes in short-chain fatty acid yield and human intestinal microbiota were examined. This study will shed light on the extraction of dietary fiber from M. importuna as a functional food ingredient to promote human gut health.
2. Materials and methods
2.1. Materials and chemicals
M. importuna was purchased from Yunnan Qidao Agricultural Technology Development Co., Ltd. The reagents (hydrochloric acid, sodium hydroxide, absolute ethanol, sodium nitrite, p-Aminobenzene Sulfonic Acid, naphthalene ethylenediamine hydrochloride, glacial acetic acid, hydrochloric acid, and concentrated sulfuric acid) were obtained from Tianjin Zhiyuan Chemical Reagent Co., Ltd. (China), and they were analytically pure. L-cysteine hydrochloride, bile salt and hemin were purchased from Solarbio (Beijing, China).
2.2. Soluble dietary fiber (SDF) extraction from M. importuna
2.2.1. Preliminary treatment of M. importuna
In this study, the non-graded dried M. importuna products were used as raw materials [Supplemental content 1]. At first, the raw materials were cleaned dried in an oven to constant weight, ground, and sieved (80 mesh) to obtain M. importuna powders, and then the powders were stored in a self-sealing bag prior to extraction.
2.2.2. Extraction of soluble dietary fiber
SDF was extracted from M. importuna by alkaline hydrolysis extraction method with minor modifications (Karra et al., Citation2020). The 5 g M. importuna powder was mixed with NaOH solution at the solid–liquid ratio of 1:15, followed by dietary fiber extraction in water bath. The extraction temperature and time were set according to the requirements. Afterwards, extraction solution was centrifuged, and the supernatant was collected. Then, 10% HCl was added into supernatant, and the pH of the mixture was adjusted to 3.8. After protein and impurities were removed through centrifugation, the supernatant was collected and measured subsequently, the supernatant was mixed with 4-fold volume of absolute ethyl alcohol solution, and mixture solution was stood at room temperature for 24 hours. The precipitate from mixture solution was rinsed repeatedly with 100% ethanol until it was neutral, centrifuged at 6000 r/min for 10 min, and dried overnight in an oven (40°C). Finally, the dried samples were ground and sieved (80 microns) to obtain M. importuna-derived soluble dietary fiber. The final sample was named MI-SDF.
2.3. Experimental design
Based on the single-factor analysis, three parameters lye concentration, extraction temperature, and extraction time were determined as three key independent variables and respectively defined as X1, X2, and X3, for subsequent response surface analysis. As shown in , 17 experiments were designed based on these three independent variables and their three levels by using a Box–Behnken Design (BBD) in design-expert 10.0 software. The extraction yield was calculated as the final MI-SDF weight divided by the initial M. importuna powder weight. The extraction yield of MI-SDF was defined as the response value which was determined for three times and listed in . The nonlinear quadratic model equation of response surface was as follows:
Table 1. Experimental design and results of the Box–Behnken design.
Tabla 1. Diseño experimental y resultados del diseño Box–Behnken.
where Y indicated the predicted response value; Xi and Xj were the independent variables; β0 was a constant; βi, βii, and βij were linear, squared and interaction terms, respectively.
2.4. Scanning electron microscopy (SEM)
Scanning electron microscope (SEM) was used to examine the microstructure and morphology of M. importuna powder prepared in 2.2.1 and MI-SDF samples. Before observation, samples were treated as follows. A small amount of each sample was taken and stuck onto the conductive solid glue. The excess sample was carefully blown off with an ear washing ball, and then the sample on glue was sprayed with gold powders immediately and observed by a scanning electron microscope (Nano SEM-450, FEI, Czech) at 1500× and 4000× magnification with the accelerating voltage set as 5 kV (Kurek et al., Citation2018).
2.5. Fourier transform-infrared spectrometry (FT-IR)
The MI-SDF samples were evenly mixed with potassium bromide powder at a ratio of 1:200, and then the mixture was ground into a fine powder. Using the potassium bromide pressing method (K. L. Wang et al., Citation2021), a small amount of the sample was loaded into the grinding tool and pressed into a thin slice in the pressing machine. The thickness, transparency, and uniformity of the slice met the specified requirements. Finally, the Fourier transform infrared spectrometer (Nexus 5DXC, FT-IR, Nicolet) was used to scan sample slices, and the absorption spectra were recorded at 500 cm−1 to 4000 cm−1 wavelengths. Potassium bromide was used as a blank control.
2.6. X-Ray diffraction (XRD)
The samples were placed into a dedicated sample slot, compacted with a glass sheet, and transferred onto a diffractometer (D8 ADVANCE A25, Bruker, Germany) to determine their crystallinity. The diffraction intensities were set between 3° and 70° (2θ angle range). The step length was set as 0.02, and the scanning speed was set as 1.5 seconds per step. The operating voltage and current were 40 kV and 40 mA, respectively (Karaman et al., Citation2017).
2.7. Thermogravimetric analysis (TG)
The thermogravimetric analysis (TGA) and differential thermal gravity analysis (DTG) was performed by previously reported method with minor modifications (Y. X. Li et al., Citation2022). The MI-SDF samples were put into a small crucible, and the weight of the samples was recorded. The small crucible containing samples was put into a thermogravimetric analyzer (TGA/DSC 3+, Mettler Toledo, Switzerland) and heated from 30°C to 600°C at a heating rate of 10 °C/min and a nitrogen flow rate of 20 mL/min.
2.8. Nitrite ion adsorption capacity (NIAC)
NIAC was measured according to the previously reported method with slight modifications (K. L. Wang et al., Citation2021). In brief, 10 MI-SDF samples (1 g) were mixed with 100 mL of NaNO2 solution (5 μg/mL). Ten sample solutions were divided into two groups with five in each group. One group was adjusted to pH 2.0 with 10% HCl, and the other group to pH 7.0 with NaOH to simulate the stomach and intestine environment, respectively. Afterwards, the one group of mixture solution reacted at 37°C for 15, 30, 60, 90, and 120 min, and centrifuged at 4000 rpm for 20 min to determine the NaNO2 level in the supernatant. Moreover, the above experiments were repeated on 10 solutions without additional dietary fiber which was used as a blank control. The NIAC was measured according to Equation (2).
where C1 indicated the concentration of the nitrite ion solution before adsorption; C2 was the concentration of nitrite ion solution after adsorption; M was the relative molecular weight of nitrite ion; m was the weight of dietary fiber; and V was the total volume of the treated sample.
2.9. In vitro fermentation experiments
The simulated human large intestinal fecal fermentation was performed by previously described method with slight modifications (Huang et al., Citation2019). The fermentation medium (1.0 L) was composed of 2.0 g yeast extract, 2.0 g peptone, 0.1 g NaCl, 0.04 g K2HPO4, 0.01 g MgSO4·7 H2O, 0.01 g CaCl2, 0.02 g hemin, 0.5 g L-cysteine hydrochloride, 0.5 g bile salt, 2.0 mL Tween-80, 1.0 mL 1% resazurin, and 10 μg vitamin K1. The medium was neutralized to pH 7.0 with 0.1 mol/L HCl, sterilized at 121°C for 20 min. The fresh human fecal samples were collected from four healthy volunteers with a regular diet (two men and two women, 22–30 years old without taking antibiotics or suffering from digestive diseases for at least three months). These samples were transferred into sterilized phosphate buffered saline (PBS) solution to prepare 30% (w/v) fecal bacterium suspension. The obtained suspension was homogenized and filtered through sterile gauze to obtain the final human fecal inoculum. Then, 1.0 mL of the final human fecal inoculum was added into sealed penicillin bottles, and then mixed with 9.0 mL of basal medium added with 6 mg/mL MI-SDF (L group, low), 10 mg/mL MI-SDF (M group, meduim), and 14 mg/mL MI-SDF (H group, high), and 10 mg/mL inulin (INL group, positive control), respectively. The basal medium without a carbon source was used as the blank control (BLK group, negative control). At 37°C five treatment groups were cultured in an AnaeroPack system (Mitsubishi Gas Chemical Co., Tokyo, Japan). The fermented samples were collected at 0, 6, 12, 24, and 48 h post fermentation for further study. The experiment was conducted in triplicates.
2.10. MI-SDF and its ferment product component analysis
2.10.1. Gut microbiota analysis
The EZNA DNA Kit (Omega Bio-tek, Norcross, GA, USA) was used to extract the genomic DNA from five groups of samples. The 16S ribosomal RNA (16S rRNA) gene in the V4 variable region was sequenced by Ouyi Biotechnology Co., Ltd. (Shanghai, China). Five treatment groups including the BLK group, the INL group, the MI-SDF L group, MI-SDF M group, and MI-SDF H groups were subjected to in vitro 48-h fermentation. Finally, different species were screened based on sequence reads and operational taxonomic units (OTUs) at a minimum confidence score of 0.8.
2.10.2. Short-chain fatty acid determination
Short-chain fatty acids were determined by a gas chromatography system (GC, Thermo Scientific, Germany) according to previously reported method with slight modifications (M. J. Wang et al., Citation2019). In short, an HP-5 MS capillary column was used, and the temperatures of the injector and detector were both maintained at 230°C The initial column temperature was held at 100°C for 1 min. Then column temperature was raised to 180°C at a rate of 5 °C/min and maintained for 4 min.
2.11. Statistical analysis
OriginPro 2021 software (OriginLab Corporation, North-ampton, Mass., USA) and R package were used for plotting. The data were presented as mean ± standard deviations (SD). One-way analysis of variance (ANOVA) was performed using SPSS 20.0 software (IBM Inc, Chicago, IL, USA). p < .05 was regarded as statistically significant.
3. Results and discussion
3.1. Single-factor experiments
It has reported that lye concentration, extraction temperature, and extraction time are the most important factors influencing the extraction yield of SDF (Jia et al., Citation2020). Based on this, the present work explored the optimization of these three conditions during SDF extraction from M. importuna through single-factor experiments.
As shown in , when the extraction temperature and time were 70°C and 2 h respectively, the extraction yield of SDF was gradually increased with lye concentration increasing from 1.5% to 3%, then reached a maximum of 10.95 ± 0.21%. The major reason for this phenomenon might be that the increased osmotic pressure enhanced the diffusivity of the solvent into the cell (Ding et al., Citation2020). When the lye concentration exceeded 3%, the extraction yield began to decrease, which might be due to the denaturation and precipitation of proteins in SDF and the rupture of hydrogen bonds between cellulose and hemicellulose. Therefore, 3% lye concentration was determined as an optimal extraction condition in this work.
Figure 1. Effects of lye concentration (a), the extraction temperature (b) and extraction time (c) on the extraction yield of soluble dietary fiber from Morchella importuna. Data are expressed as the mean ± standard deviation (n = 3).
Figura 1. Efectos de la concentración de lejía (a); la temperatura de extracción (b); y el tiempo de extracción (c) en el rendimiento de extracción de la fibra dietética soluble de Morchella importuna. Los datos se presentan como la media ± desviación estándar (n = 3).
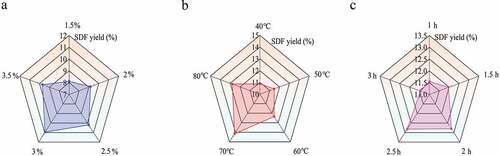
Extraction temperature is of great importance for the extraction yield of SDF since it affects the heat generation in the solvent and the solubility of SDF. demonstrated that the extraction yield of SDF was considerably increased with the increasing extraction temperature, reached its peak value (14.5 ± 0.24%) at 70°C and then fell back slightly when extraction temperature was above 70°C This SDF yield increase tendency might be attributed to the fact that higher temperature could accelerate the collision between molecules, thus enhancing the diffusivity of solvent into the cell. The decrease in case of extraction temperature >70°C might be because the structure of pectin in SDF was susceptible to rupture at high temperature (K. J. Wang et al., Citation2021). Considering both yield and energy cost, 70°C was determined as the optimal extraction temperature.
As shown in , when extraction time extended from 1 h to 2.5 h, the extraction yield showed a trend of slow increase, reached the peak value (12.80 ± 0.20%) at 2 h and then decreased. The possible reason for this lay in that the decrease in the reactant concentration inhibited alkaline hydrolysis. In addition, hydrogen bond broke with the extraction time extending, thus resulting in the hydrolysis of SDF into small molecules which could not precipitate in ethanol solution, finally decreasing the yield of SDF (Behrouzian et al., Citation2016). Considering the experiment cost and extraction yield, 2 h was determined as the optimal extraction time.
3.2. RSM optimization
3.2.1. Model fitting and statistical analysis
Based on the above single-factor experiments, lye concentrations of 2.5% ~ 3.5%, extraction temperature of 60°C 80°C and extraction time of 1.5 h 2.5 h were employed to further optimize the extraction yield of MI-SDF via Box–Behnken Design (BBD) experiments. The 17 runs of BBD experiments were performed, and the results are shown in . The extraction yield of MI-SDF was calculated according to the following quadratic polynomial function obtained from multiple regression analysis:
where Y was the extraction yield of MI-SDF (%), X1, X2, and X3 were lye concentration, extraction temperature, and extraction time, respectively.
The obtained results were analyzed by ANOVA showing that the selected quadratic models accurately predicted the extraction yield of MI-SDF (). The correlation coefficient of the model (R2 = 0.9265 > 0.9) showed that the fitting degree of the model was satisfactory. Moreover, the F-value and the lack-of-fit of the model were within the acceptable range, indicating that the model was sufficiently accurate for predicting the extraction yield of MI-SDF. As shown in , the two linear coefficients X1 and X3 and the quadratic coefficients X12 and X32 all reached a significant level of 1%. The regression analysis results indicated that extraction time exhibited the greatest effect on the extraction yield of MI-SDF (%), followed by lye concentration and extraction temperature.
Table 2. Analysis of variance for the results of Box–Behnken design.
Tabla 2. Análisis de la varianza para los resultados del diseño Box–Behnken.
3.2.2. Response surface analysis
The effects of three dependent variables on the extraction yield of MI-SDF and their interaction were visualized through the 3D response surface plots (Pourfarzad et al., Citation2013). The steepness of the 3D response surface plots reflected the degree to which the interaction of the two factors affected the extraction yield of MI-SDF. shows that the pairwise interactive response surfaces among the three factors were relatively gentle three-dimensional surfaces, which was consistent with the results that the interactions among these variables were insignificant (p > .05, ). As shown in , the response surface steepness of MI-SDF extraction yield with lye concentration was larger than that with extraction temperature, suggesting that lye concentration exhibited a greater effect on the extraction yield of MI-SDF (%) than extraction temperature. Similarly, shows that extraction time displayed a more significant effect on the extraction yield of MI-SDF (%) than lye concentration. The effect order of three factors on the extraction yield was as follows: extraction time > lye concentration > extraction temperature.
Figure 2. Response surface plots (a–c) showing the effects of different variables (lye concentration, %; extraction temperature, °C and extraction time, h) on the extraction yield of soluble dietary fiber from Morchella importuna.
Figura 2. Diagramas de superficie de respuesta (a-c) que muestran los efectos de diferentes variables (concentración de lejía, %; temperatura de extracción, °C; y tiempo de extracción, h) sobre el rendimiento de extracción de la fibra dietética soluble de Morchella importuna.
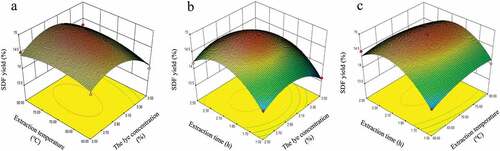
According to the results of response surface analysis, the optimal extraction conditions of MI-SDF were as follows: a lye concentration of 2.9%, an extraction temperature of 71°C and an extraction time of 2 h. Under these optimal conditions, the predicted extraction yield of MI-SDF was 14.90%. To verify the results predicted by the model, three experiments were performed under the optimized extraction conditions. The experiment results showed that actual extraction yield of MI-SDF (15.38 ± 0.16%) was within the acceptable margin of error, indicating that the model was applicable for predicting the extraction yield of MI-SDF.
3.3. Structural characteristics of MI-SDF
3.3.1. Scanning electron microscopy analysis
The scanning electron microscopy images (with 1500 × and 4000 × magnification) of prepared M. importuna powder and MI-SDF samples are shown in . The surface morphology of the M. importuna powder and MI-SDF samples were observed to be significantly different ( and 3B, 1500 × magnification). As shown in , the microstructure of the M. importuna powder presented an uneven, irregular, and coarse flake-like structure. After alkaline hydrolysis, the surface of the sample changed significantly. As shown in , the surface of MI-SDF powder became rough, loose, and porous, exhibiting a honeycomb shape, which was like a three-dimensional cavity left after water drainage.
Figure 3. SEM images of Morchella importuna powder (a, ×1500; c, ×4000); M. importuna-derived soluble dietary fiber (b, ×1500; d, ×4000).
Figura 3. Imágenes SEM del polvo de Morchella importuna (a, ×1500; c, ×4000); fibra dietética soluble derivada de M. importuna (b, ×1500; d, ×4000).
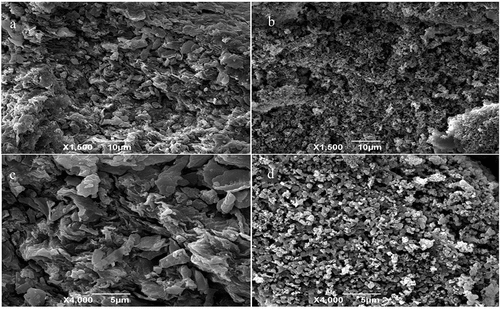
At 4000 × magnification, the microstructure of M. importuna powder presented a clear wrinkle-like structure on the surface (). The irregular flake structure with extremely smooth surface accumulated, which increased the contact area between M. importuna powder and alkali liquor, thus raising the reaction rate. Alkaline hydrolysis destroyed the originally dense surface flake structure on M. importuna power surface, resulting in the formation of a clear pore-like structure on MI-SDF surface (). These pores increased MI-SDF’s specific surface area, which was conducive to the entry and retention of water molecules. Alkali hydrolysis has been reported to destroy the bonds between the fiber reticular structures, making the structure of MI-SDF more rough, which contributed to the improvement of SDF functions such as the adsorption capacity of nitrite ions (Ding et al., Citation2020). Therefore, after the extraction through alkaline hydrolysis, the microstructure of MI-SDF changed. The new loose and porous structure endowed MI-SDF with better physical and chemical properties.
3.3.2. FT-IR analysis
FT-IR analysis was carried out to reveal the spectroscopic features of MI-SDF (). The broad peak between 3500 cm−1 and 3100 cm−1 was assigned to the stretching vibration of O-H, which resulted from intramolecular and intermolecular hydrogen bond reaction, indicating that MI-SDF might have some hydroxyl groups. The absorption peak near 2933.19 cm−1 was assigned to the C-H stretching vibration of the methyl group and methylene group on sugar molecules, suggesting that MI-SDF might have the typical structure of cellulose polysaccharides. The absorption peaks at 1664.53 cm−1 and 1631.81 cm−1 resulted from the asymmetric stretching of cellulose C=O, indicating that MI-SDF might contain uronic acid. The above results were in line with the previous report on the structure of SDF extracted from defatted coconut flour (X. J. Du et al., Citation2021). The peaks at 1381.47 cm−1 and 1351.39 cm−1 might result from the variable angle vibration of C-H, and the peaks in the 1200 cm−1 1420 cm−1 region were characteristic absorption peaks of saccharides. The broad absorption peak composed of several small peaks near 1080.53 cm−1 was caused by the C-O contraction vibration of cellulose and hemicellulose (Kurek et al., Citation2018). Taken together, infrared spectrum analysis indicated that the alkali-extracted MI-SDF had the characteristic absorption peak of polysaccharides, thus the main components of MI-SDF might be polysaccharides.
Figure 4. (a) Fourier transform infrared spectroscopy (FT-IR) spectra, (b) X-ray diffraction (XRD) pattern, (c) Thermogravimetric analysis (TGA) curves, (d) Differential thermal gravity analysis (DTG) curves of the Morchella importuna-derived soluble dietary fiber (MI-SDF).
Figura 4. (a) Espectroscopia infrarroja por transformada de Fourier (FT-IR); (b) Patrón de difracción de rayos X (XRD); (c) Curvas de análisis termogravimétrico (TGA); (d) Curvas de análisis de gravedad térmica diferencial (DTG) de la fibra dietética soluble derivada de Morchella importuna (MI-SDF).
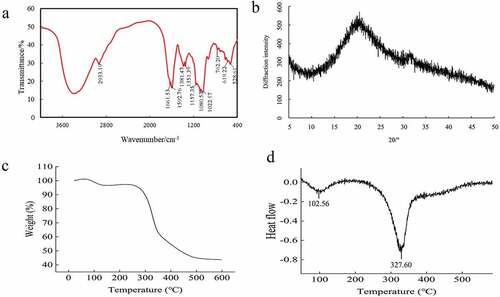
3.3.3. X-ray diffraction analysis
The disordered amorphous region and the ordered crystalline region together constitute the crystal region of cellulose (Karaman et al., Citation2017). As shown in , a crystal characteristic diffraction peak of MI-SDF was observed at 2θ = 21.05° with the diffraction intensity of 529.76 cps. In addition, a weak diffraction peak was found at 2θ = 32.33° with the diffraction intensity of 338.15 cps. These two peaks belonged to the characteristic peaks of cellulose type I and exhibited the same crystal structure with prosomillet bran-derived soluble dietary fibers (Y. X. Li et al., Citation2022). Taken together, MI-SDF exhibited wide diffraction peaks, indicating its low crystallinity, and its diffraction peak belonged to the typical high-molecular polymer diffraction peak (Jiang et al., Citation2020). These results suggested that MI-SDF might be a mixed polysaccharide composed of multiple polysaccharides.
3.3.4. Thermal properties
The thermal stability of the MI-SDF (with initial weight of 3.4038 mg) was analyzed by TGA. The TGA curve indicated that MI-SDF exhibited multi-step thermal degradation (). The first weight loss of sample was observed within the temperature range of 30°C – 250°C in which the weight of MI-SDF decreased most slowly, which might mainly be due to the evaporation of free water and crystal water from the sample (K. L. Wang et al., Citation2021). Approximately at 102.56°C MI-SDF exhibited the maximum weight loss rate. The sample’s second weight loss was observed under the temperature of 250°C–400°C with most rapid pyrolysis rate, the weight of MI-SDF decreased from 96.66% to 54.28%. At about 327.60°C the pyrolysis rate of MI-SDF reached the maximum, and the degradation of MI-SDF at this temperature might mainly be related to the loss of hydroxyl groups and a series of oxidation reactions after dehydration (Munoz et al., Citation2021). The last weight loss occurred under the temperature of 400°C–600°C with a slow sample weight loss, which might be attributed to the completion of high-temperature pyrolysis of MI-SDF samples (X. J. Du et al., Citation2021). At the last weight loss stage, the MI-SDF weight reduced from 54.28% to 43.66% with the final weight being 43.66% of initial weight. Our results indicated that MI-SDF had a superior thermal stability, and that it was stable below 250°C. Therefore, MI-SDF could be applied in heat treatments such as baking, frying, and high-temperature sterilization in food processing.
3.4. Nitrite ion adsorption capacity (NIAC)
Under the gastric acid environment, nitrite can form nitrosamines which could induce cancer and cause fetal malformations (Lundberg et al., Citation2009). shows the adsorption capacity of MI-SDF on nitrite ions at pH 2.0 (simulated gastric environment) and pH 7.0 (simulated intestinal environment) at different time points. At pH 2.0, the NIAC of MI-SDF was slightly decreased at 30 min and then increased rapidly. At 90 min, the NIAC of MI-SDF reached maximum (215.10 ± 0.31 μg/g), which was similar to that of grapefruit peel-derived SDF (219.43 ± 0.33 μg/g) (Gan et al., Citation2020) and higher than that of kiwifruit-derived SDF (138.8 ± 0.39 μg/g) (K. L. Wang et al., Citation2021), and then fell back slightly. At pH 7.0, the NIAC of MI-SDF was increased with time extending, reaching its the peak value (167.7 μg/g) at 60 min, and then became stable. MI-SDF exhibited a lower NIAC at pH 2.0 than at pH 7.0 when the reaction time was within 30 min, but it displayed a higher NIAC at pH 2.0 than at pH 7.0 when the reaction time was longer than 1 hour. It has been reported that the strongly electrophilic substance N2O3 is formed by NO2- and H+ under acidic conditions, and that N2O3 can bind the negatively charged oxygen atom of the phenolic acid group (Honikel, Citation2008). However, phenolic acid group of fiber cannot adsorb nitro due to neutralization of OH- when phenolic acid group of fiber was in a neutral or alkaline environment. Therefore, the NIAC of MI-SDF was higher under acidic conditions when the time was longer than 1 hour. The above results suggested that MI-SDF had a good adsorption capacity on nitrite ions under the condition of simulated gastric (pH 2.0) and intestinal environment (pH 7.0).
Figure 5. Nitrite ions adsorption capacity of Morchella importuna-derived soluble dietary fiber (MI-SDF). Data are expressed as the mean ± standard deviation (n = 3).
Figura 5. Capacidad de adsorción de iones nitrito de la fibra dietética soluble derivada de Morchella importuna (MI-SDF). Los datos se presentan como la media ± desviación estándar (n = 3).
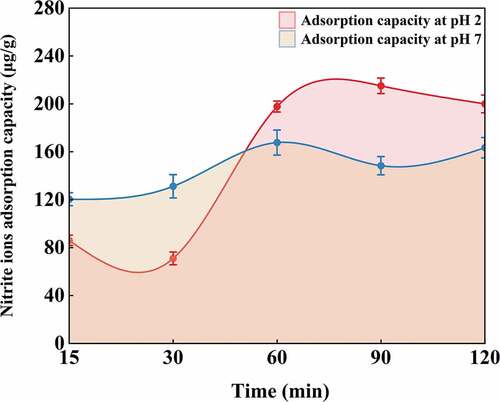
3.5. Effects of in vitro fermentation of MI-SDF
3.5.1. Effects of MI-SDF on gut microbiota
3.5.1.1. Changes in gut microbiota abundance and diversity
To determine the effect of MI-SDF on the gut microbial diversity, we further analyzed the sequences of 16S rRNA genes in each sample group. shows the data of α-diversity indices in various groups at 48 h post fermentation. In the microbial community diversity analysis, Chao1, PD, Shannon, and Simpson indices were investigated. Chao1 index is used to estimate the OTU numbers in the samples, while the PD index reflects phylogenetic diversity. Both the Simpson and Shannon indices usually depict the microbial diversity in samples (Liu et al., Citation2021). Our data showed that the Chao1 index and PD index in all the MI-SDF groups were higher than those in the BLK and INL groups, indicating that MI-SDF could promote the microbial proliferation. The Simpson and Shannon indices also confirmed that each sample group exhibited the same upward trend in bacterial uniformity and diversity. In addition, the MI-SDF H group displayed the highest relative abundance of gut microbiota among all MI-SDF groups.
Figure 6. Analysis of microbial Alpha diversity (a), and Beta diversity (b) during fermentation. Data are expressed as the mean ± standard deviation (n = 3). Morchella importuna-derived soluble dietary fiber was expressed as MI-SDF. The BLK group was fermented in vitro without carbon source; the INL group was fermented in vitro with 10 mg/ml inulin addition; the MI-SDF L group (6 mg/ml MI-SDF addition), MI-SDF M group (10 mg/ml MI-SDF addition), and MI-SDF H group (14 mg/ml MI-SDF addition) were fermented in vitro.
Figura 6. Análisis de la diversidad microbiana Alfa (a) y Beta (b) durante la fermentación. Los datos se presentan como la media ± desviación estándar (n = 3). La fibra dietética soluble derivada de Morchella importuna se presenta como MI-SDF. El grupo BLK se fermentó in vitro sin fuente de carbono; el grupo INL se fermentó in vitro con 10 mg/ml de adición de inulina; el grupo MI-SDF L (6 mg/ml de adición de MI-SDF), el grupo MI-SDF M (10 mg/ml de adición de MI-SDF) y el grupo MI-SDF H (14 mg/ml de adición de MI-SDF) se fermentaron in vitro.
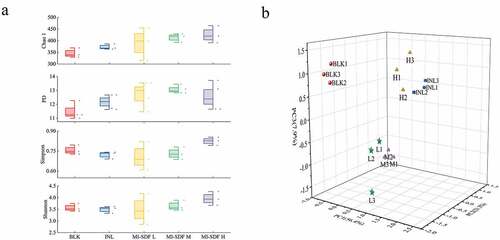
The diversity of the gut microbiota at 48 h post fermentation was further evaluated through three-dimensional principal coordinate analysis (3D PCA). PCA, as a typical β-diversity analysis method, can reveal the similarity of microbial community composition among different sample groups and the main factors affecting microbial diversity. The higher the similarity of the sample microbial composition, the closer the distance between the samples in the PCA plot (L. G. Chen et al., Citation2019). As shown in , PC1 (56.40%), PC2 (21.30%), and PC3 (7.90%) accounted for 85.60% of the total variation, indicating that the three-dimensional plane composed of these three lines can explain most of the data. We compared the distance between BLK group and INL group, MI-SDF L group, MI-SDF M group, or MI-SDF H group in PCA plot. The results showed that the INL group was far away from the BLK group, and the distances between BLK group and MI-SDF addition groups were increased with the addition of MI-SDF, indicating the addition of MI-SDF increase the difference in microbial community composition. The above results further indicated that the MI-SDF had effect on the microbial community composition.
3.5.1.2. Changes in gut microbiota structure at OTU, phylum, and genus levels
The gut microbiota structure changes at the OTU level were investigated after MI-SDF in vitro fermentation, and the OTUs of different groups were shown in Venn diagram (). Venn diagrams can be used to evaluate the number of both common and unique species in multiple samples and visually display the similarity and overlap of species composition among different samples (Ma et al., Citation2021). As shown in , the OTU number overlapped by the BLK group, INL group, and 3 MI-SDF addition groups (low, medium, and high) was 236, accounting for around 68.41% of the total OTU number. The OTU number of both MI-SDF group (339) and INL group (336) was higher than that of the BLK group (242). The number of OTUs shared by MI-SDF and INL groups was 94, which was significantly higher than that shared by BLK and MI-SDF groups, and that shared by BLK and INL groups. exhibits the similarity of species composition among the BLK group, INL group (10 mg/mL inulin), MI-SDF L group (6 mg/mL), MI-SDF M group (10 mg/mL), and MI-SDF H group (14 mg/mL). The OTU number shared by these five groups was 182, accounting for 52.75% of the total OTU number, of which the OTU number of MI-SDF L group, MI-SDF M group, and MI-SDF H group was 269, 287, and 290, respectively. These results indicated that the number of gut microbiota species was positively influenced by the MI-SDF and inulin. In vivio studies of a water-soluble polysaccharide (MP) extracted and isolated from wild morels have also shown that MP can increase the number of OTUs and the α-diversity index of gut microbiota (Huo, Feng, et al., Citation2020).
Figure 7. Effects of Morchella importuna-derived soluble dietary fiber (MI-SDF) in vitro fermentation on gut microbiota structure at OTU level (a&b) phylum level (c), and genus level (d); the Firmicutes to Bacteroidetes ratio at phylum level (e); the abundances of eight dominant genera except Bacteroides with varied prevalence among fermented samples (f); Heatmap analysis of the relative abundance at the genus level (g). Data are expressed as the mean ± standard deviation (n = 3). The full names and abbreviations of nouns are shown in .
Figura 7. Efectos de la fermentación in vitro de la fibra dietética soluble derivada de Morchella importuna (MI-SDF) en la estructura de la microbiota intestinal a nivel de OTU (a y b); a nivel de filo (c); y a nivel de género (d); la ratio entre Firmicutes y Bacteroidetes a nivel de filo (e); las abundancias de ocho géneros dominantes, excepto Bacteroides, con una prevalencia variada entre las muestras fermentadas (f); análisis de mapa de calor de la abundancia relativa a nivel de género (g). Los datos se presentan como la media ± desviación estándar (n = 3). Los sustantivos completos y las abreviaturas de los géneros aparecen en la Figura 6.
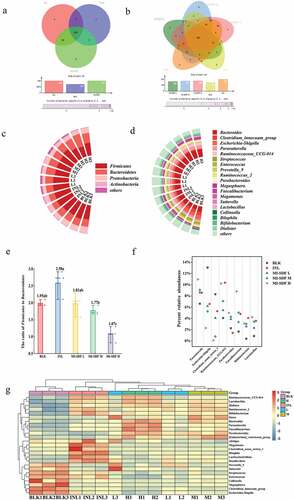
shows that at the level of phylum, Firmicutes, Bacteroidetes, Proteobacteria, and Actinobacteria were the dominant gut microbial communities. The relative abundance of Actinobacteria in INL group and 3 MI-SDF addition groups was significantly higher than that in the BLK group, while that of Proteobacteria was significantly lower (p < .05) [Supplemental content 2]. Bacteroidetes has been reported to be able to generate several short-chain fatty acids (SCFAs) (such as acetic acid and propionic acid) through a range of glycoside hydrolases and carbohydrate metabolic pathways (L. G. Chen et al., Citation2019). Previous research has shown that lowering the Firmicutes to Bacteroidetes ratio in the human gut is conducive to reducing the risk of obesity and insulin resistance (Fu et al., Citation2018; M. Y. Li et al., Citation2021; L. Li et al., Citation2022; Ma et al., Citation2021). In this study, relative abundance of Firmicutes and Bacteroidetes was not significantly different among BLK, MI-SDF L, MI-SDF M groups at the level of the phylum (p < .05). Firmicutes and Bacteroidetes accounted for 51.91 ± 2.12% and 26.16 ± 0.57% of the gut microbiota in the BLK group, respectively. Of all the groups, the MI-SDF H group exhibited the highest relative abundance of Bacteroidetes (39.29 ± 5.74%), but the lowest relative abundance of Firmicutes (41.07 ± 3.66%) [Supplemental content 2]. As shown, the ratio of Firmicutes to Bacteroidetes in the MI-SDF H group was significantly lower than that in the BLK group (p < .05), suggesting that MI-SDF played a key role in the intestinal microbiota’s health. In the study of wild morels polysaccharide (MP) changing the spatial structure of gut microbiota in mice, Huo et al. found that MP treatment induced a significant decrease in the number of Firmicutes and a significant increase in the number of Bacteroidetes in the small intestine microbiota (Huo, Qi, et al., Citation2020). In addition, Fu et al. also found that the ratio of Firmicutes and Bacteroides decreased after Sargassum thunbergii polysaccharides (ST-P2) fermentation compared with the control group (Fu et al., Citation2018).
The changes in gut microbiota structure and abundance at the genus level are shown in . Compared with those in the BLK group, the relative abundances of Bacteroides, Ruminococcus_2, Parasutterella, Ruminococcaceae_UCG-014, Parabacteroides, Bifidobacterium, Dialister, Faecalibacterium, and Lactobacillus in 3 MI-SDF addition groups were significantly (p < .05) increased, while those of Escherichia-Shigella and Clostridium innocuum group were markedly decreased [Supplemental content 3]. As an efficient glycan and protein degrader, Bacteroides was in absolute dominance in the MI-SDF H group, accounting for 29.13 ± 1.56% of total intestinal microbiota, which was the highest value among all the groups. The relative abundances of Ruminococcus_2, Parasutterella, and Ruminococcaceae_UCG-014 (key butyrate-producing taxa) were negatively correlated with intestinal inflammation, irritable bowel syndrome, obesity, and metabolic dysfunction (Y. J. Chen et al., Citation2018; Fu et al., Citation2018; Liu et al., Citation2021; Makki et al., Citation2018). Ruminococcus (propionic acid and butyric acid producers), Faecalibacterium (butyric acid producers), and Parabacteroides (succinate and secondary bile acids producers) have been well demonstrated to be responsible for the degradation of polysaccharides and fibers, thus relieving intestinal inflammation (Makki et al., Citation2018; K. Wang et al., Citation2019; Wu, Fu, et al., Citation2021). Escherichia-Shigella (belonging to the Proteobacteria phylum) has been identified as a harmful Gram-negative bacterium, and it is usually associated with many human gut pathogens (L. G. Chen et al., Citation2019; Fu et al., Citation2018; Liu et al., Citation2021; Wu, Fu, et al., Citation2021). Clostridium innocuum group is a vancomycin-resistant pathogen, and it can cause antibiotic-associated diarrhoea (Mefferd et al., Citation2020). In this study, the total relative abundance of two harmful bacteria including Escherichia-Shigella and Clostridium innocuum group in the BLK group was 24.21 ± 2.63%, which was significantly higher than that in the MI-SDF H group (3.26 ± 1.11%) and INL group (10.81 ± 3.53%). The inhibitory effect of MI-SDF H on above-mentioned harmful bacteria was more obvious than that of the INL group. Our data indicated that harmful pathogenic bacteria were markedly decreased by the intervention of MI-SDF. The potato residue-derived dietary fiber promoted the growth of Bifidobacterium, Lactobacillus, and Ruminococcaceae and inhibited the proliferation of Escherichia-Shigella and Clostridium perfringens (Ma et al., Citation2021), which was consistent with our results. A study demonstrated that wild morels polysaccharide (MP) could change the gut microbiota in both non-treated and cyclophosphamide (CP)-treated mice by increasing the population of SCFA-producing bacteria, such as Lachnospiraceae, Ruminococcaceae, and Erysipelotrichaceae (Huo, Feng, et al., Citation2020).
Compared with the BLK group, the INL group exhibited a higher relative abundance of beneficial bacteria such as Parasutterella, Lactobacillus, and Bifidobacterium and a lower relative abundance of harmful bacteria. Compared with INL group, the MI-SDF H group exhibited significantly higher relative abundances of Bacteroides, Parasutterella, Dialister, Parabacteroides, Faecalibacterium, and Lactobacillus (p < .05), while a significantly lower relative abundances of Escherichia-Shigella, Clostridium innocuum group, Streptococcus, Enterococcus, Megamonas, Bilophila and Bifidobacterium (p < .05). Inulin, a polymer of fructose and glucose, has previously been reported to stimulate the proliferation of Parasutterella, Bifidobacterium and Lactobacillus, which was in accordance with our results (Fu et al., Citation2018; Pérez-Burillo et al., Citation2019). As shown in , the relative abundances of intestinal beneficial bacteria Parasutterella, Ruminococcaceae_UCG-014, Faecalibacterium, and Lactobacillus were positively correlated with the dose of MI-SDF. The MI-SDF H group exhibited more species of beneficial bacteria than all other groups, and displayed the highest relative abundance of the following beneficial bacteria Bacteroides (29.13 ± 1.56%), Parasutterella (10.93 ± 0.40%), Faecalibacterium (8.81 ± 1.08%), Parabacteroides (4.98 ± 0.29%), Dialister (4.85 ± 0.27%), and Lactobacillus (4.24 ± 0.23%), indicating that MI-SDF at a high dose (14 mg/mL) was conducive to the proliferation of beneficial bacteria () [Supplemental content 3].
The heatmap analysis of the intestinal microbiota in five different groups showed that the relative abundances of Bacteroides, Parasutterella, Faecalibacterium, and Parabacteroides were significantly up-regulated in the MI-SDF H group, when compared with those in the BLK group, whereas the relative abundances of Collinsella, Megasphaera, Escherichia-Shigella, and Clostridium innocuum group were dramatically down-regulated (). The relative abundances of Megamonas, Bilophila, and Bifidobacterium were significantly up-regulated, while those of Collinsella, Megasphaera, Escherichia-Shigella, and Clostridium innocuum group were significantly down-regulated in the INL group, when compared with the BLK group. Collectively, both MI-SDF and inulin in vitro fermentation had a significant impact on the microbial communities. Notably, the high dose of MI-SDF (14 mg/mL) exhibited a great ability to regulate the gut metabolism function. Therefore, MI-SDF had potential prebiotic function by modulating the composition and abundance of gut microbiota.
3.5.2. Effect of MI-SDF in vitro fermentation on short-chain fatty acid yield
Short-chain fatty acids (SCFAs) are organic fatty acids with less than 6 carbon atoms in the carbon chain, and they are the major metabolites of dietary fiber fermentation by gut microbiota. SCFAs exert important effect on intestinal health. Specifically, SCFAs can promote acidification of the intestinal environment and influence the activities of microbial enzymes, thereby regulating intestinal immunity and maintaining colonic homeostasis (Huang et al., Citation2020). shows the changes in the yield of acetic acid, propionic acid, n-butyrate, and total acid during in vitro fermentation in the BLK, INL, MI-SDF L, MI-SDF M, and MI-SDF H groups at different time points. The concentration of total SCFAs in MI-SDF H group was increased to 26.89 ± 0.05 mM (at 48 h), which was significantly higher than that in the BLK group (3.87 ± 0.45 mM), INL group (14.67 ± 0.12 mM), MI-SDF L group (18.38 ± 0.30 mM), and MI-SDF M group (22.94 ± 0.75 mM) (p < .05). As shown in , there was no significant difference in the concentration of each type of SCFA among all groups at 0 h. The levels of total SCFA and individual SCFAs in all the five groups were gradually increased as the fermentation progressed. The concentrations of acetic acid, propionic acid, n-butyric acid, and the total acid in the samples were significantly increased with the dose of MI-SDF increasing at 12 h post fermentation, when compared with the BLK group. The results indicated that the MI-SDF at high dose had the best effect on the acceleration of the SCFA production.
Figure 8. Concentration changes of total and individual SCFAs during in vitro fermentation in the BLK, INL, MI-SDF L, MI-SDF M and MI-SDF H groups at different time points. Data are expressed as the mean ± standard deviation (n = 3), and different lowercase letters indicated statistical significance (p < .05). The full names and abbreviations of nouns are shown in .
Figura 8. Cambios en la concentración de SCFA totales e individuales durante la fermentación in vitro en los grupos BLK, INL, MI-SDF L, MI-SDF M y MI-SDF H en diferentes momentos. Los datos se presentan como la media ± la desviación estándar (n = 3), y las distintas letras minúsculas indican una diferencia estadística significativa (p < .05). Los nombres completos y las abreviaturas de los sustantivos aparecen en la Figura 6.
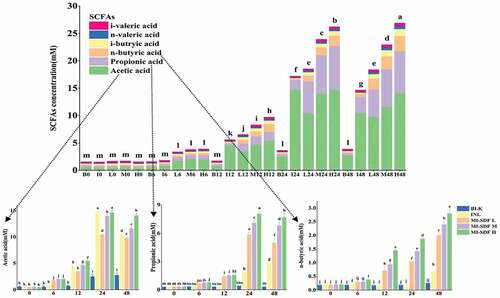
Acetic acid, which is mainly produced by Bifidobacterium and Lactobacillus, can keep the intestinal tract at an appropriate acidic pH level, and it can also combine with some receptors in the intestinal tract to control appetite and regulate fat storage. Moreover, acetic acid can also nourish butyric acid-producing bacteria in the intestine and promote the diversity of beneficial bacteria in the intestine (Huang et al., Citation2019). In addition to its function to lower cholesterol, reduce fat storage, prevent and control cancer and inflammation, propionic acid can regulate the release of orexin peptides YY and GLP-1 to prevent excessive intake of calories (L. G. Chen et al., Citation2019; M. M. Wang et al., Citation2019). In this study, acetic acid accounted for the highest proportion of individual SCFAs detected from the samples in five groups, followed by propionic acid and n-butyric acid. At 24 h post fermentation, the level of acetic acid in the MI-SDF H group was increased to 14.65 ± 0.41 mM, which was significantly higher than that in the BLK group (2.51 ± 0.41 mM), the MI-SDF L group (10.42 ± 0.17 mM), and the MI-SDF M group (13.97 ± 0.30 mM) (p < .05), while it was no significantly different from that in the INL group (14.68 ± 0.27 mM) (p > .05). The high level of acetic acid in the INL group and the MI-SDF H group might be due to the proliferation of Bifidobacterium and Lactobacillus. At 12 h post fermentation, the levels of propionic acid and n-butyric acid in the INL group were significantly lower than that in all the MI-SDF addition groups. At 48 h post fermentation, the concentrations of acetic and propionic acids exhibited a significant decline, suggesting that intestinal microbiota had exhausted the metabolic substrates (Guan et al., Citation2020). Our results were consistent with some study reports on the SCFAs change during fermentation of Sargassum thunbergii polysaccharides, loquat leaf polysaccharides, and snow chrysanthemum polysaccharides (Fu et al., Citation2018; Wu, Fu, et al., Citation2021; Wu, Yuan, et al., Citation2021). Butyric acid has been reported to have great antioxidant and anticancer properties, and it provides more than 90% of the total energy needed by colon cells (Ma et al., Citation2021). The high level of n-butyric acid in the MI-SDF H group was probably due to the enhancement of the relative abundance of Ruminococcaceae, Ruminococcus, and Faecalibacterium (Fu et al., Citation2018; Liu et al., Citation2021; Makki et al., Citation2018; Wu, Fu, et al., Citation2021). It is reported that wild morels polysaccharide also promoted the production of SCFAs in mice, such as butyric acid and valeric acid (Huo, Qi, et al., Citation2020). Taken together, our results indicated that MI-SDF had excellent prebiotic potential by promoting SCFA production.
4. Conclusions
In the present study, soluble dietary fiber was extracted from spent M. importuna, and its extraction process was optimized. The structural characteristic of MI-SDF and the effects of MI-SDF in vitro fermentation on intestinal microbiota composition and SCFA production were investigated. Response surface analysis results indicated that the extraction yield of MI-SDF reached 15.38 ± 0.16% under the optimized conditions (with an optimal lye concentration of 2.9%, an optimal extraction temperature of 71°C and an optimal extraction time of 2 h). MI-SDF had the typical structures of porous fiber, polysaccharide functional groups, and crystal structure of cellulose. MI-SDF exhibited a high nitrite ion adsorption capacity (NIAC), and it also displayed excellent thermal stability, thus it was applicable for food processing at <250°C. During in vitro fecal fermentation, the microbial composition was reshaped by MI-SDF. The relative abundances of beneficial bacteria including Parasutterella, Ruminococcaceae_UCG-014, Faecalibacterium, and Lactobacillus were significantly increased in a dose-dependent manner, while those of harmful bacteria such as Escherichia-Shigella and Clostridium innocuum group were markedly decreased. Meanwhile, the levels of total SCFA and individual SCFAs were significantly increased, particularly that of acetic acid and propionic acid. MI-SDF at a high dose (14 mg/mL) exhibited a better ability to regulate SCFA production and intestinal microbiota composition than MI-SDF at low (6 mg/mL) and medium (10 mg/mL) doses. Indeed, the actual in vivo fermentation of MI-SDF remains to be further investigated. SDF extraction is a deep processing of M. importuna, which can increase the additional value of the edible fungi industry. Our study indicates the great potential of MI-SDF as functional food ingredient in the food industry.
Author contribution
Jiayu Lei: Conceptualization, Methodology, Investigation, Writing-original draft; Yuting Zhang: Methodology, Investigation. Dongdong Guo: Software; Junlong Meng: Conceptualization, Methodology; Cuiping Feng: project administration; Lijing Xu: Resources; Yanfen Cheng: Formal analysis, Visualization; Rongzhu Liu: Visualization; Mingchang Chang: Resources, Data curation; Xueran Geng: Supervision, Writing – review and editing. All authors have read and agreed to the published version of the manuscript.
Supplemental Material
Download MS Word (29.7 KB)Acknowledgements
This work was supported by the Youth Project of Basic Research Program in Shanxi (20210302124071). The authors thank each teacher of Shanxi Key Laboratory of Edible Fungi for Loess Plateau for their contribution to this article. Great gratitude goes to linguistics Prof. Ping Liu from Huazhong Agriculture University, Wuhan, China for her work at English editing and language polishing.
Disclosure statement
No potential conflict of interest was reported by the author(s).
Supplementary material
Supplemental data for this article can be accessed online at https://doi.org/10.1080/19476337.2022.2093979.
Additional information
Funding
References
- Ajanth Praveen, M., Karthika Parvathy, K. R., Jayabalan, R., & Balasubramanian, P. (2019). Dietary fiber from Indian edible seaweeds and its in-vitro prebiotic effect on the gut microbiota. Food Hydrocolloids, 96, 343–353. https://doi.org/10.1016/j.foodhyd.2019.05.031
- Badshah, S. L., Riaz, A., Muhammad, A., Tel Cayan, G., Cayan, F., Emin Duru, M., & Jaremko, M. (2021). Isolation, characterization, and medicinal potential of Polysaccharides of Morchella esculenta. Molecules, 26(5), 1459. https://doi.org/10.3390/molecules26051459
- Behrouzian, F., Amini, A. M., Alghooneh, A., & Razavi, S. M. A. (2016). Characterization of dietary fiber from coffee silverskin: An optimization study using response surface methodology. Bioactive Carbohydrates and Dietary Fibre, 8(2), 58–64. https://doi.org/10.1016/j.bcdf.2016.11.004
- Cantu-Jungles, T. M., Ruthes, A. C., El-Hindawy, M., Moreno, R. B., Zhang, X. W., Cordeiro, L. M. C., Hamaker, B. R., & Iacomini, M. (2018). In vitro fermentation of Cookeina speciosa glucans stimulates the growth of the butyrogenic Clostridium cluster XIVa in a targeted way. Carbohydrate Polymers, 183, 219–229. https://doi.org/10.1016/j.carbpol.2017.12.020
- Caz, V., Gil-Ramirez, A., Largo, C., Tabernero, M., Santamaria, M., Martin-Hernandez, R., Reglero, G., & Soler-Rivas, C. (2015). Modulation of cholesterol-related gene expression by dietary fiber fractions from edible mushrooms. Journal of Agricultural and Food Chemistry, 63(33), 7371–7380. https://doi.org/10.1021/acs.jafc.5b02942
- Chen, C., Zhang, B., Fu, X., You, L. J., Abbasi, A. M., & Liu, R. H. (2016). The digestibility of mulberry fruit polysaccharides and its impact on lipolysis under simulated saliva, gastric and intestinal conditions. Food Hydrocolloids, 58, 171–178. https://doi.org/10.1016/j.foodhyd.2016.02.033
- Chen, Y. J., Wu, H., Wu, S. D., Lu, N., Wang, Y. T., Liu, H. N., Dong, L., Liu, T. T., & Shen, X. Z. (2018). Parasutterella, in association with irritable bowel syndrome and intestinal chronic inflammation. Journal of Gastroenterology and Hepatology, 33(11), 1844–1852. https://doi.org/10.1111/jgh.14281
- Chen, L. G., Liu, J. W., Ge, X. D., Xu, W., Chen, Y., Li, F. W., Cheng, D. L., & Shao, R. (2019). Simulated digestion and fermentation in vitro by human gut microbiota of polysaccharides from Helicteres angustifolia L. International Journal of Biological Macromolecules, 141, 1065–1071. https://doi.org/10.1016/j.ijbiomac.2019.09.073
- Comino, P., Williams, B. A., & Gidley, M. J. (2018). In vitro fermentation gas kinetics and end-products of soluble and insoluble cereal flour dietary fibres are similar. Food & Function, 9(2), 898–905. https://doi.org/10.1039/c7fo01724c
- Deng, Z. Z., Wu, N., Wang, J., & Zhang, Q. B. (2021). Dietary fibers extracted from Saccharina japonica can improve metabolic syndrome and ameliorate gut microbiota dysbiosis induced by high fat diet. Journal of Functional Foods, 85, 104642. https://doi.org/10.1016/j.jff.2021.104642
- Ding, Q. Z., Li, Z. K., Wu, W., Su, Y. Y., Sun, N. Z., Luo, L., Ma, H. L., & He, R. H. (2020). Physicochemical and functional properties of dietary fiber from Nannochloropsis oceanica: A comparison of alkaline and ultrasonic-assisted alkaline extractions. Lwt, 133, 110080. https://doi.org/10.1016/j.lwt.2020.110080
- Du, X. H., Zhao, Q., O’Donnell, K., Rooney, A. P., & Yang, Z. L. (2012). Multigene molecular phylogenetics reveals true morels (Morchella) are especially species-rich in China. Fungal Genetics and Biology, 49(6), 455–469. https://doi.org/10.1016/j.fgb.2012.03.006
- Du, X. H., Zhao, Q., & Yang, Z. L. (2014). Diversity, evolutionary history and cultivation of morels: A review. Mycosystema, 33(2), 183–197. (in Chinese). https://doi.org/10.13346/j.mycosystema.130260
- Du, X. J., Wang, L., Huang, X., Jing, H. J., Ye, X., Gao, W., Bai, X. P., & Wang, H. X. (2021). Effects of different extraction methods on structure and properties of soluble dietary fiber from defatted coconut flour. Lwt, 143, 111031. https://doi.org/10.1016/j.lwt.2021.111031
- Fu, X., Cao, C. L., Ren, B. B., Zhang, B., Huang, Q., & Li, C. (2018). Structural characterization and in vitro fermentation of a novel polysaccharide from Sargassum thunbergii and its impact on gut microbiota. Carbohydrate Polymers, 183, 230–239. https://doi.org/10.1016/j.carbpol.2017.12.048
- Gan, J. P., Huang, Z. Y., Yu, Q., Peng, G. Y., Chen, Y., Xie, J. H., Nie, S. P., & Xie, M. Y. (2020). Microwave assisted extraction with three modifications on structural and functional properties of soluble dietary fibers from grapefruit peel. Food Hydrocolloids, 101, 105549. https://doi.org/10.1016/j.foodhyd.2019.105549
- Guan, N. N., He, X. W., Wang, S. K., Liu, F. T., Huang, Q., Fu, X., Chen, T. T., & Zhang, B. (2020). Cell wall integrity of pulse modulates the in vitro fecal fermentation rate and microbiota composition. Journal of Agricultural and Food Chemistry, 68(4), 1091–1100. https://doi.org/10.1021/acs.jafc.9b06094
- He, Y., Wang, B. X., Wen, L. K., Wang, F. Z., Yu, H. S., Chen, D. X., Su, X., & Zhang, C. (2022). Effects of dietary fiber on human health. Food Science and Human Wellness, 11(1), 1–10. https://doi.org/10.1016/j.fshw.2021.07.001
- Honikel, K.-O. (2008). The use and control of nitrate and nitrite for the processing of meat products. Meat Science, 78(1–2), 68–76. https://doi.org/10.1016/j.meatsci.2007.05.030
- Hua, M., Lu, J. X., Qu, D., Liu, C., Zhang, L., Li, S. S., Chen, J. B., & Sun, Y. S. (2019). Structure, physicochemical properties and adsorption function of insoluble dietary fiber from ginseng residue: A potential functional ingredient. Food Chemistry, 286, 522–529. https://doi.org/10.1016/j.foodchem.2019.01.114
- Huang, F., Liu, Y., Zhang, R. F., Bai, Y. J., Dong, L. H., Liu, L., Jia, X. C., Wang, G. J., & Zhang, M. W. (2019). Structural characterization and in vitro gastrointestinal digestion and fermentation of litchi polysaccharide. International Journal of Biological Macromolecules, 140, 965–972. https://doi.org/10.1016/j.ijbiomac.2019.08.170
- Huang, F., Hong, R. X., Yi, Y., Bai, Y. J., Dong, L. H., Jia, X. C., Zhang, R. F., Wang, G. J., Zhang, M. W., & Wu, J. (2020). In vitro digestion and human gut microbiota fermentation of longan pulp polysaccharides as affected by Lactobacillus fermentum fermentation. International Journal of Biological Macromolecules, 147, 363–368. https://doi.org/10.1016/j.ijbiomac.2020.01.059
- Huo, W. Y., Feng, Z. P., Hu, S. Y., Cui, L. J., Qiao, T., Dai, L., & Li, J. Z. (2020). Effects of polysaccharides from wild morels on immune response and gut microbiota composition in non-treated and cyclophosphamide-treated mice. Food & Function, 11(5), 4291–4303. https://doi.org/10.1039/d0fo00597e
- Huo, W. Y., Qi, P., Cui, L. J., Zhang, L. G., Dai, L., Liu, Y., & Li, J. Z. (2020). Polysaccharide from wild morels alters the spatial structure of gut microbiota and the production of short-chain fatty acids in mice. Bioscience of Microbiota Food and Health, 39(4), 219–226. https://doi.org/10.12938/bmfh.2020-018
- Jia, F. J., Yang, S. F., Ma, Y. Y., Gong, Z. Q., Cui, W. J., Wang, Y. S., & Wang, W. L. (2020). Extraction optimization and constipation-relieving activity of dietary fiber from Auricularia polytricha. Food Bioscience, 33, 100506. https://doi.org/10.1016/j.fbio.2019.100506
- Jiang, Y. L., Yin, H., Zheng, Y. R., Wang, D. F., Liu, Z. M., Deng, Y., & Zhao, Y. Y. (2020). Structure, physicochemical and bioactive properties of dietary fibers from Akebia trifoliata (Thunb.) Koidz. seeds using ultrasonication/shear emulsifying/microwave-assisted enzymatic extraction. Food Research International, 136, 109348. https://doi.org/10.1016/j.foodres.2020.109348
- Karaman, E., Yılmaz, E., & Tuncel, N. B. (2017). Physicochemical, microstructural and functional characterization of dietary fibers extracted from lemon, orange and grapefruit seeds press meals. Bioactive Carbohydrates and Dietary Fibre, 11, 9–17. https://doi.org/10.1016/j.bcdf.2017.06.001
- Karra, S., Sebii, H., Yaich, H., Bouaziz, M. A., Blecker, C., Danthine, S., Attia, H., & Besbes, S. (2020). Effect of extraction methods on the physicochemical, structural, functional, and antioxidant properties of the dietary fiber concentrates from male date palm flowers. Journal of Food Biochemistry, 44(6), e13202. https://doi.org/10.1111/jfbc.13202
- Kedia, G., Vazquez, J. A., Charalampopoulos, D., & Pandiella, S. S. (2009). In vitro fermentation of oat bran obtained by debranning with a mixed culture of human fecal bacteria. Current Microbiology, 58(4), 338–342. https://doi.org/10.1007/s00284-008-9335-1
- Kurek, M. A., Karp, S., Wyrwisz, J., & Niu, Y. (2018). Physicochemical properties of dietary fibers extracted from gluten-free sources: Quinoa (Chenopodium quinoa), amaranth (Amaranthus caudatus) and millet (Panicum miliaceum). Food Hydrocolloids, 85, 321–330. https://doi.org/10.1016/j.foodhyd.2018.07.021
- Laforteza, J. C., Reyes, R. G., & Trinidad, T. P. (2020). dietary fiber contents and its fermentabilityin vitro of Pleurotus ostreatus cv. Florida Mycelia (Agaricomycetes). International Journal of Medicinal Mushrooms, 22(7), 651–657. https://doi.org/10.1615/intjmedmushrooms.2020035449
- Li, S. H., Gao, A., Dong, S., Chen, Y., Sun, S., Lei, Z. F., & Zhang, Z. Y. (2017). Purification, antitumor and immunomodulatory activity of polysaccharides from soybean residue fermented with Morchella esculenta. International Journal of Biological Macromolecules, 96, 26–34. https://doi.org/10.1016/j.ijbiomac.2016.12.007
- Li, M. Y., Yu, L. L., Zhao, J. X., Zhang, H., Chen, W., Zhai, Q. X., & Tian, F. W. (2021). Role of dietary edible mushrooms in the modulation of gut microbiota. Journal of Functional Foods, 83, 104538. https://doi.org/10.1016/j.jff.2021.104538
- Li, L., Zhao, Y. F., Li, J. Q., Ban, L. Z., Yang, L., Wang, S. N., Zhu, L. J., Song, H., & Liu, H. (2022). The adhesion of the gut microbiota to insoluble dietary fiber from soy hulls promoted the proliferation of probiotics in vitro. Lwt, 153, 112560. https://doi.org/10.1016/j.lwt.2021.112560
- Li, Y. X., Niu, L., Guo, Q. Q., Shi, L. C., Deng, X., Liu, X. B., & Xiao, C. X. (2022). Effects of fermentation with lactic bacteria on the structural characteristics and physicochemical and functional properties of soluble dietary fiber from prosomillet bran. Lwt, 154, 112609. https://doi.org/10.1016/j.lwt.2021.112609
- Liang, J. J., Zhang, M. N., Wang, X. N., Ren, Y. C., Yue, T. L., Wang, Z. L., & Gao, Z. P. (2021). Edible fungal polysaccharides, the gut microbiota, and host health. Carbohydrate Polymers, 273, 118558. https://doi.org/10.1016/j.carbpol.2021.118558
- Liu, C., Du, P., Cheng, Y. L., Guo, Y. H., Hu, B., Yao, W. R., Zhu, X., & Qian, H. (2021). Study on fecal fermentation characteristics of aloe polysaccharides in vitro and their predictive modeling. Carbohydrate Polymers, 256, 117571. https://doi.org/10.1016/j.carbpol.2020.117571
- Lundberg, J. O., Gladwin, M. T., Ahluwalia, A., Benjamin, N., Bryan, N. S., Butler, A., Cabrales, P., Fago, A., Feelisch, M., & Ford, P. C. (2009). Nitrate and nitrite in biology, nutrition and therapeutics. Nature Chemical Biology, 5(12), 865–869. https://doi.org/10.1038/nchembio.260
- Ma, Q. Y., Wang, W. X., Ma, Z. Y., Liu, Y. Q., Mu, J. L., Wang, J., Stipkovits, L., Wu, G., Sun, J. F., & Hui, X. D. (2021). Enzymatic-Modified dietary fibre fraction extracted from potato residue regulates the gut microbiotas and production of short-chain fatty acids of C57BL/6 mice. Journal of Functional Foods, 84, 104606. https://doi.org/10.1016/j.jff.2021.104606
- Makki, K., Deehan, E. C., Walter, J., & Backhed, F. (2018). The impact of dietary fiber on gut microbiota in host health and disease. Cell Host & Microbe & Cell Host & Microbe, 23(6), 705–715. https://doi.org/10.1016/j.chom.2018.05.012
- McRae, M. P. (2018). The benefits of dietary fiber intake on reducing the risk of cancer: An umbrella review of meta-analyses. Journal of Chiropractic Medicine, 17(2), 90–96. https://doi.org/10.1016/j.jcm.2017.12.001
- Mefferd, C. C., Bhute, S. S., Phan, J. R., Villarama, J. V., Do, D. M., Alarcia, S., Abel-Santos, E., & Hedlund, B. P. (2020). A high-fat/high-protein, Atkins-type diet exacerbates Clostridioides (Clostridium) difficile infection in mice, whereas a high-carbohydrate diet protects. mSystems, 5(1), e00765–19. https://doi.org/10.1128/mSystems.00765-19
- Munoz, L. A., Vera, C. N., Zuniga-Lopez, M. C., Moncada, M., & Haros, C. M. (2021). Physicochemical and functional properties of soluble fiber extracted from two phenotypes of chia (Salvia hispanica L.) seeds. Journal of Food Composition and Analysis, 104, 104138. https://doi.org/10.1016/j.jfca.2021.104138
- Neyrinck, A. M., Rodriguez, J., Zhang, Z., Seethaler, B., Sánchez, C. R., Roumain, M., Hiel, S., Bindels, L. B., Cani, P. D., Paquot, N., Cnop, M., Laville, M., Muccioli, G. G., Thissen, J. P., Bischoff, S. C., Walter, J., & Delzenne, N. M. (2020). Fecal metabolites reflecting the interaction between prebiotic dietary fiber and the gut microbiota in obese patients. Clinical Nutrition ESPEN, 40, 521–522. https://doi.org/10.1016/j.clnesp.2020.09.346
- Ng, S. H., Robert, S. D., Wan Ahmad, W. A. N., & Wan Ishak, W. R. (2017). Incorporation of dietary fibre-rich oyster mushroom (Pleurotus sajor-caju) powder improves postprandial glycaemic response by interfering with starch granule structure and starch digestibility of biscuit. Food Chemistry, 227, 358–368. https://doi.org/10.1016/j.foodchem.2017.01.108
- Pérez-Burillo, S., Mehta, T., Pastoriza, S., Kramer, D. L., Paliy, O., & Rufián-Henares, J. Á. (2019). Potential probiotic salami with dietary fiber modulates antioxidant capacity, short chain fatty acid production and gut microbiota community structure. Lwt, 105, 355–362. https://doi.org/10.1016/j.lwt.2019.02.006
- Pourfarzad, A., Mahdavian-Mehr, H., & Sedaghat, N. (2013). Coffee silverskin as a source of dietary fiber in bread-making: Optimization of chemical treatment using response surface methodology. Lwt, 50(2), 599–606. https://doi.org/10.1016/j.lwt.2012.08.001
- Tejada-Ortigoza, V., Garcia-Amezquita, L. E., Campanella, O. H., Hamaker, B. R., & Welti-Chanes, J. (2022). Extrusion effect on in vitro fecal fermentation of fruit peels used as dietary fiber sources. Lwt, 153, 112569. https://doi.org/10.1016/j.lwt.2021.112569
- Tietel, Z., & Masaphy, S. (2018). True morels (Morchella)-nutritional and phytochemical composition, health benefits and flavor: A review. Critical Reviews in Food Science and Nutrition, 58(11), 1888–1901. https://doi.org/10.1080/10408398.2017.1285269
- Wang, H. Y., Hong, T., Li, N., Zang, B., & Wu, X. W. (2018). Soluble dietary fiber improves energy homeostasis in obese mice by remodeling the gut microbiota. Biochemical and Biophysical Research Communication, 498(1), 146–151. https://doi.org/10.1016/j.bbrc.2018.02.017
- Wang, K., Liao, M. F., Zhou, N., Bao, L., Ma, K., Zheng, Z. Y., Wang, Y. J., Liu, C., Wang, W. Z., Wang, J., Liu, S. J., & Liu, H. W. (2019). Parabacteroides distasonis alleviates obesity and metabolic dysfunctions via production of succinate and secondary bile Acids. Cell Reports, 26(1), 222–235. https://doi.org/10.1016/j.celrep.2018.12.028
- Wang, M. J., Chen, G. J., Chen, D., Ye, H., Sun, Y., Zeng, X. X., & Liu, Z. H. (2019). Purified fraction of polysaccharides from Fuzhuan brick tea modulates the composition and metabolism of gut microbiota in anaerobic fermentation in vitro. International Journal of Biological Macromolecules, 140, 858–870. https://doi.org/10.1016/j.ijbiomac.2019.08.187
- Wang, M. M., Wichienchot, S., He, X. W., Fu, X., Huang, Q., & Zhang, B. (2019). In vitro colonic fermentation of dietary fibers: Fermentation rate, short-chain fatty acid production and changes in microbiota. Trends in Food Science & Technology, 88, 1–9. https://doi.org/10.1016/j.tifs.2019.03.005
- Wang, K. J., Guo, J. T., Cheng, J. X., Zhao, X. H., Ma, B. H., Yang, X. B., & Shao, H. J. (2021). Ultrasound-Assisted extraction of polysaccharide from spent Lentinus edodes substrate: Process optimization, precipitation, structural characterization and antioxidant activity. International Journal of Biological Macromolecules, 191, 1038–1045. https://doi.org/10.1016/j.ijbiomac.2021.09.174
- Wang, K. L., Li, M., Wang, Y. X., Liu, Z. H., & Ni, Y. Y. (2021). Effects of extraction methods on the structural characteristics and functional properties of dietary fiber extracted from kiwifruit (Actinidia deliciosa). Food Hydrocolloids, 110, 106162. https://doi.org/10.1016/j.foodhyd.2020.106162
- Wang, L. W., Brennan, M. A., Guan, W. Q., Liu, J. F., Zhao, H., & Brennan, C. S. (2021). Edible mushrooms dietary fibre and antioxidants: Effects on glycaemic load manipulation and their correlations pre-and post-simulated in vitro digestion. Food Chemistry, 351, 129320. https://doi.org/10.1016/j.foodchem.2021.129320
- Wang, W. L., Yang, S. F., Song, S. S., Zhang, J., & Jia, F. J. (2021). Flammulina velutipes mycorrhizae dietary fiber improves lipid metabolism disorders in obese mice through activating AMPK signaling pathway mediated by gut microbiota. Food Bioscience, 43, 101246. https://doi.org/10.1016/j.fbio.2021.101246
- Wen, Y., Peng, D., Li, C. L., Hu, X. J., Bi, S. X., Song, L. Y., & Yu, R. M. (2019). A new polysaccharide isolated from Morchella importuna fruiting bodies and its immunoregulatory mechanism. International Journal of Biological Macromolecules, 137, 8–19. https://doi.org/10.1016/j.ijbiomac.2019.06.171
- Wen, Y., Bi, S. X., Hu, X. J., Yang, J. N., Li, C. L., Li, H., Yu, D. B., Zhu, J. H., Song, L. Y., & Yu, R. M. (2021). Structural characterization and immunomodulatory mechanisms of two novel glucans from Morchella importuna fruiting bodies. International Journal of Biological Macromolecules, 183, 145–157. https://doi.org/10.1016/j.ijbiomac.2021.04.084
- Wu, D. T., Yuan, Q., Guo, H., Fu, Y., Li, F., Wang, S. P., & Gan, R. Y. (2021). Dynamic changes of structural characteristics of snow chrysanthemum polysaccharides during in vitro digestion and fecal fermentation and related impacts on gut microbiota. Food Research International, 141, 109888. https://doi.org/10.1016/j.foodres.2020.109888
- Wu, D. T., Fu, Y., Guo, H., Yuan, Q., Nie, X. R., Wang, S. P., & Gan, R. Y. (2021). In vitro simulated digestion and fecal fermentation of polysaccharides from loquat leaves: Dynamic changes in physicochemical properties and impacts on human gut microbiota. International Journal of Biological Macromolecules, 168, 733–742. https://doi.org/10.1016/j.ijbiomac.2020.11.130
- Xu, R., Liu, Y. P., Zhang, X. Y., L, T., Su, X., & La, B. (2016). Study on nutritional components of Morchella vulgaris from Beishan Forest in Huzhu of Qinghai Province. Northern Horticulture, 4(10), 138–140. (in Chinese). https://doi.org/10.11937/bfyy.201610035
- Xue, Z. H., Ma, Q. Q., Chen, Y., Lu, Y. P., Wang, Y. J., Jia, Y. N., & Zhang, M. &.Chen, H. X. (2020). Structure characterization of soluble dietary fiber fractions from mushroom Lentinula edodes (Berk.) Pegler and the effects on fermentation and human gut microbiota in vitro. Food Research International, 129, 108870. https://doi.org/10.1016/j.foodres.2019.108870
- Yang, Y. X., Chen, J. L., Lei, L., Li, F. H., Tang, Y., Yuan, Y., & Ming, J. (2019). Acetylation of polysaccharide from Morchella angusticeps peck enhances its immune activation and anti-inflammatory activities in macrophage RAW264.7cells. Food and Chemical Toxicology, 125, 38–45. https://doi.org/10.1016/j.fct.2018.12.036
- Zhang, N. N., Ma, H., Zhang, Z. F., Zhang, W. N., Chen, L., Pan, W. J., & Chen, Y. (2022). Characterization and immunomodulatory effect of an alkali-extracted galactomannan from Morchella esculenta. Carbohydrate Polymers, 278, 118960. https://doi.org/10.1016/j.carbpol.2021.118960
- Zhang, Z., Shi, M. H., Zheng, H. Y., Ren, R. F., Zhang, S. P., & Ma, X. L. (2022). Structural characterization and biological activities of a new polysaccharide isolated from Morchella Sextelata. Glycoconjugate Journal 39(3), 369–380. https://doi.org/10.1007/s10719-022-10058-8
- Zhao, R. Q., Yang, W. J., Pei, F., Zhao, L. Y., & Hu, Q. H. (2018). In vitro fermentation of six kinds of edible mushrooms and its effects on fecal microbiota composition. Lwt, 96, 627–635. https://doi.org/10.1016/j.lwt.2018.06.012