ABSTRACT
Osteoporosis (OP) is one of the epidemic diseases worldwide. Recently, a high-fat diet (HFD) makes the effects on skeletal microarchitecture still unclear. To explain the problem, we searched studies from PubMed, Embase, Web of Science, and Cochrane library to investigate the effect of HFD-induced obesity on bone structure and metabolism for mice from June 2010 to June 2020. Finally, eight publications were included. The results showed BV/TV was significantly decreased in HFD than control diet (CD) (SMD −4.24, 95% CI: −6.60, −1.87, p < .001), Tb.N (SMD −2.98, 95% CI: −4.89, −1.06, p = .002) and Tb.Th (SMD −1.26, 95% CI: −1.93, −0.59, p < .001) were significantly decreased. Tb.Sp (SMD 2.38, 95% CI:1.04, 3.72, p < .001) and SMI (SMD 3.86, 95% CI: 1.79, 5.93, p < .001) were significantly increased in HFD. In conclusion, HFD accelerated OP progression which reflected on LDL, leptin, osteocalcin (OCN) and osteoprotegerin (OPG).
KEYWORDS:
1. Introduction
Osteoporosis (OP) is a very common disease in our lives, and the pathogenesis of OP remains unclear. OP is defined by the World Health Organization (WHO) as a “Progressive systemic skeletal disease characterized by decreased bone density and bone mass, microarchitectural deterioration of bone tissue, increased bone fragility and prone to fracture” (Kanis et al., Citation1994). The dietary structure is essential for human health, high-fat intake or excessive proportion of energy from fat in the diet has been claimed as responsible for obesity (OB), which was considered to be harmful to health in an excessive accumulation of fat way (Bosello et al., Citation2016). Studies related to human have shown that a high-fat diet (≥30% of energy from fat) can easily induce OB (French & Robinson, Citation2003; Schrauwen & Westerterp, Citation2000). HFD and OP have usually been considered separately, but recently, an increasing number of clinical and experimental data have established a close relationship between them (Qiao et al., Citation2021).
OP and dietary habits have potential interactions and common characteristics like multifactorial etiologies, including lifestyle and environmental components. But the relationship between HFD and OP was controversial in past studies. An increase in body weight has been considered a factor that increases bone mass and decreases the risk of fracture (De Laet et al., Citation2005; Sontam et al., Citation2017). Meanwhile, some of the studies in recent years indicated that HFD with an accumulation of fat mass may have a negative impact on the risk of bone fragility and fracture (Kanis et al., Citation2004). Recently, increasing studies revealed that HFD as an indispensable risk factor for OP, and its effect on bone metabolism and skeletal structure may underestimate, this conclusion was supported by animal experiments, and HFD caused lower bone perimeters (Montalvany-Antonucci et al., Citation2018; Yoneda et al., Citation2017). The relationship between OP and HFD was influenced by many complex factors, which establish on the influence of BMI on fracture risk is non-linear and associated with their weights of themselves.
Since underestimate of caloric intake is an important bias in epidemiological studies on dietary measurement, and related bone metabolism diseases in human group (Hebert et al., Citation2003; Poppitt et al., Citation1998). Furthermore, dietary habit also effects the bone health. In human studies, a western diet has a negative impact on BMD and accelerate bone loss with a higher risk of fractures (Kwon et al., Citation2015; Qiao et al., Citation2021). Animal models by HFD-induced have been widely used for experiments on these disorders (Holmes et al., Citation2015; Young & Kirkland, Citation2007). Some studies have shown that HFD feeding leads to a greater loss of bone and prevents the preservation of the trabecular micro-architecture in mice (Gautam et al., Citation2014).
Accumulating evidence indicated that HFD plays a key role in the progression of OP, but the experimental results are still controversial. In the present study, we aim to explore the relationship between OP and HFD, predominantly focus on systematically evaluate the effects of HFD caused the progression of OP in mice.
2. Methods
This meta-analysis was conducted following the Preferred Reporting Items for Systematic Reviews and Meta-analyses (PRISMA) guidelines (Liberati et al., Citation2009).
2.1. Search strategy
To identify all the primary studies, a systematic literature search was conducted using the PUBMED, EMBASE, Web of Science, and Cochrane library from June 2010 to June 2020. Keywords included “high-fat diet”, “obesity”, “osteoporosis”, and “mice”, they are combined during the search process. The reference list of potentially eligible studies was also examined. No language restriction was used.
2.2. Inclusion criteria
All the included studies should match the following criteria: 1) randomized controlled trials or controlled observational studies; 2) enrolled mice to study OP and obesity; 3) compared HFD, defined as at least 30% of caloric intake from fat, to a control diet (CD) consisting of a low-fat diet or standard chow; 4) measured bone metabolism or alteration; 5) full-text articles. We excluded studies with cointerventions (e.g. high-fat – high-sucrose diet) and studies without a control group.
2.3. Outcomes
Bone metabolism parameters measured by Micro-CT were the primary outcomes. In detail, Bone Volume/Total Volume (BV/TV); Trabecular Number (Tb.N); Trabecular Thickness (Tb.Th); Trabecular Spacing (Tb.Sp); Structure Model Index (SMI) were used as a cancellous bone parameter, Cortical bone Area (Ct.Ar); Cortical bone Thickness (Ct.Th) were used as a cortical bone parameter.
To assess the link between HFD and the progression of OP, as secondary outcomes, data reported on cytokines and biomarkers of metabolism related to OP were extracted and evaluated with a summary.
2.4. Data extraction
Two authors independently extracted the data from studies that were included in the meta-analysis. The following information was recorded: 1) characteristics of the studies (author’s name, publication year); 2) characteristics of animals (sex, age, strain); 3) data about Micro-CT based bone metabolism; and 4) indications of systemic changes in bone metabolism or lipid metabolism.
2.5. Risk of bias assessment
The Systematic Review Centre for Laboratory Animal Experimentation (SYRClE) risk of bias tool for animal studies (Hooijmans et al., Citation2014) was used to evaluate the studies’ quality by two independent researchers. This tool contains nine domains (sequence generation, baseline characteristics, allocation concealment, housing, blinding researchers, random outcome assessment, blinding outcome assessors, incomplete outcome data, and selective outcome reporting) relating to five types of bias: selection, performance, detection, attrition, and reporting bias. Every domain was classified as having either a “low”, “high”, or “unclear” risk of bias based on the information reported in each study.
2.6. Statistical analysis
We used standardized mean difference (SMD) with a 95% confidence interval (CI) according to all the bone parameters measured by Micro-CT to quantify the pooled effects of the primary outcome. The heterogeneity of HFD effects among the studies was tested using I2 statistics. Values of P < .10 and I2 >50% were considered to indicate significant heterogeneity. Due to the huge difference in the selection of measurement sites among the studies included in this systematic review, data were meta-analyzed using the random effect models. All comparisons were performed using Review Manager (RevMan5) software version 5.3 (RevMan; Cochrane, London, UK).
3. Results
A total of 781 articles were identified according to the search strategy (), of which 336 were duplicates. After the title and abstract screening of 445 articles, 80 publications were selected for full-text evaluation. Finally, 72 articles were excluded based on Methods and Outcomes, of which 8 papers met the inclusion criteria. All studies included the primary outcome indicators of the effect of HFD on bone metabolism (Cao & Gregoire, Citation2016; Gautam et al., Citation2014, Citation2016, Citation2017; Jatkar et al., Citation2017; Ma et al., Citation2011; McCabe et al., Citation2019; Silva et al., Citation2019) and secondary outcome indicators. However, the data on secondary outcomes was inappropriate for Meta-analysis, so we only summarized these data. Details of the 8 included papers were outlined in .
Figure 1. Preferred reporting items for systematic reviews and meta-analyses (PRISMA) flow diagram of the study selection process for this systematic review.
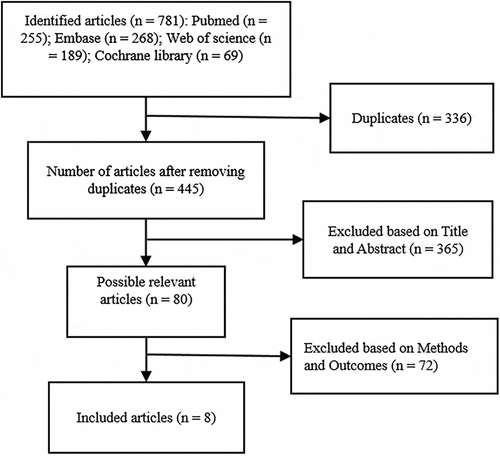
Table 1. Summary of included studies and characteristics of experimental animals.
3.1. Characteristics of animals and diets
All included studies were conducted in mice. 5 papers used male C57BL/6 mice (Gautam et al., Citation2014, Citation2016, Citation2017; Ma et al., Citation2011; McCabe et al., Citation2019), the other 3 papers used female C57BL/6 mice (Cao & Gregoire, Citation2016), male BALB/c mice (Jatkar et al., Citation2017), and LG/J female & SM/J male inbred mice (Silva et al., Citation2019). At baseline, the age of the mice varied from 3 to 17 weeks with a mean age of 6.5 weeks. The maximum duration of the diets varied between 10 and 22 weeks, and the mean percentage of calories derived from fat was 49% (40% to 60%) for an HFD and 13% (4% to 16%) for a CD ().
3.2. Risk of bias assessment
shows the risk of bias reported for each paper included in this meta-analysis. Overall, the majority of studies were judged at low risk of bias. 7 of the 8 studies reported measures used to house the animals within the animal room. 4 studies reported the baseline characteristics of animals included. However, only 1 study reported the sequence generation and none described the methods used to generate and conceal the allocation sequence. 5 studies were at high risk of blinding researchers. 1 study was at a low risk of Blinding outcome assessment and 2 studies were at a low risk of Random outcome assessment. 12 studies describe the details of each main outcome, so they were at low risk of attrition bias. All studies were at low risk of reporting bias.
3.3. Primary outcomes
Skeletal parameters measured by Micro-CT were used as the primary outcomes to reflect the effects of HFD on the bone of experimental animals. The measurement sites included the radius, tibia, femur, and vertebra. Micro-CT data was divided into cancellous bone parameters and cortical bone parameters.
3.3.1. Cancellous bone parameters
3.3.1.1 The effects of HFD on BV/TV
5 of 8 studies used BV/TV to reflect the impact of HFD on bones, the measurement sites included the tibia and femur, (). Our meta-analysis showed that HFD-fed mice have significantly reduced BV/TV values when compared with CD-fed mice (SMD −4.24, 95% CI −6.60 to −1.87; p = .0004, Z-test). Substantial heterogeneity was found in all studies (I2 = 92%).
3.3.1.2 The effects of HFD on Tb.N
7 of the 8 studies included Tb.N data and the measurement sites included the tibia, femur, and vertebra (). The pooled effects of the data showed that mice fed HFD are more likely to develop OP when compared with CD (SMD −2.98, 95% CI −4.89 to −1.06; p = .002, Z-test). But the substantial heterogeneity was found in these studies (I2 = 94%).
3.3.1.3 The effects of HFD on Tb.Th
5 of the 8 studies contained Tb.Th data, from the measurement sites, included the tibia, femur, and vertebra (). The overall results showed that the Tb.Th values significantly decreased when mice were fed HFD (SMD −1.26, 95% CI −1.93 to −0.59; p = .0002, Z-test). Slightly heterogeneity was found in these studies (I2 = 66%).
3.3.1.4 The effects of HFD on Tb.Sp
7 studies used Tb.Sp to reflect the impact of HFD on bones and the measurement sites included the tibia, femur, and vertebra (). The overall Tb.Sp values significantly increased in HFD mice (SMD 2.38, 95% CI 1.04 to 3.72; p = .0005, Z-test). But there was also substantial heterogeneity in studies (I2 = 90%).
3.3.1.5 The effects of HFD on SMI
6 studies contained SMI data, and the tibia and femur were measured by Micro-CT (). Meta-analysis results showed that HFD-fed mice have significantly increased SMI values when compared with CD-fed mice (SMD 3.86, 95% CI 1.79 to 5.93; p = .0003, Z-test). Substantial heterogeneity was found in all studies (I2 = 92%)
3.3.2. Cortical bone parameters
3.3.2.1 The effects of HFD on Ct.Ar
3 studies contained Ct.Ar data, the measurement sites included radius and femur (). The overall results showed that CD-mice have higher Ct.Ar values than HFD mice, but not statistically significant (SMD −0.71, 95% CI −1.50 to 0.08; p = .08, Z-test). Substantial heterogeneity was found in these studies (I2 = 96%).
3.3.2.2 The effects of HFD on Ct.Th
4 studies contained Ct.Th data, and the radius and femur were measured by Micro-CT (). The pooled effects of the data showed that HFD-mice have higher Ct.Th values than CD mice, but not statistically significant (SMD 0.13, 95% CI −0.13 to 0.38; p = .34, Z-test). There was slight heterogeneity in all studies (I2 = 59%).
3.4. Secondary outcomes
All included papers in this review reported data for cytokines in mice. These data can be found in . The results are divided into lipid metabolism-related factors and bone metabolism-related factors. These cytokines were quantified via various methods, including quantitative real-time PCR (RT-PCR), enzyme-linked immunosorbent assay (ELISA), and colorimetric assay (CA). All increases and decreases mentioned below are the result of HFD intervention and were statistically significant when compared with a CD (p < .05).
Table 2. A summary of OP related metabolism indicators and cytokines in all studies.
3.4.1. Lipid metabolism-related factors
Regarding the changes in blood lipids, CA results of serum samples showed that compared with a CD, TC (Gautam et al., Citation2014, Citation2016, Citation2017), TG (Gautam et al., Citation2014, Citation2016, Citation2017), VLDL (Gautam et al., Citation2016, Citation2017), LDL (Gautam et al., Citation2014, Citation2016, Citation2017) increased and HDL (Gautam et al., Citation2014, Citation2016, Citation2017) decreased in HFD-mice. ELISA analysis of serum samples showed that Estradiol increased in one study (Cao & Gregoire, Citation2016), TNF-α (Gautam et al., Citation2017), Insulin (Ma et al., Citation2011), Leptin (Jatkar et al., Citation2017; Ma et al., Citation2011; Silva et al., Citation2019), Resistin (Ma et al., Citation2011), and PAI-1 (Ma et al., Citation2011) was significantly increased in HFD-mice compared with the CD-mice. Gene expression of the bone and white adipose tissue (WAT) showed that an HFD, compared with a CD, induced an increase of the TNF-α (Gautam et al., Citation2016, Citation2017), Leptin (Gautam et al., Citation2017), PPAR-γ (Gautam et al., Citation2014, Citation2017), C/EBPα (Gautam et al., Citation2017), and aP2 (Gautam et al., Citation2014, Citation2016).
3.4.2. Bone metabolism-related factors
ELISA analysis of serum samples showed that OCN was increased in 2 studies (Gautam et al., Citation2014, Citation2017), no differences in 3 studies (Cao & Gregoire, Citation2016; Ma et al., Citation2011; McCabe et al., Citation2019), OPG (Ma et al., Citation2011), TRAP (Cao & Gregoire, Citation2016), CTx (Gautam et al., Citation2014) was significantly increased in HFD-mice compared with the CD-mice. However, gene expression of the bone showed that when comparing an HFD with a CD, OCN expression was significantly decreased in 2 studies (Gautam et al., Citation2014, Citation2016), TRAP (Gautam et al., Citation2014), RANKL (Gautam et al., Citation2014) was significantly increased and Col-1 (Gautam et al., Citation2014), OPG/RANKL (Gautam et al., Citation2014, Citation2016) was significantly decreased.
4. Discussion
Considering our systematic review to examine the evidence for the relationship between HFD and bone structures and metabolisms in mice. We performed Meta-analysis on the primary outcomes, which included cancellous bone parameters and cortical bone parameters of experimental mice. And secondary outcomes include lipid or bone metabolism-related factors. All the HFD animals included in the review had significantly higher body weights than CD animals at the end of the experiment (unpublished data). Based on that, we could think that the mice fed by HFD had reached obesity compared with CD mice, but more rigorously, the objective was to clarify the effects on bone structures and metabolisms in mice that were exposed to HFD.
It is well known that the trabecular bone is the primary target for anabolic or catabolic factors and the most active bone site. Therefore, the detection of this region was more detailed in most studies, and there were more parameters. Our analysis results included BV/TV, Tb.N, Tb.Th, Tb.Sp and SMI as the cancellous bone parameters. The pooled effects of the data showed that when compared with a CD, HFD-mice showed a significantly lower BV/TV (SMD −4.24; P = .0004, Z-test) when measured by microCT, which means stronger bone anabolic metabolism in CD-mice compared to HFD-mice. In addition, the overall effects showed that obesity can also significantly reduce Tb.N values in mice (SMD −2.98; P = .002, Z-test). Similar to the results of Tb.N, Tb.Th values in HFD mice were significantly reduced, compared with CD- mice(SMD −1.26; P = .0002, Z-test). Different from the two parameters described above, a larger Tb.Sp value means loose trabeculae and poor bone quality, the overall effects of Tb.Sp data showed that when compared with a CD, HFD-mice showed significantly higher Tb.Sp (SMD 2.38; P = .0005, Z-test). The last parameter, SMI is a composition ratio of plate-trabeculae and rod-trabeculae in the trabecular structure. If there were more plate-trabecular bones, the SMI is close to 0; conversely, if there were more rod-trabecular bones, the SMI is close to 3. When osteoporosis occurs, the trabecular bone changes from plate-like to rod-like, and this value increases. The data of our analysis showed that the SMI value of HFD-mice was close to 3 (SMD 3.86; p = .0003, Z-test), which means that these animals were more prone to osteoporosis. In summary, all of these cancellous bone parameters have statistical significance, which proved that HFD could damage the cancellous bone structure and significantly induce the onset of OP in mice.
There were fewer studies of OP on cortical bone than trabeculae. Our results showed that there was currently no evidence to prove that HFD can adversely affect cortical bone. In a study comparing the difference in mechanical loading between the cancellous bone and cortical bone, Kelly et al. found that cortical and cancellous bone exhibited distinct differences in response to mechanical loading, revealing key differences between cortical and cancellous genetic regulation in response to mechanical loading (Kelly et al., Citation2016). This may explain why HFD affects cancellous bone more than cortical bone.
There was a great disparity in high-fat content among the studies. This systematic review included an HFD, which we defined as a dietary HF content of 30%. The lowest percentage of HFD (used in 2 of the 8 papers) contained 40% kcal from fat, and the highest percentage of HFD (used in 3 of the 8 papers) contained 60% kcal from fat. Among the control groups, the high-fat content was similar, with an average of 13%, except for one article, the high-fat content was much lower than other articles (4%). Besides, it is also necessary to consider the difference in animal strain, the inconsistency of measurement sites, and the inherent biological variation of mice, which might all cause high heterogeneity. Our review included mice’s four experimental sites (tibia, femur, radius, and vertebra). In our Meta-analysis results, except for Tb.Th and Ct.Th, the BV/TV, Tb.N, Tb.Sp, SMI, and Ct.Ar data showed a very high heterogeneity, we hypothesized that these controversial results may be due to differences in animal age or strain and the skeleton site measured as well as different measuring techniques. Even if for the same skeleton site, the slight difference in the region of interest selection, which is hard to avoid, the final parameters will be very different. But we believed that these results can still reflect the part of the relationship between these two diseases. It is important to note that HFD severely damages the trabecular bone structure, including the significant reduction of BV/TV, Tb.N, Tb.Th and the significantly increasing of Tb.Sp, SMI. However, HFD seems to be less able to affect the cortical bone function of animals. The last variable is the inherent biological variation of animals. Although all of the experimental animals in this review were seemingly homogeneous, considerable biological variation could have existed among them. One study showed that mice have different sensitivities to high-fat diets. With the same feeding conditions, some mice were described as being obesity-resistant, while other was described as being obesity-prone (Choi et al., Citation2016). Furthermore, in the other study of mice on an HFD, some animals gained more weight than others in response to the same HFD feeding, so researchers could divide mice into high-gainer and low-gainer phenotypes according to weight (Griffin et al., Citation2010). In one of the included studies, the bones used for Micro-CT scan were obtained from 1139 mice from 137 full-sib families of the F34 generation of the LG/J by SM/J AI line, which originated from an intercross of LG/J female and SM/J male inbred mice. They represent a strain with high body weight and a strain with low body weight, respectively. Although this study minimizes mice obesity susceptibility, its experimental results contain less data than we need (Silva et al., Citation2019). Despite this, future studies are needed to learn this research’s methods to improve the experimental results’ accuracy. Thus, we assumed that there might be a relationship between the susceptibility of animals to HFD, although the included studies do not mention these data, and the progression of OP, which may help to explain these controversial results (Gautam et al., Citation2014; McCabe et al., Citation2019).
Moreover, HFD can also affect the expression of related cytokines and genes, which play an important role in the development of OP. A recent study performed an analysis of LDL, HDL, and TG levels on BMD and fracture, the results suggested a causal effect of LDL-C on eBMD, total body BMD and potentially on lumbar spine BMD, and proved that statins on eBMD and total body BMD are at least partly due to their LDL lowering effect (Zheng et al., Citation2020). As expected, an increase of several factors was observed in the HFD mice when compared with CD mice, such as TC, TG, VLDL, and LDL (Gautam et al., Citation2014, Citation2016, Citation2017); but another lipoprotein that can enhance the metabolism of blood lipids, HDL level was reduced in 3 papers (Gautam et al., Citation2014, Citation2016, Citation2017). Furthermore, an increase of TNF-α and PAI-1 was observed in the HFDs when compared with CDs. These inflammatory cytokines were produced directly or indirectly by adipose tissue, which may negatively interfere with the balance between bone resorption and formation (Sukumar et al., Citation2011). According to some research, leptin seems to work through two contradictory mechanisms. People with high serum leptin levels have increased bone mineral density (Barbour et al., Citation2011). However, leptin can also decrease bone formation via the central nervous system (Karsenty & Ferron, Citation2012). After summarizing the secondary outcomes of included studies, we found that whether in serum or WAT (Gautam et al., Citation2017; Jatkar et al., Citation2017; Silva et al., Citation2019), leptin levels were elevated. Furthermore, the major adipogenic transcription factors PPAR-γ and C/EBPα, which are known to be associated with the regulation of both bone mass and fat (Kawai & Rosen, Citation2010), increasing the commitment of pluripotent stem cells to adipocytes and inhibiting commitment to the osteoblast lineage, were increased in two studies (Gautam et al., Citation2014, Citation2017). Finally, aP2, a cytokine that regulates the differentiation and maturation of fat cells increased in two studies (Gautam et al., Citation2014, Citation2016).
In addition to adipocytokines, HFD can also affect bone metabolism-related factors. The results of serum OCN measured by ELISA increased in two studies (Gautam et al., Citation2014, Citation2017), and three studies showed no statistically significant differences, but when analyzed via gene expression of the bone tissue, OCN significantly decreased in HFD animals, compared with a CD (Gautam et al., Citation2014, Citation2016). This discrepancy may have resulted from variations in the methodology used in these papers. One study showed that the TRAP level increased in serum, and one study showed that it increased in bone tissue. Similarly, CTx level also showed a significant increase in HFD-animals compared with CD-animals (Gautam et al., Citation2014). The increase in these factors proves that osteoclast differentiation and proliferation are very active in HFD animals. TNF-α, increased in two studies (Gautam et al., Citation2016, Citation2017), was proposed to induce resorption in bone indirectly by affecting the production of the essential osteoclast differentiation factor, receptor activator of NF-kB ligand (RANKL), or its soluble decoy receptor, osteoprotegerin (OPG) (Boyce et al., Citation2005; Zhang et al., Citation2015). The decrease in the OPG/RANKL ratio, as we discovered in two studies (Gautam et al., Citation2014, Citation2016), may well account for an enhanced ability of preosteoblastic cells to support osteoclast development, leading to the observed marked imbalance between bone formation and resorption and inducing rapid bone loss condition (Khosla, Citation2001).
Recently, the relationship between HFD-induced overweight and OP has been widely studied. Many evidences of studies point to that the relationship between bone mass and weight were nonlinearity. A study confirmed that the inverse relationship between bone mass and fat mass, after the mechanical loading effects due to total body weight were controlled (Zhao et al., Citation2007). Although this review was conducted in animals, Panchal and Brown (Panchal & Brown, Citation2011) concluded that an HFD mimics most of the symptoms of metabolic syndrome in humans. Therefore, these findings may provide new insights into the pathogenesis of human OP.
5. Conclusion
In the present systematic review and meta-analysis, we proved that HFD unequivocally exacerbated the progression of OP compared with CD, and the main structure affected by it is cancellous bone rather than cortical bone. Future studies need larger sample sizes and more appropriate experimental animals to prove the reliability of those ambiguous results further, such as results on cortical bone and biomechanics. If the HFD-induced method established obesity animal model reliably leads to OP, it would facilitate further exploration of the discovery of dietary and pharmacological preventions and treatments for this disease.
Authors’ contributions
QQ Lian and HT Li: study conception and design, participant assessment protocol, data collection, and statistical analysis. QQ Lian and HT Li: manuscript draft preparation. FM Tian: participant assessment, data collection, and review of the manuscript. Y Gou and YP Hu: study conception and design, critical review of the manuscript. L Zhang: study conception and design. All authors have read and approved the final manuscript.
Disclosure statement
No potential conflict of interest was reported by the author(s).
Additional information
Funding
References
- Barbour, K. E., Zmuda, J. M., Boudreau, R., Strotmeyer, E. S., Horwitz, M. J., Evans, R. W., Kanaya, A. M., Harris, T. B., Bauer, D. C., & Cauley, J. A. (2011). Adipokines and the risk of fracture in older adults. Journal of Bone and Mineral Research: The Official Journal of the American Society for Bone and Mineral Research, 26(7), 1568–9. https://doi.org/10.1002/jbmr.361
- Bosello, O., Donataccio, M. P., & Cuzzolaro, M. (2016). Obesity or obesities? Controversies on the association between body mass index and premature mortality. Eating and Weight Disorders: EWD, 21(2), 165–174. https://doi.org/10.1007/s40519-016-0278-4
- Boyce, B. F., Li, P., Yao, Z., Zhang, Q., Badell, I. R., Schwarz, E. M., O’Keefe, R. J., & Xing, L. (2005). TNF-alpha and pathologic bone resorption. The Keio Journal of Medicine, 54(3), 127–131. https://doi.org/10.2302/kjm.54.127
- Cao, J. J., & Gregoire, B. R. (2016). A high-fat diet increases body weight and circulating estradiol concentrations but does not improve bone structural properties in ovariectomized mice. Nutrition Research, 36(4), 320–327. https://doi.org/10.1016/j.nutres.2015.12.008
- Choi, J. Y., McGregor, R. A., Kwon, E. Y., Kim, Y. J., Han, Y., Park, J. H. Y., Lee, K. W., Kim, S.-J., Kim, J., Yun, J. W., & Choi, M.-S. (2016). The metabolic response to a high-fat diet reveals obesity-prone and -resistant phenotypes in mice with distinct mRNA-seq transcriptome profiles. International Journal of Obesity, 40(9), 1452–1460. https://doi.org/10.1038/ijo.2016.70
- De Laet, C., Kanis, J. A., Odén, A., Johanson, H., Johnell, O., Delmas, P., Eisman, J. A., Kroger, H., Fujiwara, S., Garnero, P., McCloskey, E. V., Mellstrom, D., Melton, L. J., Meunier, P. J., Pols, H. A. P., Reeve, J., Silman, A., & Tenenhouse, A. (2005). Body mass index as a predictor of fracture risk: A meta-analysis. Osteoporosis International: A Journal Established as Result of Cooperation Between the European Foundation for Osteoporosis and the National Osteoporosis Foundation of the USA, 16(11), 1330–1338. https://doi.org/10.1007/s00198-005-1863-y
- French, S., & Robinson, T. (2003). Fats and food intake. Current Opinion in Clinical Nutrition & Metabolic Care, 6(6), 629–634. https://doi.org/10.1097/00075197-200311000-00004
- Gautam, J., Choudhary, D., Khedgikar, V., Kushwaha, P., Singh, R. S., Singh, D., Tiwari, S., & Trivedi, R. (2014). Micro-architectural changes in cancellous bone differ in female and male C57BL/6 mice with high-fat diet-induced low bone mineral density. The British Journal of Nutrition, 111(10), 1811–1821. https://doi.org/10.1017/S0007114514000051
- Gautam, J., Khedgikar, V., Choudhary, D., Kushwaha, P., Dixit, P., Singh, D., Maurya, R., & Trivedi, R. (2016). An isoflavone cladrin prevents high-fat diet-induced bone loss and inhibits the expression of adipogenic gene regulators in 3T3-L1 adipocyte. The Journal of Pharmacy and Pharmacology, 68(8), 1051–1063. https://doi.org/10.1111/jphp.12562
- Gautam, J., Khedgikar, V., Kushwaha, P., Choudhary, D., Nagar, G. K., Dev, K., Dixit, P., Singh, D., Maurya, R., & Trivedi, R. (2017). Formononetin, an isoflavone, activates AMP-activated protein kinase/β-catenin signalling to inhibit adipogenesis and rescues C57BL/6 mice from high-fat diet-induced obesity and bone loss. The British Journal of Nutrition, 117(5), 645–661. https://doi.org/10.1017/S0007114517000149
- Griffin, T. M., Fermor, B., Huebner, J. L., Kraus, V. B., Rodriguiz, R. M., Wetsel, W. C., Cao, L., Setton, L. A., & Guilak, F. (2010). Diet-induced obesity differentially regulates behavioral, biomechanical, and molecular risk factors for osteoarthritis in mice. Arthritis Research & Therapy, 12(4), R130. https://doi.org/10.1186/ar3068
- Hebert, J. R., Patterson, R. E., Gorfine, M., Ebbeling, C. B., St Jeor, S. T., & Chlebowski, R. T. (2003). Differences between estimated caloric requirements and self-reported caloric intake in the women’s health initiative. Annals of Epidemiology, 13(9), 629–637. https://doi.org/10.1016/S1047-2797(03)00051-6
- Holmes, A., Coppey, L. J., Davidson, E. P., & Yorek, M. A. (2015). Rat models of diet-induced obesity and high fat/low dose streptozotocin type 2 diabetes: Effect of reversal of high fat diet compared to treatment with enalapril or menhaden oil on glucose utilization and neuropathic endpoints. Journal of Diabetes Research, 2015, 307285. https://doi.org/10.1155/2015/307285
- Hooijmans, C. R., Rovers, M. M., de Vries RB, Leenaars, M., Ritskes-Hoitinga, M., & Langendam, M. W. (2014). SYRCLE’s risk of bias tool for animal studies. BMC Medical Research Methodology, 14(1), 43. https://doi.org/10.1186/1471-2288-14-43
- Jatkar, A., Kurland, I. J., & Judex, S. (2017). Diets high in fat or fructose differentially modulate bone health and lipid metabolism. Calcified Tissue International, 100(1), 20–28. https://doi.org/10.1007/s00223-016-0205-8
- Kanis, J. A., Johnell, O., De Laet, C., Johansson, H., Oden, A., Delmas, P., Eisman, J., Fujiwara, S., Garnero, P., Kroger, H., McCloskey, E. V., Mellstrom, D., Melton, L. J., Pols, H., Reeve, J., Silman, A., & Tenenhouse, A. (2004). A meta-analysis of previous fracture and subsequent fracture risk. Bone, 35(2), 375–382. https://doi.org/10.1016/j.bone.2004.03.024
- Kanis, J. A., Melton, L. J., 3rd, Christiansen, C., Johnston, C. C., & Khaltaev, N. (1994). The diagnosis of osteoporosis. Journal of Bone and Mineral Research: The Official Journal of the American Society for Bone and Mineral Research, 9(8), 1137–1141. https://doi.org/10.1002/jbmr.5650090802
- Karsenty, G., & Ferron, M. (2012). The contribution of bone to whole-organism physiology. Nature, 481(7381), 314–320. https://doi.org/10.1038/nature10763
- Kawai, M., & Rosen, C. J. (2010). PPARγ: A circadian transcription factor in adipogenesis and osteogenesis. Nature Reviews Endocrinology, 6(11), 629–636. https://doi.org/10.1038/nrendo.2010.155
- Kelly, N. H., Schimenti, J. C., Ross, F. P., & van der Meulen MC. (2016). Transcriptional profiling of cortical versus cancellous bone from mechanically-loaded murine tibiae reveals differential gene expression. Bone, 86, 22–29. https://doi.org/10.1016/j.bone.2016.02.007
- Khosla, S. (2001). Minireview: The OPG/RANKL/RANK system. Endocrinology, 142(12), 5050–5055. https://doi.org/10.1210/endo.142.12.8536
- Kwon, M., Kim, G. W., Yim, H. W., Paek, Y. J., & Lee, K. S. (2015). Association between dietary fat intake and bone mineral density in Korean adults: Data from Korea national health and nutrition examination survey IV (2008 ~ 2009). Osteoporosis International, 26(3), 969–976. https://doi.org/10.1007/s00198-014-2977-x
- Liberati, A., Altman, D. G., Tetzlaff, J., Mulrow, C., Gøtzsche, P. C., Ioannidis, J. P., Clarke, M., Devereaux, P. J., Kleijnen, J., & Moher, D. (2009). The PRISMA statement for reporting systematic reviews and meta-analyses of studies that evaluate healthcare interventions: Explanation and elaboration. BMJ, 339(jul21 1), b2700. https://doi.org/10.1136/bmj.b2700
- Ma, H., Turpeinen, T., Silvennoinen, M., Torvinen, S., Rinnankoski-Tuikka, R., Kainulainen, H., Timonen, J., Kujala, U. M., Rahkila, P., & Suominen, H. (2011). Effects of diet-induced obesity and voluntary wheel running on the microstructure of the murine distal femur. Nutrition and Metabolism, 8(1), 1. https://doi.org/10.1186/1743-7075-8-1
- McCabe, L. R., Irwin, R., Tekalur, A., Evans, C., Schepper, J. D., Parameswaran, N., & Ciancio, M. (2019). Exercise prevents high fat diet-induced bone loss, marrow adiposity and dysbiosis in male mice. Bone, 118, 20–31. https://doi.org/10.1016/j.bone.2018.03.024
- Montalvany-Antonucci, C. C., Zicker, M. C., Ferreira, A. V. M., Macari, S., Ramos-Junior, E. S., Gomez, R. S., Pereira, T. S. F., Madeira, M. F. M., Fukada, S. Y., Andrade, I., & Silva, T. A. (2018). High-fat diet disrupts bone remodeling by inducing local and systemic alterations. The Journal of Nutritional Biochemistry, 59, 93–103. https://doi.org/10.1016/j.jnutbio.2018.06.006
- Panchal, S. K., & Brown, L. (2011). Rodent models for metabolic syndrome research. Journal of Biomedicine & Biotechnology, 2011, 351982. https://doi.org/10.1155/2011/351982
- Poppitt, S. D., Swann, D., Black, A. E., & Prentice, A. M. (1998). Assessment of selective under-reporting of food intake by both obese and non-obese women in a metabolic facility. International Journal of Obesity and Related Metabolic Disorders: Journal of the International Association for the Study of Obesity, 22(4), 303–311. https://doi.org/10.1038/sj.ijo.0800584
- Qiao, J., Wu, Y., & Ren, Y. (2021, 15). The impact of a high fat diet on bones: Potential mechanisms. Food & Function, 12(3), 963–975. https://doi.org/10.1039/d0fo02664f
- Schrauwen, P., & Westerterp, K. R. (2000). The role of high-fat diets and physical activity in the regulation of body weight. The British Journal of Nutrition, 84(4), 417–427. https://doi.org/10.1017/s0007114500001720
- Silva, M. J., Eekhoff, J. D., Patel, T., Kenney-Hunt, J. P., Brodt, M. D., Steger-May, K., Scheller, E. L., & Cheverud, J. M. (2019). Effects of high-fat diet and body mass on bone morphology and mechanical properties in 1100 advanced intercross mice. Journal of Bone and Mineral Research: The Official Journal of the American Society for Bone and Mineral Research, 34(4), 711–725. https://doi.org/10.1002/jbmr.3648
- Sontam, D. M., Vickers, M. H., Firth, E. C., & O’Sullivan, J. M. (2017, July 7). A memory of early life physical activity is retained in bone marrow of male rats fed a high-fat diet. Frontiers in Physiology, 8, 476. https://doi.org/10.3389/fphys.2017.00476
- Sukumar, D., Schlussel, Y., Riedt, C. S., Gordon, C., Stahl, T., & Shapses, S. A. (2011). Obesity alters cortical and trabecular bone density and geometry in women. Osteoporosis International: A Journal Established as Result of Cooperation Between the European Foundation for Osteoporosis and the National Osteoporosis Foundation of the USA, 22(2), 635–645. https://doi.org/10.1007/s00198-010-1305-3
- Yoneda, T., Tomofuji, T., Kunitomo, M., Ekuni, D., Irie, K., Azuma, T., Machida, T., Miyai, H., Fujimori, K., & Morita, M. (2017, 13). Preventive effects of drinking hydrogen-rich water on gingival oxidative stress and alveolar bone resorption in rats fed a high-fat diet. Nutrients, 9(1), 64. https://doi.org/10.3390/nu9010064
- Young, G. S., & Kirkland, J. B. (2007). Rat models of caloric intake and activity: Relationships to animal physiology and human health. Applied Physiology, Nutrition, and Metabolism, 32(2), 161–176. https://doi.org/10.1139/h06-082
- Zhang, K., Wang, C., Chen, Y., Ji, X., Chen, X., Tian, L., & Yu, X. (2015). Preservation of high-fat diet-induced femoral trabecular bone loss through genetic target of TNF-α. Endocrine, 50(1), 239–249. https://doi.org/10.1007/s12020-015-0554-5
- Zhao, L. J., Liu, Y. J., Liu, P. Y., Hamilton, J., Recker, R. R., & Deng, H. W. (2007). Relationship of obesity with osteoporosis. The Journal of Clinical Endocrinology and Metabolism, 92(5), 1640–1646. https://doi.org/10.1210/jc.2006-0572
- Zheng, J., Brion, M. J., Kemp, J. P., Warrington, N. M., Borges, M.-C., Hemani, G., Richardson, T. G., Rasheed, H., Qiao, Z., Haycock, P., Ala‐Korpela, M., Davey Smith, G., Tobias, J. H., & Evans, D. M. (2020, March 2). The effect of plasma lipids and lipid-lowering interventions on bone mineral density: A Mendelian randomization study. Journal of Bone and Mineral Research, 35(7), 1224–1235. https://doi.org/10.1002/jbmr.3989