ABSTRACT
Fungi can thrive in strawberries, mainly Botrytis cinerea, which causes relevant fruit losses. Moreover, thermal preservation results in phytonutrient losses. This study evaluated the effects of high hydrostatic pressure (HHP, 0, 400, and 600 MPa/5 min) processing with potassium sorbate (PS) or avocado seed acetogenins (ASA) on the nutritional, microbiological, and physicochemical properties of strawberry puree inoculated with Botrytis cinerea. Sole inoculation reduced L-ascorbic acid (L-AA, 84%) and total ascorbic acid (TAA, 38%). Instrumental color Hue angles were reduced from 35.0 to 29.4°, likewise the contents of pelargonidin−3-glucoside (25%) and ellagic acid (38%). Both HHP-PS treated samples contained no viable conidia and high TAA; the no-preservative and HHP-ASA (661 µg/mL, 400 MPa/5 min) purees contained high TAA but had viable conidia, which can induce further phytonutrient losses. HHP and antifungal additives maintained ellagic acid, increased pelargonidin−3-glucoside contents, and inactivated conidia, strengthening the value of combined non-thermal technologies for the preservation of strawberry puree
1. Introduction
Strawberries are eaten fresh or processed into various foods/ingredients such as concentrate, juice, and puree. They contain bioactive compounds such as vitamin C and phenolic compounds, including flavonoids and anthocyanins (Teribia et al., Citation2021). For the food industry, strawberry puree represents an interesting natural ingredient for desserts, ice creams, and milkshakes (Teribia et al., Citation2021). Thermally processed strawberry puree results in degradation of its bioactive compounds such as ascorbic acid, p-hydroxybenzoic acid, ellagic acid, and pelargonidin−3-O-glucoside (Gao et al., Citation2016; Marszałek et al., Citation2017). However, thermal pasteurization is commonly applied to achieve microbial safety and the inactivation of undesirable enzymes. A color change is also a common processing effect, red color changes due to anthocyanin degradation that results in brown color development (Skrede et al., Citation1992).
Strawberry products are very susceptible to the development of fungi, mainly Botrytis cinerea Pers (Chakraborty et al., Citation2014; Hurtado et al., Citation2017; León López et al., Citation2014), which causes gray rot, a disease with great economic and scientific relevance since it can be responsible for up to 89% of postharvest losses of the fruit (Ugolini et al., Citation2014). Contamination with fungi and their spores is a major problem in processed fruits due to their high sugar content and low pH levels; light brown spots and damp strawberry surface areas are associated with spoilage, which later gets covered with a gray powder or mycelium (Pravallika & Chakraborty, Citation2022). Botrytis cinerea counts reported in literature include a prior storage study with whole fresh strawberries that reported yeast and mold counts of 4.5–5 log CFU/g (Nakata & Izumi, Citation2020). Likewise, Graca et al. (Citation2017) studied contamination in strawberries and found 5.2 log CFU/g mold counts. A similar study sampled fresh-cut fruits from the market, without visible signs of decay, and reported yeast and mold counts that ranged from 3.15–6.7 log CFU/g (Jang et al., Citation2021)
Consumer rejection due to the loss of sensory acceptability generates considerable economic losses and food security problems (Pinto et al., Citation2020).
Villa-Rojas et al. (Citation2012) demonstrated the inactivation of Botrytis cinerea conidia by applying a heat treatment of 42°C for 45 min. However, heat treatments are known to affect bioactive compounds and, consequently, strawberry puree’s organoleptic and nutritional characteristics (Marszałek et al., Citation2017). High hydrostatic pressure (HHP) is feasible non-thermal alternative pasteurization technique for foods (Wang et al., Citation2017). HHP processing has been reported to inactivate vegetative forms of pathogenic and spoilage bacteria, yeasts, and molds (Silva & Evelyn, Citation2020), preserving bioactive compounds of nutritional value (Marszałek et al., Citation2017). However, commercial pressures alone have been observed to result in minor inactivation of fungal and bacterial spores in fruit products, which limits shelf-life (Evelyn & Silva, Citation2019; Sarker et al., Citation2015). High-pressure, high-temperature processing (HPHT), also known as pressure-assisted thermal processing (PATP), are needed to achieve efficient spore inactivation (Luu-Thi et al., Citation2014). Silva and Evelyn (Citation2020) reported on the pressure resistance of Byssochlamys nivea and Aspergillus fischeri fungi spores inoculated into strawberry puree and subjected to HHP (600 MPa) combined with high temperatures (75°C) for 15 min; treatments resulted in 1.7 and 1.4 log reductions in the germination of Byssochlamys nivea spores after 12 weeks in potato dextrose agar. Therefore, scientific development challenges remain unsolved for HHP processing for the inactivation of resistant spores, as industrial-scale HHP units can operate at maximum pressures of 650 Mpa with or without combined temperatures up to 50°C (Sarker et al., Citation2015).
In addition to heat treatments, additives such as potassium sorbate (PS) are used in foods as preservatives (Mohammadzadeh-Aghdash et al., Citation2018). PS usage levels of 1 g/kg demonstrated inhibition of fungi growth in fruit preparations (Dehghan et al., Citation2018; Quispe et al., Citation2010). Although PS is considered safe and approved to be used in foods, consumers are moving away from synthetic additives. Possibly because at higher intakes (>25 mg/kg), additives such as PS, have been reported to cause cytotoxic and genotoxic effects by producing mutagenic compounds that induce chromosomal aberrations and sister chromatid exchange (Dehghan et al., Citation2018). For the above, consumers and industries have a growing demand for new antimicrobials of natural origin (Nazir et al., Citation2019). In this sense, avocado seeds have been studied, which have a content of bioactive compounds equivalent to or even higher than its pulp, finding nine lipid molecules with antimicrobial and antioxidant properties (Rodríguez Sánchez et al., Citation2019). Prior literature indicates that persenone-A, persenone-B, and persenone-C isolated from avocado seeds inhibited the germination of Clostridium sporogenes endospores and the growth of vegetative cells (Rodríguez Sánchez et al., Citation2013). It has also been shown that the germination of C. sporogenes endospores was inhibited by an avocado seed oil enriched in acetogenins (Pacheco et al., Citation2017). Previous works also documented that avocado seed oil was effective as a natural alternative for controlling Botrytis cinerea (Echenique Martínez et al., Citation2021). Therefore, the objective of this work was to evaluate the effects of HHP (0, 400, and 600 MPa/5 min) processing with potassium sorbate (PS, 1000 µM/mL) or avocado seed acetogenins (ASA, 0, 661, and 7,500 µg/mL), on the nutritional, microbiological, and physicochemical properties of strawberry puree inoculated with Botrytis cinerea conidia.
2. Materials and methods
The experimental design included two studies. In the first study, the effect of Botrytis cinerea inoculation on the phytonutrient stability of strawberry puree was analyzed. The second study explored the effects of HHP processing of strawberry puree inoculated with Botrytis cinerea conidia and added with potassium sorbate and ASA as combined preservation approaches.
2.1. Strawberry puree preparation
Strawberries (Fragaria x ananassa) Festival variety was obtained by a donation from the company ALPEFRESH (Michoacán, México) through the HEB supermarket (HEB S.A. de C.V., Monterrey, NL México), with a ripeness index #06 (red color that covers 100% of the surface) according to NMX-FF−062-2002 (Official Mexican Standard, Citation2002b). Fresh strawberries free of mechanical injury and microorganisms were selected. Cleaning and disinfection with 150 mg/L NaClO for 2 min were performed, followed by four more washes with distilled water. Strawberry crowns were removed, and the puree was prepared in an industrial blender (Torrey LM−12, TORREY, Nuevo Leon, México). Strawberry puree was homogenized and stored at 4°C for immediate preparation of treatments.
2.2. Treatments
2.2.1. Study I- Botrytis cinerea inoculation
Conidia suspension was prepared as described in detail by Echenique Martínez et al. (Citation2021). Briefly, a colony of Botrytis cinerea incubated for 7-days was prepared. 0.1% Tween 80 was added to cover the colony, and it was scraped with a Drigalsky spatula to release the conidia.
Conidia were then collected, and the viable conidia concentration was determined using the Neubauer chamber. Germination was considered viable when the length of germ tube reached half the size of the conidia. Furthermore, a preliminary study was conducted to standardize the time to verify counts within strawberry puree after the initial inoculation. Strawberry puree samples were stored under refrigeration (4°C ± 2.5°C) for 24 h, then plated into Czapek medium, and viable conidia were counted after 5 h at 4°C ± 2.5°C. Two strawberry mashes were prepared, including a strawberry puree without inoculum (control) and a strawberry puree (3.5 kg) inoculated with a conidia suspension (70 mL) to deliver 6.3 logs of Botrytis cinerea conidia/mL. Purees were stirred manually with a circular agitation movement for 30 s to obtain a homogenous mixture. Subsequently, the samples were packed in using a Evd−20 Torrey vacuum sealer (Monterrey, NL, Mexico) set at 3.2 s/2 psi. Triplicate samples were placed into nylon-polyethylene stomacher bags (3 mm standard barrier, 10 × 12”, oxygen transmission rate: 63 cm3/m2, for 24 h at 23°C dry; moisture vapor transmission rate 4.8 g/m2, for 24 h at 38°C and 90% relative humidity, Unline, Monterrey, Mexico). All samples for microbiological analyses were collected and stored under refrigeration conditions (4.0 ± 2.5°C). Bacterial analyses were plated the same day after HHP processing. Strawberry puree samples were stored under refrigeration (4°C ± 2.5°C) for 24 h to allow conidia germination, then plated into Czapek medium, kept at 4.0 ± 2.5°C, and viable conidia were counted after 5 has described in the preliminary conidia germination study conducted in the puree matrix. Samples for physicochemical analyses were stored at −80.0 ± 1.0°C to stop oxidation and enzymatic activities until further testing.
2.2.2. Study II- Botrytis cinerea inoculation before high hydrostatic pressure (HHP) processing
Following the same inoculation procedure described for Study I, three different treatments were prepared for the second study, including strawberry puree added with 6.3 log conidia/mL + 661 µg/mL of ASA, strawberry puree + 6.3 log conidia/mL + 7,500 µg/mL of ASA, and strawberry puree + 6.3 log of conidia/mL +1 g/Kg of potassium sorbate. ASA concentrations were selected based on prior studies by Echenique Martínez et al. (Citation2021) on the growth kinetics of Botrytis cinerea conidia as affected avocado acetogenins. Subsequently, the treatments were vacuum packed and stored as described in Study I and treatment with HHP as described in the following section.
2.3. High hydrostatic pressure (HHP) processing
Experiments were carried out in high-pressure processing equipment (2 L, Avure Technologies, Middletown, OH, U.S.A) using water as pressurizing medium. Equipment was operated at pressures between 400 and 600 MPa for 5 min, and recorded water temperature (after reaching the pressure level) ranged from 18 to 32°C due to adiabatic heating during the holding time. Compression rates were within 420–630 MPa/min; the decompression was almost instantaneous. The bags were introduced into the high-pressure equipment, processed, and immediately stored at 4°C. Triplicate HHP processing treatments were performed, untreated control treatments were also submerged in the equipment chamber for 5 min, and after processing, all treatments were stored immediately at −80.0 ± 1°C until their subsequent analysis. Treatments from studies I and II were analyzed according to the techniques described below.
2.4. Physicochemical analysis
Physicochemical measurements of strawberry puree included pH, titratable acidity, total soluble solids, water activity, and color, according to Association of Official Analytical Chemists International (AOAC, Citation2002) methodologies. Titratable acidity was determined using titration equipment (Mettler Toledo-DL21), soluble solids using a refractometer (PR−32 ATAGO), water activity using a hydrometer (HP23-AW, Rotronic®, Switzerland). Instrumental color was determined with a Konica MinoltaTM CM Series 600 d colorimeter (Osaka, Japan), using system scale CIELAB with a standard illuminant D65 and a viewing angle of 10. The instrument was calibrated with a white mosaic as a color standard, where L* represents the values from 0 to 100, meaning shades from black (0) to white (100). Instrumental color was expressed in polar coordinates as Chroma (C*) = [(a*2 + b*2)1/2] and Hue angle (h*) = [arctan (b*/a*) x 180/π]. Instrumental color measurements were determined ten times.
2.5. Analysis of bioactive compounds
2.5.1. Determination of vitamin C
L-ascorbic acid, L-dehydroascorbic acid, and total ascorbic acid were extracted according to Marszałek et al. (Citation2017) with slight modifications. Treatments (2 g) were titrated with 0.01% metaphosphoric acid (HPO3) (10 mL), shaken manually, and sonicated (Branson Ultrasonics™ S800) for 10 min/40 Hzh at 25°C in the dark with N2 in the headspace. Samples were then centrifuged at 3700 × g for 10 min at 4°C, and the supernatant was recovered. Sep-pack C18 columns were conditioned, and the eluents from the treatments were recovered. Aliquots were taken for L-ascorbic acid and aliquots for L-dehydroascorbic acid, to which Dithiothreitol (8 mM) was added and left to settle for 1 h. All treatments were filtered using PVDF syringe filters with 0.22 µm membranes (Millex®GV, Millipore, Billerica, MA, U.S.A), placed in amber vials, and N2 was flushed into the headspace. L-ascorbic acid, L-dehydroascorbic acid, and total ascorbic acid were quantified in a UPLC unit (Acquity, Waters, Milford, MA. U.S.A) coupled to a diode array detector (DAD) and quantified at a wavelength of 245 nm (λ: 210-400 nm). Chromatographic separation was achieved using a Nova Pack C18 column (4 µm, 3.9 × 150 mm) maintained at 25°C. The mobile phase consisted of 0.01% HPO3 using the isocratic method and a flow rate of 0.7 mL/min; a volume of 20 µL of the treatments was injected at 10°C for 10 min. L-ascorbic acid quantification was performed with calibration curves of commercial L-ascorbic acid standards and DL-dithiothreitol (Sigma Aldrich, U.S.A) to determine total ascorbic acid.
2.5.2. Determination of phenolic compounds
Strawberry phenolic compounds, including p-hydroxybenzoic acid and ellagic acid, were extracted according to Irakli et al. (Citation2012) and Marszałek et al. (Citation2017) with slight modifications. Samples of puree were weighed (5 g) and mixed with MeOH:H2O:HCl (15 mL, 80:19.9:0.1), shaken and sonicated (Branson Ultrasonics™ S800) for 10 min/40 Hz/25°C in the dark with N2 in the headspace. Samples were centrifuged at 3700 × g for 5 min at 4°C, and the supernatant was recovered, filtered through Whatman #01 paper, and evaporated under vacuum at 200 rpm/30 min/35°C obtaining 20 mL. Samples were adjusted to 25 mL with 0.1% H2O:PO4 (v/v), and the treatments were kept at −20°C until later use. 1 mL of the extract was weighed and added to 39 mL of MeOH:HCl:H2O (56:25(6 M):19), then it was hydrolyzed at 120°C/90 min, allowed to cool to room temperature and made alkaline with NaOH (10 M) at pH 3.5. An SP-C18 MeOH:H2O (50:50)(pH2)) column was conditioned twice, ending with MeOH; subsequently, 5 mL of the extract was added to the SP-C18, and the eluent was recovered, 5 mL of H2O (pH 3.5) was added to the same SP-C18, and it was homogenized with the eluent. Phenolics were eluted with 10 mL of MeOH:H2O:HCl (80:19.9:0.1). The treatments were filtered using PVDF syringe filters with 0.22 µm pores and made up to 25 mL with MeOH:H2O:HCl (90:9.9:0.1) and stored at −20°C. To determine the content of p-hydroxybenzoic acid and ellagic acid, the equipment and column mentioned in step 2.5.1. were used, at a wavelength of 270 nm (260–280 nm). The mobile phases consisted of A: MeOH:H2O:CH3-CO2H (10:88:2; v/v/v) and B: MeOH:H2O:CH3-CO2H (90:8:2; v/v/v). The solvent flow rate was 0.7 mL/min, using gradients of 100% A (0–2 min); 100 to 75% A (2–8 min); 75 to 60% A (8–22 min); 60 to 55% A (22–23 min); from 55 to 100% A (23–28 min). p-hydroxybenzoic acid and ellagic acid were quantified using commercial standard calibration curves prepared in MeOH:H2O:HCl (50:49:1).
2.5.3. Determination of anthocyanins
Pelargonidin−3-O-glucoside quantification was measured according to Marszałek et al. (Citation2017) with slight modifications. Extracts (10 mL) from the previous step (2.5.2. determination of phenolic compounds) were filtered on a previously conditioned SP-C18 column, the eluent was discarded and the SP-C18 fraction was eluted with 5 mL of MeOH:H2O:HCl (75:24.9:0.1), the eluent was recovered and filtered through PTFE with 0.22 µm membranes and stored at −20°C. Quantification of pelargonidin−3-O-glucoside, the equipment, column, flow rate, and mobile phase mentioned in the previous step were used at a wavelength of 520 nm. Gradients from 100% A (0–2 min), from 100 to 63% A (2–6 min), from 63 to 62% A (6–9 min) were used; 62 to 61% A (9–10 min); 61 to 50% A (0–11 min); from 50 to 100% A (11–18 min). Pelargonidin−3-O-glucoside was quantified using calibration curves from a commercial standard.
2.6. Microbiological analysis
Total aerobic and Botrytis cinerea conidia were counted following the procedure described by NOM−213-SSA1-2002 (Official Mexican Standard, Citation2002a) and by the U.S. FDA-BAM (2022).
2.7. Statistical analysis
A completely randomized design with 3 × 4 factorial arrangement was used to analyze the effect of high hydrostatic pressures assisted with ASA and PS on the physicochemical quality, bioactive compounds, and microbiology of strawberry puree. High-pressure treatments were applied at three levels (0, 400, and 600 MPa/5 min) and antifungal food additives at four levels, including two ASA concentrations (661 and 7,500 µg/mL), one concentration of PS (1,000 µg/mL) and control without preservative (0 µg/mL). Treatments and analytical determinations were experimented in triplicate. Normality and equality of variance assessments were conducted by the Shapiro-Wilk and Levene’s tests, respectively. Parametric variables were analyzed by Analysis of variance (ANOVA) and multiple pairwise comparisons were conducted by the Tukey-Kramer multiple comparison post-hoc tests. Non-parametric data was analyzed by Kruskal-Wallis with Dunn post-hoc tests with Bonferroni correction for mean comparisons. Significant differences were determined at a p < .05 using XLSTAT software (XLSTAT, 2022.4.1, Addinsoft, New York, U.S.A).
3. Results and discussion
3.1. Study I: Physicochemical quality of strawberry puree without and with Botrytis cinerea inoculum
Inoculation of the phytopathogenic fungus into strawberry puree resulted in higher pH and soluble solid values (p < .05) (). At the same time, initial titratable acidity and water activity values decreased. Some instrumental color parameters, such as lightness values, did not change when Botrytis cinerea was inoculated. However, the C* values and H* angles decreased, indicating a less vivid color (opaque) and modifications in the characteristic red color tone, respectively. Contamination with fungi is known to change fruit appearance that result in quality losses. In the case of juices, fungi can cause turbidity; however, in semi-solid foods such as purees, it is possible to see the mycelium growing (a cottony mass of the characteristic color of the developing phytopathogenic fungus) on the matrix, which causes color changes, sour flavors, etc (Pravallika & Chakraborty, Citation2022). In agreement with the observations described for strawberry puree (), a previous study with red peppers contaminated with Fusarium spp also reported decreases in Hue angles of instrumental color (Frans et al., Citation2021). Potential mechanisms for reduction of bioactive compounds seem to be related to plant-pathogen interactions, in which there is an increase in ROS, known as oxidative burst upon pathogen infections that plays a signaling role, leading to enhanced disease resistance in plants (Wang et al., Citation2019). However, excessive ROS accumulation in the infection sites damages cellular membranes through lipid peroxidation (Chen et al., Citation2019) and could degrade pigments and nutrients. The fungi’s impact on the cell wall may also result in loss of integrity and leaching of components, which may explain the observed increases of soluble solids and the pH of the strawberry purees.
Table 1. Effect of Botrytis cinerea conidia inoculated in strawberry puree on physicochemical analyses.
3.2. Study I: Stability of bioactive compounds in strawberry puree without and with Botrytis cinerea inoculum
3.2.1. Vitamin C
Inoculation of strawberry puree with Botrytis cinerea conidia generated highly significant vitamin C degradation (p < .05) (). L-ascorbic acid and total ascorbic acid are reduced by 84% and 38%, respectively. Conversely, L-dehydroascorbic acid, the oxidized form of the vitamin, increased ( and Supplementary Table S1). The loss of ascorbic acid in whole fruits such as oranges, pineapple, and bell peppers, contaminated by fungi such as Sclerotium rolfsii, has been reported by other authors (Frans et al., Citation2021). Potential plant metabolism mechanisms include the fruit’s physiological response to pathogenesis involving oxidation reactions in which ascorbic acid participates. L-ascorbic acid is the primary antioxidant that operates in the oxidation response by forming non-oxidizing products such as dehydro-ascorbate and 2,3 diketogulonic acid (Frans et al., Citation2021; Javanmardi et al., Citation2023). In addition, it has been reported that pathogenic fungi such as Rhizopus stolonifer, Aspergillus flavus, Penicillium digitatum, Curvularia lunata, and Fusarium moniliforme produce toxins that decrease the concentration of total ascorbic acid (Sawant & Gawai, Citation2011). In other studies, without fungi inoculation, it was observed that anthocyanins and color remained unchanged during the holding time between grinding and pasteurization, but L-AA was oxidized to DHA (Teribia et al., Citation2021). In the present work, the conversion appeared exacerbated by the fungi, leaving only 15% of the original L-AA levels in a reduced form ().
Figure 1. Effects of Botrytis cinerea conidia inoculated into strawberry puree on the stability of bioactive compounds. A) L-ascorbic acid, L-dehydroascorbic acid and total ascorbic acid; B) p-hydroxybenzoic acid and ellagic acid; C) pelargonidin − 3-glucoside. Error bars (‡) represent the standard deviations of means (n = 3). Different lowercase letters above the graph bars indicate statistical significance (p < .05).
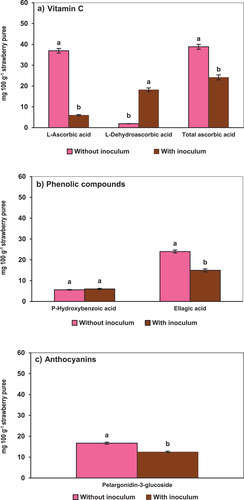
3.2.2. Phenolic compounds
In the inoculated puree, the concentration of p-hydroxybenzoic acid () did not present significant differences (p < .05) in reference to controls; however, a slight increase (7%) was observed. On the contrary, ellagic acid was degraded by 38% (p < .05) ( and Supplementary Table S1). Other authors have reported the reduction of phenolic compounds in whole strawberries contaminated by Botrytis cinerea and attributed it to oxidation reactions (Javanmardi et al., Citation2023). As previously discussed, plant-pathogen interactions usually coincide with the accumulation of reactive oxygen species (ROS); the infection also damages cell membranes through lipid peroxidation. Additionally, faced with the proliferation of ROS in infected plants, their response is to increase antioxidant enzymatic activities to eliminate ROS under stressful conditions (Ahammed et al., Citation2020; Chen et al., Citation2019). Furthermore, the damaged tissue manufactures various secondary metabolites to enhance resistance to biotic and abiotic stressors. Flavonoids, phenols, and lignin are the major groups of secondary metabolites that play an essential role in plant defense against pathogens (El-Sharkawy et al., Citation2018; Wang et al., Citation2019).
3.2.3. Anthocyanins content
Pelargonidin−3-glucoside is one of the anthocyanins responsible for strawberry color. In the presence of Botrytis conidia, it was degraded by 25% ( and Supplementary Table S1). The instrumental color changes of C* and h* functions () were correlated with pelargonidin−3-glucoside losses. Degradation was possibly caused by oxidative reactions related to the plant-pathogen interaction (Wang et al., Citation2019). In industrial settings, unit operations such as crushing, and sieving also generate opportunities for fungi interaction with the fruit tissues. Furthermore, industrial processing times can vary, the mash can be stored in holding tanks prior to pasteurization. Based on the present results, fungi contamination can cause extreme phytonutrient degradation during industrialization in addition to known color changes caused by enzymatic and non-enzymatic anthocyanin oxidation (Marszałek et al., Citation2017; Pinto et al., Citation2020; Teribia et al., Citation2021).
3.3. Study II: microbiological and physicochemical quality of strawberry puree inoculated with Botrytis cinerea conidia, as affected by high hydrostatic pressure and antifungal food additives
3.3.1. Microbial counts
shows that colony-forming units (CFUs) of aerobic bacteria were below 100 CFU/mL for all treatments, including unprocessed purees (0 MPa), indicating that the product complied with good manufacturing standards by NOM−130 (Official Mexican Standard, Citation2002a). HHP processing resulted in complete reductions of aerobic counts to non-detectable levels in all puree samples, with or without antifungal food additives (). In contrast, microbial determinations for the fungi indicated that no Botrytis cinerea conidia were detected in the purees treated with 600 MPa/5 min for either of the treatments (). However, at lower pressures (400 MPa, conidia remained viable in the treatments without preservatives and in the low ASA concentration treatments (661 ug/mL). In contrast, there were non-viable conidia in the other two treatments subjected to the same HHP level; therefore, both potassium sorbate and higher ASA level (7500 ug/mL) assisted the 400 MPa/5 min pressures in the inactivation of conidia. Interestingly, for unprocessed samples (0 MPa/min) the ASA addition also resulted in lower viable conidia counts in reference to samples without preservatives (), however higher ASA levels (7500 ug/mL) did not provide significantly lower counts in reference to the lower ASA levels (661 ug/mL). Observations are possibly related to the limited solubility of ASA in the strawberry matrix due to their lipidic nature. Based on results HHP 400 MPa samples facilitated the capacity of ASA (7500 ug/mL) to inactivate conidia by a facilitation of the interaction between acetogenins in a more homogeneous matrix. Acetogenin inactivation mechanism was not the focus of the present work; however, Domergue et al. (Citation2000) studied the antifungal properties of two acetogenin molecules (persin and persenone A), also present in the ASA extract evaluated in the current work. The authors concluded that these compounds have antifungal activity since they inhibit the germination and elongation of the germ tube of the phytopathogenic fungus Colletotrichum gloeosporioides affecting the morphology of the conidia. Salinas et al. (Citation2017) reported on the antibacterial properties of acetogenins and observed that their action was comparable to that of the lipophilic synthetic product lauroyl ethyl arginate (LAE). The antimicrobial spectrum of LAE has been reported to inhibit molds and yeasts at lower doses. LAE application damaged structures of fungal and bacterial cells, loss of intracellular protein and nucleic acid. The compound also reduces the membrane potential and depolarization ratios, causing a rough surface, irregular cellular organelles, protoplast shrinkage, intracytoplasmic coagulation, and empty cavities (Xu et al., Citation2018). According to prior works, the mechanism of action of ASA involves cytoplasmic membranes of bacteria, altering their metabolic processes and inhibiting their standard cycle-producing cell lysis (Salinas et al., Citation2017).
Figure 2. Microbial counts in strawberry puree inoculated with conidia of Botrytis cinerea as affected by high hydrostatic pressure processing in the presence of potassium sorbate (PS) or avocado seed acetogenins (ASA). A) total aerobic on plate count agar; B) Botrytis cinerea conidia on potato dextrose agar. Error bars (‡) represent the standard deviations of means (n = 3). Different lowercase letters above the graph bars indicate statistical significance (p < .05). ND= not detected.
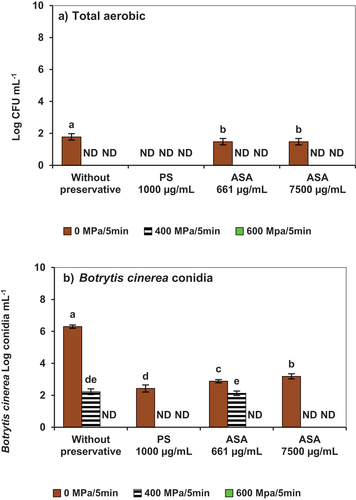
In the present work, potassium sorbate assisted pressure (400 MPa) in the inactivation of Botrytis conidia (). The antifungal effect of the additive has been attributed to its double bonds, which interfere with the catalytic activity of critical enzymes needed for microbial growth (Lück & Jager, Citation2000). Acetogenins mechanisms of action are less known, but ASA extracts also contain double bonds in their aliphatic chain; also, trans-enone groups and other chemical features, such as a terminal acetate group, have been reported to potentiate antibacterial activity (Pacheco et al., Citation2017; Rodríguez Sánchez et al., Citation2019; Salinas et al., Citation2017; Villarreal Lara et al., Citation2019). Although more testing is required, it can be hypothesized that the lipophilic nature of acetogenins allows them to penetrate the cell membrane of conidia and exert interferences with the catalytic activity of enzymes. Potassium sorbate has been reported to affect enzymes responsible for growth, attributing its fungistatic effect (Dehghan et al., Citation2018; Quispe et al., Citation2010). Another hypothetical mechanism involves stabilizing free radicals by the fungi metabolism, resulting in increased membrane permeability and cell lysis (Echenique Martínez et al., Citation2021). Song et al. (Citation2022) mentioned that germinating bacterial spores are more susceptible to stressors than their dormant forms. In this sense, implementing spore germination before the HHP step can potentially eradicate several spores at higher pressures (>400 MPa), bacterial spore germination can be induced by opening channels that allow the loss of dipicolinic acid. High pressures also may directly impact the cell wall and membrane of the conidia of fungi, causing a possible leakage of the plasmatic constituents, as described by Balasubramaniam et al. (Citation2016). In this context, Van-Leeuwen et al. (Citation2013) suggested that fungal conidia’s stress resistance can thrive during germination’s early stages. This related phenomenon can affect the development process, as explained by Choquer et al. (Citation2007). In addition, González et al. (Citation2015) identified a mitochondrial precursor (peroxiredoxin−5), two proximal dismutase, and two superoxide dismutase enzymes in latent cells of Botrytis cinerea during germination of conidia, which is considered that the conidia will be viable; Botrytis cinerea induces an oxidative shock in plant cells during the early stages of the infection process, accumulating hydrogen peroxide in the germ tube, as well as peroxiredoxin and catalase, these enzymes regulate processes to maintain high levels of hydrogen peroxide in the germ tube during germination. In the present study, it was observed that water activity did not change for samples with preservatives and low HHP (400 MPa/5 min) (p < .05) () in reference to the same purees without antifungal additives subjected to the same pressure. However, at higher pressures (600 MPa/5 min), the water activity decreased for the HHP-ASA and HHP-PS samples.
Table 2. Effect of high hydrostatic pressures in the presence of potassium sorbate or avocado seed acetogenins (ASA) in strawberry puree inoculated with Botrytis cinerea conidia (6.3 log conidia/mL) on physicochemical analyses.
3.3.2. Physicochemical parameters
It was observed that the soluble solids were not affected by ASA addition. On the contrary, the addition of potassium sorbate resulted in a slight decline in soluble solids (p < .05) (). As in study I, the presence of Botrytis conidia caused color changes in all samples (p < .05), specifically in the h* and C* values (). ASA increased h* angles, which were higher with both high-pressure treatments (400 and 600 MPa/5 min). However, those colorimetric functions were not affected by HHP processing in the samples that contained potassium sorbate (). Hurtado et al. (Citation2017) reported HPP treated red-fruit smoothies had a higher oxidization tendency and presented undesirable changes in colorimetric functions.
3.3.3. Vitamin C
As previously discussed, inoculation with Botrytis cinerea had a significant impact on the vitamin C profiles of the strawberry puree; L-ascorbic was no longer the main form of the vitamin (). The oxidized form (L-DHA) was the main vitamin form (). In that highly oxidized scenario, some of the treatment combinations of high pressure and antifungal additives were able to inactivate conidia and prevent further vitamin degradation. Processing resulted in further L-AA losses, ranging from 7.7 to 100% (Supplementary Table S2). The highest L-AA retention was achieved in the no-preservative and HHP-ASA (661 µg/mL) samples pressurized at 600 MPa/min (p < .05) (). HHP at 400 MPa/5 min resulted in L-AA declines and conversion into L-DHA. The HHP-ASA samples that contained 7500 µg/mL did not result in higher vitamin retention. As for L-DHA levels (), the use of potassium sorbate resulted in significantly higher concentrations that corresponded with the L-AA interconversion (p < .05). The overall vitamin C balance summarized as TAA () indicated that HHP-PS samples conserved higher levels (p < .05) and contained no viable conidia (). The no-preservative and HHP-ASA (661 µg/mL) samples also contained higher TAA; however, those samples contained viable conidia () that could result in further phytonutrient losses during the product shelf-life. Results were like those reported by Hurtado et al. (Citation2017, Citation2019). who observed that the application of 350 MPa inactivated bacteria, yeasts, and fungi in red-fruit smoothie blends containing strawberries, but the ascorbic acid content was reduced; and vitamin C profiles indicated that the predominant form was L-DHA. Botrytis cinerea conidia growth has been reported to produce the enzyme ascorbate oxidase, which catalyzes the conversion of L-AA into DHA (González et al., Citation2014; González et al., Citation2015), a possible explanation for the intense oxidation observed in the present work.
Figure 3. Stability of vitamin C contents in strawberry puree inoculated with conidia of Botrytis cinerea as affected by high hydrostatic pressure processing in the presence of potassium sorbate (PS) or avocado seed acetogenins (ASA). A) L-ascorbic acid; B) L-dehydroascorbic acid; C) total ascorbic acid. Error bars (‡) represent the standard deviations of means (n = 3). Different lowercase letters above the graph bars indicate statistical significance (p < .05). ND= not detected.
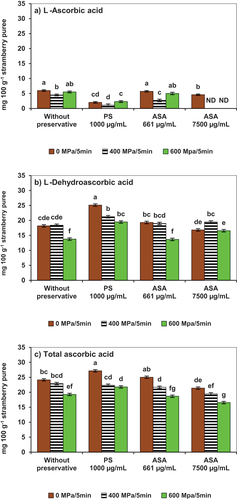
3.3.4. Phenolic compounds
The highest concentrations of p-hydroxybenzoic acid were recorded in all the unprocessed before the application of HHP () (p < .05). Samples without preservative and the HHP-ASA (661 µg/mL) purees processed at 400 MPa contained the highest p-hydroxybenzoic acid concentrations (p < .05), and they were also the HHP treated samples with viable conidia (). The viable fungi may have been an additional stressor in those samples, which resulted in higher concentrations of that specific phenolic compound.
Figure 4. Stability of phenolic compounds in strawberry puree inoculated with conidia of Botrytis cinerea as affected by high hydrostatic pressure processing in the presence of potassium sorbate (PS) or avocado seed acetogenins (ASA). A) p-hydroxybenzoic acid; B) ellagic acid; C) pelargonidin − 3-glucoside. Error bars (‡) represent the standard deviations of means (n = 3). Different lowercase letters above the graph bars indicate statistical significance (p < .05).
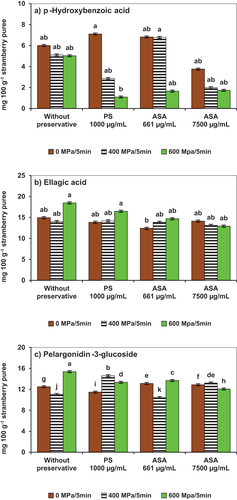
Ellagic acid () concentrations increased, particularly in samples without preservatives and with potassium sorbate when processed at 600 MPa/5 min (p < .05). In the HHP-ASA (661 µg/mL) samples, ellagic acid concentrations also increased, although values were not significantly different from the untreated control. As summarized in a review by Navarro-Baez et al. (Citation2022), various fruit and vegetable products treated with HHP have shown higher phenolic contents than their untreated counterparts. These results have been associated with higher extractability from cellular tissues and de novo biosynthesis. Cell disruption induced by HHP processing with pressures of 500 to 600 MPa has been reported to release cell-wall bound phenolics. However, HHP processing at lower pressures (15 to 100 MPa) have been reported to induce de novo biosynthesis of phenolics resulting in increases of 155% in comparison to initial concentrations (Navarro-Baez et al., Citation2022). In addition to pressure, biotic stress can also be caused by the action of fungi that attack plants by secreting enzymes to break down tissues and may also release phenolic compounds (Vidal, Citation2010).
It has been proposed that the effects of pressure as an abiotic stressor to plant cells starts with the physical fracture or loosening of cell wall polymers (Ortega et al., Citation2013), a perturbation that, when sensed by the plant cells begins the abiotic responses (Liang, Citation2018). Ślesak et al. (Citation2007) described the cascade of events that follow in plants after sensing abiotic stresses, including the production of oxidative molecules such as H2O2. The presence of H2O2 induces gene expression focused on strengthening the damaged cell walls by signaling Ca+2, enzymes, and hormones required for the reparations. As a signaling molecule in activating metabolic pathways, H2O2 activates phenylalanine ammonium lyase activity, synthesizing simple phenols derived from cinnamic acid (Gordo, Citation2018; Jacobo Velázquez et al., Citation2021). Increases in phenolic contents up to 154.9% have been reported in carrots treated with HHP at 100 MPa (Yasunaga et al., Citation2018). The increment in phenolic compounds observed after HHP is not always attributed to biosynthesis. The release of phytochemicals by extraction from specific organelles could also result in the increments (Gómez-Maqueo et al., Citation2020). As previously discussed, increases in phenolics at pressure levels above 100 MPa have been attributed to cell membrane disruption and release of bound phenolics, which differs from biosynthesis, therefore a dual stress-response related to ATP, ROS, and involvement of enzymes is a possible mechanism (Navarro-Baez et al., Citation2022). In addition to this, the activities of PPO and POD can increase in HHP treated samples; therefore, modifications or decreases in the concentration could also be attributed to oxidative stress (Zhang et al., Citation2021), as well as by the polymerization induced by high-pressure enzymatic reactions (Szczepańska et al., Citation2020).
3.3.5. Anthocyanins content
Pelargonidin−3-glucoside contents were affected by the different treatments studied in the present work. As previously described inoculation resulted in degradation of 25% of the initial concentrations present in the strawberry puree, which had an impact in the red color of the product.
Starting in that highly oxidative environment it was observed that the application of HHP resulted in higher concentrations in all treatments regardless the type of antifungal additive and concentration (). But with two exceptions to that generalization, the HHP samples treated at 400 MPa/5 min without preservative and with ASA (600 µg/mL) resulted in the degradation of pelargonidin−3-glucoside (−12 and −21%, respectively). Both samples contained viable conidia (), confirming its relevant role in the degradation of strawberry anthocyanins and its negative impacts on nutritional and color characteristics of the fruit. In plants, anthocyanins are in vacuoles, and HHP processing has reported to affect the vacuole membrane. In prior works, HHP made the extraction of anthocyanins from strawberry pulps more accessible (Cao et al., Citation2011). Some studies have shown that HPPs can affect the microstructure of food plant tissues, increasing the extractability of bioactive compounds (Pimenta et al., Citation2020; Szczepańska et al., Citation2020; Vázquez Gutiérrez et al., Citation2013; Xi & Luo, Citation2016). Rodríguez Roque et al. (Citation2015) observed that HHP increased the bioaccessibility of phenolic compounds from fruit juices and related beverages. Additionally, PPO and POD activities have been reported to increase in HHP samples, therefore oxidative stress induced biosynthesis can also take place in the plant tissues (Szczepańska et al., Citation2020; Zhang et al., Citation2021).
4. Conclusion
In this study, fungi-induced oxidative stress generated the highest phytonutrient losses (L-AA was reduced by 84%, total vitamin C by 38%, ellagic acid by 38%, and pelargonidin−3-glucoside content by 25%). The highest pressures (600 MPa for 5 min) resulted in complete conidia inactivation, with or without antifungal additives. For the lower pressure treatments (400 MPa for 5 min), the presence of ASA (7500 µg/mL) and potassium sorbate-assisted treatments achieved non-viable Botrytis cinerea conidia inactivation. HHP samples resulted in higher concentrations of p-hydroxybenzoic acid, ellagic acid, and pelargonidin−3-glucoside retention. However, the two HHP samples treated at 400 MPa/5 min that contained viable conidia, without preservatives and with ASA (661 µg/mL), resulted in pelargonidin−3-glucoside degradation. L-AA was also unstable in the presence of ASA (7500 µg/mL) and in samples with viable conidia. The present study established that inoculation of strawberries with Botrytis cinerea caused high vitamin C and phytonutrient losses; therefore, field, and post-harvest contamination must be prevented. Processing steps such as sanitizing of fruit prior to mashing may alleviate phytonutrient losses in subsequent processing steps. HHP processing at lower pressures (400 MPa/5 min) in combination with potassium sorbate or avocado acetogenins can reduce vitamin and pigment degradation, and higher pressures (600 MPa/5 min) inactivated conidia without the need of preservatives. However further studies are recommended to study phytonutrient storage stability.
Practical application
This project focused on the phytonutrient stability of strawberry puree in the presence of 6.3 logs of Botrytis cinerea conidia/mL. High hydrostatic pressure (HHP) processing and two antifungal food additives (potassium sorbate and avocado seed acetogenins (ASA)) were explored as combined preservation approaches. Fungi-induced oxidative stress generated the highest phytonutrient losses. HHP processing combined with antifungal additives treatments resulted in slight amelioration of further phytonutrient losses, including higher retention of vitamin C, ellagic acid, and pelargonidin−3-glucoside. Non-viable Botrytis cinerea conidia were observed in the lower-pressure treatments with some food additives, strengthening the value of combined non-thermal technologies for fungi inhibition and further reducing detrimental nutritional impact.
Supplemental Material
Download MS Word (44.9 KB)Acknowledgement
This research was funded by the Mexican National Council for Research and Technology (CONACyT) scholarship awarded to author Ariel Arami Echenique Martínez. Funding was also provided by Centro de Investigación en Alimentación y Desarrollo A.C. (CIAD), Tecnológico de Monterrey through Translational-Omics Group (GIEE) and The Institute for Obesity Research. The authors are grateful to HEB S.A de C.V. (Monterrey, México) and ALPEFRESH (Michoacán, México), for providing the strawberry fruits used in this study.
Disclosure statement
Some of the potential commercial uses of acetogenins in the food, pharmaceutical, and personal care industries are protected under granted patents and patent applications including US2018013671A1, EP2851062B1, US 10582707B2 and CA2807779. The authors declare that the research was conducted in the absence of any commercial or financial relationships that could be construed as a potential conflict of interest.
Supplementary data
Supplemental data for this article can be accessed online at https://doi.org/10.1080/19476337.2023.2222812.
Additional information
Funding
References
- Ahammed, G. J., Li, C. X., Li, X., Liu, A., Chen, S., & Zhou, J. (2020). Overexpression of tomato RING E3 ubiquitin ligase gene SlRING1 confers cadmium tolerance by attenuating cadmium accumulation and oxidative stress. Physiologia Plantarum, 173(1), 449–459. https://doi.org/10.1111/ppl.13294
- Association of Official Analytical Chemists International. (2002). Official methods of analysis of AOAC international (Vol. I and II, 17th ed.). AOAC International.
- Balasubramaniam, V. M., Barbosa-Cánovas, G. V., Barbosa-Cánovas, G. V., & Lelieveld, H. L. M. (Eds.). (2016). High pressure processing of food: Principles, technology and applications. Springer. https://doi.org/10.1007/978-1-4939-3234-4
- Cao, X., Zhang, Y., Zhang, F., Wang, Y., Yi, J., & Liao, X. (2011). Effects of high hydrostatic pressure on enzymes, phenolic compounds, anthocyanins, polymeric color and color of strawberry pulps. Journal of the Science of Food and Agriculture, 91(5), 877–885. https://doi.org/10.1002/jsfa.4260
- Chakraborty, S., Kaushik, N., Rao, P. S., & Mishra, H. N. (2014). High-pressure inactivation of enzymes: A review on its recent applications on fruit purees and juices. Comprehensive Reviews in Food Science and Food Safety, 13(4), 578–596 752. https://doi.org/10.1111/1541-4337.12071
- Chen, S.-C., Ren, J.-J., Zhao, H.-J., Wang, X.-L., Wang, T.-H., Jin, S.-D., Wang, Z.-H., Li, C.-Y., Liu, A.-R., Lin, X.-M., & Ahammed, G. J. (2019). Trichoderma harzianum improves defense against Fusarium oxysporum by regulating ROS and RNS metabolism, redox balance, and energy ow in cucumber roots. Phytopathology, 109(6), 972–982. https://doi.org/10.1094/PHYTO-09-18-0342-R
- Choquer, M., Fournier, E., Kunz, C., Levis, C., Pradier, J.-M., Simon, A., & Viaud, M. (2007). Botrytis cinerea virulence factors: New insights into a necrotrophic and polyphageous pathogen. FEMS Microbiology Letters, 277(1), 1–10. https://doi.org/10.1111/j.1574-6968.2007.00930.x
- Dehghan, P., Mohammadi, A., Mohammadzadeh-Aghdash, H., & Dolatabadi, J. E. N. (2018). Pharmacokinetic and toxicological aspects of potassium sorbate food additive and its constituents. Trends in Food Science & Technology, 80, 123–130. https://doi.org/10.1016/j.tifs.2018.07.012
- Domergue, F., Helms, G. L., Prusky, D., & Browse, J. (2000). Antifungal compounds from idioblast cells isolated from avocado fruits. Phytochemistry, 54(2), 183–189. https://doi.org/10.1016/s0031-9422(00)00055-8
- Echenique Martínez, A. A., Rodriguez-Sanchez, D. G., Troncoso-Rojas, R., Hernández-Brenes, C., Robles-Ozuna, L. E., & Montoya-Ballesteros, L. C. (2021). Antifungal effect of acetogenins from avocado (Persea americana Mill.) seed against the fungus Botrytis cinerea. International Food Research Journal, 28(5), 1078–1087. https://doi.org/10.47836/ifrj.28.5.21
- El-Sharkawy, H. H. A., Rashad, Y. M., & Ibrahim, S. A. (2018). Biocontrol of stem rust disease of wheat using arbuscular mycorrhizal fungi and Trichoderma spp. Physiological and Molecular Plant Pathology, 103, 84–91. https://doi.org/10.1016/j.pmpp.2018.05.002
- Evelyn, & Silva, F. V. M. (2019). Heat assisted HPP for the inactivation of bacteria, moulds and yeasts spores in foods: Log reductions and mathematical models. Trends in Food Science & Technology, 88, 143–156. https://doi.org/10.1016/j.tifs.2019.03.016
- Frans, M., Aerts, R., Ceusters, N., Luca, S., & Ceusters, J. (2021). Possibilities of modified atmosphere packaging to prevent the occurrence of internal fruit rot in bell pepper fruit (Capsicum annuum) caused by Fusarium spp. Postharvest Biology and Technology, 178, 111545. https://doi.org/10.1016/j.postharvbio.2021.111545
- Gao, G., Ren, P., Cao, X., Yan, B., Liao, X., Sun, Z., & Wang, Y. (2016). Comparing quality changes of cupped strawberry treated by high hydrostatic pressure and thermal processing during storage. Food and Bioproducts Processing, 100, 221–229. https://doi.org/10.1016/j.fbp.2016.06.017
- Gómez-Maqueo, A., Welti-Chanes, J., & Cano, M. P. (2020). Release mechanisms of bioactive compounds in fruits submitted to high hydrostatic pressure: A dynamic microstructural analysis based on prickly pear cells. Food Research International, 130, 108909. https://doi.org/10.1016/j.foodres.2019.108909
- González, M., Brito, N., & González, C. (2014). Identification of glycoproteins secreted by wild-type Botrytis cinerea and by protein O-mannosyltransferase mutants. BMC Microbiology, 14(1), 254. https://doi.org/10.1186/s12866-014-0254-y
- González, V. E., Liñeiro, E., Colby, T., Harzen, A., Garrido, C., Cantoral, J. M., & Fernández-Acero, F. J. (2015). Proteomic profiling of Botrytis cinerea conidial germination. Archives of Microbiology, 197(2), 117–133. https://doi.org/10.1007/s00203-014-1029-4
- Gordo, D. A. M. (2018). Los compuestos fenólicos, un acercamiento a su biosíntesis, síntesis y actividad biológica. Revista de Investigación Agraria y Ambiental, 9(1), 81–104. https://doi.org/10.22490/21456453.1968
- Graca, A., Esteves, E., Nunes, C., Abadias, M., & Quintas, C. (2017). Microbiological quality and safety of minimally processed fruits in the marketplace of Southern Portugal. Food Control, 73(Part B), 775–783. https://doi.org/10.1016/j.foodcont.2016.09.046
- Hurtado, A., Guàrdia, M. D., Picouet, P., Jofré, A., Bañón, S., & Ros, J. M. (2019). Extended shelf life of multi-vegetable smoothies by high-pressure processing compared to heat treatment. Part II: Retention of selected nutrients and sensory quality. Journal of Food Processing and Preservation, 43(11), e14210. https://doi.org/10.1111/jfpp.14210
- Hurtado, A., Guàrdia, M. D., Picouet, P., Jofré, A., Ros, J. M., & Bañón, S. (2017). Stabilization of red fruit-based smoothies by high-pressure processing. Part II: Effects on sensory quality and selected nutrients. Journal of the Science of Food and Agriculture, 97(3), 777–783. https://doi.org/10.1002/jsfa.7795
- Irakli, M. N., Samanidou, V. F., Biliaderis, C. G., & Papadoyannis, I. N. (2012). Simultaneous determination of phenolic acids and flavonoids in rice using solid‐phase extraction and RP‐HPLC with photodiode array detection. Journal of Separation Science, 35(13), 1603–1611. https://doi.org/10.1002/jssc.201200140
- Jacobo Velázquez, D. A., Santana-Gálvez, J., & Cisneros-Zevallos, L. (2021). Designing next-generation functional food and beverages: Combining non-thermal processing technologies and postharvest abiotic stresses. Food Engineering Reviews, 13(3), 592–600. https://doi.org/10.1007/s12393-020-09244-x
- Jang, A. R., Han, A., Lee, S., Jo, S., Song, H., Kim, D., & Lee, S.-Y. (2021). Evaluation of microbiological quality and safety of fresh-cut fruit products at retail levels in Korea. Food Science Biotechnology, 30(30), 1393–1401. https://doi.org/10.1007/s10068-021-00974-0
- Javanmardi, Z., Saba, M. K., Nourbakhsh, H., & Amini, J. (2023). Efficiency of nanoemulsion of essential oils to control Botrytis cinerea on strawberry surface and prolong fruit shelf life. International Journal of Food Microbiology, 384, 109979. https://doi.org/10.1016/j.ijfoodmicro.2022.109979
- León López, L., Guzmán-Ortíz, D. L. A., García Berumen, J. A., Chávez Marmolejo, C. G., & Peña-Cabriales, J. J. (2014). Considerations for improving competitiveness of the “El Bajío” region in domestic strawberry production. Revista Mexicana de Ciencias Agrícolas, 5(4), 673–686. https://doi.org/10.29312/remexca.v5i4.929
- Liang, D. (2018). A salutary role of reactive oxygen species in intercellular tunnel-mediated communication. Frontiers in Cell and Developmental Biology, 6, 2. https://doi.org/10.3389/fcell.2018.00002
- Luck, E., & Jager, M. (2000). Conservación química de los alimentos: Características, usos, efectos (2nd ed., pp. 346). Editorial Acribia. https://books.google.com.mx/books/about/Conservaci%C3%B3n_qu%C3%ADmica_de_los_alimentos.html?id=au0TPwAACAAJ&redir_esc=y
- Luu-Thi, H., Grauwet, T., Vervoort, L., Hendrickx, M., & Michiels, C. W. (2014). Kinetic study of Bacillus cereus spore inactivation by high pressure high temperature treatment. Innovative Food Science & Emerging Technologies, 26, 12–17. https://doi.org/10.1016/j.ifset.2014.07.005
- Marszałek, K., Woźniak, Ł., Skąpska, S., & Mitek, M. (2017). High pressure processing and thermal pasteurization of strawberry purée: Quality parameters and shelf-life evaluation during cold storage. Journal of Food Science and Technology, 54(3), 832–841. https://doi.org/10.1007/s13197-017-2529-4
- Mohammadzadeh-Aghdash, H., Sohrabi, Y., Mohammadi, A., Shanehbandi, D., Dehghan, P., & Dolatabadi, J. E. N. (2018). Safety assessment of sodium acetate, sodium diacetate and potassium sorbate food additives. Food Chemistry, 257, 211–215. https://doi.org/10.1016/j.foodchem.2018.03.020
- Nakata, Y., & Izumi, H. (2020). Microbiological and quality responses of strawberry fruit to high CO2 controlled atmosphere and modifies atmosphere storage. HortScience, 55(3), 386–391. https://doi.org/10.21273/HORTSCI14771-19
- Navarro-Baez, J. E., Martínez, L. M., Welti-Chanes, J., Buitimea-Cantúa, G. V., & Escobedo-Avellaneda, Z. (2022). High hydrostatic pressure to increase the biosynthesis and extraction of phenolic compounds in food: A review. Molecules, 27(5), 1502. https://doi.org/10.3390/molecules27051502
- Nazir, M., Arif, S., Khan, R. S., Nazir, W., Khalid, N., & Maqsood, S. (2019). Opportunities and challenges for functional and medicinal beverages: Current and future trends. Trends in Food Science & Technology, 88, 513–526. https://doi.org/10.1016/j.tifs.2019.04.011
- Official Mexican Standard (NOM). (2002a). NOM-213-SSA1 - Products and services. Processed meat products. Sanitary specifications. test methods.
- Official Mexican Standard (NOM). (2002b). NOM-FF-062 - on industrialized food products for human consumption - fresh fruit - strawberry (Fragaria x ananassa, Dutch) - specifications and test method.
- Ortega, V. G., Ramírez, J. A., Velázquez, G., Tovar, B., Mata, M., & Montalvo, E. (2013). Effect of high hydrostatic pressure on antioxidant content of Ataulfo mango during postharvest maturation. Food Science & Technology, 33(3), 561–568. https://doi.org/10.1590/S0101-20612013005000062
- Pacheco, A., Rodríguez‐Sánchez, D. G., Villarreal‐Lara, R., Navarro‐Silva, J. M., Senés‐Guerrero, C., & Hernández‐Brenes, C. (2017). Stability of the antimicrobial activity of acetogenins from avocado seed, under common food processing conditions, against Clostridium sporogenes vegetative cell growth and endospore germination. International Journal of Food Science and Technology, 52(11), 2311–2323. https://doi.org/10.1111/ijfs.13513
- Pimenta, I., Nuñez, S., Martínez-Blázquez, J. A., Tomás-Barberán, F. A., Perrone, D., & Monteiro, M. (2020). Effect of high hydrostatic pressure and drying methods on phenolic compounds profile of jabuticaba (Myrciaria jaboticaba) peel and seed. Food Chemistry, 309(2020), 125794. https://doi.org/10.1016/j.foodchem.2019.125794
- Pinto, C. A., Moreira, S. A., Fidalgo, L. G., Inácio, R. S., Barba, F. J., & Saraiva, J. A. (2020). Effects of high‐pressure processing on fungi spores: Factors affecting spore germination and inactivation and impact on ultrastructure. Comprehensive Reviews in Food Science and Food Safety, 19(2), 553–573. https://doi.org/10.1111/1541-4337.12534
- Pravallika, K., & Chakraborty, S. (2022). Effect of nonthermal technologies on the shelf life of fruits and their products: A review on the recent trends. Applied Food Research, 2(2), 100229. https://doi.org/10.1016/j.afres.2022.100229
- Quispe, J., Saldaña, J., Verde, T., & Valderrama, S. (2010). Effect of potassium sorbate at different concentrations and exposure time on the cell cycle and the genetic material in root meristems of Allium cepa L “onion”. Revista del Encuentro Científico Internacional, 7(1), 71–78. https://revistaeciperu.com/wp-content/uploads/2019/01/20100011.pdf
- Rodríguez Roque, M. J., de Ancos, B., Sánchez-Moreno, C., Cano, M. P., Elez-Martínez, P., & Martín-Belloso, O. (2015). Impact of food matrix and processing on the in vitro bioaccessibility of vitamin C, phenolic compounds, and hydrophilic antioxidant activity from fruit juice-based beverages. Journal of Functional Foods, 14, 33–43. https://doi.org/10.1016/j.jff.2015.01.020
- Rodríguez Sánchez, D. G., Pacheco, A., García-Cruz, M. I., Gutierrez-Uribe, J. A., Benavides-Lozano, J. A., & Hernandez-Brenes, C. (2013). Isolation and structure elucidation of avocado seed (Persea americana) lipid derivatives that inhibit Clostridium sporogenes endospore germination. Journal of Agricultural and Food Chemistry, 61(30), 7403–7411. https://doi.org/10.1021/jf401407s
- Rodríguez Sánchez, D. G., Pacheco, A., Villarreal Lara, R., Ramos-González, M. R., Ramos-Parra, P. A., Granados-Principal, S., Hernández-Brenes, C., García-Rivas, G., & Hernández-Brenes, C. (2019). Chemical profile and safety assessment of a food-grade acetogenin-enriched antimicrobial extract from avocado seed. Molecules, 24(13), 2354. https://doi.org/10.3390/molecules24132354
- Salinas, C., Hernández, C., Rodríguez, D. G., Castillo, E., Navarro, J., & Pacheco, A. (2017). Inhibitory activity of avocado seed fatty acid derivatives (acetogenins) against Listeria monocytogenes. Journal of Food Science, 82(1), 134–144. https://doi.org/10.1111/1750-3841.13553
- Sarker, M. R., Akhtar, S., Torres, J. A., & Paredes-Sabja, D. (2015). High hydrostatic pressure-induced inactivation of bacterial spores. Critical Reviews in Microbiology, 41(1), 18–26. https://doi.org/10.3109/1040841X.2013.788475
- Sawant, S. G., & Gawai, D. U. (2011). Effect of fungal infections on the nutritional value of papaya fruits. Current Botany, 2(1). https://updatepublishing.com/journal/index.php/cb/article/view/1312
- Silva, F. V. M., & Evelyn, E. (2020). Resistant molds as pasteurization target for cold distributed high pressure and heat assisted high pressure processed fruit products. Journal of Food Engineering, 282, 109998. https://doi.org/10.1016/j.jfoodeng.2020.109998
- Skrede, G., Wrolstad, R., Lea, P., & Enersen, G. (1992). Color stability of strawberry and blackcurrant syrups. Journal of Food Science, 57(1), 172–177. https://doi.org/10.1111/j.1365-2621.1992.tb05449.x
- Ślesak, I., Libik, M., Karpinska, B., Karpinski, S., & Miszalski, Z. (2007). The role of hydrogen peroxide in regulation of plant metabolism and cellular signalling in response to environmental stresses. Acta Biochimica Polonica, 54(1), 39–50. https://doi.org/10.18388/abp.2007_3267
- Song, B., Zhu, P., Zhang, Y., Ju, N., Si, X., Pang, X., & Zhang, S. (2022). Preparation and quality assessment of cream cheese processed by combined high hydrostatic pressure thermal processing and spore-induced germination. Journal of Food Engineering, 341, 111319. https://doi.org/10.1016/j.jfoodeng.2022.111319
- Szczepańska, J., Barba, F. J., Skąpska, S., & Marszałek, K. (2020). High pressure processing of carrot juice: Effect of static and multi-pulsed pressure on the polyphenolic pro le, oxidoreductase activity and colour. Food Chemistry, 307, 125549. https://doi.org/10.1016/j.foodchem.2019.125549
- Teribia, N., Buvé, C., Bonerz, D., Aschoff, J., Hendrickx, M., & Van Loey, A. (2021). Impact of processing and storage conditions on color stability of strawberry puree: The role of PPO reactions revisited. Journal of Food Engineering, 294, 110402. https://doi.org/10.1016/j.jfoodeng.2020.110402
- Ugolini, L., Martini, C., Lazzeri, L., & D’avino, L. (2014). Control of postharvest gray mold (Botrytis cinerea Per.: Fr.) on strawberries by glucosinolate-derived allyl-isothiocyanate treatments. Postharvest Biology and Technology, 90, 34–39. https://doi.org/10.1016/j.postharvbio.2013.12.002
- Van Leeuwen, M., Krijgsheld, P., Bleichrodt, R., Menke, H., Stam, H., Stark, J., Wösten, H. A. B., & Dijksterhuis, J. (2013). Germination of conidia of Aspergillus niger is accompanied by major changes in RNA profiles. Studies in Mycology, 74, 59–70. https://doi.org/10.3114/sim0009
- Vázquez Gutiérrez, J. L., Plaza, L., Hernando, I., Sánchez-Moreno, C., Quiles, A., De Ancos, B., & Cano, M. P. (2013). Changes in the structure and antioxidant properties of onions by high pressure treatment. Food & Function, 4(4), 586–591. https://doi.org/10.1039/c3fo30253a
- Vidal, A. M. (2010). Respuestas fisiológicas de los cítricos sometidos a condiciones de estrés biótico y abiótico. Aspectos comunes y específicos [ Tesis Doctoral]. Universitat Jaume I. http://hdl.handle.net/10803/22656
- Villa-Rojas, R., Sosa-Morales, M. E., López-Malo, A., & Tang, J. (2012). Thermal inactivation of Botrytis cinerea conidia in synthetic medium and strawberry puree. International Journal of Food Microbiology, 155(3), 269–272. https://doi.org/10.1016/j.ijfoodmicro.2012.02.021
- Villarreal Lara, R., Rodríguez-Sánchez, D. G., Díaz De La Garza, R. I., García-Cruz, M. I., Castillo, A., Pacheco, A., & Hernández-Brenes, C. (2019). Purified avocado seed acetogenins: Antimicrobial spectrum and complete inhibition of Listeria monocytogenes in a refrigerated food matrix. CyTA-Journal of Food, 17(1), 228–239. https://doi.org/10.1080/19476337.2019.1575908
- Wang, Q., Chen, X., Chai, X., Xue, D., Zheng, W., Shi, Y., & Wang, A. (2019). The involvement of jasmonic acid, ethylene, and salicylic acid in the signaling pathway of Clonostachys rosea-Induced resistance to gray mold disease in tomato. Phytopathology, 109(7), 1102–1114. https://doi.org/10.1094/PHYTO-01-19-0025-R
- Wang, M., Jiang, N., Wang, Y., Jiang, D., & Feng, X. (2017). Characterization of phenolic compounds from early and late ripening sweet cherries and their antioxidant and antifungal activities. Journal of Agricultural and Food Chemistry, 65(26), 5413–5420. https://doi.org/10.1021/acs.jafc.7b01409
- Xi, J., & Luo, S. (2016). The mechanism for enhancing extraction of ferulic acid from Radix Angelica sinensis by high hydrostatic pressure. Separation and Purification Technology, 165, 208–213. https://doi.org/10.1016/j.seppur.2016.04.011
- Xu, X. H., Jiang, Z. L., Feng, F. Q., & Lu, R. R. (2018). Mechanisms of Nα-lauroyl arginate ethyl ester against Penicillium digitatum and Pectobacterium carotovorum subsp. carotovorum. Journal of Food Science and Technology, 55(9), 3675–3682. https://doi.org/10.1007/s13197-018-3296-6
- Yasunaga, E., Fukuda, S., Takata, D., Spreer, W., Sardsud, V., & Nakano, K. (2018). Quality changes in fresh mango fruits (Mangifera indica L. ‘Nam Dok Mai’) under actual distribution temperature profile from Thailand to Japan. Environmental Control in Biology, 56(2), 45–49. https://doi.org/10.2525/ecb.56.45
- Zhang, W., Liang, L., Pan, X., Lao, F., Liao, X., & Wu, J. (2021). Alterations of phenolic compounds in red raspberry juice induced by high-hydrostatic-pressure and high-temperature short-time processing. Innovative Food Science & Emerging Technologies, 67, 102569. https://doi.org/10.1016/j.ifset.2020.102569