ABSTRACT
Superfine grinding is a cutting-edge food powder processing technology that produces particle sizes as small as the nano-level, resulting in products with exceptional characteristics. This review evaluates the potential benefits of superfine grinding in modifying functional properties, enhancing nutritional qualities, antioxidant activities, and sensory acceptability of food products. The technology has proven useful in producing functional foods, pharmaceuticals, nutraceuticals, and instant foods. By reducing particle size, superfine grinding increases surface area, resulting in powders that disperse easily, have excellent infusion properties, and release more efficient bioactive substances. Superfine grinding technology is vital for modifying functional properties and improving the bioavailability of nutrients and antioxidants, which conventional grinding methods cannot achieve. Moreover, superfine ground products have better sensory acceptability than their coarse counterparts. However, more research is needed to determine the effects of superfine grinding on anti-nutritional factors, heat-sensitive vitamins, and minerals in food products.
1. Introduction
Particle size is a vital parameter that governs the functional property, the release of the active components, of the flour (Xiao et al., Citation2017). It is also used in the quality evaluation of end products. Grinding is an important process during powder production, and it is regarded as one of the effective ways to reduce the problem related to a large particle size of bran and germ for a consumer (Niu et al., Citation2014). Superfine grinding is one of the most effective techniques to produce micro, submicron, or nano (100 μm–1 nm) powders (Gao et al., Citation2020a), which are utilized to make convenient and ready-to-use foods. The food processing industry uses a variety of grinding techniques today to reduce particle size and form a powder (Huang et al., Citation2018; Zhao et al., Citation2010) depending on the nature of raw materials and the desired product characteristics (Balasubramanian et al., Citation2012).
It is challenging to obtain the superfine powder’s particle size of less than 25 µm using traditional milling techniques (Hu et al., Citation2012; Zhang et al., Citation2010). shows comparisons between superfine and conventionally ground food powders for different grinding durations.
Figure 1. Scanning electron microscope image of superfine and conventionally ground food powders. (a) – food powder from conventional grinding; (b) – food powder from 1 min superfine grinding; (c) – food powder from 5 min superfine grinding; (d) – food powder from 10 min superfine grinding (source: Zhang et al., Citation2021).
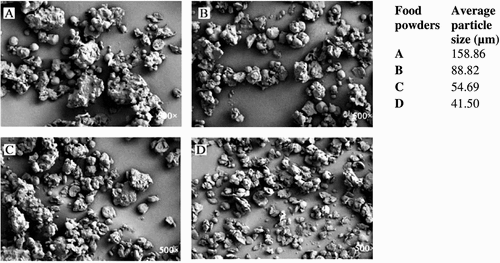
Superfine grinding is an emerging technology that reduces particle size and modifies surface properties (Hu et al., Citation2012). It is a novel processing method that is capable of degrading the organization of plant materials and thereby modifying the performance and the release of nutrients (Gao et al., Citation2020a).
To boost the use of dietary fibre in food preparation and enhance product nutritional quality through decreasing particle size, the food manufacturing industries are utilising superfine grinding technology (Niu et al., Citation2014; Wu et al., Citation2012). Improved surface properties, superior food properties, higher water solubility and dispersibility, increased bioactive contents, and improved antioxidant activity are just a few of the distinctive qualities of superfine powdered powder (Zhang et al., Citation2014). The qualities of the powder are changed by superfine grinding technology, resulting in the production of superior attributes that are not present in coarse powder (Zhang et al., Citation2005). The reduced particle size of Asparagus pomace during superfine grinding treatment resulted in good physicochemical properties (Gao et al., Citation2020b). Various research findings have proven that superfine grinding improves nutritional quality and alters the functional properties of the powder; superfine ground okra powders demonstrated good cholesterol assimilation potential compared with the traditional milled powder (Chen et al., Citation2015b; Eke-Ejiofor et al., Citation2021). Superfine ground powder of wheat bran and okra has been reported to have better functional properties and flowability (Chen et al., Citation2015b, He et al., Citation2018; Zhao et al., Citation2010).
When compared to coarse particles, the physicochemical qualities are often improved by the reduction of particle size during the superfine grinding process (Meng et al., Citation2019). The superfine grinding process enhances bioactive compounds’ availability by changing the bioactive components’ molecular weight and behaviour of food powders (Chen et al., Citation2015a, He et al., Citation2018; Zhu et al., Citation2015). With the lowering of particle size distribution during superfine grinding, the antioxidant content of whole wheat flour was increased (for instance, phenolic acids, anthocyanins, and carotenoid concentrations) (Brewer et al., Citation2014).
Food powder’s physicochemical characteristics, antioxidant activity, and mineral profiles are significantly altered by superfine grinding (Wu et al., Citation2021). Superfine ground items can be easily absorbed by the body while also enhancing the quality and safety of food products (Zhu et al., Citation2010). It is widely used to extract carbohydrates with greater extraction performance and increased antioxidant properties from various biosources (Chun-Yu et al., Citation2004; Hu et al., Citation2012; Zhang et al., Citation2014). Many fields, including biotechnology, the pharmaceutical sector, and the food processing business, use superfine grinding technology (Zhao et al., Citation2009; Zhong et al., Citation2016). According to Zhang et al. (Citation2014), superfine grinding offers enormous potential for use in the production of functional foods and medications. This review was initiated to assess available literature regarding the importance of superfine grinding technology in improving functional properties, nutritional and sensory quality, and bioavailability and antioxidant capacities of ground products. Furthermore, the review was intended to synthesize information on applications of superfine grinding technologies in various disciplines.
2. Survey methodology
In this review, a search strategy of scientific journal articles on superfine grinding of food powder was developed by consulting and collating materials from Google Scholar, Scopus, and Web of Science. The available literature concerning the impacts of superfine grinding on functional properties, nutritional quality, sensory quality, and antioxidant contents of the powder was gathered. Furthermore, information factors that affect the superfine processing conditions were assessed and compiled. Superfine grinding was a keyword used to collect information based on relevancy and year of publication (papers that have been published over the last 20 years were included). The data collected were critically reviewed and arranged systematically with 108 references.
3. Superfine grinding technology
Superfine grinding refers to the process of reducing the material particle size to the microlevel using mechanical equipment (Chen et al., Citation2018). Various grinding technologies are used in superfine grinding depending on the nature of raw materials and the desired product characteristics (Balasubramanian et al., Citation2012). Different superfine grinding technologies have been developed based on materials, such as cryogenic grinding, vacuum grinding, and roller grinding (Chen et al., Citation2018), shear crushing, and airflow comminution (Xu et al., Citation2021; Zhang et al., Citation2023), jet milling (Hou et al., Citation2023; Wu et al., Citation2012; Zhong et al., Citation2016), universal pulverizer (Gong et al., Citation2022), vibrating mill (Yan et al., Citation2023) and ball mill (Zhang et al., Citation2020; Zhao et al., Citation2023) are equipment widely applied in superfine grinding among the device reported for grinding to superfine level. Different superfine grinding techniques have been shown to exhibit different morphological properties with some particles appearing broken, ovoid, deformed and irregular in shape () (Yu et al., Citation2023). Ball milling is the most commonly reported superfine grinding device (Huang et al., Citation2020; Sun et al., Citation2015; Wang et al., Citation2020; Zhang et al., Citation2020; Zhao et al., Citation2023). Overall the above-mentioned grinding methods are used for reducing the particle size of solid materials, high-pressure homogenization, ultrasonic homogenization, and micro fluidization are used for liquid particle reduction (Chen et al., Citation2018; Gao et al., Citation2020a). The superfine grinding methods were reviewed in detail (Chen et al., Citation2018; Gao et al., Citation2020a).
Figure 2. Scanning electron micrographs of different superfine ground mung bean flour. (a) – crushing machine, (b) – ball mill, and (c) – Vibratory mill (adapted from Yu et al., Citation2023).
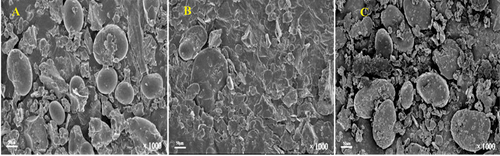
The different milling methods (high-speed universal grinder, wet-milling, and a stone mill) resulted in powder with different physicochemical properties due to differences in milling conditions, including mechanical forces, milling intensity, temperature, and time (Yu et al., Citation2018). For example, jet milling involves grinding with pressure, collision, and abrasion by high-pressure airflow, which was produced from compressed air expansion through the nozzle (Zhong et al., Citation2016), while MZF-4 L impact superfine grinder reduces particle size by the collision among the steel bars or between the steel bars and the inner wall of the grinding bow (Niu et al., Citation2014). The ball milling process reduces particle size by subjecting the product to be ground for pressure collision and abrasion between the grinding balls and the inner bottom of the jar (Meng et al., Citation2017; Zhang et al., Citation2014).
Furthermore, high-pressure homogenization generates intense turbulence, vibration, cavitation, and hydraulic shear. These powerful forces can break microdroplets into nanodroplets (Zhang & Haque, Citation2015). Microfluidization on the other hand is a synergistic processing mechanism involving hydrodynamic cavitation, intense shear rates, ultrahigh pressure, instantaneous pressure drops, high-velocity impact forces, and high-frequency vibrations, all achieved within a short treatment time (McClements & Rao, Citation2011; Mert, Citation2020). Ultrasonic homogenization involves the utilization of high-intensity ultrasonic waves to create strong shear and pressure variations, leading to the disruption of emulsion droplets (Chen et al., Citation2018). This disruption of droplets is primarily due to the occurrence of cavitation. Ultrasonic homogenization effectively reduces the size of already existing emulsions.
While selecting a superfine grinding milling technique, several factors such as the nature of the material, the material’s properties (hardness, brittleness, heat sensitivity), required particle size distribution, potential contamination, throughput requirements, production capacity, and energy efficiency are taken into account to achieve the desired output. In other words, the application of a specific superfine grinding method is dependent exclusively on the material and the operational goals. For instance, ball milling is suitable for grinding hard and brittle materials such as minerals, metals, and ceramics (Zhang et al., Citation2020; Zhao et al., Citation2023). It can also be used to grind non-heat-sensitive or low melting point food materials such as grains and legumes to achieve desired particle size. It is widely used in the production of fine powders in the food, electronics, metallurgical, and/or ceramic industries (Wang et al., Citation2020). Furthermore, vibration milling is appropriate for grinding heat-sensitive materials or products that require minimal contamination such as pigments, seeds, dried vegetables, and pharmaceuticals to preserve sensory qualities such as colour and taste (Baláž, Citation2003; Min et al., Citation2021; Yan et al., Citation2023). Similarly, cryogenic milling is often employed to grind heat-sensitive materials such as coffee beans, spices, and certain nuts by reducing the temperature of the food material with liquid nitrogen in order to preserve and maintain its quality (aroma, flavour, and essential oil) (Balasubramanian et al., Citation2012; Chen et al., Citation2018; Ghodki & Goswami, Citation2016). Also, it is evident that the choice of milling technique between wet and dry superfine grinding demonstrates a distinct preference for certain raw materials and outcomes (desired particle size). This implies that raw materials or products can only be processed via a selected method specific to the desired preference.
4. Working principles of superfine grinding
Size reduction from micron to nanoscale has recently been used in the food industry and is a rapidly evolving technology (Chen et al., Citation2018). The surface area increases as a result of superfine grinding, which reduces the size of the food powder particle. Moreover, the breaking down of cell walls during the grinding process boosts nutrient bioactivity and extraction (Zhao et al., Citation2014). An environmental Scanning Electron Microscopy (SEM) study has revealed that the mechanical shear stress during superfine grinding plays a significant influence in the degradation of the Sanchi flower powders and results in an increase in surface area (Wu et al., Citation2021). The mechanical force used in the superfine grinding process may cause chemical compositional changes, including increases in the surface area to volume ratio, the rupturing of the material’s cell wall, which increases accessibility, the release of biologically active compounds, and changes in dietetic ingredients (Waleed Aboshora, Citation2016). The application of mechanical force during the superfine grinding process of chitin causes the degradation of intermolecular bonds between chitin molecules, leading to the release of bioactive compounds (Zhang et al., Citation2020). Moreover, the reactive surface is increased by the smaller particle size to a greater extent (Jin & Chen, Citation2006). Smaller particle size, which improves mass transfer and enables the synthesis of extracts with more bioactive chemicals, favours the improvement in the quality of the superfine ground powder (Cacace & Mazza, Citation2003; Rosa et al., Citation2013).
The evidence suggests that the mechanical pressure used during the grinding process is mostly to blame for the breakdown of the food ingredients’ cell walls. The release of active chemicals and improvement in nutritional and sensory quality is caused by an increase in surface area and a reduction in particle size. The working principles of how superfine grinding improves quality are summarized in below.
5. Advantage of superfine grinding over conventional grinding
Superfine grinding is an emerging milling technology that has outstanding contributions to the production of functional food products and nutraceuticals (Chen et al., Citation2006) which cannot be achieved using conventional grinding. Superfine grinding has numerous advantages; it significantly improves the capacity to maintain high-quality, nutrient-rich, and biologically active substances, and is effective in promoting the release of cellular nutrition, which can be readily assimilated by the human body (Gao et al., Citation2013; Zhang et al., Citation2014; Zhu et al., Citation2014). Also, the decrease of different food components’ particle sizes to the micro- or nanoscale causes some modifications to their structure and surface area as well as the introduction of some new, standout properties. These qualities include surface effects, mini-size effects, macro quantum channel effects, optical, magnetic, mechanical, chemical, and catalytic capabilities, which may have new uses in both academia and industry (Huang et al., Citation2018, Citation2008; Niu et al., Citation2014; Zhao et al., Citation2009, Citation2010).
The efficacy of superfine grinding technology in extracting functional components today is of tremendous interest, primarily due to its ability to save time, solvent, and energy. In addition, superfine grinding can significantly increase the efficiency of the extraction and its eco-friendly powder processing technology (Xu et al., Citation2021). Furthermore, superfine grinding technology was reported to consume less energy than traditional mechanical grinding (Wu et al., Citation2012). Compared to powder samples from the ordinary or traditional grinding procedure, superfine ground powder has demonstrated good physical properties such as lowering the interfacial tension and influencing a steric hindrance (Zhu et al., Citation2011). The superfine ground powder has demonstrated altered physicochemical properties, including enhanced hydration and fluidity, increased in vivo or in vitro bioavailability and bioactivity, stronger radical scavenging activity, and minimal interfacial tension (Hu et al., Citation2012; Ofoedu et al., Citation2021; Zhong et al., Citation2016), and good flavour release and mouthfeel (Muttakin et al., Citation2015). In addition to boosting its functional and other nutritional attributes, superfine grinding can enhance the cooking characteristics, cohesion, toughness, and modify the microstructure of whole wheat (Niu et al., Citation2014). Also, the superfine ground powder is simple to mix into food structures and has a higher added value than products made using conventional milling techniques (Chen et al., Citation2018; Ihediohanma et al., Citation2014; Ming et al., Citation2015; Ofoedu et al., Citation2020). Besides superfine grinding being an eco-friendly technology with less energy consumption, generally, the available literature revealed that superfine grinding has a lot of advantages over conventional grinding in improving the functional and nutritional quality of the food powder.
6. Factors affecting superfine grinding
The superfine grinding process is affected by various factors like the temperature, grinding equipment/device used, nature of the material to be ground, and grinding conditions (wet/dry, time, temperature, and speed). According to Augusto-Obara et al. (Citation2019), superfine grinding processes are influenced by the chemical composition of the (food) raw material, its physical properties, and the superfine grinding technique used. Grinding time is one of the factors that affect the quality of superfine ground products. Li et al. (Citation2020) reported that too long superfine grinding time would damage soluble dietary fibers and carbohydrate contents of the powders. Rizlan and Mamat (Citation2014) also reported that different operational variables such as the milling speed, time, and volume of the milling media influence the superfine grinding process. Cui et al. (Citation2019) also reported that the extended time of superfine grinding from 15 to 60 min resulted in a decreased particle size of pineapple leaf fiber powders from 41.51 to 18.209 µm respectively.
The finding of Hemery et al. (Citation2011) indicated that the temperature of ultrafine and superfine grinding has shown significant effects on wheat bran functional properties. According to Ghodki and Goswami (Citation2016) on the effect of temperature on superfine grinding of food products (black pepper), the fineness of the powder attained at a lower grinding temperature of −120°C was relatively higher as compared to that of powder at 40°C. The specific surface area of the powder particles was also observed to increase significantly with the decrease in the grinding temperature (40 to − 120°C). The powder obtained at the lowest grinding temperature has the highest flavourings and mineral compositions with the least energy requirement for the cryogenic milling process. Temperature control during the superfine grinding process is important because it affects the quality of the final product. For instance, if the temperature is too high, it can cause damage to the nutrients and flavour of the food product. On the other hand, if the temperature is too low, it can affect the grinding efficiency and the quality of the final product. Therefore, selecting the best grinding temperature according to different raw materials is crucial to achieving the desired grinding fineness of the food product (Yang et al., Citation2016).
Furthermore, the intrinsic characteristics of bran could be affected by grinding behaviour and grinding methods used for the superfine grinding process. Xu et al. (Citation2021) compared the effects of different grinding methods (wet grinding, airflow comminution, and shear crushing) on buckwheat bran properties. The authors’ findings demonstrated that wet grinding exhibited the highest protein, superior water-holding capacity, solubility, and highest bulk, and tap densities than shear crushing and airflow comminution. Similar findings were also reported by Ihediohanma et al. (Citation2014). Hu et al. (Citation2012) suggested that heat control during superfine grinding is important to maintain the green tea powder quality, in addition to controlling superfine grinding duration within 30–50 min. Zhao et al. (Citation2015) suggested that low temperature and short-time superfine grinding treatment may not reduce the antioxidant (polyphenol and flavanol) contents of grape pomace powders. The finding of Zhu et al. (Citation2015) indicated that superfine grinding can affect the macromolecule matrix and result in changes in some phenolic compounds. Gao et al. (Citation2020b) confirmed that the decrease in particle size as a result of the superfine grinding process may not improve all parameters consistently, suggesting that size reduction should be determined according to the target parameters. Sun et al. (Citation2019) reported that monitoring the degree of grinding is required as it can affect the functional and antioxidant contents of a powder. The study report highlighted that various processing parameters such as types of superfine grinding methods used, processing time and temperature, degree of grinding and the nature of raw material to be ground can influence the superfine grinding processes. Therefore, there is a need to optimize the processing conditions of different food products for the enhanced nutritional quality of the superfine ground food powders.
7. Applications of superfine grinding technology
The superfine grinding technique has shown potential for application in many disciplines (Wu et al., Citation2012). In a wide range of industries, including paper making, ceramics, pharmaceutics, electronic materials, and chemicals, superfine grinding is used to reduce particle size and improve reactive surfaces. The development of new functional materials for a variety of industries, including biotechnology and food products, uses superfine grinding (Rajkhowa et al., Citation2008; Zhang et al., Citation2005). The production of nutraceuticals, pharmaceuticals, health foods, component extraction, and animal feeding have all shown the potential for using superfine grinding technology (Chen et al., Citation2006; Sun et al., Citation2019; Xu et al., Citation2018). According to Ming et al. (Citation2015), super-finely ground L. edodes powders can be used as food or pharmaceutical additives in a variety of industries. Meng et al. (Citation2017) reported that the smaller particle size of the superfine ground D. Officinale products can be easier to fill into food; hence, the superfine ground powder might be used in nutraceuticals and medicines.
As this type of size reduction has shown potential use in the biomedical and food manufacturing industries, superfine grinding technology has attracted enormous interest (Muttakin et al., Citation2015). According to Liu et al.’s (Citation2016) study on oat bran polysaccharides, there is a good chance that functional foods will be produced using superfine grinding technology. The solubility and nutritional value of Taraxacum mongolicum powder were improved after the process of superfine grinding, and the human body can now easily absorb it (Song et al., Citation2002). The results of Zhao et al. (Citation2015) were similar in that they suggested that superfine powdered grape pomace powders might be employed as a source of natural ingredients for food and nutraceuticals. The manufacture of functional foods, pharmaceuticals, nutraceuticals, and rapid food processing can all benefit from the use of superfine grinding technology. Overall, super grinding technology can be used in a variety of fields, including medicine, biotechnology, nutraceuticals, feed processing, and the food sector.
8. Influence of superfine grinding on food powder quality characteristics
Compared to the classic or standard grinding method, superfine grinding creates small particles with remarkable product features, which suggests that superfine grinding has a significant potential for the manufacture of improved functional and nutritional foods (Zhang et al., Citation2014). Compared to the coarse particle produced by normal grinding, superfine grinding has increased the nutritional quality and antioxidant activity of D. officinale, for greater absorption and free radical scavenging capacity (Meng et al., Citation2017). The finding of Hou et al. (Citation2020) has shown that superfine grinding can minimize cooking time, and cooking loss while at the same time enhancing the texture and sensory acceptability of noodles as compared to traditional grinding. Due to this, it will be explored how superfine grinding affects the functional properties, nutritional value, antioxidant characteristics, and sensory qualities of food powders.
8.1. Particle size distribution
The surface area per unit mass of the material increases when particle size is reduced during superfine grinding. This can have an effect on a variety of properties, including reactivity, dissolving rate, and adsorption capacity. Increased surface area can improve performance in some circumstances, such as catalysts or medications designed for fast absorption. Further, superfine grinding has significant impact on food product particle size distribution as indicated in . A more narrow particle size distribution is achieved through superfine grinding. This results in a more consistent range of particle sizes, with fewer particles at the extreme ends of the size spectrum. For instance, the British Standards definition designates the width of the particle size distribution as the “span.” A smaller span value indicates a more uniform size distribution (Chew & Chan, Citation2002). The span values of micronized (superfine ground) Koehne fruit powders were 4.21 lower than that of the coarse powder, indicating that a narrow width of particle size distribution and uniform size had appeared (Zhang et al., Citation2009). The study by Ming et al. (Citation2015) found that superfine grinding significantly reduced the span values for both cap and stipe powders. As a result, the particle size distributions of these powders became narrower and more uniform than those of traditionally pulverized powders. This can be useful in situations where particle size consistency is critical, such as in pharmaceuticals or certain food products. Similarly, Zhao et al. (Citation2009) reported that ginger powder particles become narrow and uniform after superfine grinding and exhibit favourable surface and processing properties. Sun et al. (Citation2015) also reported that superfine grinding reduced the mean particle size of whey protein.
Figure 4. Particle size distribution of superfine ground food products (source: Meng et al., Citation2017).
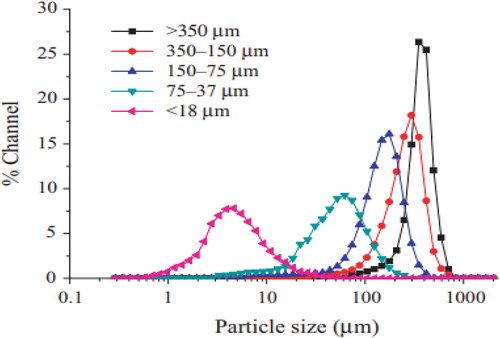
8.2. Functional properties
Functional property is one of the important parameters that could be used to determine the quality of powder in the food industry. The dispersibility, dissolution, and functional properties of superfine powder were modified following the superfine grinding process (Zhong et al., Citation2016). The decrease in particle size resulting from superfine grinding has led to a consistent pattern of decline in bulk and tapped density of powder (Wu et al., Citation2021). The superfine grinding technique considerably enhanced the water holding capacity, emulsion activity index, and emulsion stability index, according to Sun et al. (Citation2016). This improved the visco-elasticity and stability of protein dispersion. According to the research carried out by Li et al. (Citation2020), superfine grinding alters the functional characteristics of soybean residual powder. As a result of the particle size reduction, water holding capacity decreased from 7.86 to 4.39 g/g and oil binding capacity decreased from 1.78 to 1.42 g/g. Particle (as the name of the by-product indicates) is a by-product of grinding. According to Zhao et al. (Citation2015), red grape pomace’s functional qualities (bulk density, tapped density, and fluidity) can decrease as a result of the superfine grinding procedure. In their investigation, Zhong et al. (Citation2016) found that superfine ground powder had a larger bulk density than coarse pomegranate peel particles. This might be because the inter-particle vacuum was reduced as a result of the great uniformity of superfine ground powder particle size (Eke-Ejiofor et al., Citation2021). The superfine ground Lycium ruthenicum Murray powders, according to Zhang et al. (Citation2020), also had a much better water retention capacity and water absorption index than the powder produced by the traditional grinding procedure. During the process of superfine grinding, the bulk density of red rice powder was raised while the particle size was decreased (Chen et al., Citation2015a). The information that is now available shows that the functional (water holding capacity, oil absorption capacity, and water solubility index) qualities of a powder have changed as a result of the smaller particle size produced by superfine grinding.
Moreover, superfine grinding also impacts food products’ cation exchange capacity (CEC). CEC refers to the ability of powdered substances to bind or exchange ions within a solution as the product’s side groups are exposed due to the breakage of fiber chains (Wang et al., Citation2019). Yan et al. (Citation2023) observed that subjecting apples to superfine grinding increased their CEC from 0.07 mmol g − 1 to 0.13 mmol g − 1. Similar findings were reported by Yu et al. (Citation2023) after studying the effect of different superfine grinding methods of mung bean on CEC. The results showed that the exchange capacity of ultrafine ground mung bean flour cations was significantly increased but varied according to the different milling technique employed. The increased CEC was attributed to the increased comminution and mechanical forces that enhanced the exposure of more side chain groups required for the exchange reaction to occur, due to the disruption of the fiber mesh structure by the superfine grinding process (Yu et al., Citation2023).
8.3. Nutritional quality
Reduction in food particle size with the aid of superfine grinding technology has been shown to improve the nutritional value of such food products as well as their cooking qualities (Niu et al., Citation2014). A significant reduction in moisture contents associated with high heat generated during the superfine grinding has been reported (Hou et al., Citation2020). Furthermore, protein and polysaccharide solubilization of the superfine ground powder has significantly improved following superfine treatment (Meng et al., Citation2017; Zhao et al., Citation2010). The finding of Meng et al. (Citation2017) has shown that the decrease in particle size after superfine grinding resulted in a significant increment in protein and polysaccharide solubility and antioxidant activities. Similarly, Song et al. (Citation2022) reported that the total protein and soluble protein contents of superfine ground sprouting quinoa were increased with a decrease in particle size.
According to the findings of Becker et al. (Citation2016), the reduction of particle size of Hypericum perforatum and Achillea millefolium powders from 20 to 500 μm has resulted in decreased carbohydrate contents while other components (lipids, proteins, and ashes) increased. The decreased particle size during the superfine grinding process increases the fat contents of potato residue powder compared with coarse particle size (Waliullah et al., Citation2021). A decrease in particle size increases mineral contents according to Wu et al. (Citation2021), who found that minerals like calcium, sodium, zinc, and manganese have demonstrated a substantial fluctuation in particle size. Moreover, Ghodki and Goswami (Citation2016) showed that a decrease in particle size increased the potassium, calcium, and chlorine concentrations of superfine ground powder. Moreover, the constant rise in mineral availability was a result of the growing grinding temperature.
In contrast, Memon et al. (Citation2020) found that the protein content of wheat cultivars decreased as a result of the reduction in particle size. Similar research supported the finding that many food products’ protein amounts decreased as particle size was reduced (Ahmed, Citation2015; Blanchard et al., Citation2012; de la Hera et al., Citation2013). Particle size has an impact on the total starch contents of potato residual flour; a decrease in particle size leads to an increase in total starch content (Waliullah et al., Citation2021).
Superfine grinding has been shown to impact changes in some dietary components. Lin et al. (Citation2021) reported that superfine grinding caused the rearrangement of insoluble dietary fiber to a soluble fraction compared to coarse bran powder. Also, the superfine grinding process has been found to increase the mineral content of the powder. The breakdown of intact cell walls and reduction of particle size during superfine grinding results in the release of more bonded nutrients like minerals, ash, fat, and polysaccharides. Superfine grinding enhances the nutritional composition of food products by reducing the particle size of food materials to a powder form. This process modifies their chemical composition and disrupts their internal structure (Liu et al., Citation2011; Zhao et al., Citation2009).
8.4. Antioxidant properties
Superfine grinding alters the bioactive characteristics of the ground powder; the solution behaviour, molecular weight of biologically active compounds, and antioxidant properties of the powder were enhanced (Li et al., Citation2020; Zhao et al., Citation2015). It has been reported that superfine grinding contributes to improving the accessibility of anthocyanins and polysaccharides contents of powders. Furthermore, superfine grinding also enhanced the antioxidant (DPPH, ABTS, and FRAP) capacity of Lycium ruthenicum Murray powders (Zhang et al., Citation2020). According to Zhu et al. (Citation2015) ‘s research, superfine grinding improved the bran dietary fibre from Qingke’s total phenolic content, ferric reducing antioxidant power, and DPPH. Furthermore, according to Hu et al. (Citation2012), superfine grinding greatly enhanced the amount of polyphenols that were extracted from green tea, giving it a stronger capacity to scavenge free radicals. The polyphenols and flavonol contents of grape pomace were improved significantly with particle size reduction as a result of superfine grinding, which could enhance the antioxidant activities of the powder (Zhao et al., Citation2015). The decrease of mulberry leaves powder size, up to 10 µm during the superfine grinding process enhanced the contents of bioactive molecules such as polyphenol, and polysaccharide powder (Mena et al., Citation2016). Superfine grinding reduces the particle size and increases the chance of the release of biologically active substances (Ramachandraiah & Chin, Citation2016). The antioxidant activity of superfine powder was rapidly increased as a result of the release of antioxidant components in soybean residual powder as powder size was reduced (Li et al., Citation2020). The antioxidant activity of ginger extracts is greatly impacted by the particle size, according to Ratnawati et al. in 2021. Ginger with the largest particle size has the least antioxidant action. Numerous findings have confirmed that superfine grinding has contributed to the release of a significant quantity of antioxidants (Hu et al., Citation2012; Ramachandraiah & Chin, Citation2016; Zhao et al., Citation2015). On the other hand, Hu et al. (Citation2012) reported that the polyphenols and catechins contents of green tea powder decreased as the tea particle size decreased. This might be due to increasing heat during superfine grinding, types of ingredients, and devices used to grind. Similarly, Sheng et al. (Citation2017) reported the reduction of total phenolic compounds as a result of the superfine grinding treatment of grape pomace due to thermal degradation. The review suggests that the particle size reduction following the superfine grinding process has considerably enhanced the bioactive contents of the powder as indicated in . This might be due to the release of bioactive compounds resulting from the degraded cell wall and reduced particle size of the ground powder.
Table 1. Effects of size reduction on antioxidant contents of products.
8.5. Sensory quality
Superfine grinding not only improves the nutritional quality and functional properties of a product but also the sensory acceptance of the superfine ground product is superior. Reports from various studies indicated that the superfine grinding process can significantly increase the brightness of wheat bran (Jin et al., Citation2020; Niu et al., Citation2014). According to Sun et al. (Citation2016), whey protein concentrate powders brightened significantly and also varied greatly in colour with the decrease in particle size. The sensory acceptability score and texture of noodles prepared from superfine ground wheat and black soybean flour showed higher values than those of conventional grinding (80-mesh four) at similar proportions (Hou et al., Citation2020; Ibeabuchi et al., Citation2017). The objective colour analysis of mung bean powders milled using different superfine grinding equipment (vibratory mill, ball mill and a crushing machine) () showed significant variations in colour values (L*a*b*) as the chroma and hue values appeared distinct (Yu et al., Citation2023).
Figure 5. Colour of mung bean powder under different grinding methods. (a) – crushing machine, (b) – ball mill, and (c) – Vibratory mill (Adapted from Yu et al., Citation2023).
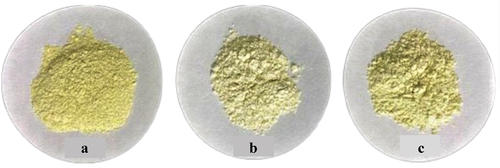
Superfine ground Tremella aurantialba powder had better sensory attributes, sweetness was enhanced with a decline in particle size whereas stickiness, bitterness, and roughness decreased with particle size (Zhao et al., Citation2018a). Sensory evaluation of green tea powder (GTPs) indicated that the colour became greener and lighter and the taste became sweeter and purer with the decrease in particle size of green tea powder during superfine grinding (Hu et al., Citation2012). The improved sensory acceptance of the superfine ground product may be related to the degradation of compounds that impart sensory preference. On the other hand, flavour compounds like aldehydes, alcohols, and alkanes can be released easily from superfine ground products. The degradation of polysaccharides into their simple sugar during superfine treatment can also contribute to the better taste of the products.
9. Effects of superfine grinding on digestibility and bio-accessibility of nutrients
The health benefits of phenolic compounds depend on the amount accessible for assimilation in the upper or lower portion of the digestive tract (bioaccessibility) (Jakobek & Matić, Citation2019; Ribas-Agustí et al., Citation2018). The bioaccessibility of nutrients refers to the amount of nutrients released from the food matrix through enzymatic hydrolysis after digestion (Zhao et al., Citation2018). However, most of the phenolic compounds are in the bound form (Li et al., Citation2022). Furthermore, some other phenolic compounds also are “entrapped” inside the food matrix via hydrogen bonds (Li et al., Citation2022).
Various research findings have confirmed that superfine grinding resulted in significant improvement in the starch digestibility of wheat bran (Li et al., Citation2022); black soybean (Yang et al., Citation2022); navy bean flour (Luhovyy et al., Citation2017); germinated brown rice (Lee et al., Citation2019). The particle size plays a vital role in the delivery of functional properties during in vitro gastrointestinal digestion (Li et al., Citation2022) and improves bioavailability (Shu et al., Citation2019). The digestibility of starch increased as the particle size of purple corn flour decreased from coarse to superfine (Guo et al., Citation2022). The superfine ground wheat bran (19.16 μm) has increased the phenolic compounds’ bioaccessibility more than five times compared to coarse wheat bran (1110.39 μm) (Li et al., Citation2022). Moreover, Hu et al. (Citation2022) reported that the in vitro digestibility of α-Lac and laccase-treated α-Lac was enhanced by 5.77% and 4.47% in the intestine following superfine grinding for 12 h.
Superfine milling is a feasible method to enhance the phenolic compounds’ bioaccessibility in cereals (Li et al., Citation2022). Superfine grinding significantly altered phenolics’ bioaccessibility and bitter melon’s antioxidant potential during in vitro digestion (Zhu et al., Citation2022). Similarly, Gao et al. (Citation2019) reported that after digestion the anthocyanins and polyphenols released from superfine ground downy rose-myrtle berry is significantly higher than the coarse powders. Moreover, the superfine ground rice bran insoluble dietary fiber powder was reported to enhance the phenolic compound bioaccessibility with the phenolic bioaccessibility values of 18.82%, 23.02%, and superfine 32.34% coarse, fine, and superfine, respectively (Zhao et al., Citation2018).
The available information confirms that the increase in the bioaccessibility of phenolic compounds and starch following the super fine-grinding of food products could be associated with the degradation of the cell wall and starch which facilitates water absorption and promotes enzyme activities. In addition, it has been reported that superfine grinding could damage the starch and expose more enzyme-binding sites (Tian et al., Citation2022). The increase in the bioaccessibility of phenolic compounds could be due to the fragmentation of the cell wall matrix and the hydrolysis of digestive enzymes and bile salt (Li et al., Citation2022).
10. Future trends
A wide range of applications for superfine grinding technology remains to be explored, including creating new functional food ingredients, enhancing the bioavailability of bioactive chemicals, changing flavour and aroma, and developing high-quality instant food products.
It is well-known that superfine ground food powders have improved functional properties such as foaming, emulsification, and gelation due to changes in particle size resulting in the development of novel food ingredients. Besides the functional properties, superfine grinding can enhance the nutritional quality of food by improving the bioavailability of bioactive substances (antioxidants, vitamins, and minerals) because of decreased particle size and increased surface area of food powders. For instance, bioactive compounds with antioxidants and anti-inflammatory qualities such as gingerols and curcumin can be easily released when ground into a very fine powder. Similarly, the absorption of minerals, (zinc, calcium, and iron) from diets are significantly improved in foods containing superfine ground food powders. From a sensory perspective, the increased surface area and decreased particle sizes in superfine ground food powders enhance the release of volatile compounds in food, thus improving the food powder’s overall aroma and flavour profile.
As a result of the potential of superfine grinding technology, some of the innovative and promising fields for future research include a.) Developing high production efficiency and low-cost processes and equipment for superfine grinding of different food materials, such as cereals, fruits, vegetables, spices, herbs, etc. b.) Optimizing the product size and specific surface area, chemical composition, crystal shape, surface morphology, defects, roughness and other quality parameters of superfine ground food powders to meet different application requirements. c.) Exploring the effects of superfine grinding on the release and stability of volatile compounds, antioxidants, vitamins, minerals, and other bioactive substances in food powders, and developing methods to enhance their bioavailability and biological activity. d.) Investigating the interactions between superfine ground food powders and other food components, such as water, oil, proteins, polysaccharides, etc., and their impacts on the foaming, emulsification, gelation, solubility, dispersibility, and other functional properties of food systems. e.) Designing novel food products based on superfine ground food powders, such as sauces, confections, dressings, ice cream, whipped toppings, aerated foods, instant foods, etc., with improved sensory quality and consumer acceptance.
11. Conclusion
This review highlighted the superfine grinding technology and its importance in food powder processing. Decreasing particle sizes of food materials to micro or nano levels would alter product characteristics like structure, surface area, physicochemical, and functional properties. Moreover, the superfine grinding treatment can improve bioavailability and bioactivities and enhance flavour release and mouthfeel due to cell wall disintegration, reduced mass transfer resistance and increased reactive surface. Generally, this review revealed that the superfine grinding process can be applied in the food manufacturing industry to decrease the particle size of food materials and consequently enhance the functional properties, nutritional, and sensory quality of the products. Superfine grinding technology has a great potential application in the production of functional food, pharmaceutics, nutraceuticals, and instant food processing. Further investigations on the effects of superfine grinding on anti-nutritional factors, heat-sensitive vitamins, and minerals contents of the superfine ground product are required.
Ethics approval
This study does not involve any human or animal testing.
Acknowledgment
The authors HTD, AOA, CEO, and JSC appreciate the financial support from the Chinese Government Scholarship and South China University of Technology, Guangzhou-Guangdong, China.
Disclosure statement
No potential conflict of interest was reported by the author(s).
Data availability statement
The data that support the findings of this study are available from the corresponding author upon request.
Additional information
Funding
References
- Aboshora, W. (2016). Functional foods: Effect of superfine grinding on functional properties and antioxidant capacities of dietary fiber from cereal bran, fruits and vegetables. Bioaccent Online Publishing Nutrition, 1(1), 1–2. https://doi.org/10.24947/baojn/2/1/00109
- Ahmed, J. (2015). Effect of barley β-glucan concentrate on oscillatory and creep behavior of composite wheat flour dough. Journal of Food Engineering, 152, 85–94. https://doi.org/10.1016/j.jfoodeng.2014.11.018
- Augusto-Obara, T. R., Oliveira, J. D., Gloria, E. M. D., Spoto, M. H. F., Godoy, K., Vieira, T. M. F. D. S., & Scheuermann, E. (2019). Benefits of superfine grinding method on antioxidant and antifungal characteristic of Brazilian green propolis extract. Scientia Agricola, 76(5), 398–404. https://doi.org/10.1590/1678-992X-2018-0056
- Balasubramanian, S., Gupta, M. K., & Singh, K. K. (2012). Cryogenics and its application with reference to spice grinding: A review. Critical Reviews in Food Science and Nutrition, 52(9), 781–794. https://doi.org/10.1080/10408398.2010.509552
- Baláž, P. (2003). Mechanical activation in hydrometallurgy. International Journal of Mineral Processing, 72(1–4), 341–354. https://doi.org/10.1016/S0301-7516(03)00109-1
- Becker, L., Zaiter, A., Petit, J., Zimmer, D., Karam, M. C., Baudelaire, E., Scher, J., & Dicko, A. (2016). Improvement of antioxidant activity and polyphenol content of hypericum perforatum and Achillea millefolium powders using successive grinding and sieving. Industrial Crops and Products, 87, 116–123. https://doi.org/10.1016/j.indcrop.2016.04.036
- Blanchard, C., Labouré, H., Verel, A., & Champion, D. (2012). Study of the impact of wheat flour type, flour particle size and protein content in a cake-like dough: Proton mobility and rheological properties assessment. Journal of Cereal Science, 56(3), 691–698. https://doi.org/10.1016/j.jcs.2012.08.005
- Brewer, L. R., Kubola, J., Siriamornpun, S., Herald, T. J., & Shi, Y. C. (2014). Wheat bran particle size influence on phytochemical extractability and antioxidant properties. Food Chemistry, 152, 483–490. https://doi.org/10.1016/j.foodchem.2013.11.128
- Cacace, J. E., & Mazza, G. (2003). Mass transfer process during extraction of phenolic compounds from milled berries. Journal of Food Engineering, 59(4), 379–389. https://doi.org/10.1016/S0260-8774(02)00497-1
- Chen, Q. M., Fu, M. R., Yue, F. L., & Cheng, Y. Y. (2015b). Effect of superfine grinding on physicochemical properties, antioxidant activity and phenolic content of red rice (Oryza sativa L.). Food and Nutrition Sciences, 6(14), 1277–1284. https://doi.org/10.4236/fns.2015.614133
- Chen, H., Weiss, J., & Shahidi, F. (2006). Nanotechnology in nutraceuticals and functional foods. Food Technology (Chicago), 60(3), 30–36.
- Chen, T., Zhang, M., Bhandari, B., & Yang, Z. (2018). Micronization and nanosizing of particles for an enhanced quality of food: A review. Critical Reviews in Food Science and Nutrition, 58(6), 993–1001. https://doi.org/10.1080/10408398.2016.1236238
- Chen, Y., Zhang, B. C., Sun, Y. H., Zhang, J. G., Sun, H. J., & Wei, Z. J. (2015a). Physicochemical properties and adsorption of cholesterol by okra (Abelmoschus esculentus) powder. Food & Function, 6(12), 3728–3736. https://doi.org/10.1039/C5FO00600G
- Chew, N. Y., & Chan, H. K. (2002). Effect of powder polydispersity on aerosol generation. Journal of Pharmacy & Pharmaceutical Sciences: A Publication of the Canadian Society for Pharmaceutical Sciences, Societe Canadienne des Sciences Pharmaceutiques, 5(2), 162–168.
- Chun-Yu, Y., Di, F., Ping, W., & Yan, M. (2004). Preparation technology of ultra-fine powders of auricularia auricular. Journal of Forestry Research, 15(2), 150–152. https://doi.org/10.1007/BF02856752
- Cui, L., Li, J., Wang, Z., Wei, X., Xia, W., & Zhou, W. Effects of superfine grinding on the structure, bioactive components and antioxidant activity of pineapple leaf fiber. (2019). IOP Conference Series: Materials Science & Engineering, 611(1), 12052. https://doi.org/10.1088/1757-899X/611/1/012052
- de la Hera, E., Martinez, M., & Gómez, M. (2013). Influence of flour particle size on quality of gluten-free rice bread. LWT-Food Science and Technology, 54(1), 199–206. https://doi.org/10.1016/j.lwt.2013.04.019
- Eke-Ejiofor, J. N., Ojimadu, A. E., Wordu, G. O., & Ofoedu, C. E. (2021). Functional properties of complementary food from millet (Pennisetum glaucum), African yam bean (Sphenostylis stenocarpa), and jackfruit (Artocarpus heterophyllus) flour blends: A comparative study. Asian Food Science Journal, 20(9), 45–62. https://doi.org/10.9734/AFSJ/2021/v20i930342
- Gao, W., Chen, F., Wang, X., & Meng, Q. (2020a). Recent advances in processing food powders by using superfine grinding techniques: A review. Comprehensive Reviews in Food Science and Food Safety, 19(4), 2222–2255. https://doi.org/10.1111/1541-4337.12580
- Gao, W., Chen, F., Zhang, L., & Meng, Q. (2020b). Effects of superfine grinding on asparagus pomace. Part I: Changes on physicochemical and functional properties. Journal of Food Science, 85(6), 1827–1833. https://doi.org/10.1111/1750-3841.15168
- Gao, Y., Zhang, M., Chen, G., & Wang, Y. (2013). Effect of micronization on physicochemical properties of small yellow croaker (Pseudosciaena polyactis) skull. Advanced Powder Technology, 24(6), 932–938. https://doi.org/10.1016/j.apt.2013.01.009
- Gao, X., Zhu, D., Liu, Y., Zha, L., Chen, D., & Guo, H. (2019). Physicochemical properties and anthocyanin bioaccessibility of downy rose-myrtle powder prepared by superfine grinding. International Journal of Food Properties, 22(1), 2022–2032. https://doi.org/10.1080/10942912.2019.1702999
- Ghodki, B. M., & Goswami, T. K. (2016). Effect of grinding temperatures on particle and physicochemical characteristics of black pepper powder. Powder Technology, 299, 168–177. https://doi.org/10.1016/j.powtec.2016.05.042
- Gong, P., Huang, Z., Guo, Y., Wang, X., Yue, S., Yang, W., Chen, F., Chang, X., & Chen, L. (2022). The effect of superfine grinding on physicochemical properties of three kinds of mushroom powder. Journal of Food Science, 87(8), 3528–3541. https://doi.org/10.1111/1750-3841.16239
- Guo, X., Dai, T., Chen, M., Deng, L., Chen, J., & Liu, C. (2022). Steam bread made by superfine purple corn flour: Texture characteristics and in vitro starch digestibility. LWT, 169, 113967. https://doi.org/10.1016/j.lwt.2022.113967
- He, S., Li, J., He, Q., Jian, H., Zhang, Y., Wang, J., & Sun, H. (2018). Physicochemical and antioxidant properties of hard white winter wheat (Triticum aestivm L.) bran superfine powder produced by eccentric vibratory milling. Powder Technology, 325, 126–133. https://doi.org/10.1016/j.powtec.2017.10.054
- Hemery, Y., Chaurand, M., Holopainen, U., Lampi, A. M., Lehtinen, P., Piironen, V., Sadoudi, A., & Rouau, X. (2011). Potential of dry fractionation of wheat bran for the development of food ingredients, part I: Influence of ultra-fine grinding. Journal of Cereal Science, 53(1), 1–8. https://doi.org/10.1016/j.jcs.2010.09.005
- Hou, D., Duan, W., Xue, Y., Yousaf, L., Hu, J., & Shen, Q. (2020). Effects of superfine grinding and extrusion on dough mixing properties and noodle quality of black soybean flour. Journal of Food Measurement and Characterization, 14(1), 125–134. https://doi.org/10.1007/s11694-019-00274-6
- Hou, S., Zhao, Q. S., Chang, S., Li, H., Zha, S. H., Li, Q., Weng, D., Qin, Q., Zhao, B., & Zhang, J. (2023). Effect of superfine grinding on the physico‐chemical and antioxidant properties of cistanche deserticola powders. Journal of Food Process Engineering, 46(5), e14319. https://doi.org/10.1111/jfpe.14319
- Huang, X., Dou, J. Y., Li, D., & Wang, L. J. (2018). Effects of superfine grinding on properties of sugar beet pulp powders. LWT, 87, 203–209. https://doi.org/10.1016/j.lwt.2017.08.067
- Huang, X., Liang, K. H., Liu, Q., Qiu, J., Wang, J., & Zhu, H. (2020). Superfine grinding affects physicochemical, thermal and structural properties of moringa oleifera leaf powders. Industrial Crops and Products, 151, 112472. https://doi.org/10.1016/j.indcrop.2020.112472
- Huang, Y. L., Sheu, F., Lee, M. H., & Chau, C. F. (2008). Effects of particle size reduction of insoluble fibres by micron technology on various caecal and faecal indices. Journal of the Science of Food and Agriculture, 88(3), 435–441. https://doi.org/10.1002/jsfa.3104
- Hu, J., Chen, Y., & Ni, D. (2012). Effect of superfine grinding on quality and antioxidant property of fine green tea powders. LWT-Food Science and Technology, 45(1), 8–12. https://doi.org/10.1016/j.lwt.2011.08.002
- Hu, J., Ma, L., Liu, X., Li, H., Zhang, M., Jiang, Z., & Hou, J. (2022). Superfine grinding pretreatment enhances emulsifying, gel properties and in vitro digestibility of laccase-treated α-lactalbumin. Lwt, 157, 113082. https://doi.org/10.1016/j.lwt.2022.113082
- Ibeabuchi, J. C., Okafor, D. C., Peter–Ikechukwu, A., Agunwa, I. M., Eluchie, C. N., Ofoedu, C. E., & Nwatu, N. P. (2017). Comparative study on the proximate composition, functional and sensory properties of three varieties of beans Phaseolus lunatus. Phaseolus Vulgaris and Vigna Umbellata, International Journal of Advancement in Engineering Technology, Management and Applied Science (IJAETMAS), 5(1), 1–23. https://doi.org/10.9734/cjast/2019/v33i430090
- Ihediohanma, N. C., Ofoedu, C. E., Ojimba, N. C., Okafor, D. C., & Adedokun, A. O. (2014). Comparative Effect of milling methods on the proximate composition and functional properties of cowpea (Vigna unguiculata). International Journal of Life Sciences, 3(4), 170–177.
- Jakobek, L., & Matić, P. (2019). Non-covalent dietary fiber-polyphenol interactions and their influence on polyphenol bioaccessibility. Trends in Food Science & Technology, 83, 235–247. https://doi.org/10.1016/j.tifs.2018.11.024
- Jin, S., & Chen, H. (2006). Superfine grinding of steam-exploded rice straw and its enzymatic hydrolysis. Biochemical engineering journal, 30(3), 225–230. https://doi.org/10.1016/j.bej.2006.05.002
- Jin, X., Lin, S., Gao, J., Wang, Y., Ying, J., Dong, Z., & Zhou, W. (2020). How manipulation of wheat bran by superfine-grinding affects a wide spectrum of dough rheological properties. Journal of Cereal Science, 96, 103081. https://doi.org/10.1016/j.jcs.2020.103081
- Lee, Y. T., Shim, M. J., Goh, H. K., Mok, C., & Puligundla, P. (2019). Effect of jet milling on the physicochemical properties, pasting properties, and in vitro starch digestibility of germinated brown rice flour. Food Chemistry, 282, 164–168. https://doi.org/10.1016/j.foodchem.2018.07.179
- Li, G., Guo, W., Gao, X., Wang, Y., & Sun, S. (2020). Effect of superfine grinding on physicochemical and antioxidant properties of soybean residue powder. Food Science & Nutrition, 8(2), 1208–1214. https://doi.org/10.1002/fsn3.1409
- Li, Y., Li, M., Wang, L., & Li, Z. (2022). Effect of particle size on the release behavior and functional properties of wheat bran phenolic compounds during in vitro gastrointestinal digestion. Food Chemistry, 367, 130751. https://doi.org/10.1016/j.foodchem.2021.130751
- Lin, S., Jin, X., Gao, J., Qiu, Z., Ying, J., Wang, Y., Dong, Z., & Zhou, W. (2021). Impact of wheat bran micronization on dough properties and bread quality: Part I–bran functionality and dough properties. Food Chemistry, 353, 129407. https://doi.org/10.1016/j.foodchem.2021.129407
- Liu, T. Y., Ma, Y., Yu, S. F., Shi, J., & Xue, S. (2011). The effect of ball milling treatment on structure and porosity of maize starch granule. Innovative Food Science & Emerging Technologies, 12(4), 586–593. https://doi.org/10.1016/j.ifset.2011.06.009
- Liu, R., Zhu, T., Li, J., Wu, T., Li, Q., Meng, Y., Cao, Q., & Zhang, M. (2016). Physicochemical and antioxidative properties of superfine-ground oat bran polysaccharides. Food Science and Technology Research, 22(1), 101–109. https://doi.org/10.3136/fstr.22.101
- Luhovyy, B. L., Hamilton, A., Kathirvel, P., & Mustafaalsaafin, H. (2017). The effect of navy bean flour particle size on carbohydrate digestion rate measured in vitro. Cereal Foods World, 62(5), 208–213. https://doi.org/10.1094/CFW-62-5-0208
- McClements, D. J., & Rao, J. (2011). Food-grade nanoemulsions: Formulation, fabrication, properties, performance, biological fate, and potential toxicity. Critical Reviews in Food Science and Nutrition, 51(4), 285–330. https://doi.org/10.1080/10408398.2011.559558
- Memon, A. A., Mahar, I., Memon, R., Soomro, S., Harnly, J., Memon, N., Bhangar, M. I., & Luthria, D. L. (2020). Impact of flour particle size on nutrient and phenolic acid composition of commercial wheat varieties. Journal of Food Composition and Analysis, 86, 103358. https://doi.org/10.1016/j.jfca.2019.103358
- Mena, E. H., Liu, T., Liao, X., Olajide, T. M., & Huang, J. (2016). Effect of superfine grinding on the phytochemicals and antioxidant activities of mulberry leaves. Science, 4(3), 138–146. https://doi.org/10.11648/j.sjph.20160403.11
- Meng, Q., Chen, F., Xiao, T., & Zhang, L. (2019). Superfine grinding of dendrobium officinale: The finer the better? International Journal of Food Science & Technology, 54(6), 2199–2208. https://doi.org/10.1111/ijfs.14129
- Meng, Q., Fan, H., Xu, D., Aboshora, W., Tang, Y., Xiao, T., & Zhang, L. (2017). Superfine grinding improves the bioaccessibility and antioxidant properties of dendrobium officinale powders. International Journal of Food Science & Technology, 52(6), 1440–1451. https://doi.org/10.1111/ijfs.13405
- Mert, I. D. (2020). The applications of microfluidization in cereals and cereal-based products: An overview. Critical Reviews in Food Science and Nutrition, 60(6), 1007–1024. https://doi.org/10.1080/10408398.2018.1555134
- Min, C., Baoguo, L., Xianzhou, C., & Mingxu, W. (2021). Effect of grinding medium characteristics of vibration mill on superfine grinding of wheat bran. Transaction of the Chinese Society of Agricultural Engineering, 37(23), 256–263. https://doi.org/10.11975/j.issn.1002-6819.2021.23.030
- Ming, J., Chen, L., Hong, H., & Li, J. (2015). Effect of superfine grinding on the physico‐chemical, morphological and thermogravimetric properties of lentinus edodes mushroom powders. Journal of the Science of Food and Agriculture, 95(12), 2431–2437. https://doi.org/10.1002/jsfa.6967
- Muttakin, S., Kim, M. S., & Lee, D. (2015). Tailoring physicochemical and sensorial properties of defatted soybean flour using jet-milling technology. Food Chemistry, 187, 106–111. https://doi.org/10.1016/j.foodchem.2015.04.104
- Niu, M., Hou, G. G., Wang, L., & Chen, Z. (2014). Effects of superfine grinding on the quality characteristics of whole-wheat flour and its raw noodle product. Journal of Cereal Science, 60(2), 382–388. https://doi.org/10.1016/j.jcs.2014.05.007
- Ofoedu, C. E., Osuji, C. M., Omeire, G. C., Ojukwu, M., Okpala, C. O. R., & Korzeniowska, M. (2020). Functional properties of syrup from malted and unmalted rice of different varieties: A comparative study. Journal of Food Science, 85(10), 3081–3093. https://doi.org/10.1111/1750-3841.15446
- Ofoedu, C. E., You, L., Osuji, C. M., Iwouno, J. O., Kabuo, N. O., Ojukwu, M., Agunwah, I. M., Chacha, J. S., Muobike, O. P., Agunbiade, A. O., Sardo, G., Bono, G., Okpala, C. O. R., & Korzeniowksa, M. (2021). Hydrogen peroxide effects on natural-sourced polysaccharides: Free radical formation/production, degradation process, and reaction mechanism-A critical synopsis. Foods, 10(4), 699. https://doi.org/10.3390/foods10040699
- Prasedya, E. S., Frediansyah, A., Martyasari, N. W. R., Ilhami, B. K., Abidin, A. S., Padmi, H. F., Juanssilfero, A. B., Widyastuti, S., & Sunarwidhi, A. L. (2021). Effect of particle size on phytochemical composition and antioxidant properties of sargassum cristaefolium ethanol extract. Scientific Reports, 11(1), 1–9. https://doi.org/10.1038/s41598-021-95769-y
- Qiu, L., Zhang, M., Xu, B., & Wang, B. (2022). Effects of superfine grinding on the physicochemical properties, antioxidant capacity, and hygroscopicity of Rosa rugosa cv. Plena powders. Journal of the Science of Food and Agriculture, 102(10), 4192–4199. https://doi.org/10.1002/jsfa.11768
- Rajkhowa, R., Wang, L., & Wang, X. (2008). Ultra-fine silk powder preparation through rotary and ball milling. Powder Technology, 185(1), 87–95. https://doi.org/10.1016/j.powtec.2008.01.005
- Ramachandraiah, K., & Chin, K. B. (2016). Evaluation of ball-milling time on the physicochemical and antioxidant properties of persimmon by-products powder. Innovative Food Science & Emerging Technologies, 37, 115–124. https://doi.org/10.1016/j.ifset.2016.08.005
- Ribas-Agustí, A., Martín-Belloso, O., Soliva-Fortuny, R., & Elez-Martínez, P. (2018). Food processing strategies to enhance phenolic compounds bioaccessibility and bioavailability in plant-based foods. Critical Reviews in Food Science and Nutrition, 58(15), 2531–2548. https://doi.org/10.1080/10408398.2017.1331200
- Rizlan, Z., & Mamat, O. (2014). Process parameters optimization of silica sand nanoparticles production using low speed ball milling method. Chinese Journal of Engineering, 2014, 1–4. https://doi.org/10.1155/2014/802459
- Rosa, N. N., Barron, C., Gaiani, C., Dufour, C., & Micard, V. (2013). Ultra-fine grinding increases the antioxidant capacity of wheat bran. Journal of Cereal Science, 57(1), 84–90. https://doi.org/10.1016/j.jcs.2012.10.002
- Sheng, K., Qu, H., Liu, C., Yan, L., You, J., Shui, S., & Zheng, L. (2017). A comparative assess of high hydrostatic pressure and superfine grinding on physicochemical and antioxidant properties of grape pomace. International Journal of Food Science & Technology, 52(9), 2106–2114. https://doi.org/10.1111/ijfs.13489
- Shu, Y., Li, J., Yang, X., Dong, X., & Wang, X. (2019). Effect of particle size on the bioaccessibility of polyphenols and polysaccharides in green tea powder and its antioxidant activity after simulated human digestion. Journal of Food Science and Technology, 56(3), 1127–1133. https://doi.org/10.1007/s13197-019-03573-4
- Song, L. L., Fan, B. Y., Jiang, S. Z., & Zhang, D. L. (2002). Probe into characteristics of taraxacum mongolicum ultramicro-power. Zhongguo Zhong Yao Za Zhi= Zhongguo Zhongyao Zazhi= China Journal of Chinese Materia Medica, 27(1), 12–15.
- Song, L., Song, L., Su, H., Ma, F., & Zhang, B. (2022). Superfine grinding affects particle size, chemical ingredients, and physicochemical properties of sprouting quinoa. Cereal Chemistry, 99(3), 520–529. https://doi.org/10.1002/cche.10515
- Sun, C., Liu, R., Ni, K., Wu, T., Luo, X., Liang, B., & Zhang, M. (2016). Reduction of particle size based on superfine grinding: Effects on structure, rheological and gelling properties of whey protein concentrate. Journal of Food Engineering, 186, 69–76. https://doi.org/10.1016/j.jfoodeng.2016.03.002
- Sun, C., Wu, T., Liu, R., Liang, B., Tian, Z., Zhang, E., & Zhang, M. (2015). Effects of superfine grinding and microparticulation on the surface hydrophobicity of whey protein concentrate and its relation to emulsions stability. Food Hydrocolloids, 51, 512–518. https://doi.org/10.1016/j.foodhyd.2015.05.027
- Sun, X., Zhang, Y., Li, J., Aslam, N., Sun, H., Zhao, J., Wu, Z., & He, S. (2019). Effects of particle size on physicochemical and functional properties of superfine black kidney bean (Phaseolus vulgaris L.) powder. PeerJ, 7, e6369. https://doi.org/10.7717/peerj.6369
- Waliullah, M. H., Mu, T., Ma, M., & Chen, J. (2021). Effects of particle size on structural, physicochemical, and functional properties of potato residue from starch isolation and quality characteristics of residue-based starch noodles. Food Science and Technology International, 27(5), 392–403. https://doi.org/10.1177/1082013220954606
- Wang, X., Kristo, E., & LaPointe, G. (2019). The effect of apple pomace on the texture, rheology and microstructure of set type yogurt. Food Hydrocolloids, 91, 83–91. https://doi.org/10.1016/j.foodhyd.2019.01.004
- Wang, H., Tao, M., Zhang, H., Cheng, S., Zhang, L., & Liu, Z. (2020). The mechanism on decreasing the microbiological contamination of superfine green tea powder by ball milling. LWT, 134, 109966. https://doi.org/10.1016/j.lwt.2020.109966
- Wu, Z., Ameer, K., & Jiang, G. (2021). Effects of superfine grinding on the physicochemical properties and antioxidant activities of sanchi (Panax notoginseng) flower powders. Journal of Food Science and Technology, 58(1), 62–73. https://doi.org/10.1007/s13197-020-04514-2
- Wu, G. C., Zhang, M., Wang, Y. Q., Mothibe, K. J., & Chen, W. X. (2012). Production of silver carp bone powder using superfine grinding technology: Suitable production parameters and its properties. Journal of Food Engineering, 109(4), 730–735. https://doi.org/10.1016/j.jfoodeng.2011.11.013
- Xiao, W., Zhang, Y., Fan, C., & Han, L. (2017). A method for producing superfine black tea powder with enhanced infusion and dispersion property. Food Chemistry, 214, 242–247. https://doi.org/10.1016/j.foodchem.2016.07.096
- Xu, Q., Huang, R., Yang, P., Wang, L., Xing, Y., Liu, H., Wu, L., Che, Z., & Zhang, P. (2021). Effect of different superfine grinding technologies on the physicochemical and antioxidant properties of tartary buckwheat bran powder. RSC Advances, 11(49), 30898–30910. https://doi.org/10.1039/D1RA05093A
- Xu, X., Xu, Y., Wang, N., & Zhou, Y. (2018). Effects of superfine grinding of bran on the properties of dough and qualities of steamed bread. Journal of Cereal Science, 81, 76–82. https://doi.org/10.1016/j.jcs.2018.04.002
- Yang, D., Qu, M., Tianmei, Z., & Lihan, F. (2016). Research on production technology of low-temperature superfine grinding jujube. Advance Journal of Food Science & Technology, 12(6), 331–336. https://doi.org/10.19026/ajfst.12.2969
- Yang, L., Wang, S., Zhang, H., Du, C., Li, S., & Yang, J. (2022). Effects of black soybean powder particle size on the characteristics of mixed powder and wheat flour dough. LWT, 167, 113834. https://doi.org/10.1016/j.lwt.2022.113834
- Yan, T., Liu, R., Shi, L., Wang, Y., Meng, X., & Shen, Y. (2023). Superfine grinding improves the physicochemical, sensory and functional characteristics of hanfu apple pomace. International Journal of Food Science & Technology, 58(4), 2077–2084. https://doi.org/10.1111/ijfs.15934
- Yu, D., Chen, J., Ma, J., Sun, H., Yuan, Y., Ju, Q., Teng, Y., Yang, M., Li, W., Fujita, K., Tatsumi, E., & Luan, G. (2018). Effects of different milling methods on physicochemical properties of common buckwheat flour. LWT, 92, 220–226. https://doi.org/10.1016/j.lwt.2018.02.033
- Yu, S., Wu, Y., Li, Z., Wang, C., Zhang, D., & Wang, L. (2023). Effect of different milling methods on physicochemical and functional properties of mung bean flour. Frontiers in Nutrition, 10, 1117385. https://doi.org/10.3389/fnut.2023.1117385
- Zhang, Z. Q., Chen, S. C., Wang, Q. L., Liu, C. Q., Xiao, J. H., & Huang, D. W. (2023). Effects of traditional grinding and superfine grinding technologies on the properties and volatile components of protaetia brevitarsis larvae powder. LWT, 173, 114307. https://doi.org/10.1016/j.lwt.2022.114307
- Zhang, J., Dong, Y., Nisar, T., Fang, Z., Wang, Z. C., & Guo, Y. (2020). Effect of superfine-grinding on the physicochemical and antioxidant properties of Lycium ruthenicum Murray powders. Powder Technology, 372, 68–75. https://doi.org/10.1016/j.powtec.2020.05.097
- Zhang, X., & Haque, Z. Z. (2015). Generation and stabilization of whey-based monodisperse nanoemulsions using ultra-high-pressure homogenization and small amphipathic co-emulsifier combinations. Journal of Agricultural & Food Chemistry, 63(45), 10070–10077. https://doi.org/10.1021/acs.jafc.5b03889
- Zhang, L. H., & Li, S. F. (2009). Effects of micronization on properties of Chaenomeles sinensis (Thouin) Koehne fruit powder. Innovative Food Science & Emerging Technologies, 10(4), 633–637. https://doi.org/10.1016/j.ifset.2009.05.010
- Zhang, M., Wang, F., Liu, R., Tang, X., Zhang, Q., & Zhang, Z. (2014). Effects of superfine grinding on physicochemical and antioxidant properties of Lycium barbarum polysaccharides. LWT-Food Science and Technology, 58(2), 594–601. https://doi.org/10.1016/j.lwt.2014.04.020
- Zhang, T., Xiao, S.-Y., Ding, Z.-H., & Song, Y.-T. (2021). Effects of superfine grinding on physicochemical properties and morphological structure of coix seed powders. Journal of Cereal Science, 102, 103361. https://doi.org/10.1016/j.jcs.2021.103361
- Zhang, J., Yu, Y., & Xu, G. (2010). Technology of superfine grinding and its application in food industry. Journal of Agricultural Science, 31(1), 51–54.
- Zhang, M., Zhang, C. J., & Shrestha, S. (2005). Study on the preparation technology of superfine ground powder of agrocybe chaxingu huang. Journal of Food Engineering, 67(3), 333–337. https://doi.org/10.1016/j.jfoodeng.2004.04.036
- Zhao, S., Baik, O. D., Choi, Y. J., & Kim, S. M. (2014). Pretreatments for the efficient extraction of bioactive compounds from plant-based biomaterials. Critical reviews in food science and nutrition, 54(10), 1283–1297. https://doi.org/10.1080/10408398.2011.632698
- Zhao, Z., Dai, Z., Jiang, X., Yu, L., Hu, M., Peng, J., & Zhou, F. (2023). Influence and optimization of long-time superfine grinding on the physicochemical features of green tea powder. Journal of Food Composition and Analysis, 117, 105124. https://doi.org/10.1016/j.jfca.2022.105124
- Zhao, X., Du, F., Zhu, Q., Qiu, D., Yin, W., & Ao, Q. (2010). Effect of superfine pulverization on properties of astragalus membranaceus powder. Powder Technology, 203(3), 620–625. https://doi.org/10.1016/j.powtec.2010.06.029
- Zhao, X., Liu, H., Zhang, X., & Ao, Q. (2018b). Effect of pressure grinding technology on the physicochemical and antioxidant properties of tremella aurantialba powder. Journal of Food Processing and Preservation, 42(12), e13833. https://doi.org/10.1111/jfpp.13833
- Zhao, X., Yang, Z., Gai, G., & Yang, Y. (2009). Effect of superfine grinding on properties of ginger powder. Journal of Food Engineering, 91(2), 217–222. https://doi.org/10.1016/j.jfoodeng.2008.08.024
- Zhao, G., Zhang, R., Dong, L., Huang, F., Tang, X., Wei, Z., & Zhang, M. (2018a). Particle size of insoluble dietary fiber from rice bran affects its phenolic profile, bioaccessibility and functional properties. LWT, 87, 450–456. https://doi.org/10.1016/j.lwt.2017.09.016
- Zhao, X., Zhu, H., Zhang, G., & Tang, W. (2015). Effect of superfine grinding on the physicochemical properties and antioxidant activity of red grape pomace powders. Powder Technology, 286, 838–844. https://doi.org/10.1016/j.powtec.2015.09.025
- Zhong, C., Zu, Y., Zhao, X., Li, Y., Ge, Y., Wu, W., Zhang, Y., Li, Y., & Guo, D. (2016). Effect of superfine grinding on physicochemical and antioxidant properties of pomegranate peel. International Journal of Food Science & Technology, 51(1), 212–221. https://doi.org/10.1111/ijfs.12982
- Zhu, Y., Bai, J., Qian, X., Yang, X., Zhou, X., Zhao, Y., Dong, Y., & Xiao, X. (2022). Effect of superfine grinding on physical properties, bioaccessibility, and anti‐obesity activities of bitter melon powders. Journal of the Science of Food and Agriculture, 102(11), 4473–4483. https://doi.org/10.1002/jsfa.11802
- Zhu, Y., Dong, Y., Qian, X., Cui, F., Guo, Q., Zhou, X., Wang, Y., Zhang, Y., & Xiong, Z. (2011). Effect of superfine grinding on antidiabetic activity of bitter melon powder. International Journal of Molecular Sciences, 13(12), 14203–14218. https://doi.org/10.3390/ijms131114203
- Zhu, F. M., Du, B., & Li, J. (2014). Effect of ultrafine grinding on physicochemical and antioxidant properties of dietary fiber from wine grape pomace. Food Science and Technology International, 20(1), 55–62. https://doi.org/10.1177/1082013212469619
- Zhu, F., Du, B., & Xu, B. (2015). Superfine grinding improves functional properties and antioxidant capacities of bran dietary fibre from qingke (hull-less barley) grown in Qinghai-Tibet Plateau, China. Journal of Cereal Science, 65, 43–47. https://doi.org/10.1016/j.jcs.2015.06.006
- Zhu, K., Huang, S., Peng, W., Qian, H., & Zhou, H. (2010). Effect of ultrafine grinding on hydration and antioxidant properties of wheat bran dietary fiber. Food Research International, 43(4), 943–948. https://doi.org/10.1016/j.foodres.2010.01.005