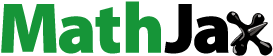
ABSTRACT
There is a constant search for novel and inexpensive materials for the encapsulation of cells. The present study aimed to evaluate the efficiency of mucilage from young cladodes of Opuntia robusta in the encapsulation of Lactiplantibacillus plantarum 1449. The mucilage was mixed with alginate at ratios of 2:1, 1:1, and 1:2 (alginate:mucilage, AM). The mixtures were used to encapsulate L. plantarum 1449. The microcapsules were characterized by size, weight, shape, color, and stability. The results showed that increased amounts of mucilage in the mixtures influenced the stability of the microcapsules, leading to an increased amount of irregular shapes and greater variability in size and weight. The AM 1:1 showed good encapsulation efficiency (96.1 ± 0.5%) and the viability of L. plantarum 1449 after three months of storage at 4°C was 77.2 ± 2.4%. In conclusion, mucilage combined with alginate has great potential as an encapsulating agent.
Introduction
Opuntia species are endemic to Mexico. Some of them are of great interest due to their biotechnological potential. Opuntia spp. is considered an important nutritional source in several countries (Shapiro & Gong, Citation2002). Despite the existence of many species in Mexico and other countries, the most widely studied is Opuntia ficus indica, a domesticated plant cultivated for commercial purposes that can grow under conditions of scarce resources. Since ancient times, the fruits and cladodes of this plant species have been consumed by humans. The cladodes, known as “nopalitos”, are used in folk medicine due to their high content of bioactive compounds which have health promoting properties (Silva et al., Citation2021). Wild Opuntia species such as Opuntia robusta is endemic to northern Mexico and are consumed locally by people. They are consumed alone or combined with other wild Opuntia spp. but only as young cladodes, which is why their consumption occurs only once a year. The main component of the cladodes is mucilage, which is a water-soluble heteropolysaccharide, negatively charged and with high molecular weight. Its heteropolysaccharide chains repel among themselves, which causes them to stretch throughout a solute, increasing the viscosity of the mixture (Trachtenberg & Mayer, Citation1980). Mucilage has applications in the food and pharmaceutical industries due to its properties as thickener, emulsifier, and gelling agent (Malviya, Citation2011). Some of the functional properties of Opuntia spp. such as antioxidative, cholesterol-lowering, antiulcer and anti-inflammatory activities, have been attributed to mucilage (Galati et al., Citation2001; Y. Cárdenas et al., Citation2019). Other applications of mucilage include the production of biopolymers for packaging purposes as edible films (Espino-Díaz et al., Citation2010), and serving as an encapsulating agent for active ingredients (Otálora et al., Citation2015). Currently, mucilage has not been evaluated in the encapsulation of bacteria. Encapsulation is a good alternative for protecting bacteria and maintaining their viability for a long time, because it protects microbial cells from adverse environmental conditions by entrapping them within a matrix of biopolymeric material (Liu et al., Citation2019; Tiani et al., Citation2018). Several encapsulating agents based on proteins or carbohydrates have been investigated for microencapsulation. The main carbohydrate-based materials used for encapsulation are alginate, chitosan, carrageenan, arabic gum, pectins, starch, pullulan, ethylcellulose and maltodextrins (Kowalska et al., Citation2022). Sodium alginate has been one of the most used materials for this purpose because it has the advantage of being compatible with other materials and is non-toxic. The encapsulating process using sodium alginate is also relatively simple (Etchepare et al., Citation2015; Krasaekoopt et al., Citation2003). Alginate has been used in combination with mucilage from mutamba and psyllium seeds, as well as with gums, for the encapsulation of Lactobacillus reuteri, increasing its viability and resistance and allowing it to produce reuterin (Rodrigues et al., Citation2022). The most widely used techniques for the encapsulation of microbial cells are extrusion, emulsion, and spray drying. The extrusion method is based on mixing the bacteria cells with the polymeric solution, followed by extruding a crosslinking solution such as calcium chloride through a syringe needle or nozzle (Lee et al., Citation2019). The size of the microcapsules is influenced by different factors, including nozzle size, the viscosity of the polymeric solution, and the distance between the syringe needle and the surface of the crosslinking solution (Rodrigues et al., Citation2020). The L. plantarum 1449 strain was isolated in our laboratory and characterized as a potential probiotic. It was shown to possess good resistance to simulated conditions of the gastrointestinal tract. It was also able to lower the cholesterol concentration in vitro. Another interesting property of this strain is its potential to synthesize silver nanoparticles in Man Rogosa and Sharpe medium supplemented with silver nitrate (Reyes-Escogido et al., Citation2017). Therefore, when used to encapsulate cells, it should be able to maintain their viability by protecting them from adverse factors, allowing them to show their probiotic potential. This strain has been used for the production of silver nanoparticles. However, the purification of the nanoparticles is problematic due to the presence of cell debris during the purification process. The use of encapsulated cells could make the purification of the nanoparticles more efficient. Since there is no information about the use of wild cactus mucilage as an encapsulating agent for bacterial cells, in the present study we evaluated the encapsulating potential of mucilage obtained from young O. robusta cladodes. We encapsulated L. plantarum 1449 cells, a bacterial strain of great interest.
Materials and methods
Microbial strain and chemicals
L. plantarum 1449 was obtained from the Culture Collection of the Laboratory of Metabolism Research of the University of Guanajuato, Mexico. Culture medium Man Rogosa and Sharpe (MRS) broth and agar (Difco BD, U.S.A), ethanol 95%, sodium alginate, calcium chloride, and sodium citrate, were all purchased from Sigma-Aldrich (St. Louis, MO, U.S.A).
Vegetal material
Young cladodes of wild O. robusta (2–3 weeks old) were collected from a locality in the state of Zacatecas, Mexico (22° 13´ 35.76''N and 101° 44´ 19.85'' W). After removing the thorns, the cladodes were washed with water to eliminate residual thorns and other impurities. The excess of water in the cladodes was removed using absorbent paper. The moisture content of the cladodes was determined after drying at 105°C for 6 h to constant weight.
Mucilage extraction
Mucilage was extracted from the cladodes following the method from Dick et al. (Citation2019). Briefly, nopal cladodes were cut into slices of approximately 1x1 cm2. The slices were weighed and put into a container. Distilled water was added in a ratio of 1:2 w/v (nopal:water). The dispersion was kept in agitation at 80°C for 2 h. After cooling to room temperature, the fibrous residues were eliminated by decantation and the aqueous phase was filtered through a fine cloth, followed by centrifugation at 3200 g for 20 min and 20°C. The mucilage was recovered from the supernatant by precipitating with 3 volumes of ethanol 95% and it was kept overnight at 4°C. The mucilage was dried at 45°C in a convection heat oven for 6 h. The dry mucilage was weighed, and the percentage yield of mucilage was calculated and reported as the dry basis. The mucilage was ground with a mortar and pestle and sieved through a 50-mesh to obtain mucilage powder. The powder was then stored in a closed container for future analysis.
Scanning electron microscopy and energy dispersive X-ray spectroscopy of the mucilage extracted from O. robusta
Morphological analysis of mucilage powder was performed at magnifications of 250x, 1000x and 5000x using a JSM-7600F field emission scanning electron microscope (JEOL). The determination of the relative content of minerals was performed using an EDS system combined with SEM.
Preparation of encapsulating agents
Four suspensions of encapsulating agents were prepared; alginate (Alg), and three mixtures of alginate-mucilage (AM) at ratios 2:1 (AM 2:1), 1:1 (AM 1:1), and 1:2 (AM 1:2). Alginate and AM mixtures were dissolved in distilled water by stirring. Afterward, the suspensions were kept all night at 4°C for their complete hydration. The pH was adjusted to 7.3 ± 0.2 and sterilized at 121°C for 15 min.
Preparation of L. plantarum 1449 cells
The L. plantarum 1449 strain was activated in de Man Rogosa and Sharpe (MRS) broth at 37°C for 24 h in an anaerobic jar and then reactivated for 24 h. The L. plantarum 1449 culture was centrifuged at 2600 g for 10 min. The pellet was washed twice with sterile saline solution (0.85% [w/v] NaCl). The pelleted cells were resuspended in the saline solution and cellular concentration was adjusted to obtain a final turbidity equivalent to McFarland Standard 4 (1.2 x 109 CFU/mL). The cell suspension was subsequently used for encapsulation. The viability of L. plantarum in the suspension was evaluated by serial dilution and plating on MRS agar, and incubated at 37°C for 48 h. The result was reported as CFU/mL.
Microencapsulation by extrusion
The microencapsulation process was performed using the extrusion technique. The previously prepared cellular suspension (1.2 x 109 CFU/mL) was added to each of the freshly prepared encapsulating agents (Alg or AM) at a final concentration of 108 CFU/mL. The suspensions were homogenized carefully by stirring at 200 rpm for 20 min. Each suspension was extruded through a 21-gauge nozzle into a sterile, magnetically stirring solution of CaCl2 (0.15 M). The distance between the nozzle and the surface of the CaCl2 solution was 10 cm. After extrusion, stirring continued for 20 min to complete the formation of the beads. The beads were recovered by filtration using sterile Whatman filter paper No. 54. Non-encapsulated cells were determined in the supernatant by plating as previously described. The beads were washed twice with sterile saline solution to eliminate residual CaCl2, then transferred to a sterile tube and stored at 4°C for further analysis.
Characterization of microcapsules (size, weight and color)
Fifty microcapsules were measured and weighed using a calibrated vernier and an analytical balance. The size was reported in mm and the weight in mg.
The color parameters (CIELab) of the microcapsules obtained from the mucilage-alginate mixture, and their comparison with alginate beads, were determined using a Chroma Meter (Konica Minolta, Co. Osaka, Japan). The ΔE* values were obtained by comparing data from AM mixtures with Alg alone. All results were reported as mean and standard deviation (±SD).
Encapsulation efficiency (EE) and viability
To count the number of encapsulated cells, one gram of the microcapsules was dissolved in 9 ml of sterile 2% (w/v) sodium citrate under horizontal shaking at 150 rpm until the dissolution of the microcapsules was completed. Serial dilutions were made and plated by spreading 0.1 mL on MRS agar. The plates were incubated at 37°C for 48 h. The microcapsules were stored at 4°C for three months. After this time, the count of viable cells was done as described above.
The number of viable cells was calculated using the equation:
Encapsulation efficiency was calculated using the following equation (Fareez et al., Citation2015):
Where N is the number of viable bacteria in CFU/g of microcapsules and N0 is the number of free viable bacteria in CFU/mL added to the encapsulating agent during the preparation of microcapsules.
Statistical analysis
All tests were performed in triplicate. Results were expressed as mean ± Standard Deviation (±SD). Statistical analyses were performed with the SPSS 26.0 software (IBM SPSS Statistics for Windows version 26.0. Armonk, NY, IBM Corp.). An analysis of variance was performed to identify differences between means. Differences were considered significant at p < .05.
Results and discussion
Yield of mucilage
The moisture content of young cladodes of O. robusta was 92.3% of their total weight. In general, Opuntia species have high moisture content, with reported values in the range of 90% to 95% (Mendez et al., Citation2015; Rocchetti et al., Citation2018). The results of the present study are consistent with those results reported before. The yield of recovered mucilage powder was 0.41%, based on fresh weight (FW). The dry weight (DW) yield was 5.86%. There are no previous reports of the content of mucilage in O. robusta, but the yield obtained in the present study was similar to the reported yield for other cacti species such as O. dillenii (6.2%) (Kalegowda et al., Citation2017) and O. ficus indica under different conditions (4.23–8.95%) (Felkai-Haddache et al., Citation2016), and lower than the yield reported for O. monacantha (12%) (Dick et al., Citation2019). The variability in yield results is explained by the differences in the method used for mucilage extraction, weather at harvest time, species, age of the cladode, and soil type (Sáenz et al., Citation2004). The mucilage yield values reported for different species have been obtained from cladodes in an age range of 1 to 3 months (Contreras-Padilla et al., Citation2016), while the cladodes used in this work were in a range of 2 to 3 weeks old. The difference in the results was expected because mucilage content in nopal increases with the edge.
Scanning electron microscopy and energy dispersive X-ray spectroscopy of mucilage
The image analysis of mucilage at magnifications of 250x, 1000x and 5000x reveals particles of irregular shape and different sizes. As can be seen in , an SEM micrograph of the mucilage powder shows a high level of porosity, similar to a sponge. This aspect was described as typical of hygroscopic material (Conceição et al., Citation2014). The mineral composition of mucilage powder from O. robusta was determined using energy dispersive X-rays spectroscopy (EDX). As can be appreciated in , a high content of carbon and oxygen indicates a mainly organic composition. Potassium was the most abundant mineral in the mucilage, followed by sulfur, magnesium, and chlorine. Surprisingly, no calcium was identified, in contrast with previous reports where calcium was the main mineral component of mucilage from O. robusta (du Toit et al., Citation2018), O. ficus indica (Procacci et al., Citation2021) and O. monocantha (Dick et al., Citation2019). Other minerals such as manganese and phosphorus (0.14%) were also identified in the present study. Previously, it was reported that the maturity stage of cacti species is related to the calcium content, which means that older cladodes have more calcium than younger ones. The values reported values in other studies correspond to cladodes between 36 and 60 days old, while in the values found in the present work correspond to cladodes between 2 and 3 weeks old. The pH measurements revealed that at a concentration of 1% (w/v), mucilage is slightly acidic (5.09 ± 0.03), with similar values to those reported for O. cochenillifera (4.8–5.0) (Monrroy et al., Citation2017) and lower than the pH values reported for O. dilenii (7.10 ± 0.06) (Kalegowda et al., Citation2017), O. ficus indica (6.43 ± 0.06) and O. streptacantha (6.93 ± 0.06) (Gebresamuel & Gebre-Mariam, Citation2012). All these pH values were determined in mucilage solutions with the same concentrations used in the present study. The acidic pH indicates the presence of uronic acids in the structure of mucilage (Gebresamuel & Gebre-Mariam, Citation2012). Conductivity was 1.11 ± 0.01 mS/cm, lower than the values reported for O. cochenillifera (2.3) (Monrroy et al., Citation2017), O. ficus indica (2.73 ± 0.11) and O. streptacantha (2.02 ± 0.01) (Gebresamuel & Gebre-Mariam, Citation2012). The conductivity value found in the present study indicates the presence of a high number of divalent and monovalent ions. The low conductivity compared to other mucilages is due to the absence of Ca2+. Both pH and conductivity influence the viscosity and gelation properties of mucilage (Trachtenberg & Mayer, Citation1980), which are important for the encapsulation process.
Figure 1. Scanning electron micrographs (SEM) of mucilage from wild Opuntia robusta at 250x (a), 1000x (b) and 5000x (c).
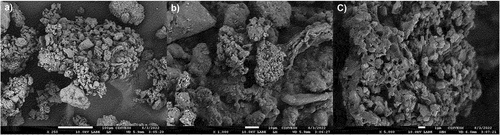
Table 1. Mineral composition of mucilage powder determined by Energy Dispersive X-rays Spectroscopy. Data are presented as mean (n = 3) ± SD.
Opuntia robusta mucilage as encapsulating agent
There is a growing interest in the development of encapsulating agents based on natural polymers. Mucilage is a natural polymer that is biodegradable, biocompatible, water-soluble, widely available, and low-cost. In addition, it can be used as a biofilm for food packaging and other applications (Gheribi & Khwaldia, Citation2019). Regarding the functionality of mucilage for the encapsulation of L. plantarum 1449, mucilage alone cannot be used as encapsulating agent since it cannot form stable capsules. However, when mucilage is mixed with alginate the microcapsules acquire stability, which is associated with the alginate-mucilage ratio. It has been reported that the age of cladodes is an important factor that influences the rheological properties of mucilage in solution. At lower concentrations of mucilage, the viscoelastic behavior of the solution is enhanced and it shows higher viscosity and elasticity, with a gel-like behavior (Rodríguez-González et al., Citation2021). A solution of mucilage (1% w/v) has a conductivity of 1.11 mS/cm, which indicates the presence of ions. Ions have a high influence on the rheological properties of mucilage. Viscosity is one of the properties affected by them. It has been reported that the presence of ions decrease viscosity (Majdoub et al., Citation2001). Ions such as Ca2+ influence the viscoelastic and gelation properties of mucilage, as well as other factors, including pH, temperature, sugar content and pectin content (A. Cárdenas et al., Citation2008). Although the present work did not identify the presence of calcium in mucilage, other factors influenced its properties. According to previous reports, the content of minerals and other components is associated with the age of cladodes. Since the cladodes under study were very young, they contained few minerals, and calcium is one of the minerals that increases with age.
According to the EDS analysis, the composition of minerals in the mucilage of O. robusta was K, Mg, Cl, and S. All these minerals can be present as ions. As has been mentioned, minerals greatly influence the behavior of mucilage, while the shape, size, color, and stability of microcapsules are influenced by the amount of mucilage in the mixture. As can be seen in , the shape of alginate microcapsules was spherical while AM mixtures in ratios of 2:1 and 1:1 produced some semispherical shapes. The microcapsules obtained with AM ratio of 1:2 showed great variability in shape (irregular shapes), weight and size. It was difficult to separate the microcapsules to carry out a characterization, which is why the data are not shown. There were no significance differences (p > .05) in size and weight between microcapsules made from Alg AM 2:1 and AM 1:1 (). As mentioned before, a high concentration of ions has a large influence on the properties of mucilage. A higher amount of mucilage implies a higher concentration of ions and greater instability of the solution. According to the evidence, mucilage alone has no gelling capacity, so it is necessary to add alginate or other gelling agent to form capsules.
Encapsulation efficiency and viability
The encapsulation efficiency (EE) of each mixture was determined. shows that the mixture of AM 1:1 was the best encapsulating agent (p < .05) being this the best option. Although there were no significative differences in EE between Alg, AM 2:1 and AM 1:2, the microcapsules obtained with AM 1:2 had many irregular shapes, a watery appearance and they began to disintegrate, which made them unstable. This was expected since, as mentioned before, the stability of microcapsules is influenced by the presence of ions and the amount of mucilage (A. Cárdenas et al., Citation2008; Majdoub et al., Citation2001). After three months stored at 4°C, the alginate, AM 2:1 and AM 1:1 microcapsules were stable. As can be observed in , the viability values obtained with the mixtures were better than with alginate alone, which suggests that mucilage may even have stimulated the growth of L. plantarum 1449. It has been previously reported that using mucilage from linseed and Botryosphaeria for encapsulation increases the viability of bacterial cells and the presence of natural polymers such as fructooligosaccharides in the encapsulating matrix, also increases the viability of the cells (Rodrigues et al., Citation2017). The use of prebiotics in the encapsulating matrix is a good strategy to maintain the viability of the cells for a prolonged time (Krasaekoopt & Watcharapoka, Citation2014). As have been reported, the mucilage of nopal has prebiotic properties (Guevara-Arauza et al., Citation2012), thus, the high survival rate shown by L. plantarum 1449 in the AM mixtures could be due to the mucilage.
Table 2. Encapsulation efficiency and viability after three months of L. plantarum 1449 in Alg and AM mixtures.
Color parameters
The concentration of mucilage in the mixtures influenced the color parameters (L*, a*, b* and ΔE*) of the AM mixtures (). The luminosity values (L*) decreased significantly (p < .05) in the mixtures of AM 1:1 and AM 1:2, compared with Alg alone. The mixture with the highest content of alginate (AM 2:1) showed an L* value similar to that of alginate alone. When the alginate-mucilage ratio decreased, luminosity also decreased. This was associated with the yellow color taken by the mixtures in which the weight ratio favored mucilage over alginate. This result is consistent with a previous study that found that the ratio of the mixture components influenced the color parameters (Otálora et al., Citation2022). The chromaticity was in the yellow-reddish spectrum for all mixtures of AM regardless of the alginate-mucilage ratio. The lowest value of a* was obtained with AM 2:1 (p < .05), although all samples showed low a* values, close to neutral, with no reddish influence on the final color. Regarding the b* values, they increased significantly (p < .05) in the three mixtures. The increases were related to the amount of mucilage, which gave a yellow color to the microcapsules, as can be observed in . The ΔE* values showed that the greatest differences in total color were between Alg and AM 1:2, according to the amount of mucilage present in the mixtures. As reported by a previous study, the nature and concentration of the materials played an important role in the color parameters of the microcapsules (Osorio et al., Citation2011). The ΔE* value of the microcapsules made from AM 2:1 means that adding mucilage in this ratio does not influence their color, since the difference is not perceptible by the human eye, in contrast with the AM 1:1 and AM 1:2 mixtures, in which the yellow color is easily distinguishable. The color of microcapsules could be an important factor in the acceptability of a product.
Figure 4. Color parameters: (a) L*, (b) a* and (c) b* of the microcapsules made from Alg and AM 2:1, AM 1:1 and AM 1:2 mixtures. L*, a*, and b* data are the mean of measured values (n = 5) ± SD. Different letters for each color parameter indicate statistical differences (p < .05).
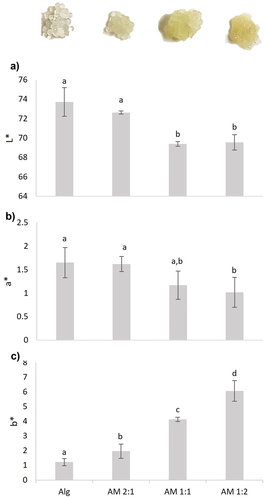
Table 3. Color parameters (L*, a*, b*and ΔE*) of the obtained microcapsules. The data are the mean of measured values (n = 5) ± SD.
Conclusions
The mucilage from Opuntia spp. is an inexpensive material with prebiotic and viscoelastic properties that give a wide range of applications in the pharmaceutical and food industries. Its usefulness as an edible film and encapsulating agent is of great interest, although it has been used mainly to encapsulate metabolites. The present study showed the possibility of encapsulating bacterial cells using mucilage extracted from young cladodes of O. robusta, a non-commercial specie that is nevertheless consumed by humans. Although mucilage alone is not useful for encapsulation, it can be used for this purpose when combined with alginate. The alginate-mucilage ratio is important for the stability of the microcapsules. When the amount of mucilage is greater than that of mucilage, the microcapsules lose stability, so it is important to maintain at least a 1:1 ratio (alginate-mucilage). There are not previous encapsulation studies using mucilage from young wild nopal cactuses. The results of the present study are a first approximation to the study of its use as an encapsulating agent in addition to its role as a prebiotic. Based on these results, we propose to use encapsulated cells as immobilized cells to produce metabolites of industrial interest. The stability of microcapsules in simulated gastrointestinal conditions must be evaluated to further assess the use of L. plantarum as probiotic.
Acknowledgements
DBV and LGZM thank the University of Guanajuato for the 27th edition of the science summer program.
Disclosure statement
No potential conflict of interest was reported by the author(s).
References
- Cárdenas, A., Goycoolea, F. M., & Rinaudo, M. (2008). On the gelling behaviour of ‘nopal’ (Opuntia ficus indica) low methoxyl pectin. Carbohydrate Polymers, 73(2), 212–222. https://doi.org/10.1016/j.carbpol.2007.11.017
- Cárdenas, Y., Ríos-Silva, M., Huerta, M., López, M., Bricio-Barrios, J., Ortiz-Mesina, M., Urzúa, Z., Saavedra-Molina, A., & Trujillo, X. (2019). The comparative effect of Nopal and mucilage in metabolic parameters in rats with a high-fructose diet. Journal of Medicine Food, 22(5), 538–541. https://doi.org/10.1089/jmf.2018.0124
- Conceição, M. C., Junqueira, L. A., Guedes Silva, K. C., Prado, M. E. T., & de Resende, J. V. (2014). Thermal and microstructural stability of a powdered gum derived from Pereskia aculeata Miller leaves. Food Hydrocolloids, 40, 104–114. https://doi.org/10.1016/j.foodhyd.2014.02.015
- Contreras-Padilla, M., Rodríguez-García, M. E., Gutiérrez-Cortez, E., Valderrama-Bravo, M. D. C., Rojas-Molina, J. I., & Rivera-Muñoz, E. M. (2016). Physicochemical and rheological characterization of Opuntia ficus mucilage at three different maturity stages of cladode. European Polymer Journal, 78, 226–234. https://doi.org/10.1016/j.eurpolymj.2016.03.024
- Dick, M., Dal Magro, L., Rodrigues, R. C., Rios, A. D. O., & Flôres, S. H. (2019). Valorization of Opuntia monacantha (Willd.) Haw. cladodes to obtain a mucilage with hydrocolloid features: Physicochemical and functional performance. International Journal of Biological Macromolecules, 123, 900–909. https://doi.org/10.1016/j.ijbiomac.2018.11.126
- du Toit, A., de Wit, M., & Hugo, A. (2018). Cultivar and harvest month influence the nutrient content of Opuntia spp. cactus pear cladode mucilage extracts. Molecules, 23(4). https://doi.org/10.3390/molecules23040916
- Espino-Díaz, M., de Jesús Ornelas-Paz, J., Martínez-Téllez, M. A., Santillán, C., Barbosa-Cánovas, G. V., Zamudio-Flores, P. B., & Olivas, G. I. (2010). Development and characterization of edible films based on mucilage of Opuntia ficus-indica (L.). Journal of Food Science, 75(6), E347–352. https://doi.org/10.1111/j.1750-3841.2010.01661.x
- Etchepare, M. D. A., Barin, J. S., Cichoski, A. J., Jacob-Lopes, E., Wagner, R., Fries, L. L. M., & Menezes, C. R. D. (2015). Microencapsulation of probiotics using sodium alginate. Ciência Rural, 45(7), 1319–1326. https://doi.org/10.1590/0103-8478cr20140938
- Fareez, I. M., Lim, S. M., Mishra, R. K., & Ramasamy, K. (2015). Chitosan coated alginate-xanthan gum bead enhanced pH and thermotolerance of Lactobacillus plantarum LAB12. International Journal of Biological Macromolecules, 72, 1419–1428. https://doi.org/10.1016/j.ijbiomac.2014.10.054
- Felkai-Haddache, L., Remini, H., Dulong, V., Mamou-Belhabib, K., Picton, L., Madani, K., & Rihouey, C. (2016). Conventional and microwave-assisted extraction of mucilage from Opuntia ficus-indica Cladodes: Physico-chemical and rheological properties. Food and Bioprocess Technology, 9(3), 481–492. https://doi.org/10.1007/s11947-015-1640-7
- Galati, E. M., Monforte, M. T., Tripodo, M. M., D’Aquino, A., & Mondello, M. R. (2001). Antiulcer activity of Opuntia ficus indica (L.) Mill. (Cactaceae): Ultrastructural study. Journal of Ethnopharmacology, 76(1), 1–9. https://doi.org/10.1016/s0378-8741(01)00196-9
- Gebresamuel, N., & Gebre-Mariam, T. (2012). Comparative physico-chemical characterization of the mucilages of two cactus pears (Opuntia spp.) obtained from Mekelle, Northern Ethiopia. Journal of Biomaterials and Nanobiotechnology, 03(01), 8. Article 17004. https://doi.org/10.4236/jbnb.2012.31010.
- Gheribi, R., & Khwaldia, K. (2019). Cactus mucilage for food packaging applications. Coatings, 9(10), 655. https://doi.org/10.3390/coatings9100655
- Guevara-Arauza, J. C., de Jesús Ornelas-Paz, J., Pimentel-González, D. J., Rosales Mendoza, S., Soria Guerra, R. E., & Paz Maldonado, L. M. T. (2012). Prebiotic effect of mucilage and pectic-derived oligosaccharides from nopal (Opuntia ficus-indica). Food Science and Biotechnology, 21(4), 997–1003. https://doi.org/10.1007/s10068-012-0130-1
- Kalegowda, P., Chauhan, A. S., & Nanjaraj Urs, S. M. (2017). Opuntia dillenii (Ker-Gawl) Haw cladode mucilage: Physico-chemical, rheological and functional behavior. Carbohydrate Polymers, 157, 1057–1064. https://doi.org/10.1016/j.carbpol.2016.10.070
- Kowalska, E., Ziarno, M., Ekielski, A., & Żelaziński, T. (2022). Materials used for the microencapsulation of probiotic bacteria in the food industry. Molecules, 27(10), 3321. https://doi.org/10.3390/molecules27103321
- Krasaekoopt, W., Bhandari, B., & Deeth, H. (2003). Evaluation of encapsulation techniques of probiotics for yoghurt. International Dairy Journal, 13(1), 3–13. https://doi.org/10.1016/S0958-6946(02)00155-3
- Krasaekoopt, W., & Watcharapoka, S. (2014). Effect of addition of inulin and galactooligosaccharide on the survival of microencapsulated probiotics in alginate beads coated with chitosan in simulated digestive system, yogurt and fruit juice. LWT - Food Science and Technology, 57(2), 761–766. https://doi.org/10.1016/j.lwt.2014.01.037
- Lee, Y., Ji, Y. R., Lee, S., Choi, M. J., & Cho, Y. (2019). Microencapsulation of probiotic Lactobacillus acidophilus KBL409 by extrusion technology to Enhance Survival under Simulated Intestinal and Freeze-Drying Conditions. Journal of Microbiology and Biotechnology, 29(5), 721–730. https://doi.org/10.4014/jmb.1903.03018
- Liu, H., Cui, S. W., Chen, M., Li, Y., Liang, R., Xu, F., & Zhong, F. (2019). Protective approaches and mechanisms of microencapsulation to the survival of probiotic bacteria during processing, storage and gastrointestinal digestion: A review. Critical Reviews in Food Science and Nutrition, 59(17), 2863–2878. https://doi.org/10.1080/10408398.2017.1377684
- Majdoub, H., Roudesli, S., Picton, L., Le Cerf, D., Muller, G., & Grisel, M. (2001). Prickly pear nopals pectin from Opuntia ficus-indica physico-chemical study in dilute and semi-dilute solutions. Carbohydrate polymers, 46(1), 69–79. https://doi.org/10.1016/S0144-8617(00)00284-8
- Malviya, R. (2011). Extraction characterization and evaluation of selected mucilage as pharmaceutical excipient. Polimery w medycynie, 41(3), 39–44.
- Mendez, L. P., Flores, F. T., Martin, J. D., Rodriguez Rodriguez, E. M., & Diaz Romero, C. (2015). Physicochemical characterization of cactus pads from Opuntia dillenii and Opuntia ficus indica. Food Chemistry, 188, 393–398. https://doi.org/10.1016/j.foodchem.2015.05.011
- Monrroy, M., García, E., Ríos, K., & García, J. R. (2017). Extraction and physicochemical characterization of mucilage from Opuntia cochenillifera (L.) Miller. Journal of Chemistry, 2017, 4301901. https://doi.org/10.1155/2017/4301901
- Osorio, C., Forero, D. P., & Carriazo, J. G. (2011). Characterisation and performance assessment of guava (Psidium guajava L.) microencapsulates obtained by spray-drying. Food Research International, 44(5), 1174–1181. https://doi.org/10.1016/j.foodres.2010.09.007
- Otálora, M. C., Carriazo, J. G., Iturriaga, L., Nazareno, M. A., & Osorio, C. (2015). Microencapsulation of betalains obtained from cactus fruit (Opuntia ficus-indica) by spray drying using cactus cladode mucilage and maltodextrin as encapsulating agents. Food Chemistry, 187, 174–181. https://doi.org/10.1016/j.foodchem.2015.04.090
- Otálora, M. C., Wilches-Torres, A., & Gómez Castaño, J. A. (2022). Spray-drying microencapsulation of pink guava (Psidium guajava) carotenoids using mucilage from Opuntia ficus-indica cladodes and aloe vera leaves as encapsulating materials. Polymers, 14(2), 310. https://doi.org/10.3390/polym14020310
- Procacci, S., Bojorquez Quintal, E., Platamone, G., Maccioni, O., Lo Vecchio, G., Morreale, V., Alisi, C., Balducchi, R., & Bacchetta, L. (2021). Opuntia ficus-indica pruning waste recycling: recovery and characterization of mucilage from cladodes. Natural Resources, 12(04), 91–107. https://doi.org/10.4236/nr.2021.124008
- Reyes-Escogido, M. L., Meneses-Rodríguez, D., & Guardado-Mendoza, R. (2017). Carbohydrate source affects the synthesis of silver nanoparticles by Lactobacillus plantarum 1449 and Lactobacillus ruminis 1313. IET Nanobiotechnology, 11(8), 1035–1039. https://doi.org/10.1049/iet-nbt.2017.0107
- Rocchetti, G., Pellizzoni, M., Montesano, D., & Lucini, L. (2018). Italian Opuntia ficus-indica cladodes as rich source of bioactive compounds with health-promoting properties. Foods, 7(2). https://doi.org/10.3390/foods7020024
- Rodrigues, F. J., Cedran, M. F., Bicas, J. L., & Sato, H. H. (2020). Encapsulated probiotic cells: Relevant techniques, natural sources as encapsulating materials and food applications – A narrative review. Food Research International, 137, 109682. https://doi.org/10.1016/j.foodres.2020.109682
- Rodrigues, F. J., Cedran, M. F., Pereira, G. A., Bicas, J. L., & Sato, H. H. (2022). Effective encapsulation of reuterin-producing Limosilactobacillus reuteri in alginate beads prepared with different mucilages/gums. Biotechnology Reports, 34, e00737. https://doi.org/10.1016/j.btre.2022.e00737
- Rodrigues, F. J., Omura, M. H., Cedran, M. F., Dekker, R. F. H., Barbosa Dekker, A. M., & Garcia, S. (2017). Effect of natural polymers on the survival of Lactobacillus casei encapsulated in alginate microspheres. Journal of Microencapsulation, 34(5), 431–439. https://doi.org/10.1080/02652048.2017.1343872
- Rodríguez-González, F., Pérez-González, J., Muñoz-López, C. N., Vargas-Solano, S. V., & Marín-Santibáñez, B. M. (2021). Influence of age on molecular characteristics and rheological behavior of nopal mucilage. Food Science & Nutrition, 9(12), 6776–6785. https://doi.org/10.1002/fsn3.2629
- Sáenz, C., Sepúlveda, E., & Matsuhiro, B. (2004). Opuntia spp mucilage’s: A functional component with industrial perspectives. Journal of Arid Environments, 57(3), 275–290. https://doi.org/10.1016/S0140-1963(03)00106-X
- Shapiro, K., & Gong, W. C. (2002). Use of herbal products for diabetes by Latinos. Journal of the American Pharmacists Association, 42(2), 278–279. https://doi.org/10.1331/108658002763508542
- Silva, M. A., Albuquerque, T. G., Pereira, P., Ramalho, R., Vicente, F., Oliveira, M., & Costa, H. S. (2021). Opuntia ficus-indica (L.) Mill.: A multi-benefit potential to be exploited. Molecules, 26(4), 951. https://doi.org/10.3390/molecules26040951
- Tiani, K. A., Yeung, T. W., McClements, D. J., & Sela, D. A. (2018). Extending viability of Lactobacillus plantarum and Lactobacillus johnsonii by microencapsulation in alginate microgels. International Journal of Food Science and Nutrition, 69(2), 155–164. https://doi.org/10.1080/09637486.2017.1343285
- Trachtenberg, S., & Mayer, A. M. (1980). Biophysical properties of Opuntia ficus-indica mucilage. Phytochemistry, 21(12), 2835–2843. https://doi.org/10.1016/0031-9422(80)85052-7