ABSTRACT
Eight Solanum lycopersicum var. cerasiforme accessions from Mexico were evaluated for total phenolics (TP) and flavonoids (TF), phenolic profiles (UPLC-DAD-MS), antioxidant capacity (AC) (ABTS, DPPH, and FRAP), and expression of transcription factors (MYB12 and MYB14) and target genes (PAL, CHS and CHI) by RT-qPCR. The AC (mmol TE/kg fw; TE, Trolox Equivalents) by ABTS (5.27–11.81), DPPH (1.43–3.67), and FRAP (4.41–9.22) correlated with TP (0.53–1.20 g GAE/kg fw; GAE, Gallic Acid Equivalents), TF (1.03–2.10 g QE/kg; QE, Quercetin Equivalents), and the levels of chlorogenic and dicaffeoylquinic acids and rutin. The expression of MYB12 and MYB14 correlated with that of PAL and CHS, while CHI was only associated with MYB12. The accessions Tumbisca and Kilim showed the highest gene expression, phenolics content, and AC, suggesting they can be used in breeding programs to produce tomatoes with better AC.
RESUMEN
Ocho accesiones de Solanum lycopersicum var. cerasiforme de México se evaluaron en contenido de fenólicos totales (FT), flavonoides totales (FLT), perfil de fenólicos (UPLC-DAD-MS), capacidad antioxidante (CAox) (ABTS, DPPH y FRAP) y expresión de factores de transcripción (MYB12 y MYB14) y genes diana (PAL, CHS y CHI) por RT-qPCR. La CAox (mmol ET/kg pf; ET, Equivalentes de Trolox) por ABTS (5.27-11.81), DPPH (1.43-3.67) y FRAP (4.41-9.22) se correlacionó con FT (0.53-1.20 g EAG/kg pf; EAG, Equivalentes de Ácido Gálico), FLT (1.03-2.10 g EQ/kg; EQ, Equivalentes de Quercetina) y los niveles de ácido clorogénico y dicafeoilquínico y rutina. La expresión de MYB12 y MYB14 correlacionó con la de PAL y CHS, mientras que CHI solo mostró asociación con MYB12. Las accesiones Tumbisca y Kilim mostraron la mayor expresión génica, contenido de fenólicos y CAox, sugiriendo que pueden ser usadas en programas de mejoramiento para producir tomates con mejor CAox.
PALABRAS CLAVE:
1. Introduction
The wild cherry tomato Solanum lycopersicum var. cerasiforme represents an important source of compounds with nutritional and biological activities (Adalid et al., Citation2010; Boches et al., Citation2011; Hanson et al., Citation2004). S. lycopersicum var. cerasiforme is widely distributed in Mexico and the accessions show significant variations in fruit quality (e.g., color, lycopene and vitamin C content) (Crisanto-Juárez et al., Citation2010; Juárez-López et al., Citation2009), as well as in the content of phenolic compounds and antioxidant and antimutagenic activities (Delgado-Vargas et al., Citation2018), characteristics that can be used to improve nutraceutical characteristics of tomato cultivars.
The synthesis of phenylpropanoids is regulated by MYB transcription factors and several researchers have used biotechnological strategies to improve the levels of bioactive compounds in tomato. The overexpression of AtMYB12 (Luo et al., Citation2008; Pandey et al., Citation2015) and AtMYB11 (Li et al., Citation2015) in tomato fruit increased significantly the synthesis of caffeoyl quinic acids and flavonols, which has been associated with the up-regulation of genes involved in the synthesis of phenylpropanoids (Ding et al., Citation2022; Li et al., Citation2021; Pandey et al., Citation2015).
Delgado-Vargas et al. (Citation2018) previously showed that the levels of phenolic compounds in some S. lycopersicum var. cerasiforme accessions from Mexico were close to those of transgenic tomato fruit overexpressing MYB transcription factors (Li et al., Citation2015; Luo et al., Citation2008), suggesting that the synthesis of phenylpropanoids is up-regulated in these tomato genotypes. The aim of this study was to analyze the expression of MYB transcription factors and target genes and its association with the levels of phenolic compounds and the antioxidant capacity in some of these wild tomato accessions.
2. Materials and methods
2.1. Plant material
Eight accessions of S. lycopersicum var. cerasiforme from seven states of Mexico and the commercial variety Moneymaker were grown under irrigation conditions (Delgado-Vargas et al., Citation2018), winter-spring season of 2018–2019, at the Faculty of Agronomy Field Station of the Autonomous University of Sinaloa, Culiacan Sinaloa, Mexico. Three replicates of five plants per accession were used and ten fruit per plant were harvested at the red-ripe stage. Yellow-fruited accessions were harvested based on maximum color intensity and fruit size. Ten fruit per replicate were used in Moneymaker. Fruit from each replicate were cut in half to remove the seeds and the pulp with a small spoon. One half of the pericarp sample from each replicate was frozen and the other was lyophilized. Both samples were stored at −70°C until use.
2.2. Preparation of methanol extracts
Methanol extracts were prepared according to Delgado-Vargas et al. (Citation2018). Lyophilized pericarp tissue from each replicate was ground to a fine powder and 0.2 g were mixed with 2 mL of methanol. The mixture was sonicated for 20 min, centrifuged (14,000 × g, 20 min, 20 °C) and the supernatant was recovered. The residue was extracted again two times, the supernatants were mixed and then dried under vacuum (40°C, BÜCHI R-124, Brinkmann Instruments, USA). The residue was resuspended in 2 mL of methanol, filtered (0.45 µm) and stored at −20°C until use.
2.3. Total phenolics and flavonoids
Methanol extracts were used to determine total phenolics content by the Folin-Ciocalteu reaction (Waterhouse, Citation2002) and total flavonoids according to Kim et al. (Citation2003). The results were expressed as grams of Gallic Acid Equivalents (GAE) and Quercetin Equivalents (QE) per kilogram of fresh weight, respectively.
2.4. Phenolic profiles
Phenolic profiles were obtained according to Delgado-Vargas et al. (Citation2018). A 5-µL aliquot of the methanol extract was analyzed with a UPLC-DAD system (ACCELA, Thermo Scientific, USA) using a Fortis C18 column (3 µm, 50 × 2.1 mm) (Fortis Technologies Ltd, UK) and a flow rate of 0.2 mL/min with a linear gradient of 1% (v/v) formic acid (A) and acetonitrile (B), 0.5 to 60% of B in 40 min. The compounds were detected at 280, 320, and 350 nm. Sinapic acid was used as internal standard. The quantification was based on calibration curves: phenolic acids (coumaric, chlorogenic, and caffeic) and flavonoids (rutin, quercetin and naringenin) (Sigma-Aldrich, St. Louis, USA). The results were expressed in milligrams per kilogram of fresh weight (mg kg−1 fw). The UPLC-DAD was coupled to a mass spectrometer with an electrospray ionization source (ESI) (LTQ XL, Thermo Scientific, USA). The analysis was carried out in negative/positive mode and full scan spectra were obtained (m/z = 50–1500). For MSn experiments the ions were fragmented by collision induced dissociation (10–45 V) using helium. The capillary tube conditions were 35 V and 300°C. Nitrogen was used for drying. The Xcalibur 2.2 software (Thermo Scientific, USA) was used for analysis of the results.
2.5. Antioxidant capacity (AC)
The AC of the methanol extracts was evaluated by three methods: 2,2´-azino-bis(3-ethylbenzothiazolin)-6-sulfonic acid (ABTS) (Re et al., Citation1999), 2,2-diphenil-1-pycrilhydrazyl (DPPH) (Brand-Williams et al., Citation1995), and ferric-reducing antioxidant power (FRAP) (Benzie & Strain, Citation1996). The three methods were performed using 96-well microplates and the results were expressed as millimoles of Trolox Equivalents per kilogram of fresh weight (mmol TE/kg fw).
2.6. Antioxidant interactions between major phenolic compounds found in the wild tomato accessions
Commercial standards corresponding to the major phenolics of the methanol extracts were used to evaluate their AC (ABTS, DPPH, FRAP) and possible interactions. Evaluations were carried out at the concentration found in the extract of the accession Tumbisca or at 50 μmol/L. For each mixture, the AC values of each compound (50 μmol/L) were added to calculate the expected activity, which was compared with the value observed in the mixture to establish the type of interaction (additive, synergistic or antagonistic).
3. RNA isolation
Total RNA was isolated using Trizol (Invitrogen, Carlsbad, USA) according to the manufacturer’s instructions. Frozen pericarp tissue from each replicate was ground in liquid nitrogen and 0.5 g were mixed with 1 mL of Trizol, incubated on ice for 5 min and then centrifuged at 12,000 × g (15 min, 4 °C). The supernatant was mixed with 0.4 mL of chloroform, incubated for 3 min and then centrifuged (12000 × g, 15 min, 4 °C). The aqueous phase was mixed with 0.5 mL of cold isopropanol, incubated on ice for 10 min and then centrifuged (12000 × g, 10 min, 4 °C). The pellet was washed with cold ethanol (75%, v/v), centrifuged (7500 × g, 10 min, 4 °C), dried and resuspended in 25 µL of water free of ribonucleases. Contaminant DNA was removed using the DNase I kit (Sigma-Aldrich, St. Louis, USA).
4. Gene expression analysis
The expression of MYB transcription factors (MYB12 and MYB14) and target genes (PAL, CHS and CHI) was determined by RT-qPCR. The RNA was quantified spectrophotometrically and 10 ng were used for the analysis using the kit SCRIPT One-Step RT-PCR (Jena Bioscience, California, USA). The amplification was performed using a real-time system model StepOnePlusTM (Applied Biosystems, Carlsbad, USA). The PCR primers (Supplementary Table S1) were designed using the Primer-BLAST program (http://www.ncbi.nlm.nih.gov/tools/primer-blast). The amplification conditions were: 50°C for 30 min for cDNA synthesis, then 95°C for 10 min (denaturation), followed by 40 cycles at 95°C (15 s) and 60°C (1 min) with a ramp rate of 2.2°C s−1. The Ubiquitin-3 (UBI3) gene was used as control and the expression values were calculated relative to the cultivar Moneymaker.
4.1. Statistical analysis
Data were analyzed by one-way analysis of variance and the Fisher test (α = 0.05). The values are the mean of three replicates. Correlation and principal component analyses were also carried out. All analyses were performed using the software STATGRAPHIC plus version 5.1 (Statistical Graphics Corporation™, USA).
5. Results and discussion
5.1. Antioxidant capacity (AC)
The AC of the methanol extracts showed a great variability among the eight accessions (). The highest AC for the three methods was observed in Tumbisca and the values were up to four times higher than those of the commercial variety Moneymaker. The AC values were similar to those observed by Delgado-Vargas et al. (Citation2018) in the whole fruit of these accessions and higher than those reported for commercial cultivars (Kaur et al., Citation2013). These results suggest that the selected accessions represent good sources of antioxidants.
Table 1. Total phenolics and flavonoids content and antioxidant capacity of the methanol extracts from the pericarp of Solanum lycopersicum var. cerasiforme accessionsz.
Tabla 1. Contenido de fenólicos y favonoides totales y capacidad antioxidante de extractos metanólicos del pericarpio de accesiones de Solanum lycopersicum var. cerasiformez.
5.2. Phenolics and flavonoids contents and their association with the antioxidant capacity
Total phenolics (TP) of the methanol extracts (0.53 to 1.20 g GAE/kg) () showed highly significant correlations with the AC measured by ABTS (r = 0.94; p ≤ 0.001), DPPH (r = 0.82; p ≤ 0.001), and FRAP (r = 0.91; p ≤ 0.001) (Supplementary Table S2). Similar results were previously observed by Delgado-Vargas et al. (Citation2018) in the whole fruit of wild tomato accessions from México.
The content of flavonoids (1.03–2.10 g QE/kg) also correlated with the AC by the three methods: ABTS (r = 0.69; p ≤ 0.001), DPPH (r = 0.75; p ≤ 0.001), FRAP (r = 0.72; p ≤ 0.001) (Supplementary Table S2). The accession Tumbisca showed the highest value, being 4-fold higher than that of Moneymaker (0.54 g QE/kg), corresponding with previous studies reporting up to 4 times more flavonoid content in wild type cherry tomato (S. pimpinellifolium and S. cheesmaniae) compared to commercial varieties (Kavitha et al., Citation2014).
The methanol extracts from the eight accessions showed similar phenolic profiles with 16 peaks () but variable abundance; the identity of these peaks is shown in Supplementary Table S3. The most abundant phenolics (mg/kg fw) were chlorogenic acid (66.5–193.4), and its derivatives di- and tricaffeoylquinic acid (8.24–125.9) (), as well as the flavonoid rutin (36.9–159.7) (). These results agree with those obtained previously in the whole fruit of the same accessions (Delgado-Vargas et al., Citation2018) and those reported for the methanol extracts in five S. lycopersicum var. cerasiforme accessions of a collection from Cornell University (Boches et al., Citation2011). Tumbisca showed the highest levels of most phenolics, followed by Kilim; the yellow-fruited accessions (Placharosa and Villamaza) showed significantly higher contents of naringenin chalcone (). The contents of chlorogenic, di- and tricaffeoylquinic acids in the accessions were up to 8 times higher than those of the commercial cultivar Moneymaker ().
Figure 1. UPLC-DAD phenolic profile of the methanol extract from the pericarp of Solanum lycopersicum var. cerasiforme accession Rincon. Similar profiles with quantitative variations were obtained for the other accessions. Peaks were identified as caffeoyl hexose I (1), cis-p-coumaric hexose acid (2), trans-p-coumaric hexose acid (3), chlorogenic acid (4), dicaffeoylquinic acid II (5), rutin hexose (6), rutin pentoside (7), rutin (8), quercetin dihexose pentoside deoxyhexose (9), naringenin chalcone hexose I (10), dicaffeoylquinic acid III (11), naringenin-O-hexose (12), naringenin chalcone hexose II (13), naringenin chalcone hexose III (14), tricaffeoylquinic acid (15), naringenin chalcone (16). Sinapic acid was used as internal standard (IS).
Figura 1. Perfil de fenólicos por UPLC-DAD del extracto metanólico del pericarpio de la accesión Rincon de Solanum lycopersicum var. cerasiforme. Todas las accesiones mostraron perfiles similares con variaciones cuantitativas. Los picos fueron identificados como cafeoil hexosa I (1), ácido cis-p-coumárico hexosa (2), ácido trans-p-coumárico hexosa (3), ácido clorogénico (4), ácido dicafeolquinico II (5), rutina hexosa (6), rutina pentósido (7), rutina (8), quercetina dihexosa pentósido desoxihexosa (9), naringenina chalcona hexosa I (10), ácido dicafeoilquínico III (11), naringenina-O-hexosa (12), naringenina chalcona hexosa II (13), naringenina chalcona hexosa III (14), ácido tricafeoilquínico (15), naringenina chalcona (16). El ácido sinápico fue usado como estándar interno (IS).
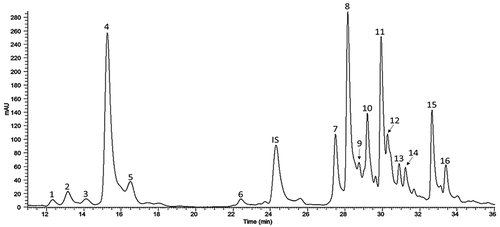
Table 2. Phenolic acids content in the methanol extracts from the pericarp of Solanum lycopersicum var. cerasiforme accessionsz.
Tabla 2. Contenido de ácidos fenólicos en extractos metanólicos del pericarpio de accesiones de Solanum lycopersicum var. cerasiformez.
Table 3. Flavonoids content in the methanol extracts from the pericarp of Solanum lycopersicum var. cerasiforme accessionsz.
Tabla 3. Contenido de flavonoides en extractos metanólicos del pericarpio de accesiones de Solanum lycopersicum var. cerasiformez.
The levels of the major phenolics in some of the accessions were close to those of transgenic Micro-Tom fruit expressing the AtMYB12 transcription factor (Luo et al., Citation2008) and similar to those of the fruit peel from the CLS cultivar transformed with AtMYB11 (Li et al., Citation2015). Thus, the high levels of phenolics in the wild tomato accessions appear to be associated with the up-regulation of the phenylpropanoid pathway.
Two principal components (PC) explained 69.7% of the total variation in phenolics content and AC (): PC1 (55.16%) included the AC and the content of total phenolics, chlorogenic acid and rutin, whereas PC2 (14.57%) was associated with the content of naringenin chalcone and naringenin hexose. This analysis highlighted that Tumbisca and Kilim were the accessions with the highest values of AC, total phenolics, chlorogenic acid and rutin.
Figure 2. Principal component analysis of eight wild tomato (Solanum lycopersicum var. cerasiforme) accessions and the commercial variety Moneymaker using the content of phenolics and the antioxidant activity (ABTS, DPPH, FRAP).
Figura 2. Análisis de componentes principales de ocho accesiones de tomate silvestre (Solanum lycopersicum var. cerasiforme) y la variedad comercial Moneymaker usando el contenido de compuestos fenólicos y la actividad antioxidante (ABTS, DPPH, FRAP).
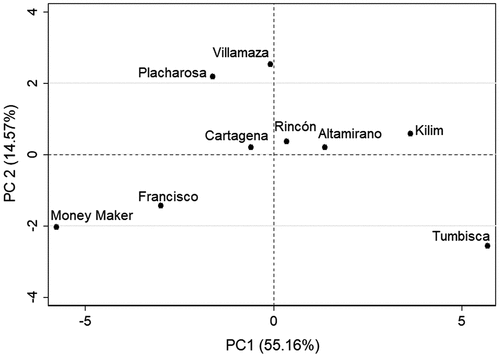
5.3. Antioxidant interactions between the major phenolic compounds of the methanol extracts
The major phenolics (chlorogenic acid, p-coumaric acid, rutin, and naringenin) were first evaluated at the same concentration found in the extract of the accession Tumbisca to determine their contribution to the AC (). Chlorogenic acid standard (417.0 µmol/L) showed the highest AC by ABTS and FRAP, whereas p-coumaric acid (9.4 µmol/L) and naringenin (26.0 µmol/L) showed the lowest values. However, the sum of the individual activities accounted for 24%, 50%, and 28% of the AC evaluated in the extract by ABTS, DPPH, and FRAP, respectively. This suggests that other minor compounds may have an important contribution to the AC or there are interactions between the compounds in the extract. The AC was also evaluated using equimolar concentrations (50 µmol/L) of each compound (). Under these conditions, the order of AC was rutin > naringenin > chlorogenic acid > p-coumaric acid for ABTS, p-coumaric acid > rutin > naringenin > chlorogenic acid for DPPH, and rutin > p-coumaric acid > chlorogenic acid > naringenin for FRAP.
Table 4. Antioxidant activity of phenolic standards evaluated at the concentration found in the methanol extract of Tumbisca and 50 µmol/L.
Tabla 4. Capacidad antioxidante de estándares de fenólicos evaluados a la concentración del extracto metanólico de Tumbisca y 50 µmol/L.
The interactions between the four phenolic compounds were evaluated at equimolar concentrations (50 µmol/L). In DPPH, all combinations showed synergistic effects while in ABTS about half of the combinations showed antagonistic effects and the rest showed synergistic and additive effects (). These results may be due to the fact that the radical-scavenging mechanism in the DPPH assay is closer to a hydrogen atom transfer mechanism, whereas the ABTS method is associated with multiple oxidation steps (Zhang et al., Citation2011). The AC by FRAP indicated antagonistic interactions for most of the combinations of the four compounds (), suggesting that the electron transfer scavenging mechanism of this assay favors the regeneration of weak antioxidants by more efficient compounds (Peyrat-Maillard et al., Citation2003).
5.4. Expression of MYB transcription factors and target genes
To investigate if the high levels of phenolics in the wild tomato accessions is associated with the up-regulation of the phenylpropanoids pathway, the expression of the transcription factors MYB12 and MYB14 and the target genes PAL, CHS and CHI was evaluated (). The expression of MYB12 varied significantly among the accessions and was higher than that of the Moneymaker variety; Kilim and Tumbisca showed the highest expression levels and corresponded with the highest phenolic and flavonoid content in these materials (). AtMYB12 is a key regulator of flavonoid biosynthesis in Arabidopsis thaliana and its expression in tomato increases significantly the content of phenylpropanoids such as chlorogenic acid and caffeoylquinic acids (Luo et al., Citation2008; Pandey et al., Citation2015). These results correspond with the correlation observed in the present study between the expression levels of MYB12 and the content of the main phenolic compounds: chlorogenic acid (r = 0.41; p ≤ 0.05) and dicaffeoylquinic acid I (r = 0.45; p ≤ 0.05). The expression of MYB12 also correlated with the AC by the three methods (r = 0.43–0.51; p ≤ 0.05) (Supplementary Table S2).
Figure 3. Relative expression of MYB transcription factors and target genes in the pericarp of Solanum lycopersicum var. cerasiforme accessions. The UBI3 gene was used as control and the expression values are relative to that of the commercial tomato Moneymaker. Bars indicate the LSD (α = 0.05). Means are significantly different when the bars do not horizontally overlap. MYB12 and MYB14: Transcription factors; PAL: Phenylalanine ammonia-lyase; CHS: Chalcone synthase; CHI: Chalcone isomerase.
Figura 3. Expresión relativa de factores de transcripción MYB y genes diana en el pericarpio de accesiones de Solanum lycopersicum var. cerasiforme. Se usó el gen UBI3 como control y los valores de expresión son relativos al de la variedad comercial de tomate Moneymaker. Las barras indican LSD (α = 0.05). Las medias son significativamente diferentes cuando las barras no se traslapan horizontalmente. MYB12 y MYB14: Factores de transcription; PAL: Fenilalanina amonio liasa; CHS: Chalcona sintasa; CHI: Chalcona isomerasa.
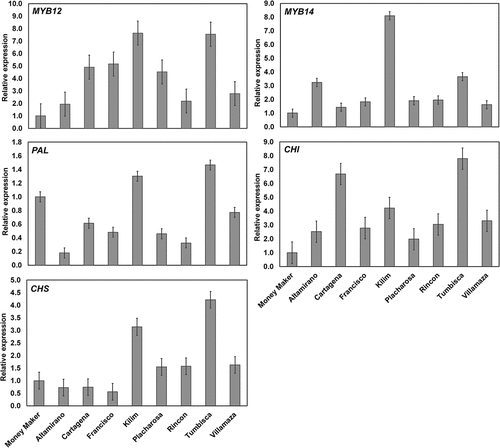
Wang et al. (Citation2018) overexpressed SlMYB12 in three cherry tomato varieties and observed significant increases in the content of flavonols and the expression of genes involved in their biosynthesis, suggesting the potential use of SlMYB12 as a marker for the accumulation of flavonoids in tomato.
MYB14 showed, in general, lower relative expression values than MYB12 in the accessions (). The highest transcript levels were observed in Kilim, followed by Tumbisca and Altamirano, which corresponded with the high content of the major phenolics observed in these accessions (). The expression levels of MYB14 correlated significantly with the content of chlorogenic acid (r = 0.73; p ≤ 0.001), rutin (r = 0.68; p ≤ 0.001) and dicaffeoylquinic acids (r = 0.58–0.65; p ≤ 0.01) and the AC (r = 0.44–0.49; p < 0.05) in the tomato accessions (Supplementary Table S2). Consistent with the possible role of MYB14 in the modulation of phenylpropanoids pathway, the overexpression of this gene in tomato plants increased the transcript levels of several genes associated with flavonoid biosynthesis (Li et al., Citation2021).
Phenylalanine ammonia-lyase (PAL), chalcone synthase (CHS), and chalcone isomerase (CHI) catalyze key steps in the phenylpropanoid pathway and the genes encoding these enzymes are targets of MYB transcription factors. The fruit-specific expression of AtMYB12 in tomato increased up to 18, 16 and 10 times the transcript levels of PAL, CHS and CHI, respectively (Ding et al., Citation2022; Zhang et al., Citation2015). In this regard, the expression of PAL varied significantly among the accessions () and correlated with the transcript levels of MYB12 (r = 0.69; p ≤ 0.001) and MYB14 (r = 0.59; p ≤ 0.01). The expression levels of PAL also correlated with the content of chlorogenic acid (r = 0.58; p ≤ 0.01), dicaffeoylquinic acids (r = 0.69–0.79; p ≤ 0.001), rutin (r = 0.54; p ≤ 0.01) and naringenin chalcone (0.84; p ≤ 0.001), and the AC by the three methods (r = 0.68–0.71; p ≤ 0.001) (Supplementary Table S2).
CHS is the first committed enzyme in flavonoid biosynthesis and its coding gene is activated by AtMYB12, which binds directly to the promoter region (Zhang et al., Citation2015). The expression of CHS in the accessions Kilim and Tumbisca was 3.1 and 4.2 times higher than that of Moneymaker (). The transcript levels of this gene correlated with those of MYB12 (r = 0.61; p ≤ 0.001) and MYB14 (r = 0.57; p ≤ 0.001). The expression of CHS also showed a highly significant correlation with the content of naringenin chalcone (r = 0.80; p ≤ 0.001) and the AC (r = 0.79–0.88; p ≤ 0.001) (Supplementary Table S2). These results agree with the key role of CHS in the synthesis of flavonoids.
CHI catalyzes the conversion of naringenin chalcone to naringenin and its expression appears to be modulated by MYB12 since the silencing of this transcription factor decreased the accumulation of naringenin chalcone in tomato fruit (Ballester et al., Citation2010). In this study, the expression of CHI varied significantly among the accessions () and correlated with the levels of MYB12 (r = 0.46; p ≤ 0.05) and the AC (r = 0.57–0.63; p ≤ 0.001) (Supplementary Table S2). The content of naringenin chalcone also correlated with the transcript levels of MYB12 (r = 0.71; p ≤ 0.001), MYB14 (r = 0.90; p ≤ 0.001) and CHI (r = 0.39; p ≤ 0.05), suggesting that higher expression levels of MYB12 and MYB14 could be increasing the content of this compound as an intermediate for the synthesis of flavonoids.
6. Conclusions
The methanol extracts from the pericarp of Solanum lycopersicum var. cerasiforme accessions showed high antioxidant capacity mainly associated with caffeoylquinic acids and rutin. The phenolic content and antioxidant capacity of the accessions showed positive correlation with the expression levels of the transcription factors MYB12 and MYB14 and their target genes PAL, CHS, and CHI. In this regard, the accessions Tumbisca and Kilim showed the highest levels of gene expression and phenolics content, suggesting they can be used in breeding programs to produce functional tomatoes with high levels of phenylpropanoids derivatives and antioxidant capacity.
Supplemental Table 3
Download MS Word (21.5 KB)Supplemental Table 2
Download MS Excel (19.9 KB)Supplemental Table 1
Download MS Word (19.4 KB)Acknowledgements
The authors thank Cruz F. López-Carrera and María F. Quintero-Soto for their technical assistance.
Disclosure statement
No potential conflict of interest was reported by the authors.
Supplementary material
Supplemental data for this article can be accessed online at https://doi.org/10.1080/19476337.2022.2144951
Additional information
Funding
References
- Adalid, A. M., Rosello, S., & Nuez, F. (2010). Evaluation and selection of tomato accessions (Solanum section Lycopersicon) for content of lycopene, beta-carotene and ascorbic acid. Journal of Food Composition and Analysis, 23(6), 613–618. https://doi.org/10.1016/j.jfca.2010.03.001
- Ballester, A.-R., Molthoff, J., de Vos, R., Te Lintel Hekkert, B., Orzaez, D., Fernández-Moreno, J.-P., Tripodi, P., Grandillo, S., Martin, C., & Heldens, J. (2010). Biochemical and molecular analysis of pink tomatoes: Deregulated expression of the gene encoding transcription factor Slmyb12 leads to pink tomato fruit color. Plant Physiology, 152(1), 71–84. https://doi.org/10.1104/pp.109.147322
- Benzie, I. F., & Strain, J. J. (1996). The ferric reducing ability of plasma (FRAP) as a measure of “antioxidant power”: The FRAP assay. Analytical Biochemistry, 239(1), 70–76. https://doi.org/10.1006/abio.1996.0292
- Boches, P., Peterschmidt, B., & Myers, J. R. (2011). Evaluation of a subset of the Solanum lycopersicum var. cerasiforme core collection for horticultural quality and fruit phenolic content. HortScience, 46(11), 1450–1455. https://doi.org/10.21273/HORTSCI.46.11.1450
- Brand-Williams, W., Cuvelier, M. E., & Berset, C. (1995). Use of a free radical method to evaluate antioxidant activity. LWT-Food Science and Technology, 28(1), 25–30. https://doi.org/10.1016/S0023-6438(95)80008-5
- Crisanto-Juárez, A. U., Vera-Guzmán, A. M., Chávez-Servia, J. L., & Carrillo-Rodríguez, J. C. (2010). Calidad de Frutos de Tomates Silvestres (Lycopersicon esculentum var. cerasiforme Dunal) de Oaxaca, México. Revista Fitotecnia Mexicana, 33(Especial_4), 7–13. https://doi.org/10.35196/rfm.2010.Especial_4.7
- Delgado-Vargas, F., Sicairos-Medina, L. Y., Luna-Mandujan, A. G., López-Angulo, G., Salazar-Salas, N. Y., Vega-García, M. O., Heredia, J. B., & López-Valenzuela, J. A. (2018). Phenolic profiles, antioxidant and antimutagenic activities of Solanum lycopersicum var. cerasiforme accessions from Mexico. CyTA - Journal of Food, 16(1), 715–722. https://doi.org/10.1080/19476337.2018.1481146
- Ding, X., Yin, Z., Wang, S., Liu, H., Chu, X., Liu, J., Zhao, H., Wang, X., Li, Y., & Ding, X. (2022). Different fruit-specific promoters drive AtMYB12 expression to improve phenylpropanoid accumulation in tomato. Molecules, 27(1), 317. https://doi.org/10.3390/molecules27010317
- Hanson, P. M., Yang, R. Y., Wu, J., Chen, J. T., Ledesma, D., Tsou, S. C. S., & Lee, T. C. (2004). Variation for antioxidant activity and antioxidants in tomato. Journal of the American Society for Horticultural Science, 129(5), 704–711. https://doi.org/10.21273/JASHS.129.5.0704
- Juárez-López, P., Castro-Brindis, R., Colinas-León, T., Ramírez-Vallejo, T., Sandoval-Villa, M., Reed, D. W., Cisneros-Cevallos, L., & King, S. (2009). Evaluation of quality in fruits of seven native tomato (Lycopersicum esculentum var cerasiforme) genotypes. Revista Chapingo Serie Horticultura, 15(4), 5–9. https://doi.org/10.5154/r.rchsh.2009.15.043
- Kaur, C., Walia, S., Nagal, S., Walia, S., Singh, J., Singh, B. B., Saha, S., Singh, B., Kalia, P., Jaggi, S., & Sarika, S. (2013). Functional quality and antioxidant composition of selected tomato (Solanum lycopersicum L) cultivars grown in Northern India. LWT - Food Science and Technology, 50(1), 139–145. https://doi.org/10.1016/j.lwt.2012.06.013
- Kavitha, P., Shivashankara, K. S., Rao, V. K., Sadashiva, A. T., Ravishankar, K. V., & Sathish, G. J. (2014). Genotypic variability for antioxidant and quality parameters among tomato cultivars, hybrids, cherry tomatoes and wild species. Journal of the Science of Food and Agriculture, 94(5), 993–999. https://doi.org/10.1002/jsfa.6359
- Kim, D.-O., Jeong, S. W., & Lee, C. Y. (2003). Antioxidant capacity of phenolic phytochemicals from various cultivars of plums. Food Chemistry, 81(3), 321–326. https://doi.org/10.1016/S0308-8146(02)00423-5
- Li, Y., Chen, M., Wang, S., Ning, J., Ding, X., & Chu, Z. (2015). AtMYB11 regulates caffeoylquinic acid and flavonol synthesis in tomato and tobacco. Plant Cell, Tissue and Organ Culture, 122(2), 309–319. https://doi.org/10.1007/s11240-015-0767-6
- Li, Z., Peng, R., & Yao, Q. (2021). Slmyb14 promotes flavonoids accumulation and confers higher tolerance to 2, 4, 6-trichlorophenol in tomato. Plant Science, 303, 110796. https://doi.org/10.1016/j.plantsci.2020.110796
- Luo, J., Butelli, E., Hill, L., Parr, A., Niggeweg, R., Bailey, P., Weisshaar, B., & Martin, C. (2008). AtMYB12 regulates caffeoyl quinic acid and flavonol synthesis in tomato: Expression in fruit results in very high levels of both types of polyphenol. The Plant Journal, 56(2), 316–326. https://doi.org/10.1111/j.1365-313X.2008.03597.x
- Pandey, A., Misra, P., Choudhary, D., Yadav, R., Goel, R., Bhambhani, S., Sanyal, I., Trivedi, R., & Trivedi, P. K. (2015). AtMYB12 expression in tomato leads to large scale differential modulation in transcriptome and flavonoid content in leaf and fruit tissues. Scientific Reports, 5(1), 12412. https://doi.org/10.1038/srep12412
- Peyrat-Maillard, M. N., Cuvelier, M. E., & Berset, C. (2003). Antioxidant activity of phenolic compounds in 2,2 ’-azobis (2-amidinopropane) dihydrochloride (AAPH)-induced oxidation: Synergistic and antagonistic effects. Journal of the American Oil Chemists Society, 80(10), 1007–1012. https://doi.org/10.1007/s11746-003-0812-z
- Re, R., Pellegrini, N., Proteggente, A., Pannala, A., Yang, M., & Rice-Evans, C. (1999). Antioxidant activity applying an improved ABTS radical cation decolorization assay. Free Radical Biology & Medicine, 26(9–10), 1231–1237. https://doi.org/10.1016/S0891-5849(98)00315-3
- Wang, S., Chu, Z., Jia, R., Dan, F., Shen, X., Li, Y., & Ding, X. (2018). SlMYB12 regulates flavonol synthesis in three different cherry tomato varieties. Scientific Reports, 8(1), 1582. https://doi.org/10.1038/s41598-018-19214-3
- Waterhouse, A. L. (2002). Determination of total phenolics. In R. E. Wrolstad (Ed.), Current protocols in food analytical chemistry (pp. I1.1.1–1.1.8). John Wiley & Sons, Inc.
- Zhang, Y., Butelli, E., Alseekh, S., Tohge, T., Rallapalli, G., Luo, J., Kawar, P. G., Hill, L., Santino, A., Fernie, A. R., & Martin, C. (2015). Multi-level engineering facilitates the production of phenylpropanoid compounds in tomato. Nature Communications, 6(1), 8635. https://doi.org/10.1038/ncomms9635
- Zhang, D., Chu, L., Liu, Y., Wang, A., Ji, B., Wu, W., Zhou, F., Wei, Y., Cheng, Q., Cai, S., Xie, L., & Jia, G. (2011). Analysis of the antioxidant capacities of flavonoids under different spectrophotometric assays using cyclic voltammetry and density functional theory. Journal of Agricultural and Food Chemistry, 59(18), 10277–10285. https://doi.org/10.1021/jf201773q